- School of Natural Sciences, National University of Ireland Galway, Galway, Ireland
• Presence of certain chemical additives (e.g. halogenated flame retardants, perfluoroalkyl substances, and phthalates) could hinder textile recycling operations as their presence renders end-of-life materials as “hazardous” and therefore not recyclable;
• In 2018, there was an estimated minimum of 1.7 million tonnes of hazardous and unrecyclable textile waste generated in the EU;
• Blanket-bans on classes of chemicals would likely be partially effective in reducing environmental uptake of and human exposure to harmful chemicals, but could also lead to increased use of “regrettable substitutions” with unforeseen implications;
• Legislative restrictions on chemicals must be accompanied with reduction in demand: reducing volume of textile waste generated by reducing volume consumed, thus eliminating necessity for huge volume of chemical additives in the first instance.
1. Introduction
Across the world, there have been major shifts in policies regarding the management of waste of all types. Ease of access to raw materials in the developed world and the relatively low economic burden of creating new products (vs. reuse of end-of-life materials) had led to the firm establishment of a linear economic model with regards to consumer goods (European Environment Agency, 2019). There are numerous factors which have led to intense scrutiny and decreasing popularity of this model over the last few decades: unsustainable single-use of plastic materials due to limited petrochemical resources; high volumes of environmental uptake of plastics, particularly in the marine environment; inadequate local waste management leading to excessive landfilling and/or incineration with less of an emphasis on recovery/recycling; emissions of toxic chemicals and pollutants from landfilling and incineration activities; undue burden on developing nations where large-scale waste management/recycling operations without proper oversight or safety considerations are available; and rapid production cycles requiring excessive amounts of natural resources accompanied by the emission of large quantities of environmental pollutants, including greenhouse gases (Ellen MacArthur Foundation, 2017). For these reasons and more, major international efforts have been focused on the widespread adoption of a circular economic model shifting toward more sustainable waste management options such as reuse and recycling, with a commensurate shift away from unsustainable options such as incineration and landfilling. These actions are exemplified by international treaties which set ambitious targets for more sustainable practices within the next several decades, such as those outlined in the UN Sustainability Goals (United Nations General Assembly, 2015) and the Paris Climate Accords (United Nations Framework Convention on Climate Change, 2015).
Though these efforts are very much an ongoing process, major strides have been made toward achieving the goal of a fully circular economy. Within the EU for example recycling targets for relevant end of life goods (plastics, cardboard, glass, metals, etc.) have been increasing year-on-year, with targets currently at 50% for certain household wastes (European Council, 2018). However, the current recycling targets do not take into account all types of end of life materials. For example, textiles are notably absent from inclusion in most recycling efforts worldwide, despite being responsible for roughly 5% of municipal waste generated annually. Globally, clothing manufacturing alone is also extremely resource-intensive, using an estimated 44 million tonnes of crude oil, 200,000 tonnes of pesticides, 8 million tonnes of fertilizers, and 43 million tonnes of additives chemicals (Ellen MacArthur Foundation, 2017), all with associated GHG emissions totalling to 1.2 billion tonnes of CO2 equivalent in 2015 alone (2.5% of global emissions for that year) (United States Environmental Protection Agency, 2018). These figures stand in stark contrast to the <1% of textiles that are currently sustainably recycled as there are currently no industrialized mechanisms to meet the surging demand (Ellen MacArthur Foundation, 2017). Within the EU, the vast majority of end of life textiles are currently shipped for reuse in developing countries or disposed of via conventional landfilling and/or incineration (Pensupa, 2020). This practice is due to be suspended by the 1st of January 2025 when, across all EU member-states, textiles are required to become a separate recycling stream as is currently in place for materials such as electrical equipment, plastics, glass, and cardboard (European Council, 2018).
There are major technical and economic challenges to implementing effective textiles recycling systems. For instance, though methods for recycling of textiles are under development, currently-available technologies are not yet suitable for the myriad of materials typical in consumer textiles (Piribauer et al., 2020; Ipsmiller and Bartl, 2021). Similarly, these systems are not currently scalable to the degree required to process the massive amounts of textile waste generated each year (Ipsmiller and Bartl, 2021): some 100 million tonnes annually (Ütebay et al., 2020), increasing by roughly 10% per year over the last 5 years and with this trend likely continuing to increase in the coming years (Pensupa, 2020).
There are additionally safety concerns regarding the recyclability of textiles due to the presence of hazardous chemical additives in various textile-based materials. EU regulations currently limit the concentrations of certain hazardous additives known to be used in textile items from entering the waste stream, such as certain brominated flame retardants (BFRs) and perfluoroalkyl substances (PFASs) (European Council, 2021), while more still are listed as Ecotoxic (European Council, 2018) or “substances of very high concern” (European Council, 2022). This is currently an ongoing issue for other polymeric materials in consumer products such as waste electrical and electronic equipment (WEEE) and building insulation foams (Drage et al., 2018; Sharkey et al., 2018; European Council, 2022). These studies outline the potential for screening methods which could separate hazardous plastics from clean and safe to recycle materials. Many of the aforementioned restricted and concerning chemicals are still in circulation and present in WEEE, building insulation foams, and vehicular textiles well-above EU-set concentration limits (Drage et al., 2018) and would remain so were these items to be recycled as (i) methods for rapidly screening those wastes for such hazardous substances are still in the developmental stages and not widely used (Zhang et al., 2015; Sharkey et al., 2018, 2020a) and (ii) methods to remove hazardous chemicals from textile waste items on the requisite scale do not yet exist. This is an important parallel to the forthcoming similar issue which will encumber safe textile screening and recycling operations in order to meet regulatory requirements. Textiles, similar to WEEE plastics and building materials, have been shown to contain excess concentrations of such hazardous chemicals additives despite restrictions (Drage et al., 2018). The need to comply with legislation regarding their presence in waste textiles coupled with the need to prevent further environmental uptake from their improper disposal (Harrad et al., 2020) constitutes a very noteworthy variable in how effective and environmentally safe textile recycling systems can realistically be.
2. Current Policies and Implications
2.1. Persistent Organic Pollutants and Other Hazardous Chemicals Associated With Restrictions
A wide range of chemicals and additives are used in consumer textiles to impart various desirable properties on the particular item(s). This policy brief will be focusing on three chemical families known or suspected to be of human health and/or environmental concern:
• Halogenated flame retardants (HFRs) which are added to impart fire-resistance or fire-suppression properties;
• Perfluoroalkyl substances (PFASs), used to impart dirt/stain-repellent properties;
• Phthalates, plasticizers used to improve certain properties of textiles such as flexibility and durability;
Table 1 below summarizes the various hazards and restrictions currently in place on some of the most widely-used HFRs, PFASs, and phthalates in textiles.
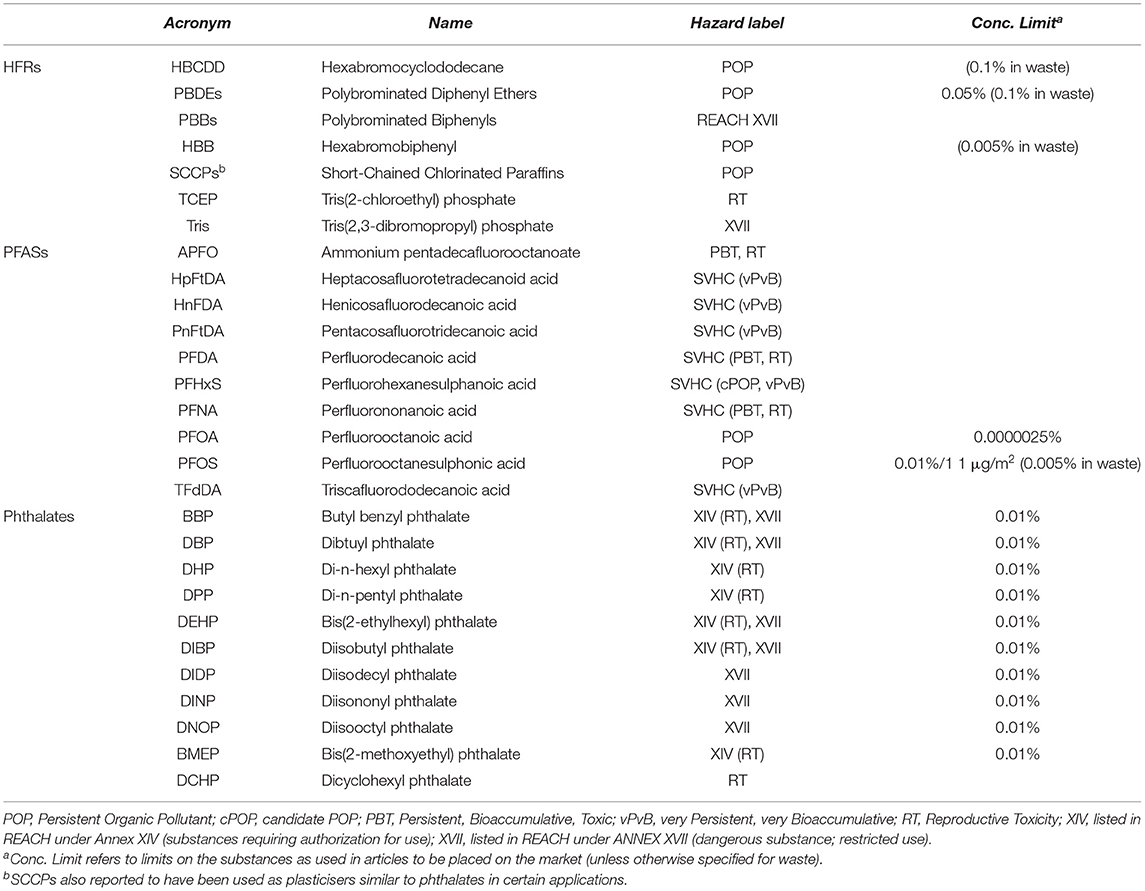
Table 1. List of BFRs, PFASs, and phthalates restricted under EU Regulations and/or listed as substances of very high concern (SVHC) and candidates for inclusion in REACH. Hazard label “POPs” covered under Regulation EU 2019/1021 (POPs); all others covered under Regulation EC 1907/2006 (REACH).
While exact figures on the extent and prevalence of these chemicals in various textile applications are not available, some estimates can be made based on experimental findings from front-line research. HFRs such as brominated flame retardants (BFRs) (Drage et al., 2018) and short-chained chlorinated paraffins (SCCPs) (Prasannamedha and Sentihilkumar, 2021) are shown to be more narrowly-used in textiles: as opposed to clothing, more regularly found in household and industrial textiles where regulations mandate a certain degree of flame retardancy from materials such as through the use of additive flame retardants. One study in Ireland estimates that roughly 2.5 kg per capita of hazardous textile waste—based on BFR-content alone—was generated in 2018 (Sharkey et al., 2018); this equates to potentially 1.1 million tonnes of hazardous textile waste generated across the EU in the same year due to restricted BFRs.1
Some estimates suggest that between 3% and 10% of textiles in the EU have used PFASs in the manufacturing process or as an additive on the finished article (Nordic Council of Ministers, 2019). In 2015, it was estimated that the 34.8% of PFASs produced were utilized in the textile sector, which was projected to rise to almost 50% by 2020. Until their phasing-out following their listing as POPs, PFOS, and PFOA dominated the market for PFASs for many years, though goods treated with these POPs are likely still in circulation as restrictive measures are slow to be adopted for various reasons (United Nations Environment Programme, 2019a). Based on the concentration limits currently in place, it is possible that the majority of treated textiles would be considered hazardous based on PFAS-content (some 0.6 million tonnes) (Nordic Council of Ministers, 2019). However, these are preliminary estimates based on available data and more precise quantification would require more in-depth study.
It's estimated that over 8 million tonnes of phthalates are produced annually, dominated by the restricted DEHP and DINP which made up over 75% of global market share in 2017 (Benjamin et al., 2017). Though a relatively small fraction of these phthalates are likely to be used in textiles compared to other applications (Li et al., 2019), this is an area which requires further investigation due to the lack of available data.
2.2. Implications of Legislative Restrictions on Textile Reuse and Recycling
Despite the fact that the EU regulations exist to limit the presence and prohibit the use of certain hazardous substances in consumer articles entering the market (as well as those that entering the waste stream), several factors prevent this from being a fool-proof mechanism for preventing these goods from circulating.
(i) The vast majority of clothing consumed in the EU, where the most comprehensive restrictions on POPs and other organic contaminants are in place, are produced outside the EU (ca. 60% produced in China, Bangladesh, India, Pakistan, Vietnam, and Indonesia) (Pensupa, 2020).
(ii) The colossal volume of textiles produced and consumed require a seamless network of transportation to operate effectively, potentially without proper screening checks to ensure compliance with transboundary movement of goods. The EU's REACH regulation requires strict oversight of import and export of articles treated with listed chemicals (including those in Table 1) (European Council, 2022), while the Stockholm Convention on Persistent Organic Pollutants require that wastes containing POPs are not transported across international boundaries (United Nations Environment Programme, 2019b). However, despite this, it was reported that 19% of apparel companies had full knowledge of their entire supply chains, while only 15% had full traceability of the materials used in manufacturing (KPMG, 2021).
(iii) Even if full supply chain knowledge was known, methods for screening articles for these hazardous substances are not currently available at the specifications nor magnitudes required for the vast volume of goods (Harrad et al., 2019). This has potential implications for (a) newly manufactured goods containing hazardous substances which were not properly screened at manufacturing stage; (b) goods made from recycled materials where those materials were fully or partially made from recycled feedstock; and (c) textiles entering the waste stream which must be diverted from recyclate and/or disposed of by special means (i.e., high temperature incineration) due to hazardous chemical content (Sharkey et al., 2020b).
The volume of textile waste generated in the EU annually which is liable to be hazardous due to the presence of these chemical additives could be as little as 1.1–1.7 million tonnes2. This equates to at least 4.7 billion euros of raw materials which cannot be exported nor can it be reused or recycled3. However, the larger issue is that screening methods for detecting these chemicals at the requisite scale are not available, thus it is currently impossible to know, given a specified volume of end of life textiles, which are safe to recycle and which contain excess hazardous chemicals (Zhang et al., 2015; Ritter et al., 2017; Sharkey et al., 2020b). Without a guarantee from the supply-chain of the lack of any hazardous chemical content in the articles or waste materials, ALL such materials (whether clean or not) would be required to be separated for specialized disposal, i.e., incineration, thus losing the finite raw material (European Council, 2018).
3. Actionable Recommendations
Textile recycling as an industrial process is relatively novel and only effectively utilized on large-scales in a few parts of the world. The vast majority of end-of-life textiles which could be recycled are still being disposed of along with general waste, i.e., landfilled or incinerated. Where good recycling operations are in effect (Nørup et al., 2018; O'Leary et al., 2021; Piribauer et al., 2021) these are largely focused on reuse and resale through charity shops or donations to third world regions, where end of life materials are ultimately disposed of without the chance for reuse. Major developments have been made in recent years to develop sustainable textile recycling methodologies, particularly for polyethylene terephthalate (PET)-based materials (Ipsmiller and Bartl, 2021; Piribauer et al., 2021); however, barriers still exist before these methods can be properly utilized. In addition to these methods requiring substantial upscaling to meet demands, they will likely also need to be individualized for various types and blends of fabrics to be effective as well as be coupled with effective collection and sorting methods to garner heterogeneous waste materials (Ipsmiller and Bartl, 2021). In terms of the raw textile waste, these methods should be further developed and adopted in order to sustainably manage the vast quantities of textile waste being produced globally. However, in terms of the hazardous chemical additives which may be present in these end-of-life materials, even more must be done to mitigate the potential environmental harm.
Blanket restrictions on certain classes of chemicals (e.g., all PFASs, all additive halogenated flame retardants, etc.) would virtually guarantee that such hazardous chemicals are not being circulated in consumer goods (Gobalakrishnan et al., 2021). However, underlying issues exist which would ultimately limit the efficacy of such restrictions or prevent actionable restrictions from being implemented in the first place. In the case of additive flame retardants, these are added in order to meet fire safety standards which are largely thought to be outdated and in need of revision to reflect modern consumer habits (ban in indoor smoking, lower smoking rates, improved electrical equipment safety ratings, consumer fire safety awareness, etc.) (Charbonnet et al., 2020). However, in certain cases, alternatives do not yet exist in cases where their use is still required such as in firefighter apparel, aircraft components, or building insulation. Environmentally safer alternatives must be posited, such as novel Poly-FRs in building insulation foams to replace now restricted hexabromocyclododecane, the former being a reactive (chemically-bound) compound as opposed to the latter being an additive and thus more likely to leach out. The use of these safer alternatives must be promoted while also ensuring the cycle of “regrettable substitutions” is prevented where replacement chemicals may have similar hazardous properties as predecessors (Fantke et al., 2015; Sharkey et al., 2020b). This must then be coupled with producers making more active efforts to know all steps of the product lifecycle (both pre- and post-consumption) as well as assessing whether the use of certain chemical additives are essential for the required properties of the final product (Cousins et al., 2019). PFASs are slightly more complex in that they are more ubiquitously used in a wider range of consumer goods and largely in response to consumer demand as opposed to legal requirements (e.g., non-stick frying pans and waterproof clothing). In this case, as well as in the case of phthalates, a shift away from those compounds shown or suspected of being hazardous toward those which may be safer in terms of health effects and/or/ environmental uptake is certainly warranted.
However, especially in the case of textiles, a bigger issue is the sheer volume of global consumption annually, only a minute fraction of which ultimately undergoes any sort of sustainable recycling (Ellen MacArthur Foundation, 2017). A radical shift away from excessive consumption and fast-fashion is therefore warranted in order to reduce the quantities of textiles being manufactured, which will commensurately reduce the quantities of hazardous chemicals being used and ultimately ending up in the environment. In the interim, further research and development of technologies for screening of these hazardous chemicals in end of life materials is needed in order to prevent the continuous recirculation and continued environmental uptake of said substances while attempting to maximize textile recycling processes (Zhang et al., 2015; Ritter et al., 2017; Sharkey et al., 2018). Additionally, the creation of a publically-available database on the hazardous chemical content in textiles specifically should be developed, similar to what has been proposed and developed for plastics (Aurisano et al., 2021).
4. Conclusions
No single legislative solution currently exists which will solve the issue of hazardous chemicals additives in textiles, either for those newly manufactured or those destined for the recycling system. Further research into the hazardous properties of textile additives should continue and, where necessary, actionable restrictions should be placed on their application in new goods and their presence in recyclable materials. Further research should also be conducted into the presence of hazardous chemicals in end of life textiles entering the waste stream as reliable figures on the volumes of hazardous wastes being generated annually are not currently available. This knowledge is required as it can aid in directing and streamlining policies for specific goods and materials which may be of a higher priority for screening and/or the imposing of specific restrictions due to particularly high hazardous chemical content, rather than blanket restrictions which lead to the disposal of large quantities of otherwise useful raw materials.
This further research must also be accompanied by a range of other measures to ease the burden faced by the burgeoning textile recycling industry: revisions to fire safety standards which unnecessarily require the use of additive HFRs; societal and behavioral changes such as reductions in textile consumption and shifting away from fast-fashion toward more sustainable models; industrial changes such as improved monitoring for additive-use in manufactured goods and imports, as well as improved and widespread use of methods for screening of end-of-life materials which may or may not contain hazardous substances; and finally, improved consumer knowledge of the hazards associated with these chemical additives to allow them to balance the necessity for their application in consumer goods with the potential environmental and human health hazards resulting from their continued use.
Author Contributions
MS led the conceptualization, research, and writing all aspects of the manuscript, while MC assisted in research, writing, and proofing all aspects of the manuscript. Both authors contributed to the article and approved the submitted version.
Conflict of Interest
The authors declare that the research was conducted in the absence of any commercial or financial relationships that could be construed as a potential conflict of interest.
Publisher's Note
All claims expressed in this article are solely those of the authors and do not necessarily represent those of their affiliated organizations, or those of the publisher, the editors and the reviewers. Any product that may be evaluated in this article, or claim that may be made by its manufacturer, is not guaranteed or endorsed by the publisher.
Footnotes
1. ^Based on an EU population of 447.01 million inhabitants in 27 EU states as of 2021 (https://european-union.europa.eu/principles-countries-history/key-facts-and-figures/life-eu_en).
2. ^Based on estimated 1.1 million tonnes of ELV textiles containing excess BFRs and 0.6 million tonnes containing excess PFASs (see Section 2.1 – note that reliable figures on phthalates are not available to include in the estimate).
3. ^Based on estimated polyester fabric cost of 2.08 USD/kg (https://www.fibre2fashion.com/texpro/raw-material) plus 34% mark-up for production and labor costs (https://sourcemygarment.com/2015/05/07/the-real-cost-of-fabric/), equating to 3.15 USD/kg (appx. 2.75 EUR/kg).
References
Aurisano, N., Weber, R., and Fantke, P. (2021). Enabling a circular economy for chemicals in plastics. Curr. Opin. Green Sustain. Chem. 31:100513. doi: 10.1016/j.cogsc.2021.100513
Benjamin, S., Masai, E., Kamimura, T., Anderson, R. C., and Fairsal, P. A. (2017). Phthalates impact on human health: epidemiological evidences and plausible mechanisms of action. J. Hazard. Mater. 340, 360–383. doi: 10.1016/j.jhazmat.2017.06.036
Charbonnet, J. A., Weber, R., and Blum, A. (2020). Flammability standards for furniture, building insulation and electronics: benefit and risk. Emerg. Contaminants 6, 432–441. doi: 10.1016/j.emcon.2020.05.002
Cousins, I. T., Goldenman, G., Herzke, D., Lohmann, R., Miller, M., Ng, C. A., et al. (2019). The concept of essential use for determining when uses of PFASs can be phased-out. Environ. Sci. Process. Impacts. 21, 1803–1815. doi: 10.1039/C9EM00163H
Drage, D. S., Sharkey, M., Abdallah, M. A. E., Berresheim, H., and Harrad, S. (2018). Brominated flame retardants in Irish waste polymers: concentrations, legislative compliance, and treatment options. Sci. Total Environ. 625, 1535–1543. doi: 10.1016/j.scitotenv.2018.01.076
Ellen MacArthur Foundation (2017). A New Textile Economy: Redesigning Fashion's Future. Available online at: https://ellenmacarthurfoundation.org/a-new-textiles-economy (accessed January 17, 2022).
European Council (2018). Directive 2008/98/EC on Waste. Available online at: ELI: http://data.europa.eu/eli/dir/2008/98/2018-07-05 (accessed January 27, 2022).
European Council (2021). Regulation (EU) 2019/1021 on Persistent Organic Pollutants. Available online at: http://data.europa.eu/eli/reg/2019/1021/oj (accessed January 27, 2022).
European Council (2022). Regulation (EC) No 1907/2006 Concerning the Registration, Evaluation, Authorisation and Restriction of Chemicals (REACH). Available online at: http://data.europa.eu/eli/reg/2006/1907/2022-01-08 (accessed January 27, 2022).
European Environment Agency (2019). Textiles in Europe's Circular Economy. Available online at: https://www.eea.europa.eu/publications/textiles-in-europes-circular-economy (accessed January 17, 2022).
Fantke, P., Weber, R., and Scheringer, M. (2015). From incremental to fundamental substitution in chemical alternative assessment. Sustain. Chem. Pharm. 1, 1–8. doi: 10.1016/j.scp.2015.08.001
Gobalakrishnan, M., Das, S., and Saravanan, D. (2021). Hazardous, Restricted, and Manufacturing Restricted Substances in Textiles and Clothing Supply Chain. Chemical Management in Textiles and Fashion – Chapter 6. Sawston: Woodhead Publishing. doi: 10.1016/B978-0-12-820494-8.00006-X
Harrad, S., Drage, D. S., Abdallah, M. A.-E., Sharkey, M., and Berresheim, H. (2019). Evaluation of Hand-Held XRF for Screening Waste Articles for Exceedances of Limit Values for Brominated Flame Retardants. Environmental Protection Agency. Available online at: https://www.epa.ie/publications/research/waste/research-272.php (accessed January 27, 2022).
Harrad, S., Drage, D. S., Sharkey, M., and Berresheim, H. (2020). Perfluoroalkyl substances and brominated flame retardants in landfill-related air, soil, and groundwater from Ireland. Sci. Total Environ. 705:135834. doi: 10.1016/j.scitotenv.2019.135834
Ipsmiller, W., and Bartl, A. (2021). “Textile Waste: An Inventory of Available and Emerging Recycling Technologies,” in Conference Proceedings- 18th International Symposium on Waste Management and Sustainable Landfilling, Sardinia, Italy.
KPMG (2021). Moving the Needle – Threading a Sustainable Future for Apparel. Available online at: https://home.kpmg/cn/en/home/insights/2021/11/moving-the-needle.html (accessed January 27, 2022).
Li, H.-L., Ma, W.-L., Liu, L.-Y., Zhang, Z., Sverko, E., Zhang, Z.-F., et al. (2019). Phthalates in infant cotton clothing: occurrence and implications for human exposure. Sci. Total Environ. 683, 109–115. doi: 10.1016/j.scitotenv.2019.05.132
Nordic Council of Ministers (2019). The Cost of Inaction – A Socioeconomic Analysis of Environmental And Health Impacts Linked to Exposure to PFAS. Available online at: http://norden.diva-portal.org/smash/get/diva2:1295959/FULLTEXT01.pdf (accessed January 27, 2022).
Nørup, N., Pihl, K., Damgaard, A., and Scheutz, C. (2018). Development and testing of a sorting and quality assessment method for textile waste. Waste Manage. 79, 8–21. doi: 10.1016/j.wasman.2018.07.008
O'Leary, E., Tobin, K., Downey, C., Hanlon, E., and Miller, S. (2021). “Nature and Extent of Post-Consumer Textiles in Ireland – Stakeholder Views”. Environmental Protection Agency of Ireland, Wexford, Ireland.
Pensupa, P. (2020). “Recycling of end-of-life clothes,” in Sustainable Technologies for Fashion and Textiles, ed R. Nayak (Sawston: Woodhead Publishing), 251–309. doi: 10.1016/B978-0-08-102867-4.00012-8
Piribauer, B., Bartl, A., and Ipsmiller, W. (2021). Enzymatic textiles recycling – best practices and outlook. Waste Manage. Res. 39, 1277–1290. doi: 10.1177/0734242X211029167
Piribauer, B., Jenull-Halver, U., Quartinello, F., Ipsmiller, W., Koch, D., Laminger, T., et al. (2020). TEX2MAT – Next level textile recycling with biocatalysts. Detritus 13, 78–86. doi: 10.31025/2611-4135/2020.14030
Prasannamedha, G., and Sentihilkumar, P. (2021). “Chemical compliance and regulations in textiles and fashion,” in Chemical Management in Textiles and Fashion, ed S. S. Muthu (Sawston: Woodhead Publishing), 135–154. doi: 10.1016/B978-0-12-820494-8.00007-1
Ritter, E. E., Dickinson, M. E., Harron, J. P., Lunderberg, D. M., DeYoung, P. A., Robel, A. E., et al. (2017). PIGE as a screening tool for Per- and polyfluorinated substances in papers and textiles. Nucl. Instrum. Methods Phys. Res. Sec. B 407, 47–54. doi: 10.1016/j.nimb.2017.05.052
Sharkey, M., Abdallah, M. A. E., Drage, D. S., Harrad, S., and Berresheim, H. (2018). Portable X-ray fluorescence for the detection of POP-BFRs in waste plastics. Sci. Total Environ. 639, 49–57. doi: 10.1016/j.scitotenv.2018.05.132
Sharkey, M., Drage, D. S., Abdallah, M. A.-E., Harrad, S., and Berresheim, H. (2020a). “Brominated flame retardants and perfluoroalkyl substances in Irish Waste,” in Society of Environmental Toxicology and Chemistry (SETAC) Europe 30th Annual Meeting. Dublin (2020).
Sharkey, M., Harrad, S., Abdallah, M. A.-E., Drage, D. S., and Berresheim, H. (2020b). Phasing-out of brominated flame retardants: the UNEP Stockholm Convention and other legislative action worldwide. Environ. Int. 144:106041. doi: 10.1016/j.envint.2020.106041
United Nations Environment Programme (2019a). Stockholm Convention on Persistent Organic Pollutants – Register of Specific Exemptions: Perfluorootanoic Acid (PFOA), Its Salts and PFOA-Related Compounds. Available online at: http://chm.pops.int/Implementation/Exemptions/SpecificExemptions/PFOARoSE/tabid/8363/Default.aspx (accessed February 09, 2022).
United Nations Environment Programme (2019b). Stockholm Convention on Persistent Organic Pollutants. Available online at: http://chm.pops.int/ (accessed January 27, 2022).
United Nations Framework Convention on Climate Change (2015). Paris Agreement. Available online at: https://treaties.un.org/ (accessed January 17, 2022).
United Nations General Assembly (2015). Transforming Our World: The 2030 Agenda for Sustainable Development. Available online at: https://sdgs.un.org/2030agenda (accessed January 17, 2022).
United States Environmental Protection Agency (2018). Climate Change Indicators: Global Greenhouse Gas Emissions. Available online at: https://www.epa.gov/climate-indicators/ (accessed January 27, 2022).
Ütebay, B., Celik, P, and Cay, A. (2020). Textile Wastes: Status and Perspectives, Waste in Textile and Leather Sectors - Chapter 3. London: IntechOpen Book Series. doi: 10.5772/intechopen.92234
Zhang, L., Luo, X., Niu, Z., Ye, X., Tang, Z., and Yao, P. (2015). Rapid screening and identification of multi-class substances of very high concern in textiles using liquid chromatography-hybrid linear ion trap orbitrap mass spectrometry. J. Chromatogr. A 1386, 22–30. doi: 10.1016/j.chroma.2015.01.077
Keywords: textile recycling, persistent organic chemical, textile waste, brominated flame retardant (BFR), perfluoroalkyl substance (PFAS), phthalates, fast-fashion, circular economy
Citation: Sharkey M and Coggins M (2022) The Invisible Barrier to Safe Textile Recycling. Front. Sustain. 3:876683. doi: 10.3389/frsus.2022.876683
Received: 15 February 2022; Accepted: 17 March 2022;
Published: 14 June 2022.
Edited by:
Lei Huang, University of Michigan, United StatesReviewed by:
Li Li, University of Nevada, United StatesRoland Weber, POPs Environmental Consulting, Germany
Copyright © 2022 Sharkey and Coggins. This is an open-access article distributed under the terms of the Creative Commons Attribution License (CC BY). The use, distribution or reproduction in other forums is permitted, provided the original author(s) and the copyright owner(s) are credited and that the original publication in this journal is cited, in accordance with accepted academic practice. No use, distribution or reproduction is permitted which does not comply with these terms.
*Correspondence: Martin Sharkey, bWFydGluLnNoYXJrZXlAbnVpZ2Fsd2F5Lmll