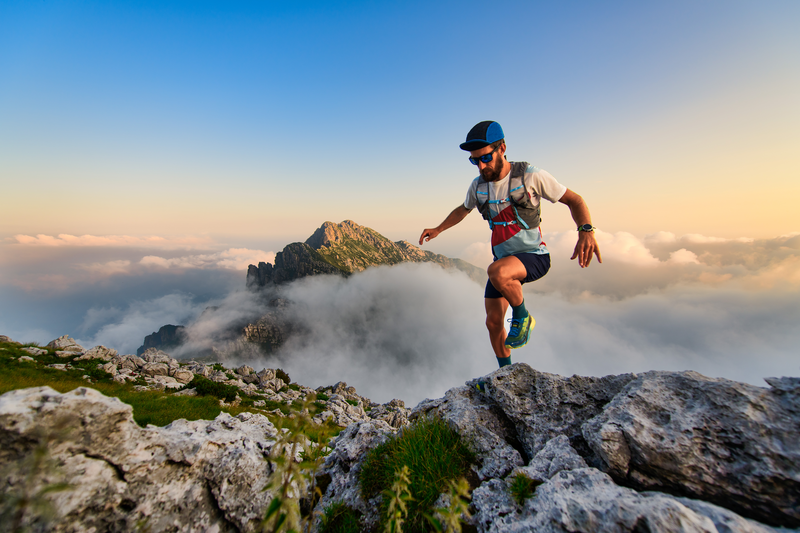
95% of researchers rate our articles as excellent or good
Learn more about the work of our research integrity team to safeguard the quality of each article we publish.
Find out more
ORIGINAL RESEARCH article
Front. Sustain. , 13 June 2022
Sec. Quantitative Sustainability Assessment
Volume 3 - 2022 | https://doi.org/10.3389/frsus.2022.900468
This article is part of the Research Topic The Renewable Power Transition: Spotlighting SDG7's Impact on other Sustainable Development Goals View all 5 articles
Renewable energy development is rapidly increasing across the world, providing affordable and more environmentally sustainable energy to many populations. However, renewable energy, such as solar and wind, can have large land footprints through conversion and modification of natural habitats. One of the more intact habitats on Earth is the desert biome, which contains large areas of roadless regions and, in some places, high biodiversity. Since they are often windy and sunny, desert regions also tend to be rich in renewable energy resources. Using publicly available geospatial data, we calculated that the highest resource areas for wind overlap with 79% of roadless areas and the highest resource areas for solar overlap 28% of roadless areas, globally. High resource wind and solar areas overlap high plant diversity regions by 56 and 79%, respectively, but because high plant diversity desert areas are localized, these overlap areas represent a tiny proportion of the total potentially economically valuable wind and solar regions. These results suggest that desert regions remaining ecologically intact are at risk from renewable energy development. However, strategic siting in abundant, lower quality desert areas might mitigate this issue, especially when areas already impacted by human activity are available. Selected regions presented in detail demonstrate the risks to these habitats and strategies to minimize ecosystem damage. We urge governments and industries to consider placement of wind and solar energy projects that minimize environmental impacts to lands that, up to this point, remain relatively untransformed by human activities.
The global energy industry is changing rapidly, with new production of renewable energy sources growing at a faster rate than traditional fossil fuels (International Energy Agency, 2021). Some fossil fuels, such as coal, appear to be in terminal decline (Mendelevitch et al., 2019), and efforts are underway in some countries to reduce oil and gas use as a way to address global climate change (Le Quéré et al., 2019). The most prominent renewable sources being developed are wind and solar, whose production has grown exponentially in the last decade (Moriarty and Honnery, 2019). While solar and wind power have limited carbon emissions, they still can have important impacts on land-use, fragmentation, and ecosystem services provision by natural habitats (McDonald et al., 2009; Hernandez et al., 2015; Grodsky and Hernandez, 2020; Rehbein et al., 2020). In some cases, solar and wind power can have comparable levels on land-use impact as some fossil fuel generation, at least in the short-term (Jordaan et al., 2021). Finding ways to mitigate this impact on natural ecosystems will be of prime importance as these renewable sources continue to expand.
Deserts cover about 13% of the Earth's surface (Costanza et al., 2014) and remain some of the least developed regions on the planet (McClung et al., 2019), partly because they are generally unsuitable for intensive agriculture, the largest historical driver of land use change (Kanianska, 2016) until recent times (Trainor et al., 2016). While deserts may be low productivity environments, they can contain high species diversity and have high rates of endemism (Johnston, 1977; Safriel et al., 2005). Some deserts represent globally important biodiversity hotspots (e.g., Karoo of South Africa and the Sonoran/Chihuahuan regions of the U.S. and Mexico; Myers et al., 2000) and support vibrant human cultures while providing numerous local and global ecosystem services (Mucina et al., 2006; Durant et al., 2012, 2014; Dimmitt et al., 2015; Taylor et al., 2017; McClung et al., 2019). Because of the generally low human population density, deserts remain some of the least fragmented habitats, allowing landscape level ecological interactions to continue in a time when most other habitats are highly fragmented and modified (Jacobson et al., 2019).
While deserts remain mostly intact, new threats are emerging that could drastically alter these lands (Hernandez et al., 2015). The world is beginning an energy transition from fossil fuels to renewable sources (Cameron et al., 2017; Ram et al., 2020; Luderer et al., 2022), with wind and solar being two important and rapidly expanding methods, in some cases already having impacts on high biodiversity areas (Rehbein et al., 2020). Deserts are, by their nature, generally sunny and windy, so it is not surprising that much solar and wind development has occurred (and continues to occur) in desert ecosystems (Copeland et al., 2011; McClung et al., 2019). For example, the American Southwest arid lands have become major suppliers of power to the U.S. electrical grid in recent years (Parker et al., 2018). In some cases, these renewable energy developments have occurred in areas that previously lacked significant industrial activity (Parker et al., 2018; McClung et al., 2019).
While deserts have ubiquitous solar and wind potential, it is unlikely that these resources are dispersed uniformly in the environment (McClung et al., 2019). The same pattern holds true for biological and ecological resources. Biodiversity and unmodified desert habitat are likely to be aggregated in relatively smaller areas compared to the vast coverage of deserts (Durant et al., 2012), similar to patterns seen in other major biomes (Myers et al., 2000). If emerging renewable energy development could be sited in a way that avoids the most valuable desert regions, future energy needs could be met while minimizing losses to biological, ecological, and cultural resources. The goal of this study was to identify the most intact and biologically valuable desert environments globally by examining plant biodiversity and roadless patterns. We then sought to identify the best wind and solar resources in deserts to determine where they overlap the most ecologically valuable desert regions. Our hope is that by identifying these desert areas of high risk, recommendations could be made on where to steer renewable energy development to minimize impacts on biodiversity and unfragmented habitats.
To answer the question of where the highest quality desert habitat remains and its risk for future development, we first had to define our quality and risk factors. We used two conservation categories to identify high quality desert: roadless areas and vascular plant diversity. Our justification for using roadless areas, defined as all lands at least 1 km from a road (Ibisch et al., 2016), was that these areas are relatively unfragmented by human activities that tend to degrade environments. While roadless is not an exact measure of environmental integrity, roads are one of the most destructive human activities and are known to degrade biodiversity, increase invasive species, and provide easy access for humans to further modify the environment (Selva et al., 2015). Our justification for using vascular plant diversity begins with the fact that global biodiversity data on fine scales are not generally available for most taxa, including taxa with high biodiversity (e.g., arthropods). However, vascular plant diversity is, in general, strongly correlated with overall biodiversity in terrestrial systems (Brunbjerg et al., 2018), and so we used vascular plant species richness as an indicator of overall biodiversity.
For future risks, we assumed that wind and solar are the main energy systems that could rapidly expand into desert regions. Most traditional energy sources (e.g., oil and gas, hydropower) in desert areas are presumably already in production and have their associated land use impacts in place. We assumed other sources of energy besides wind and solar (e.g., geothermal) were either too small a scale or localized to be of significant global impact on these environments. Recent trends in wind and solar deployment support this assumption (McClung et al., 2019).
We identified desert areas using ecoregions as defined by Dinerstein et al. (2017). We excluded polar deserts (e.g., Antarctica) from our analysis because presumably they are too distant from most population centers to be cost effective renewable energy production areas. Using ArcGIS Desktop (ESRI, 2020), we clipped layers for potential wind resources (Technical University of Denmark, 2021), potential solar resources (Solargis, 2021), roadless areas (Ibisch et al., 2016), and vascular plant diversity (Kier et al., 2005) to our desert boundaries. The wind and solar resources layers came from the Global Wind Atlas 3.0 and Solar Wind Atlas 2.0, respectively. These free, web-based applications are developed and owned by the Technical University of Denmark (wind) and Solargis (solar) and developed utilizing data provided by Vortex (wind) and Solargis (solar), with funding provided by the Energy Sector Management Assistance Program.
We identified areas of economically viable wind and solar resources by using the extract by mask tool in ArcMap to create raster datasets with values above 5.8 m/s (at 100-m) for wind (U.S. Department of Energy, 2008) and 6.5 kWh/m2 (DNI) for solar (National Renewable Energy Laboratory, 2012). We then used the extract by mask tool once again to find the regions of these energy raster datasets that overlapped with desert roadless areas and desert areas in four categories of plant diversity. These four categories represented 0–25, 25–50, 50–75, and 75–100% of the total number of plant species found in deserts, which ranged from 0 to 6,300 plant species. Once these energy raster datasets were extracted by conservation category (i.e., roadless areas or four categories of plant diversity), their values were converted to integers so that we could use the zonal histogram tool to calculate the number of cells for each conservation category on each continent. This number of cells was then multiplied by the cell area (250 m2) and summed to get total areas for each conservation category on each continent. We then calculated the proportion of total global desert area and the proportion of the total global conservation category occupied by each energy type on each continent by dividing the area for each of these categories by the total global area of deserts and the total global area of each conservation category, respectively.
As expected, many desert regions have abundant wind and solar resources (Figures 1A, 2A). Interestingly, the southern hemisphere deserts tend to have higher solar potential while the northern hemisphere deserts in Africa and Asia have the windiest regions. Perhaps surprisingly, the vast Sahara Desert has more high wind than high solar resource areas.
Figure 1. Maps showing (A) the world's deserts and overlap with economically valuable solar resources, (B) detail for North American deserts, roadless areas, and solar resources, and (C) detail for southern African deserts with economically valuable solar resources and with the highly diverse Karoo ecoregion outlined. Red-yellow shaded areas represent high solar resource levels in all panels and as described in the legend for (A).
Figure 2. Maps showing (A) the world's deserts and overlap with economically valuable wind resources, (B) for Middle Eastern deserts, roadless areas, and wind resources, and (C) detail for southern African deserts with economically valuable wind resources and with the highly diverse Karoo ecoregion outlined. Blue-shaded areas represent high wind resource levels in all panels and as described in the legend for (A).
Most of the desert areas of the world remain roadless (defined as lands more than 1 km from a road, Ibisch et al., 2016; Table 1) and, therefore, relatively unfragmented. While deserts are sometimes known for their high biodiversity, only a small proportion of desert areas contain this high diversity. In total, only 0.4% of desert areas occupy the highest 75% of percent plant species richness while almost 90% of desert lands contain the lowest 25% of plant diversity (Table 1). Therefore, plant species richness is highly concentrated in small regions of the biome. Even the top 50% areas of plant diversity are limited to the Karoo Desert of southern Africa, the Chihuahuan Desert of North America, and small isolated regions of East Africa, the Middle East, and South Asia. More than three-quarters of the roadless areas are regions that have the economically valuable wind resources while about one-quarter of the roadless areas contain regions of high solar potential (Table 2). However, almost all the best wind and solar areas fall in the low plant richness areas, which is not surprising considering the proportional dominance of the lowest 25% of richness areas (Table 2). Nonetheless, the highest 75% of plant diversity area (all in the Karoo Desert) has 56 and 79% of its area located in high resource wind and solar regions, respectively. While there is much overlap between high quality desert areas (both roadless and high diversity) and high renewable resource regions, there are also vast areas of lower quality desert available that have high solar and wind potential (Figures 1B,C, 2B,C).
Table 1. Total area (km2) and proportion of deserts that fall into the two methods of assessing conservation value: roadless areas and vascular plant diversity categories.
Table 2. Total area, proportion of total global desert area, and proportion of total desert conservation category area that contain economically viable wind and solar resources for each desert conservation category.
There is continental variation in desert resources and potential impacts, with Africa and Asia having most roadless land at risk from wind development (Supplementary Table 1), representing about two-thirds of such areas. Australia and Africa have the greatest amount of solar potential areas that overlap with roadless areas. For higher plant diversity areas (>50% of species richness), most of which are found in Africa, Asia, and North America, no continent stands out as at disproportional risk from either wind or solar (Figures 1, 2, Supplementary Table 1) except for the >75% plant diversity region of southern Africa which is almost entirely overlapping with high solar and/or wind resources (Figures 1C, 2C).
The specific regions we present in detail show the challenges and possibilities of wind and solar development. First, the deserts of southern Africa contain the highest diversity desert, but the high diversity region is mostly limited to the Karoo ecoregion, while other areas of the region have much lower diversity (Figure 1C). If the Karoo desert can be avoided, there are ample wind and solar resources elsewhere that could supply the regional human population with clean energy without impacting this biodiversity hotspot (Figures 1C, 2C). The sunniest portions of these deserts are actually outside the Karoo (mostly north and west of the biodiversity hotspot), so it would probably be most cost effective to site solar arrays outside of the most ecologically valuable areas.
Similarly, the North American Deserts (Chihuahuan, Sonoran, Great Basin, and Mojave) are heavily fragmented in the northeastern and far west regions, while virtually all the areas south of the U.S. border in Mexico and to a lesser extent, northwestern parts of the U.S. area remain intact. Concentrating wind and solar development in currently developed areas could spare the most ecologically intact regions (Figure 1A).
The Middle Eastern desert is another region with abundant wind resources overlapping large areas of unfragmented desert. In general, fragmentation is limited to lands near cities and oil producing regions, which also tend to be some of the best wind resource areas (Figure 2B). In particular, Kuwait, Qatar, eastern Iraq, and coastal Saudi Arabia (Persian Gulf side) have significant fragmentation but could also support economically viable wind production.
Our analysis shows that deserts contain a large amount of unfragmented land and that a large proportion of it could be at risk for alternative energy development. The high biodiversity desert areas are very small but have a large amount of overlap with high resource wind and solar energy regions. Fortunately, the overlap areas of high wind and solar resources with high biodiversity represent only a small portion of good potential renewable energy resources. Therefore, preventing energy development impacts on the most biological valuable regions will be relatively simple and practical compared to preventing impacts to the larger (gross and proportional) roadless areas. Nevertheless, we urge governments, energy industries, and private conservation groups to utilize our analysis to plan future alternative energy development. It seems almost certain that solar and wind energy production will continue to grow into the near and midterm future (Moriarty and Honnery, 2019), a process that is probably necessary to meet the mid-twenty-first century climate goals set forth by the U.N. to prevent more than 1.5°C warming (Fawzy et al., 2020). So, the question is probably not, will it happen, but where will it happen?
A good example from our analysis is the desert regions of Africa, which are largely roadless and contain some of the highest plant richness areas of deserts in the world (southern Africa) but could easily see rapid renewable energy development. To meet human development needs, the African continent generally needs a massive upgrade in energy production (Aliyu et al., 2018; Ouedraogo, 2019). North Africa has also been discussed as a likely place to generate renewable energy to provide parts of Europe and help that region decarbonize (Trieb et al., 2012). That puts the African deserts, which up until now have been somewhat immune to intensive development, at great future risk. Nonetheless, there are considerable areas of African deserts that are already impacted by human activity and/or relatively low diversity areas that could be utilized without damaging more ecologically sensitive regions. Furthermore, small areas of high-quality renewable energy production can supply a large piece of the vast energy needs of region (Trieb et al., 2012), supporting the argument that concentrating development in the relatively small areas already heavily impacted would be practical.
Even though quality desert habitat is at risk from increasing energy development, there are likely ways to mitigate the damage by using existing infrastructure. For instance, the U.S. portion of the Chihuahuan Desert is about one-third heavily impacted by development (energy, urban, and agriculture) and about two-thirds ecologically intact (McClung and Moran, 2018; McClung et al., 2019). If the heavily developed portion, mostly now impacted by the oil and gas industry, was utilized for wind and solar, wider impacts to the ecosystem could be minimized. Considering that much of the oil and gas infrastructure (e.g., roads and powerlines) is also useful for other energy types, it could also be cost effective to utilize currently developed areas while sparing higher quality habitat. This recommendation would be a way to limit further ecosystem services loss for the region (Jordaan et al., 2021) and maintain an ecologically well-functioning landscape. Furthermore, restoration of lands on defunct infrastructure (e.g., inactive oil and gas wells) in select regions could be an effective mitigation strategy to further strengthen connectivity on high quality regions while energy development continues or expands in currently heavily impacted areas (McClung and Moran, 2018; Haden Chomphosy et al., 2021; Jordaan et al., 2021).
In the North American example (Figure 1B), there is a stark difference between the U.S. side of the border that has intense development and the Mexican side of the border which is largely intact. While encouraging development on the U.S. would limit further damage to this desert region, it also illustrates potential inequity between communities on both sides of the border who have a right to clean power. Creative development plans, such as cost and power sharing agreements between the U.S. and Mexico should be considered so that clean energy and environmental protection benefits are available to all people of the ecoregion.
The Middle East is another intriguing example. Because of the abundant oil and gas deposits, the region produces, either directly or indirectly, and large proportion of the world's greenhouse gases. Some authors have suggested that this region could produce and export vast quantities of renewable resources (Aslani et al., 2012), and with the strong history or energy production, would have the infrastructure and knowledge to make the transition profitably. Our analysis shows that the existing population centers and petroleum producing landscapes are also ideal for renewable energy production. This strategy would allow future energy development to reduce the region's carbon footprint while sparing many of the unfragmented deserts that characterize the area.
While this paper provides a global view of desert energy resources and risks, our examples above demonstrate the need for region-specific plans. Each desert area of the world contains unique ecological, biological, and cultural interactions that make generalized recommendations difficult. Detailed local plans, such as the ones that have been produced in some regions (e.g., southern California, U.S. Bureau of Land Management, 2016), will need to be developed in other desert landscapes targeted for development if energy needs of the world (i.e., “affordable, reliable, sustainable, and modern energy for all,” United Nations, 2015) can be met while protecting unique irreplaceable resources.
Deserts remain one of more intact biomes on Earth, and while often considered as less desirable, low productivity landscapes, in reality they contain myriad unique ecosystems. While the impacts of grazing and traditional energy development (i.e., oil and gas) have impacted some areas (Moran et al., 2017; McClung et al., 2019) and defaunation remains an issue (Durant et al., 2014), deserts have largely been spared the drastic physical transformation of other habitats (e.g., conversion of grasslands to intensive agricultural landscapes; Bardgett et al., 2021). With the rapidly expanding renewable energy industry focusing on solar and wind, two resources that are abundant in desert regions, the status quo is not guaranteed. With careful planning though, our analysis shows that it would be possible to develop renewable energy resources that will help address climate change but, at the same time, not cause irreparable harm to these unique environments.
There is no doubt that energy needs will increase throughout much of the world in the future and that non-carbon energy sources, both for new energy and to replace existing carbon intensive energy, will be necessary to prevent catastrophic climate change (United Nations, 2015). These changes will put many ecologically valuable lands, such as deserts, at risk, including those that have, to date, been spared the most intense development patterns. Our analysis suggests that it is possible to provide energy needs to the world and perhaps avoid major disruptions of remaining intact and highly functional ecosystems. It will take planning, but it is planning that needs to happen now while we are in the early phase of renewable energy adoption across the world.
The original contributions presented in the study are included in the article/Supplementary Material, further inquiries can be directed to the corresponding author.
Both authors listed have made a substantial, direct, and intellectual contribution to the work and approved it for publication.
The authors declare that the research was conducted in the absence of any commercial or financial relationships that could be construed as a potential conflict of interest.
The handling editor SJ declared a past co-authorship with the authors.
All claims expressed in this article are solely those of the authors and do not necessarily represent those of their affiliated organizations, or those of the publisher, the editors and the reviewers. Any product that may be evaluated in this article, or claim that may be made by its manufacturer, is not guaranteed or endorsed by the publisher.
Many thank to S. Jordaan and R. Hernandez for giving us the opportunity to contribute to this special issue. Two reviewers provided valuable feedback to earlier versions of the manuscript.
The Supplementary Material for this article can be found online at: https://www.frontiersin.org/articles/10.3389/frsus.2022.900468/full#supplementary-material
Aliyu, A. K., Modu, B., and Tan, C. W. (2018). A review of renewable energy development in Africa: a focus in South Africa, Egypt, and Nigeria. Renew. Sust. Energ. Rev. 81, 2502–2518. doi: 10.1016/j.rser.2017.06.055
Aslani, A., Naaranoja, M., and Zakeri, B. (2012). The prime criteria for private sector participation in renewable energy investment in the Middle East (case study: Iran). Renew. Sust. Energ. Rev. 16, 1977–1987. doi: 10.1016/j.rser.2011.12.015
Bardgett, R. D., Bullock, J. M., Lavorel, S., Manning, P., Schaffner, U., Ostle, N., et al. (2021). Combatting global grassland degradation. Nat. Rev. Earth Environ. 2, 720–735. doi: 10.1038/s43017-021-00207-2
Brunbjerg, A. K., Bruun, H. H., Dalby, L., Fløjgaard, C., Frøslev, T. G., Høye, T. T., et al. (2018). Vascular plant species richness and bioindication predict multi-taxon species richness. Methods Ecol. Evol. 9, 2372–2382. doi: 10.1111/2041-210X.13087
Cameron, D. R., Crane, L., Parker, S. S., and Randall, J. M. (2017). “Solar energy development and regional conservation planning,” in Energy Sprawl Solutions: Balancing Global Development and Conservation, eds J. M. Kiesecker and D. E. Naugle (Washington, DC: Island Press), 66–75.
Copeland, H. E., Pocewicz, A., and Kiesecker, J. M. (2011). “Geography of energy development in western North America: potential impacts on terrestrial ecosystems,” in Energy Development and Wildlife Conservation in Western North America, ed D. E. Naugle (Washington, DC: Island Press), 7–22.
Costanza, R., de Groot, R., Sutton, P., Van der Ploeg, S., Anderson, S. J., Kubiszewski, I., et al. (2014). Changes in the global value of ecosystem services. Global Environ. Chang. 26, 152–158. doi: 10.1016/j.gloenvcha.2014.04.002
Dimmitt, M. A., Comus, P. W., Phillips, S. J., and Brewer, L. M. (2015). A Natural History of the Sonoran Desert. Berkeley, CA: University of California Press.
Dinerstein, E., Olson, D., Joshi, A., Vynne, C., Burgess, N. D., Wikramanayake, E., et al. (2017). An ecoregion-based approach to protecting half the terrestrial realm. BioScience 67, 534–545. doi: 10.1093/biosci/bix014
Durant, S. M., Pettorelli, N., Bashir, S., Woodroffe, R., Wacher, T., De Ornellas, P., et al. (2012). Forgotten biodiversity in desert ecosystems. Science 336, 1379–1380. doi: 10.1126/science.336.6087.1379
Durant, S. M., Wacher, T., Bashir, S., Woodroffe, R. D., De Ornellas, P., Ransom, C., et al. (2014). Fiddling in biodiversity hotspots while deserts burn? Collapse of the Sahara's megafauna. Divers. Distrib. 20, 114–122. doi: 10.1111/ddi.12157
Fawzy, S., Osman, A. I., Doran, J., and Rooney, D. W. (2020). Strategies for mitigation of climate change: a review. Environ. Chem. Lett. 18, 2069–2094. doi: 10.1007/s10311-020-01059-w
Grodsky, S. M., and Hernandez, R. R. (2020). Reduced ecosystem services of desert plants from ground-mounted solar energy development. Nat. Sustain. 3, 1036–1043. doi: 10.1038/s41893-020-0574-x
Haden Chomphosy, W., Varriano, S., Lefler, L. H., Nallur, V., McClung, M. R., and Moran, M. D. (2021). Ecosystem services benefits from the restoration of non-producing US oil and gas lands. Nat. Sustain. 4, 547–554. doi: 10.1038/s41893-021-00689-4
Hernandez, R. R., Hoffacker, M. K., Murphy-Mariscal, M. L., Wu, G. C., and Allen, M. F. (2015). Solar energy development impacts on land cover change and protected areas. Proc. Natl. Acad. Sci. U.S.A. 112, 13579–13584. doi: 10.1073/pnas.1517656112
Ibisch, P. L., Hoffmann, M. T., Kreft, S., Pe'er, G., Kati, V., Biber-Freudenberger, L., et al. (2016). A global map of roadless areas and their conservation status. Science 354, 1423–1427. doi: 10.1126/science.aaf7166
International Energy Agency (2021). Renewables 2021. Paris: International Energy Agency. Available online at: https://iea.blob.core.windows.net/assets/5ae32253-7409-4f9a-a91d-1493ffb9777a/Renewables2021-Analysisandforecastto2026.pdf (accessed December 15, 2021).
Jacobson, A. P., Riggio, J., Tait, A. M., and Baillie, J. (2019). Global areas of low human impact (‘Low Impact Areas’) and fragmentation of the natural world. Sci. Rep. 9, 14179. doi: 10.1038/s41598-019-50558-6
Johnston, M. C. (1977). “Brief resume of botanical, including vegetational, features of the Chihuahuan Desert region with special emphasis on their uniqueness,” in Transactions of the Symposium on the Biological Resources of the Chihuahuan Desert Region, United States and Mexico, eds R. H. Wauer and D. H. Riskind (Washington, DC: National Park Service), 335–359.
Jordaan, S. M., Lee, J., McClung, M. R., and Moran, M. D. (2021). Quantifying the ecosystem services values of electricity generation in the US Chihuahuan Desert: a life cycle perspective. J. Indust. Ecol. 25, 1089–1101. doi: 10.1111/jiec.13111
Kanianska, R. (2016). “Agriculture and its impact on land-use, environment, and ecosystem services,” in Landscape Ecology-The Influences of Land Use and Anthropogenic Impacts of Landscape Creation, ed A. Almusaed (London, UK: IntechOpen Limited), 1–26.
Kier, G., Mutke, J., Dinerstein, E., Ricketts, T. H., Ku, W., Kreft, H., et al. (2005). Global patterns of plant diversity and floristic knowledge. J. Biogeog. 32, 1107–1116. doi: 10.1111/j.1365-2699.2005.01272.x
Le Quéré, C., Korsbakken, J. I., Wilson, C., Tosun, J., Andrew, R., Andres, R. J., et al. (2019). Drivers of declining CO2 emissions in 18 developed economies. Nat. Clim. Chang. 9, 213–217. doi: 10.1038/s41558-019-0419-7
Luderer, G., Madeddu, S., Merfort, L., Ueckerdt, F., Pehl, M., Pietzcker, R., et al. (2022). Impact of declining renewable energy costs on electrification in low-emission scenarios. Nat. Energ. 7, 32–42. doi: 10.1038/s41560-021-00937-z
McClung, M. R., and Moran, M. D. (2018). Understanding and mitigating impacts of unconventional oil and gas development on land-use and ecosystem services in the US. Curr. Opin. Environ. Sci. Health. 3, 19–26. doi: 10.1016/j.coesh.2018.03.002
McClung, M. R., Taylor, N. T., Zamzow, B. K., Stone, E. T., Abad, H., and Moran, M. D. (2019). The threat of energy diversification to a bioregion: a landscape-level analysis of current and future impacts on the US Chihuahuan desert. Reg. Environ. Chang. 19, 1949–1962. doi: 10.1007/s10113-019-01525-4
McDonald, R. I., Fargione, J., Kiesecker, J., Miller, W. M., and Powell, J. (2009). Energy sprawl or energy efficiency: climate policy impacts on natural habitat for the United States of America. PLoS ONE 4, e6802. doi: 10.1371/journal.pone.0006802
Mendelevitch, R., Hauenstein, C., and Holz, F. (2019). The death spiral of coal in the US: will changes in US Policy turn the tide? Clim. Policy 19, 1310–1324. doi: 10.1080/14693062.2019.1641462
Moran, M. D., Taylor, N. T., Mullins, T. F., Sardar, S. S., and McClung, M. R. (2017). Land-use and ecosystem services costs of unconventional US oil and gas development. Front. Ecol. Environ. 15, 237–242. doi: 10.1002/fee.1492
Moriarty, P., and Honnery, D. (2019). “Global renewable energy resources and use in 2050,” in Managing Global Warming Academic Press, ed T. Letcher (London: Elsevier), 221–235.
Mucina, L., Jürgens, N., Le Roux, A., Rutherford, M. C., Schmiedel, U., Esler, K.J., et al. (2006). Succulent karoo biome. The vegetation of South Africa, Lesotho and Swaziland. Strelitzia 19, 221–299.
Myers, N., Mittermeier, R. A., Mittermeier, C. G., Da Fonseca, G. A., and Kent, J. (2000). Biodiversity hotspots for conservation priorities. Nature 403, 853–858. doi: 10.1038/35002501
National Renewable Energy Laboratory (2012). Concentrated Solar Resources of the United States. Washington, DC: U.S. Department of Energy.
Ouedraogo, N. S. (2019). Opportunities, barriers and issues with renewable energy development in Africa: a comprehensible review. Curr. Sustain. Renew. Energ. Rep. 6, 52–60. doi: 10.1007/s40518-019-00130-7
Parker, S. S., Cohen, B. S., and Moore, J. (2018). Impact of solar and wind development on conservation values in the Mojave Desert. PLoS ONE 13, e0207678. doi: 10.1371/journal.pone.0207678
Ram, M., Aghahosseini, A., and Breyer, C. (2020). Job creation during the global energy transition towards 100% renewable power system by 2050. Technol. Forecast. Soc. Change 151, 119682. doi: 10.1016/j.techfore.2019.06.008
Rehbein, J. A., Watson, J. E., Lane, J. L., Sonter, L. J., Venter, O., Atkinson, S. C., et al. (2020). Renewable energy development threatens many globally important biodiversity areas. Glob. Chang. Biol. 26, 3040–3051. doi: 10.1111/gcb.15067
Safriel, U., Adeel, Z., Niemeijer, D., Puigdefabregas, J., White, R., Lal, R., et al. (2005). “Dryland systems,” in Ecosystems and Human Well-being: Current State and Trends.: Findings of the Condition and Trends Working Group, eds M. El-Kassas and E. Ezcurra (Washington, DC: Island Press), 623–662.
Selva, N., Switalski, A., Kreft, S., and Ibisch, P. L. (2015). “Why keep areas road-free? The importance of roadless areas,” in Handbook of Road Ecology, eds R. van der Ree, D. J. Smith, and C. Grilo (Hoboken, NJ: Wiley-Blackwell), 16–26.
Solargis (2021). Global Solar Atlas 2.0. Solargis. Available online at: https://globalsolaratlas.info/ (accessed October 2, 2021).
Taylor, N. T., Davis, K. M., Abad, H., McClung, M. R., and Moran, M. D. (2017). Ecosystem services of the big bend region of the Chihuahuan desert. Ecosyst. Serv. 27, 48–57. doi: 10.1016/j.ecoser.2017.07.017
Technical University of Denmark (2021). Global Wind Atlas 3.0. Technical University of Denmark. Available online at: https://globalwindatlas.info/ (accessed October 2, 2021).
Trainor, A. M., McDonald, R. I., and Fargione, J. (2016). Energy sprawl is the largest driver of land use change in United States. PLoS ONE 11, e0162269. doi: 10.1371/journal.pone.0162269
Trieb, F., Schillings, C., Pregger, T., and O'Sullivan, M. (2012). Solar electricity imports from the middle east and north Africa to Europe. Energ. Policy 42, 341–353. doi: 10.1016/j.enpol.2011.11.091
U.S. Bureau of Land Management (2016). Desert Renewable Energy Conservation Plan. Grand Junction, CO: United States Bureau of Land Management; Department of Interior.
U.S. Department of Energy (2008). 20% Wind Energy by 2030: Increasing Wind Energy's Contribution to U.S. Electricity Supply. Washington, DC: U.S. Department of Energy. Available online at: https://www.nrel.gov/docs/fy08osti/41869.pdf (accessed March 15, 2022).
United Nations (2015). Transforming Our World: The 2030 Agenda for Sustainable Development. United Nations. Available online at: http://sdgs.un.org/goals/ (accessed April 5, 2022).
Keywords: biodiversity, deserts, renewable energy, roadless areas, solar power, wind power
Citation: McClung MR and Moran MD (2022) Minimizing Impacts of Future Renewable Energy Development on the World's Desert Ecosystems. Front. Sustain. 3:900468. doi: 10.3389/frsus.2022.900468
Received: 20 March 2022; Accepted: 11 May 2022;
Published: 13 June 2022.
Edited by:
Sarah Marie Jordaan, Johns Hopkins University, United StatesReviewed by:
Yang Yu, Beijing Forestry University, ChinaCopyright © 2022 McClung and Moran. This is an open-access article distributed under the terms of the Creative Commons Attribution License (CC BY). The use, distribution or reproduction in other forums is permitted, provided the original author(s) and the copyright owner(s) are credited and that the original publication in this journal is cited, in accordance with accepted academic practice. No use, distribution or reproduction is permitted which does not comply with these terms.
*Correspondence: Matthew D. Moran, bW9yYW5AaGVuZHJpeC5lZHU=
†These authors have contributed equally to this work and share first authorship
Disclaimer: All claims expressed in this article are solely those of the authors and do not necessarily represent those of their affiliated organizations, or those of the publisher, the editors and the reviewers. Any product that may be evaluated in this article or claim that may be made by its manufacturer is not guaranteed or endorsed by the publisher.
Research integrity at Frontiers
Learn more about the work of our research integrity team to safeguard the quality of each article we publish.