- 1Grupo de Investigación en Materiales Porosos con Aplicaciones Ambientales y Tecnológicas (GIMPOAT), Departamento de Química, Facultad de Ciencias, Universidad del Tolima, Ibagué, Colombia
- 2Facultad de Ciencias, Departamento de Química, Universidad Nacional de Colombia, Bogotá, Colombia
- 3Grupo de Investigación en Sólidos Porosos y Calorimetría, Departamento de Química, Facultad de Ciencias, Universidad de los Andes, Bogotá, Colombia
Water is an essential part of life, and its availability is important for all living purposes, due to industrial development and the high demand for agricultural products that humans require for their survival, it has produced a high level of environmental pollution, which has generated a problem in recent decades. Among the large number of pollutants that have been found in wastewater is the glyphosate molecule (Gly), the most widely used herbicide for agriculture. Within this context, this mini-review summarizes the current advances and discussions on the development of nanomaterials, focusing on their application to capture the glyphosate molecule and thus, improve wastewater treatment. Metallic organic framework structures, graphene, and porous organic solids are among the most versatile porous materials that have been extensively investigated for application in glyphosate capture. Under this context, the intention of the following review is to provide and summarize the discussion of research advances in the recent capture of glyphosate, from wastewater using nanostructured materials.
Introduction
Glyphosate is one of the most word widely used herbicides, which is a non-selective, broad-spectrum systemic herbicide used to control perennial and annual weeds, its action is based on the ability to inhibit the biosynthesis of aromatic amino acids necessary for the shikimic acid pathway in plant metabolism. Although it is considered as an agrochemical that can be used in pest control, there is evidence of harmful effects on the environment such as: an imbalance in the nutrients of the fertile soil, a drop in butterfly populations, harmful effects on pollinators, such as bees, on aquatic organisms (phytoplankton, annelids, fish, etc.) among others. In addition, it has been found to cause cytotoxicity and damage to human cells (Schweitzer and Noblet, 2018; Joseph et al., 2019; Dharwal et al., 2020; Xiao et al., 2021; Zhou et al., 2021). For this reason, the WHO announced that glyphosate is also a probable carcinogen to humans (Samuel et al., 2017; Pereira et al., 2021).
Taking into account the necessity of removing this contaminant from the water, technological alternatives have been used over time such as, adsorption, membrane filtration, biodegradation with microorganisms, chemical coagulation, clarification, chlorination, ozonation, and photolysis. All of the aforementioned have shown the possibility of purifying water contaminated with glyphosate, however, adsorption has been positioned as a method of interest due to its simple design of operation, using low-cost adsorbents, and presenting high efficiency. For this purpose, different adsorbent solids have been used: clays, metal-organic framework, biochar, activated carbons, and nanostructured materials, the latter being of great current interest, given their physical and chemical properties (Yang et al., 2017; Sen and Chattoraj, 2021).
The importance of the topic is very high, and for this reason, this review presents a summary and a discussion of the advances of recent research in the capture of glyphosate from water using nanostructured materials.
Types of nanomaterials to adsorb glyphosate
In recent years, nanoscience and nanotechnology have positioned themselves as two concepts of great relevance in the world. Nanotechnology has gained great strength in the scientific world, the ability to handle the matter on a nanometric scale has opened the possibility of obtaining nanomaterials in the form of metals, ceramics, polymeric materials, or composite materials, with increasingly specific properties, capable of supplying a large number of technological and industrial needs (Kumar et al., 2018; Saleh, 2020; Mazari et al., 2021; Xu et al., 2021). Within a large number of applications of nanomaterials, the removal of contaminants is an area in which they are currently being widely used, taking advantage of their properties and versatility. In this sense, different investigations have demonstrated their usefulness in the adsorption of glyphosate from water sources. The most commonly used nanomaterials for this purpose include, namely, nanoparticles of metal oxides, nanoparticles of metals Ag, Zn, Fe, carbon nanotubes, and nanocomposites among others.
Metallic nanoparticles
Nanoparticles are materials with a size between 1 and 100 nm, characterized by a cubic structure centered on the faces, which gives them a greater reactivity area and elasticity. They can be formed by metal oxides or pure metals and their several applications.
In specific, they have been used in the removal of contaminants such as glyphosate, showing their efficiency. In this regard, different studies can be mentioned such as Páez et al. (2019), who synthesized ZnO nanoparticles using the controlled precipitation method, achieving removal of glyphosate between 70 and 90% without the use of UV radiation, meanwhile, Park et al. (2020) studied the efficiency of magnetite (Fe3O4) nanoparticles in the removal of glyphosate and found that these materials can remove up to 9.5% of this herbicide, likewise, Sen and Chattoraj (2021) prepared silver nanoparticles supported on activated carbon and used them in the removal of glyphosate, obtaining an adsorption capacity of 149.25 mg g−1.
Carbon nanotubes
Carbon nanotubes are allotropes of carbon, such as diamond or fullerenes. They are classified according to the number of graphite layers that make them up, in this way single-walled and multi-walled nanotubes can be found, in which there are layers of concentrically rolled graphite sheets in which the carbon has sp2 hybridization, the structure they possess gives them properties of interest such as density, conductivity, and resistance that are useful in different uses (Andrade Guel et al., 2012; Mazari et al., 2021). These materials have been tested in the removal of glyphosate, for example, Diel and collaborators developed through green synthesis an adsorbent based on carbon nanotubes with multiple layers and another in which they supported Fe nanoparticles; these materials were used in the removal of glyphosate, achieving the removal of between 84 and 86% of the contaminant in 120 min (Diel et al., 2021).
Biochar
Biochar is defined as a porous material produced by the thermochemical degradation of biomass in an oxygen-limited environment. In recent years, this material has generated interest due to its high abundance of surface functional groups and porosity (Chausali et al., 2021). Considering the aforementioned facts, the use of biochar and nanobiochar in pesticide and herbicide removal processes has been explored (Deolikar and Patil, 2022). For example, Ramanayaka et al. (2020), prepared nanobiochar derived from bioenergy wastes to evaluate their ability to remove emerging contaminants, specifically, they tested these materials in the adsorption of glyphosate finding a maximum removal of 922 mg g−1. Likewise, Hall et al., 2018 in their investigation they prepared biochar from walnut, cherry and apple wood chips, the materials were tested in the adsorption of glyphosate, obtaining a removal of 68%.
Nanocomposites
Nanocomposites are materials composed of particles of the nanometer order dispersed in a matrix; this composition gives them physical and chemical properties that combine the benefits of the atomic or molecular scale with the macroscopic world. In recent years, they have been employed for the removal of glyphosate from water. Briceño and Reinoso (2022), prepared a simple magnetic nanocomposite on the basis of graphene, chitosan, and CoFe2O4 nanoparticles as bioadsorbent, which was presented between 48 al 99% adsorption, meanwhile, Marin et al. (2019), prepared graphene–MnFe2O4 nanocomposite supported on vegetal-activated carbon (GO–MnFe2O4/VAC), the graphene was prepared by modified Hummes method, subsequently, the functionalization of this material with Mn Fe2O4 nanoparticles was performed, to finally support the assembly on the activated carbon, with this nanocomposite a maximum adsorption capacity of glyphosate of 6,778 mg g−1 was obtained.
Table 1 shows the glyphosate adsorption capacities of different nanomaterials.
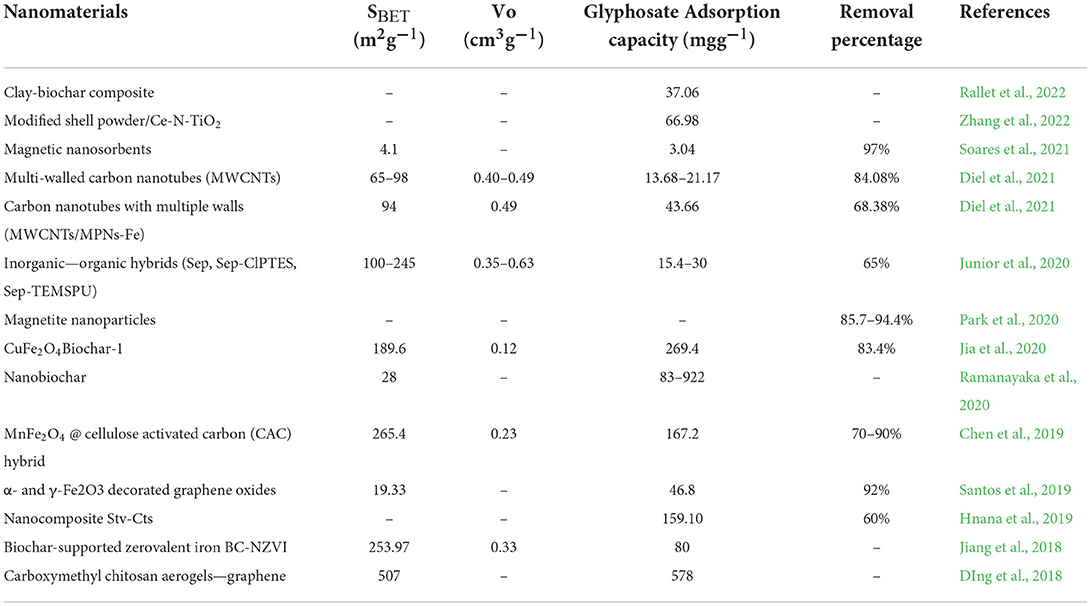
Table 1. Glyphosate adsorption capacity from aqueous solution, recently reported (2018–2022) for different materials.
The adsorption capacities of glyphosate, which nanomaterials have, as reported in different studies, show its potential in the removal of this herbicide. Despite the fact that several investigations have been carried out around the preparation of nanomaterials with specific properties for the removal of glyphosate from water, there are still many aspects to be investigated in this field of research. Among them is the possibility of improving the physical and chemical properties of nanomaterials, the feasibility of chemically modifying the nanostructures, increasing the adsorption capacity of the herbicide, and the adsorption mechanisms that occur in the adsorbate–adsorbent interaction, the useful life of adsorbents and their ability to work in cycles in such a way that the process is sustainable, among others.
Synthesis and characterization of nanomaterials to adsorb glyphosate
Different investigations have been carried out on the methods of preparing nanomaterials that allow them to be obtained with the desired shape, size and, chemical composition. Said methods can be classified into two large groups: precipitation methods and immobilization methods. Within the first category are modified emulsion precipitation, hydrothermal processing/solvothermal processing, sol–gel approach, and aerosol methods. On the contrary, the immobilization methods stand out: the gel-citrate method, the Penchini method, and low temperature combustion synthesis (Kolahalam et al., 2019; Kalaivani, 2021). The preparation techniques, mentioned earlier, lead to obtaining different forms of nanomaterials, with variable sizes and properties. In addition, they allow to induce small modifications in the synthesis processes that lead to various types of morphologies, electrical and optical properties. After the synthesis of nanomaterials, with aim to establish their characteristics in terms of size, shape, crystalline structure, chemical composition, optical, magnetic, and mechanical properties, techniques such as scanning electron microscopy (SEM), microscopy transmission electron (TEM), X-ray diffraction (XRD), high-resolution transmission electron microscopy (HRTEM), electronic energy loss spectroscopy (EELS) (Kranz and Mizaikoff, 2019; Senthil Kumar et al., 2019; Merugu and Gothalwal, 2021). Thanks to the aforementioned techniques, the properties of nanomaterials can be known, which determine their behavior and performance in different applications worldwide.
Surface modification of nanomaterials for glyphosate adsorption
As mentioned earlier, nanomaterials are characterized by presenting chemical, physical, and mechanical properties of great interest in pollutant removal processes. Different studies have shown the possibility of modifying the characteristics of these adsorbents, through different methods, through the addition of functional groups and the chemical functionalization of the surface, achieving an increase in the adsorption capacity and selectivity of the adsorbents (Diel et al., 2021; Soares et al., 2021).
Over time, different modification ways of nanomaterials have been studied, namely, covalent functionalization, non-covalent functionalization, inorganic functionalization, heteroatom-doping functionalization, immobilization functionalization, and post-synthesis functionalization. In general, these strategies lead to an increase in the surface acidity of nanomaterials, the elimination of mineral elements, the improvement of the hydrophilic nature, and the creation of surface groups (Rani and Shanker, 2020).
The most popular functionalization strategy previously mentioned is the chemical modification of nanomaterials by impregnating the surface with metallic nanoparticles, which can be achieved through chemical, physical, and biological routes. In the chemical route, the method of reduction in the liquid phase and doping is usually used using chemical agents as a source of the metal, for example, Fe3+, Mn5+, Co2+, and Cu2+ and reducing agents, through this route biochar impregnated with nanoparticles has been obtained. Fe, graphene oxide functionalized with MnFe2O4 supported on activated carbon, which has shown its effectiveness in the adsorption of glyphosate (Jiang et al., 2018; Marin et al., 2019).
However, the disadvantage of the chemical route is in the chemical agents used. For its part, the physical route presents high-energy consumption and high cost, while the biological technique is friendly to the environment, efficient, and economical. in this route extracts of plants, algae, or microorganisms are used to reduce the metallic ions at their disposal. the elemental state in a size range between 1 and 100 nm. In the process not only the metallic nanoparticles are produced but also functional groups such as hydroxyl, carboxyl, carbonyl, groups containing sulfur and nitrogen are generated in the material, which favors the interaction of the glyphosate molecule with the adsorbent, improving its retention capacity (Diel et al., 2021; Pereira et al., 2021).
The studies previously performed, aimed at the preparation of nanomaterials with potential application in the removal of glyphosate, have shown that, although the surface area of the adsorbents is an important aspect of removing this herbicide, the determining aspect to guarantee the efficiency in the adsorption process lies in the surface chemistry of the materials and the management of the pH in the process.
Since the surface charge of the adsorbents, the degree of dissociation of glyphosate and its speciation depends on it. In this sense, the chemical nature of the glyphosate molecule makes it present positive charges (in the amino group) and negative charges (in the carboxyl group and phosphonate) simultaneously (zwitterionic behavior). Because of this, glyphosate has a sequence of ionization constants that indicate the degree of dissociation of the compound as a function of pH.
Consequently, glyphosate adsorption is suitable for those materials that exhibit amphoteric behavior, which enables the surface of the adsorbents to be positively charged when the pH of the solution is lower than the pHPZC, which allows the adsorption of glyphosate by the anionic part of its structure since the molecule will be negatively charged.
It has been determined that the acidic pH between 2.29 and 6.14 is the most favorable for the removal of the herbicide, when the pH value of the solute is above the pHPZC of the adsorbent, the adsorption of glyphosate decreases with the pH of the solution due to the repulsive force between absorbents and glyphosate (Espinoza-Montero et al., 2020; Feng et al., 2020; Sen and Chattoraj, 2021).
Furthermore, the addition of metal ions in the adsorbents, such as Fe2+, Fe3+, and Cu2+, facilitates the formation of complexes with the glyphosate molecule, increasing its retention (Ighalo et al., 2021).
Mechanisms of glyphosate adsorption on nanomaterials
In order to obtain nanomaterials that are highly specific for adsorbing glyphosate, it is important to understand the adsorption mechanism of this molecule, which is carried out through physical and chemical interactions between its functional groups (-COOH, -NH2, and -PO(OH)2) and the materials (Pereira et al., 2021). In addition, the surface modification viability increases adsorbate–adsorbent affinity based on the understanding of the interaction that occurs between the glyphosate molecule with the materials. In this sense, different studies have established the possible mechanisms that may occur in the process of adsorption of this herbicide, among them: intraparticular diffusion in the pores of the adsorbent, complexation, hydrogen bonding, electrostatic interactions, and Van der Waals interactions (Guo et al., 2018; Dissanayake Herath et al., 2019; Pereira et al., 2021) (Figure 1).
The intraparticle diffusion of glyphosate through the porosity of the materials is considered the initial mechanism by which this molecule interacts with the adsorbents. In this way, the existing porous network plays a fundamental role in the removal of the herbicide since it can facilitate or restrict its passage depending on the size of the porosity that the material has (Herath et al., 2016).
For its part, the physical adsorption of glyphosate is mainly attributed to different molecular interaction forces, including permanent dipole/induced dipole interactions and quadrupole interaction, Van der Waals dispersion forces, π+-π electron donor–acceptor interactions, and hydrogen bonds through H-donor–acceptor interactions (Herath et al., 2016; Li et al., 2018; Pereira et al., 2021). In addition, the chemical adsorption of this substance on the nanomaterials must be considered, which depends on the electrostatic attractions between the molecule and the surface of the adsorbent material, the charges acquired by these two components involved in the retention mechanism are related to the pH of the medium, as discussed in the previous section.
Likewise, it is important to consider that the glyphosate molecule can behave as a Lewis base, thanks to the free electrons of the N and O atoms that compose it, and that they can establish coordinated covalent bonds with different metals, forming thermodynamically stable complexes, such as glyphosate-Ca2+, glyphosate-Zn2+, glyphosate-Ni+, glyphosate-Fe3+, and glyphosate-Mg2+. The stability of the complexes formed with glyphosate decreases in the order Fe3+> Al3+> Cu2+> Zn2+> Fe2+> Mn2+> Ca2+> Mg2+ (Jiang et al., 2018; Serra-Clusellas et al., 2019; Pereira et al., 2021).
Although, so far, the adsorption mechanisms mentioned earlier are the ones considered for the removal of glyphosate in different nanostructured materials.
Role of nanomaterials in current glyphosate removal technologies
In the past 4 years, studies on the removal of glyphosate from water have increased, due to its toxicity and environmental impact (Yaqoob et al., 2020). Different conventional and unconventional experimental techniques have been investigated, involving physicochemical, biological, and advanced oxidation processes, among others, which are used separately or in combination for the treatment of wastewater containing glyphosate.
According to the investigations, each of these alternatives has left advantages and disadvantages, however, has not been possible to establish the strategy with the greatest potential to remove this herbicide from contaminated water sources.
One of the techniques for the removal of glyphosate from wastewater is adsorption, which is a simple strategy, easy to implement, with high efficiency, low cost, high pollutant removal rate, and low risk of secondary contamination. Of a large number of existing adsorbents, it has been shown that nanomaterials have great advantages in the removal of this herbicide through adsorption processes, however, this alternative has serious disadvantages, such as the difficulty of regeneration of the materials once saturated, giving origin to a new waste that must be treated and the existence of competition with other substances present in wastewater (Espinoza-Montero et al., 2020; Feng et al., 2020; Yaqoob et al., 2020).
Despite the aforementioned disadvantages, the use of nanomaterials for glyphosate adsorption has managed to position itself as a viable and promising technology to be implemented at a practical level in the world. For example, Soares et al. (2021), synthesized magnetic nanoadsorbents made up of magnetite coated with hybrid trimethylchitosan (TMC) silica shells, which were used in the removal of glyphosate from wastewater from the Aveiro treatment plant in Portugal, achieving a 76.8% elimination of the herbicide, reaching the concentration established normatively in the water quality standards. In addition, the nanoadsorbents are regenerated by 80% after four adsorption–desorption cycles, which shows the viability of using this technology in the remediation of water contaminated with glyphosate. For his part, Park et al. (2020), synthesized magnetite nanoparticles that act as scavengers of the herbicide, they can be reactivated by thermal treatment, allowing their cyclical use, achieving a removal in real surface waters of forests and fields in Germany of up to 92.5%, with which a lower concentration value is reached to the maximum allowed for this substance. Likewise, Páez et al. (2019), demonstrated the feasibility of using ZnO nanoparticles for the removal of between 70 and 90% of glyphosate present in simulated aqueous systems.
Until now, it has not been possible to establish a single and effective method for the treatment of water contaminated with glyphosate. For this reason, the scientific community is currently conducting studies aimed at the combined use of experimental techniques. For example, the combination of adsorption with the degradation of glyphosate by microorganisms and the combination of adsorption with advanced oxidation processes (Espinoza-Montero et al., 2020; Feng et al., 2020). In both cases, the use of nanomaterials has been of great interest given the exceptional properties that these present structures.
Conclusions and future perspectives
The main intention of this article was to give a mini-review of current knowledge related to nanomaterials and their use in capturing glyphosate from water. It was evidenced that, although, a large number of studies developed the preparation, functionalization, and use of nanoparticles, metal organic framework nanoadsorbents, nanotubes, nanobiochar, nanocomposites, graphene among other nanomaterials, in the removal of glyphosate from water have different aspects and have to be still investigated.
In accordance with the above, it's important that research be carried out on: the possibility of improving the structural, and chemical properties of nanoadsorbents, incorporating changes in the synthesis processes, or post-synthesis modifications that allow increasing the glyphosate retention capacity in the structures. Likewise, the optimization in the design of nanomaterials that leads to high efficiency and easy regeneration use in several adsorption–desorption cycles, which would promote the sustainability of this technology to be able to be implemented on a large scale worldwide.
In addition, most of the research carried out around this topic in the past 4 years is limited to the study of glyphosate adsorption in model solutions prepared at the laboratory level, leaving still to be explored the behavior and efficiency of nanostructures in real wastewater and in water bodies such as rivers, lakes, streams, etc. In this sense, there is still much to investigate on this topic, in such a way that the technological developments achieved so far can be scaled up and the existing limitations overcome.
In this sense, the combination of technologies for the treatment of water contaminated with glyphosate tends to be an alternative with a high potential to be studied in the coming years, with a view to achieving high efficiency in the elimination of the herbicide from the water, without generating substances secondary toxicities, under low-cost operating conditions, easy handling and implementation. For this reason, it is important to highlight that nanotechnology must continue to be incorporated into existing water treatment technologies since it has been proving to be very useful in the processes of removal and degradation of pollutants and is positioned as a promising alternative.
Author contributions
All authors listed have made a substantial, direct, and intellectual contribution to the work and approved it for publication.
Funding
This work was supported by the Universidad de los Andes, the Universidad Nacional de Colombia, and the Universidad del Tolima. JM-P also appreciate the support provided by the vice-rectory of research of the Universidad de los Andes (Bogotá, Colombia) with the program FONDO - PUBLICA TUS NUEVOS CONOCIMIENTOS O EXPÓN TUS NUEVAS CREACIONES.
Acknowledgments
The authors thank the Faculty of Ciencias of Universidad de los Andes, the Universidad Nacional de Colombia, and the Universidad del Tolima for their support in the development of this research.
Conflict of interest
The authors declare that the research was conducted in the absence of any commercial or financial relationships that could be construed as a potential conflict of interest.
Publisher's note
All claims expressed in this article are solely those of the authors and do not necessarily represent those of their affiliated organizations, or those of the publisher, the editors and the reviewers. Any product that may be evaluated in this article, or claim that may be made by its manufacturer, is not guaranteed or endorsed by the publisher.
References
Andrade Guel, M. L., López, L. I. L., and Galindo, A. S. (2012). Nanotubos de carbono: Funcionalización y aplicaciones biológicas. Rev. Mex. Ciencias Farm. 43, 9–18. Available online at: https://www.scielo.org.mx/pdf/rmcf/v43n3/v43n3a2.pdf
Briceño, S., and Reinoso, C. (2022). CoFe2O4-chitosan-graphene nanocomposite for glyphosate removal. Environ. Res. 212, 113470–113479. doi: 10.1016/j.envres.2022.113470
Chausali, N., Saxena, J., and Prasad, R. (2021). Nanobiochar and biochar based nanocomposites: Advances and applications. J. Agric. Food Res. 5, 100191–100203. doi: 10.1016/j.jafr.2021.100191
Chen, Q., Zheng, J., Yang, Q., Dang, Z., and Zhang, L. (2019). Insights into the glyphosate adsorption behavior and mechanism by a MnFe 2 O 4 @cellulose-activated carbon magnetic hybrid. ACS Appl. Mater. Interfaces 11, 15478–15488. doi: 10.1021/acsami.8b22386
Deolikar, R., and Patil, R. (2022). ‘Recent advances in pesticides removal using agroindustry based biochar,” in Development in Wastewater Treatment Research and Processes, p. 265–290. doi: 10.1016/b978-0-323-85583-9.00021-1
Dharwal, M., Parashar, D., Shehu Shuaibu, M., Garba Abdullahi, S., Abubakar, S., and Baba Bala, B. (2020). Water pollution: effects on health and environment of Dala LGA, Nigeria. Mater. Today Proc. 49, 3036–3039. doi: 10.1016/j.matpr.2020.10.496
Diel, J. C., Franco, D. S. P., Nunes, I. D. S., Pereira, H. A., Moreira, K. S., Thiago, T. A., et al. (2021). Carbon nanotubes impregnated with metallic nanoparticles and their application as an adsorbent for the glyphosate removal in an aqueous matrix. J. Environ. Chem. Eng. 9, 105178–105191. doi: 10.1016/j.jece.2021.105178
DIng, C., Wang, X., Liu, H., Li, Y., Sun, Y., Lin, Y., et al. (2018). Glyphosate removal from water by functional three-dimensional graphene aerogels. Environ. Chem. 15, 325–335. doi: 10.1071/EN18087
Dissanayake Herath, G. A., Poh, L. S., and Ng, W. J. (2019). Statistical optimization of glyphosate adsorption by biochar and activated carbon with response surface methodology. Chemosphere. 227, 533–540. doi: 10.1016/j.chemosphere.2019.04.078
Espinoza-Montero, P. J., Vega-Verduga, C., Alulema-Pullupaxi, P., Fernández, L., and Paz, J. L. (2020). Technologies employed in the treatment of water contaminated with glyphosate: a review. Molecules 25, 5550–5576. doi: 10.3390/molecules25235550
Feng, D., Soric, A., and Boutin, O. (2020). Treatment technologies and degradation pathways of glyphosate: a critical review. Sci. Total Environ.742, 140559–140573. doi: 10.1016/j.scitotenv.2020.140559
Guo, L., Cao, Y., Jin, K., Han, L., Li, G., Liu, J., et al. (2018). Adsorption characteristics of glyphosate on cross-linked amino-starch. J. Chem. Eng. Data 63, 422–428. doi: 10.1021/acs.jced.7b00842
Hall, K. E., Spokas, K. A., Gamiz, B., Cox, L., Papiernik, S. K., and Koskinen, W. C. (2018). Glyphosate sorption/desorption on biochars - interactions of physical and chemical processes. Pest Manag. Sci. 74, 1206–1212. doi: 10.1002/ps.4530
Herath, I., Kumarathilaka, P., Al-Wabel, M. I., Abduljabbar, A., Ahmad, M., Usman, A. R. A., et al. (2016). Mechanistic modeling of glyphosate interaction with rice husk derived engineered biochar. Microporous Mesoporous Mater. 225, 280–288. doi: 10.1016/j.micromeso.2016.01.017
Hnana, K., Barhoun, A., and Draoui, K. (2019). Improvement of glyphosate adsorption using new composites based on ghassoul and chitosan: kinetics and equilibrium study. Mediterr. J. Chem. 9, 266–279. doi: 10.13171/mjc9419111121087kd
Ighalo, J. O., Adeniyi, A. G., and Adelodun, A. A. (2021). Recent advances on the adsorption of herbicides and pesticides from polluted waters: performance evaluation via physical attributes. J. Ind. Eng. Chem. 93, 117–137. doi: 10.1016/j.jiec.2020.10.011
Jia, D., Liu, M., Xia, J., and Li, C. (2020). Effective removal of aqueous glyphosate using CuFe2O4@biochar derived from phragmites. J. Chem. Technol. Biotechnol. 95, 196–204. doi: 10.1002/jctb.6221
Jiang, X., Ouyang, Z., Zhang, Z., Yang, C., Li, X., Dang, Z., et al. (2018). Mechanism of glyphosate removal by biochar supported nano-zero-valent iron in aqueous solutions. Colloids Surf. A Physicochem. Eng. Asp. 547, 64–72. doi: 10.1016/j.colsurfa.2018.03.041
Joseph, L., Jun, B. M., Jang, M., Park, C. M., Muñoz-Senmache, J. C., Hernández-Maldonado, A. J., et al. (2019). Removal of contaminants of emerging concern by metal-organic framework nanoadsorbents: a review. Chem. Eng. J. 369, 928–946. doi: 10.1016/j.cej.2019.03.173
Junior, H. B., da Silva, E., Saltarelli, M., Crispim, D., Nassar, E. J., Trujillano, R., et al. (2020). Inorganic–organic hybrids based on sepiolite as efficient adsorbents of caffeine and glyphosate pollutants. Appl. Surf. Sci. Adv. 1, 100025–100035. doi: 10.1016/j.apsadv.2020.100025
Kalaivani, K. (2021). A study on the synthesis and characterization methods of nanomaterials. Mater. Today Proc. 45, 3551–3555. doi: 10.1016/j.matpr.2020.12.1075
Kolahalam, L. A., Kasi Viswanath, I. V., Diwakar, B. S., Govindh, B., Reddy, V., and Murthy, Y. L. N. (2019). Review on nanomaterials: synthesis and applications. Mater. Today Proc. 18, 2182–2190. doi: 10.1016/j.matpr.2019.07.371
Kranz, C., and Mizaikoff, B. (2019). “Nanomaterials | Characterization methods,” in Encyclopedia of Analytical Science, eds P. Worsfold, A. Townshend, C. Poole, and M. Miro (London: Springer), 98–107. doi: 10.1016/B978-0-12-409547-2.13994-0
Kumar, P., Bansal, V., Kim, K. H., and Kwon, E. E. (2018). Metal-organic frameworks (MOFs) as futuristic options for wastewater treatment. J. Ind. Eng. Chem. 62, 130–145. doi: 10.1016/j.jiec.2017.12.051
Li, Y., Zhao, C., Wen, Y., Wang, Y., and Yang, Y. (2018). Adsorption performance and mechanism of magnetic reduced graphene oxide in glyphosate contaminated water. Environ. Sci. Pollut. Res. 25, 21036–21048. doi: 10.1007/s11356-018-2282-x
Marin, P., Bergamasco, R., Módenes, A. N., Paraiso, P. R., and Hamoudi, S. (2019). Synthesis and characterization of graphene oxide functionalized with MnFe2O4 and supported on activated carbon for glyphosate adsorption in fixed bed column. Process Saf. Environ. Prot. 123, 59–71. doi: 10.1016/j.psep.2018.12.027
Mazari, S. A., Ali, E., Abro, R., Khan, F. S. A., Ahmed, I., Ahmed, M., et al. (2021). Nanomaterials: applications, waste-handling, environmental toxicities, and future challenges - A review. J. Environ. Chem. Eng. 9, 105028–105061. doi: 10.1016/j.jece.2021.105028
Merugu, R., and Gothalwal, R. (2021). Microscopic techniques for characterisation of nanomaterials: a minireview. Mater. Today Proc. 47, 4753–4757. doi: 10.1016/j.matpr.2021.05.665
Páez, M. R., Ochoa-Muñoz, Y., and Rodriguez-Páez, J. E. (2019). Efficient removal of a glyphosate-based herbicide from water using ZnO nanoparticles (ZnO-NPs). Biocatal. Agric. Biotechnol. 22, 101434. doi: 10.1016/j.bcab.2019.101434
Park, H., May, A., Portilla, L., Dietrich, H., Münch, F., Rejek, T., et al. (2020). Magnetite nanoparticles as efficient materials for removal of glyphosate from water. Nat. Sustain. 3, 129–135. doi: 10.1038/s41893-019-0452-6
Pereira, H. A., Hernandes, P. R. T., Netto, M. S., Reske, G. D., Vieceli, V., Oliveira, L. F. S., et al. (2021). Adsorbents for glyphosate removal in contaminated waters: a review. Environ. Chem. Lett. 19, 1525–1543. doi: 10.1007/s10311-020-01108-4
Rallet, D., Paltahe, A., Tsamo, C., and Loura, B. (2022). Synthesis of clay-biochar composite for glyphosate removal from aqueous solution. Heliyon 8, e09112. 1–8. doi: 10.1016/j.heliyon.2022.e09112
Ramanayaka, S., Tsang, D. C. W., Hou, D., Ok, Y. S., and Vithanage, M. (2020). Green synthesis of graphitic nanobiochar for the removal of emerging contaminants in aqueous media. Sci. Total Environ. 706, 135725–135735. doi: 10.1016/j.scitotenv.2019.135725
Rani, M., and Shanker, U. (2020). “Remediation of organic pollutants by potential functionalized nanomaterials,” in Handbook of Functionalized Nanomaterials for Industrial Applications, ed C. M. Hussain (New York, NY: Elseivier), 327−398. doi: 10.1016/b978-0-12-816787-8.00013-2
Saleh, T. A. (2020). Nanomaterials: classification, properties, and environmental toxicities. Environ. Technol. Innov. 20, 101067–101083. doi: 10.1016/j.eti.2020.101067
Samuel, L., Wang, R., Dubois, G., Allen, R., Wojtecki, R., and La, Y. H. (2017). Amine-functionalized, multi-arm star polymers: a novel platform for removing glyphosate from aqueous media. Chemosphere 169, 437–442. doi: 10.1016/j.chemosphere.2016.11.049
Santos, T. R. T., Andrade, M. B., Silva, M. F., Bergamasco, R., and Hamoudi, S. (2019). Development of α- and γ-Fe 2 O 3 decorated graphene oxides for glyphosate removal from water. Environ. Technol. 40, 1118–1137. doi: 10.1080/09593330.2017.1411397
Schweitzer, L., and Noblet, J. (2018). “Water Contamination and Pollution,” in Green Chemistry: An Inclusive Approach, eds B. Török and T. Dransfield (New York, NY: Elsevier) 261–290. doi: 10.1016/B978-0-12-809270-5.00011-X
Sen, K., and Chattoraj, S. (2021). “A comprehensive review of glyphosate adsorption with factors influencing mechanism: Kinetics, isotherms, thermodynamics study,” in Intelligent Environmental Data Monitoring for Pollution Management, eds S. Bhattacharyya, J. Platos, P. Krömer, N. K. Mondal and V. Snasel (Czech: Academic Press), 93–125. doi: 10.1016/b978-0-12-819671-7.00005-1
Senthil Kumar, P., Grace Pavithra, K., and Naushad, M. (2019). “Characterization techniques for nanomaterials,” in Nanomaterials for Solar Cell Applications, eds S. Thomas, E. H. M. Sakho, N. Kalarikkal, S. O. O. Oluwafemi and J. Wu (New Delhi: Elsevier) 97–124. doi: 10.1016/B978-0-12-813337-8.00004-7
Serra-Clusellas, A., De Angelis, L., Beltramo, M., Bava, M., De Frankenberg, J., Vigliarolo, J., et al. (2019). Glyphosate and AMPA removal from water by solar induced processes using low Fe(III) or Fe(II) concentrations. Environ. Sci. Water Res. Technol. 5, 1932–1942. doi: 10.1039/c9ew00442d
Soares, S. F., Amorim, C. O., Amaral, J. S., Trindade, T., and Daniel-da-Silva, A. L. (2021). On the efficient removal, regeneration and reuse of quaternary chitosan magnetite nanosorbents for glyphosate herbicide in water. J. Environ. Chem. Eng. 9, 105189–105200. doi: 10.1016/j.jece.2021.105189
Xiao, L., Liu, J., and Ge, J. (2021). Dynamic game in agriculture and industry cross-sectoral water pollution governance in developing countries. Agric. Water Manag. 243, 106417–106426. doi: 10.1016/j.agwat.2020.106417
Xu, Y., Wang, H., Li, X., Zeng, X., Du, Z., Cao, J., et al. (2021). Metal–organic framework for the extraction and detection of pesticides from food commodities. Compr. Rev. Food Sci. Food Saf. 20, 1009–1035. doi: 10.1111/1541-4337.12675
Yang, Q., Wang, J., Zhang, W., Liu, F., Yue, X., Liu, Y., et al. (2017). Interface engineering of metal organic framework on graphene oxide with enhanced adsorption capacity for organophosphorus pesticide. Chem. Eng. J. 313, 19–26. doi: 10.1016/j.cej.2016.12.041
Yaqoob, A. A., Parveen, T., and Umar, K. (2020). Role of nanomaterials in the treatment of wastewater: A review. Water 2020. 12, 495–525. doi: 10.3390/w12020495
Zhang, W., Liang, Z., Lin, H., Shu, J., and Wang, A. (2022). Adsorption performance of glyphosate on modified shell powder/Ce-N-Tio 2. E3S Web Conf. 350, 01016. doi: 10.1051/e3sconf/202235001016
Keywords: nanostructured, glyphosate, adsorption, wastewater treatment, mini-review
Citation: Vargas-Delgadillo DP, Giraldo L and Moreno-Piraján JC (2022) Nanostructured materials for glyphosate capture—A mini-review. Front. Sustain. 3:888422. doi: 10.3389/frsus.2022.888422
Received: 02 March 2022; Accepted: 08 July 2022;
Published: 15 September 2022.
Edited by:
Ke Yin, Nanjing Forestry University, ChinaReviewed by:
Chen Ling, Nanjing Forestry University, ChinaCopyright © 2022 Vargas-Delgadillo, Giraldo and Moreno-Piraján. This is an open-access article distributed under the terms of the Creative Commons Attribution License (CC BY). The use, distribution or reproduction in other forums is permitted, provided the original author(s) and the copyright owner(s) are credited and that the original publication in this journal is cited, in accordance with accepted academic practice. No use, distribution or reproduction is permitted which does not comply with these terms.
*Correspondence: Juan Carlos Moreno-Piraján, anVtb3Jlbm9AdW5pYW5kZXMuZWR1LmNv
†These authors have contributed equally to this work and share first authorship