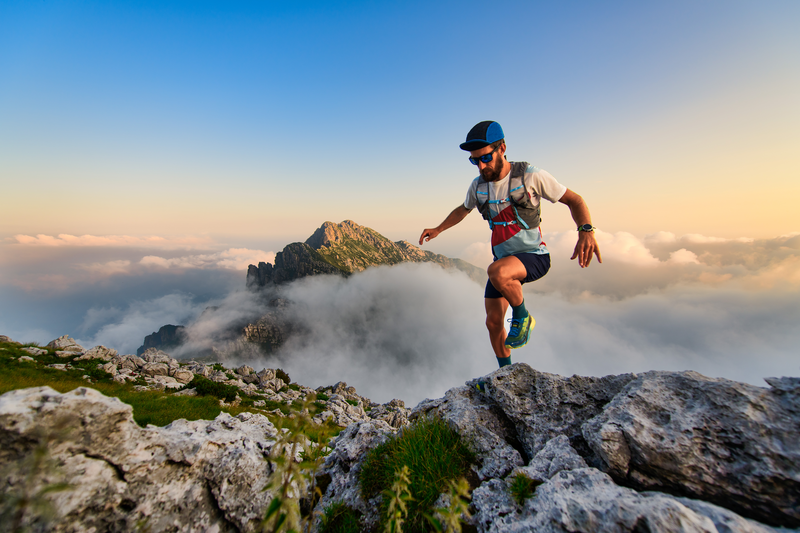
94% of researchers rate our articles as excellent or good
Learn more about the work of our research integrity team to safeguard the quality of each article we publish.
Find out more
ORIGINAL RESEARCH article
Front. Sustain. , 26 October 2022
Sec. Modeling and Optimization for Decision Support
Volume 3 - 2022 | https://doi.org/10.3389/frsus.2022.841643
The demand to enact sustainability into higher education so as to optimistically shape the wider society and biosphere has been stressed by United Nations Educational, Scientific and Cultural Organization (UNESCO). One of the approaches is through rethinking and revising education at all levels to capture obvious forms of present and imminent societies on the development of sustainability knowledge, skills, perspectives and values. Several Higher Education Institutes (HEIs) have started to implement a number of facets to that effect, such as signing a climate commitment, working towards plan to make their campuses climate-neutral, and making sustainability their guiding principles and top priorities. However, analogous modifications to the curriculum are lagging behind. As a consequence, this study is set to quantitatively validate the technical sustainability competency model suitable for incorporation into Higher National Diploma electrical/electronic engineering curriculum in Nigeria. The authors used findings of their earlier work to develop a questionnaire for collecting data from 168 respondents in the study area, and consequently subject the data to descriptive and inferential statistics with the aid of PLS-SEM approach. The study discovered competencies suitable for incorporation into the curriculum. This includes cognitive skills in Eco-design and Life-Cycle Assessment; Research; Modeling, Simulation and Optimization; and Recycling/Renewable Resources. The study also found suitable psychomotor skills in Sustainable production, Use of modern engineering software tools, Operation/troubleshooting of electrical machines and devices, Communication/Information and Communication Technology, and waste-to-energy technology. Appropriate attitudes/values in Engineering ethics, Occupational safety and health, and Inter-generational equity are also discovered. This research is purely quantitative in nature carried out through administering questionnaires to respondents in one geo-political zone of the country. Thus, conclusions derived from these sources rely on the genuineness of the information provided by the participants. The findings offer accreditation body as well as curriculum developers in Nigerian polytechnics with a validated model of technical sustainability competences. This could be useful in the events of curriculum upgrade or renewal to integrate sustainability.
The world's geometric population growth, the advent of industrial transformation, new standards of lifestyle preoccupied with growing earnings, ill-defined distribution of world's resources, and several other human factors have jointly contributed to the stressed earth's ecosystem. Chu et al. (2013) stressed that yearly CO2 secretion climbs to more than 30.6 gigatonnes worldwide. Africa, in particular, faces numerous challenges that cause negative environmental impacts including increased climate change susceptibility, desertification of arid areas, deforestation, rapid urbanization, degeneration of biological resources, degradation of coastal and marine habitats, intensified water shortage and stumpy quality, prevalent poverty, pitiable economic performance, ineffective trade policies unfavorable toward peace and development, defective technology base to meet existing demand, occurrences of civil conflicts, and escalation in unlawful trade in minerals and other natural resources (Lotz-Sisitka et al., 2015). Most of these problems have complex roots and drivers and are related to insufficient sustainability education as a driver for sustainable development and durable ecosystems. The human race and other living species have, therefore, plunged into an entangled calamity with respect to the environmental, economic, social, and technical facets of life, referred to as sustainability challenges. Sustainability researchers, such as Doppelt (2012) believed that these devastating problems continued to exist not because we lack efficient energy, technology, or policy guidelines, but because of our huge paucity of thinking in relation to human history. Our thinking, decisions, and actions will create either more scarcity and suffering or a future of greater abundance and higher quality of life. Human's “Take-Make-Waste” thinking as coined by Doppelt (2012), has aggravated atmospheric greenhouse gasses to a deadly echelon and guided the earth to the precipice of catastrophe.
Responding to these scary phenomena, sequence of conferences were organized while a number of charters and declarations were made, and outcomes were announced and published in form of action plans and policies. The salient among these is the World Commission of Environment and Development, also known as Brundtland Report which proffer a viable solution to these problems. The report coined a term “sustainable development,” and was defined as “development that meets the needs of the present without comprising the ability of the future generations to meet their own needs.” A common vital feature identified by the commission was education which was emphasized as a decisive instrument for advancing the sustainable development, as well as improving the ability of populace to tackle sustainability issues (United Nations Educational Scientific Cultural Organization, 2006; Cheah et al., 2012; Lozano et al., 2013). Accordingly, the United Nations (UN) pronounced 2005–2014 as the “UN decade for education for sustainable development” (DESD). The main object was to incorporate the philosophies, ideals and practices of sustainable development into all features of education and learning. This development warrants Higher Education Institutes (HEIs) to begin to mount sustainability programmes, and the field has already got significant institutional impetus over the years (Wiek et al., 2011). Moreover, Louw (2013) stated that several HEIs have started to implement a number of facets to that effect, such as signing a climate commitment, working towards plan to make their campuses climate-neutral, and making sustainability their guiding principles and top priorities. (Tilbury, 2011) stated that curriculum and pedagogy are at the heart of higher education experiences which require to be transformed if HEIs are to make a significant contribution to sustainable development. This is because HEIs have a range of possibilities to take on and promote sustainable human development. However, Elder (2009) posited that analogous modifications to the curriculum are lagging behind. Bauer et al. (2021) stated that: “In the face of current sustainability crises, the survival of global society depends on competencies that have so far been of little relevance due to longstanding ignorance of the consequences of our exploitative economic system” (p. 1). In line with this development, this paper therefore, validated technical sustainability competencies that were investigated in the earlier work (Mukhtar et al., 2020) conducted by the researchers, using both qualitative (document analysis and semi-structured interview), and quantitative (modified Delphi survey) methods with a fewer participants. The present study used the earlier findings to develop a questionnaire that was administered to a larger sample of the population, and subsequently used a Partial Least Square-Sequential Equation Modeling (PLS-SEM) approach in order to quantitatively validate the competencies. A model of proposed technical sustainability competences for integration into the Higher National Diploma (HND) electrical/electronic engineering curriculum in Nigeria was later formulated. Sufficient inclusion of technical sustainability competencies such as eco-design, life-cycle assessment, sustainable production, waste-to-energy technology, recycling, engineering ethics, and Occupational Safety Health (OSH) among others, into the said curriculum will help the graduates to be fully prepared to work in the manufacturing industries, and contribute in the uplifting of such industries in realizing their sustainability goals and that of the society and the country at large.
The literature review is discussed under three (3) subsections. First, we described the concept, emergence, and relevance of technical sustainability in engineering education. Second, we argued that competencies (knowledge, skills, and attitudes) in technical sustainability need to be identified and infused into the engineering curricula to promote manufacturing processes and industrial practices that are less invasive or destructive to the environment and society. We also stressed that the development of the proposed model requires being subjected to rigorous and diverse phases of validation procedures including sequential equation modeling (SEM) using the partial least squares (PLS) approach. Finally, we provide a detail explanation of the origin of the research that forms a clearly formulated research gap.
The concept of Sustainable Development (SD) generally has its roots in the Brundtland Report where it was referred to as “development that meets the needs of the present without compromising the ability of future generations to meet their own needs” (World Commission of Environment Development, 1987, p. 43). While Mihelcic et al. (2003) described sustainability as a design of human and industrial systems to ensure that humankind's use of natural resources and cycles does not lead to diminished quality of life due to either loss in further economic opportunities or adverse impacts on social conditions, human health and the environment. Three interlocking elements were also identified in the report as major constituents of SD, viz, environment, society, and economy. Several definitions, descriptions, and discussions on SD among scholars and policy makers are conducted based on the three pillar model of SD. A lot of researchers in non-engineering academic fields have advocated for the inclusion of other dimensions into the SD discourse. This includes cultural, institutional, judicial, and political sustainability (Spangenberg, 2002; Lähtinen and Myllyviita, 2015). However, researchers in engineering sustainability such as Rosen (2012) and Sianipar et al. (2013) have proposed a different pillar of sustainability which is termed ‘technical sustainability'. This is due to the inappropriateness of just 3 pillars of sustainability when engineers attempt to develop sustainable and efficient products, processes, and systems. Rosen (2012) argued that even though technical sustainability was not identified as an independent pillar of SD in the Brundtland Report, it is implicitly connected to each one.
The development of technical sustainability is paramount to adequately addressing climate and environmental problems facing the entire globe. Technical sustainability addresses a wide variety of mechanical and technical factors that constitute the design and manufacture of products. It promotes manufacturing processes and industrial practices that are less invasive or destructive to the environment, society, and economy and yields ideally, neutral or positive effects on these contexts. Kreith (2012) is of the opinion that technical sustainability stands high and that no subject is more vital to the engineering profession or the wider world that we live in. The pursuit of technical sustainability is imperative since its nature is worldwide. This is because every country in the world makes use of engineering services as well as exploits and consumes resources, which makes the environment to be the at the receiving end of the negative impacts of engineering processes (Rosen, 2012).
Technical sustainability as viewed by Rosen (2012) is the sustainable use of engineering in systems, which includes processes and technologies for reaping natural reserves, changing them to useful kinds, transportation and storage, and the use of engineering products and processes to afford useful services (p. 2,271). Hibbard (2009) provides us with six key steps to make engineering more sustainable: optimizing the use of fossil fuels, reducing or eliminating pollution, recycling, recovering energy, and saving time. Therefore, for efficient and sustainable engineering, industries and manufacturers alike must device means to reduce, reuse, and recycle materials and products. This is to say, wastes from one part of the system should be used as inputs to other parts of the system. Also, the extraction of mineral resources and the design of products and processes should be modified and improved to take the environment into consideration so as to avoid the ever-increasing depletion of the ozone layer. In the same vein, research on sustainable materials should be popularized among material scientists to replace traditional ones which help in reducing the environmental impact of their processing and application. Similarly, Information and Communication Technology (ICT) has the ability to change people's job approach and even their workplace, hence the nature of urban areas. ICT is increasingly modifying the methods that business and industrial ventures are run, which results in enhancing the effectiveness of all transportation systems, among other sectors of the economy. It is not the intention of this paper, to examine the essential factors for achieving engineering sustainability, but rather, the paper empirically investigates the suitable competencies (KSA) in technical sustainability that HND engineering graduates should acquire in order to prepare them for assisting in providing technical solutions to climate-related issues, and assessment of the relevance and applicability of carbon management standards in industries and manufacturing organizations.
Competency is a functionally linked complex of knowledge, skills, and attitudes that enable successful task performance and problem solving (Wiek et al., 2011). The United States Department of labor defined competency as “a cluster of related knowledge, skills, and abilities that affects a major part of one's job (a role or responsibility), that correlates with performance on the job, that can be measured against well-accepted standards, and that can be improved through training, development, and experience.” This definition implies that every job requires three-sided expertise for it to be accomplished. These are the theoretical cognition of the job (knowledge), the manual dexterity of executing the job (skills), and the ethics of the job (attitudes/values). It also implies that proficiency in any competency is achieved via constant practice, and has to be subjected to some standardized measurement procedures. Therefore, competency can be seen as the knowledge base (subject matter and content areas), the skills (techniques and abilities), and attitudes (personal approach and motivation) used in combination to perform a task.
Applied to sustainability, competency represents a complex and integrated set of knowledge, skills, abilities, attitudes, and values that people bring into play in different contexts (society, education, labor, and family) to address situations involving environmental, economic, social, and technical issues, as well as to act upon and transform reality according to sustainability criteria. For one to live sustainably, one requires to build up capacities to bring together a range of qualities, skills, knowledge, values, and dispositions which advance sustainability actions in a given perspective. In view of this, Wiek et al. (2011), define sustainability competencies as complexes of knowledge, skills, and attitudes that enable successful task performance and problem-solving with respect to real-world sustainability problems, challenges, and opportunities. Moreover, technical sustainability concentrates on a broad range of mechanical and technical issues that establishes the design and manufacture of products (Pappas, 2012). These include the scientific research and appropriate technology corroborating product design, function, and development; ease and efficiency of durable construction and use; maintenance and functioning capabilities that meet the objectives for which the product is designed; material selection and reduction, recovery, and reuse or disposal of parts of unused materials (Nagel et al., 2011). In view of this, competences that will help in preparing and effectively equipping the students to reasonably think and act in a sustainable manner, both in his/her work place or broader society are here referred to as “technical sustainability competences.” Technical sustainability competencies are, therefore, knowledge, skills, values, and dispositions about sustainable engineering, which is a practice that encourages, supports, and advances among others, technical sustainability via effective resource utilization, reduced emission of greenhouse gasses, as well as thought of the effects of novel technologies, procedures, and practices on the broader society. International Society of Sustainability Professionals (2010) reported that “technical expertise” came second in a survey carried out to identify top skills that students would need in order to be successful as sustainability professionals. Identifying, establishing, and developing a competency model has a long time root in human resource management (HRM). This is because, with a competency model and appraisal tools in place, corporations can clearly describe the expertises that are essential for every job as well as trace and detect the skills gaps within their employees, permitting the corporation to be governed more professionally. In support of this, Collin (1997) stressed the importance of establishing the competency of an individual and/or a competency model for an occupational role, as it is becoming an increasingly versatile and powerful tool in human resource management.
Sustainability challenges such as increase in CO2 emissions, deforestation, persistent increase in fossil fuels and biomass consumption, bushfires, use of inefficient technologies, water shortages, ecosystem loss, ocean acidification and sea level rise, disruption of food production and the food chain, more extreme weather events, and the spread of diseases occur as a result of normal systemic malfunction induced by overly complex and high-risk technologies and production systems. As a result, training the graduates to acquire adequate competences in technical sustainability is inevitable for minimizing the mentioned challenges. Nagel et al. (2011) and Hidalgo and Arjona Fuentes (2013) stressed that implementing changes in the curriculum to educate students in technical sustainability through infusing requisite competences is essential in order to produce graduates with higher-level cognitive and critical as well as problem solving skills, to supply sufficient solutions to complicated technical obstacles. Technical sustainability competences will enhance HND graduates' understanding of technical aspects of life through acquiring requisite competencies. This understanding will help them deal adequately with the future sustainability challenges in the workplace and the broader society. This requires the upcoming Twenty-first-century graduates of tertiary institutions to possess technical sustainability competencies such as innovative skills in problem-solving, and the ability for assessing the consequences of their actions outside the immediate walls of their professions.
Most of these sustainability challenges listed above are caused by the manufacturing industries that are the major employers of HND graduates (in the area of the study) and constitute the vast majority of businesses and form the economic backbone of the area in terms of employment, investment, and their overall contribution to GDP. Nevertheless, manufacturing industries are beyond doubt, the major contributors to pollution and climate change because they constitute mainly machines and other electrical/electronic equipment for their operations, and are driven or powered by fossil fuels and electricity. This is a reason why they are regarded as part of structures that contribute a lot to the emission of greenhouse gases which leads to environmental degradation and other sustainability problems. Furthermore, most of the natural resources used by the manufacturing industries are uncontrollably explored from the earth's crust without care for the environment and other life supporting systems. As such manufacturing industries are under increasing societal pressure to become more technically sustainable. One such approach is to equip the graduates, who are to become the employee, with requisite technical competences. For an HND graduate to be regarded as sustainability-literate, they have to acquire competences in technical sustainability that encourages, supports, and advances the technical context of sustainable development via effective resource utilization, lessening emission of greenhouse gases, as well as thought of the effects of novel technologies, procedures, and practices on broader society. The graduate also requires building up capacities to bring together a range of qualities, skills, knowledge, values, and dispositions which advance sustainability actions in a given perspective. Unfortunately, these challenges would continue to prevail in our societies unless necessary actions are taken from the grassroots, by way of ensuring that knowledge, skills, and attitudes (values) in technical sustainability are adequately entrenched in every level of the educational curricula.
As a prerequisite for tomorrow's electrical/electronic engineers to be able to work toward a sustainable future, they must be thoroughly trained in sustainable engineering through the acquisition of technical sustainability competencies, and this ultimately leads to sustainable thinking. Sustainable engineering education should be able to educate future engineers to think flexibly and to be adjustable because it is doubtful that their prospective careers will require them to work in one realm (Segalas et al., 2010; Nagel et al., 2011). Engineering is an innovative, resourceful, and integrated discipline; however, it is only in the last two decades that there has been a prevalent understanding of the philosophical and emergent effect engineering has on all sectors of society. Nagel et al. (2011) observed that it has come to be understood that the utmost and most urgent sustainability tribulations humanity faces are linked to our relationship with the natural world. As engineering transforms into an ever more assimilated field, engineers and engineering programs are expected to verify the suitable incorporation of sustainability into the curriculum. Sustainable engineering education is training that encourages, supports, and advances technical contexts of sustainability via effective resource utilization, lessening emission of greenhouse gases, as well as thought of the effects of novel technologies, procedures, and practices on the broader society. Engineering education takes up a very important task in dealing with those challenges as engineers provide relatively innovative technological solutions. This is an important reason why every electrical/electronic engineering student should not be left out in the training and acquisition of technical sustainability competencies.
Enormous literature exists that is devoted to sustainable education in Nigeria, including the empirical study that developed a conceptual model for integrating technical sustainability into Higher National Diploma (HND) electrical/electronic engineering curriculum by the present authors (Mukhtar et al., 2020). Although the data collection procedure for the developed model was carried out through both qualitative and quantitative methods, it still suffers certain deficiencies: Data were first collected through document analysis, and then through semi-structured interviews involving fewer participants. The quantitative section also used only 28 participants to collect data through three rounds of a modified Delphi survey (The first round survey emanated from the findings of the qualitative section). Perhaps the greatest criticism of the Delphi technique is its lack of perceived statistical rigor compared to purely quantitative research methods that are systematized and experimentally confirmed propositions of the natural sciences. Its lack of probability sampling, the impossibility of accounting for unseen events, and the absence of clearly defined procedures and processes for conducting Delphi studies are just some of the features that differentiate it from controlled scientific methodologies. In order to strengthen and clear the model away from these anomalies, there is a need to validate the findings of the earlier work. The authors, therefore, went to the field again and collected data from the larger sample of the respondents using a questionnaire emanating from the findings of the earlier work for more rigorous sequential equation modeling using Smart PLS 3. The authors presented to the larger sample, the questionnaire containing the competencies in technical sustainability as screened, rated, and confirmed in the earlier work. These larger samples were asked to go through the competencies, rate them, and re-confirmed their appropriateness or otherwise for inclusion into the HND electrical/electronic engineering curriculum in Nigeria. The authors used PLS-SEM in the analysis section primarily because the objective of the analysis is predictive in the sense that this study attempts to explain or predict the relationship between the exogenous and endogenous constructs. Also, PLS-SEM was opted for in this study, since the measurement philosophy in the model is by estimation with the composite factor model using a total variance.
The authors, in 2020, carried out and published an article titled “Conceptual Model of Technical Sustainability for Integration into Electrical/Electronic Engineering Programme in Nigerian Polytechnics.” This was published by IEEE in volume 8 of the 2020 publication (doi: 10.1109/ACCESS.2020.3002579). The earlier study was carried out through mixed-method adopting sequential exploratory design where data was first collected through document analysis and interview, and secondly using three-rounds of modified Delphi survey technique. As such, this study was delimited as only 28 participants were used in the collection of the quantitative data which was not subjected to rigorous data analysis.
The objective of this study, therefore, was to validate the earlier findings by using a structured questionnaire emanating from the said findings to collect data from more (at least 170) respondents in both academia and industry. The data were subjected to a more rigorous analysis using a PLS-SEM approach to validate the earlier findings.
This study builds on the earlier study (Mukhtar et al., 2020) carried out by the researchers with respect to the development of a conceptual model of technical sustainability, in which mixed-method research was used through sequential exploratory study. In the earlier study, the researchers collected data first through document analysis and interviews with a smaller sample of the population; and second, using a survey through three-rounds of modified Delphi technique with yet fewer participants. In the current study, the identified competencies (i.e., the findings of the first paper), were used to form a structured questionnaire and administered to a larger sample (172 subjects) of the population selected through a simple random sampling technique (Krejcie and Morgan, 1970). The development of the questionnaire in the study was streamlined by the objective of the research. Specifically, the questionnaire was constructed based on the findings of the earlier work conducted by the authors (i.e., Mukhtar et al., 2020), and consisted of 87 competencies (knowledge, skills, and attitudes) in technical sustainability (Refer to Appendix). Section A of the questionnaire solicited the personal status of the respondents. In Section B, which has 33 items (competencies), respondents were asked, based on their perceptions, to indicate the suitability or otherwise of the Technical Sustainability Knowledge (TSK) for inclusion into the curriculum. Section C contains 38 Technical Sustainability Skills (TSS), and respondents were asked to indicate those that are suitable for inclusion in the curriculum. Finally, Section D comprises 16 Technical Sustainability Attitudes (TSA), from which respondents were to indicate suitable competencies for inclusion in the curriculum.
Six experts (electrical engineers with at least 15 years of work experience from both academia and industry) validated the questionnaire by checking the face and content validation. The reliability of the instrument was established via a pilot test. The researchers administered the questionnaire to 36 experts in academia and industry in Bauchi state, Nigeria (not part of the study area). The research instrument's reliability was computed with the aid of SPSS statistical software version 20, using Cronbach's Alpha method to ascertain the extent of the homogeneity of the items. The results obtained show that the reliability coefficients for the sub-sections B–D were 0.852, 0.799, and 0.901, respectively. On the whole, the reliability coefficient of the questionnaire is 0.850 which indicates that the items in the questionnaire were internally consistent in measuring what was intended to be measured for the study. In establishing the extent of accuracy of the data collected, the researchers carried out a data screening exercise, where questionnaires having omitted value (s) or obvious errors were removed via skewness and kurtosis with the help of Smart PLS 3.0. Descriptive and inferential statistics were used to analyze the quantitative data. In particular, path modeling (PM) and structural equation modeling (SEM) with the aid of smart PLS 3.2.8 was used in analyzing the data.
Data collected was analyzed using descriptive and inferential statistics. We use descriptive statistics only to determine the demographic information considered in this study, i.e., the career of the respondents, which indicated that from the realized sample (n = 168), ninety (90) were lecturers in the polytechnics, while seventy-eight (78) were industry workers. All the respondents were graduates of electrical engineering with at least 10 years of work experience. Moreover, inferential statistics were also used with the help of smart PLS 3.2.8 in testing the hypothesized path relationships in the model.
To start with, the authors checked the data reliability using SPSS statistical software v.20. Usually Cronbach's Alpha is used to establish the reliability of survey data, and values vary between 0 indicating no reliability, and 1, indicating perfect reliability (Bryman, 2008). Alpha values of 0.897, 0.849, and 0.916 were obtained for Sections B–D respectively, whereas, an alpha value of 0.887 was obtained for the overall questionnaire indicating that the data is internally reliable. In addition, we also checked the univariate and multivariate normality of the data using skewness and kurtosis tests (https://webpower.psychstat.org/models/kurtosis). The results for the univariate normality showed that the ranges of skewness values for all variables are −0.7724 to −0.4833. While the ranges of kurtosis values for all variables are −0.8415 to +0.3497. However, the most important for this all is the multivariate normality. The researchers used Mardia's multivariate skewness and kurtosis to assess the multivariate normality. The results showed that Mardia's multivariate skewness and kurtosis are 6.006 and 41.000, respectively, which are above the cut-off point of Skewness ± 1; and Kurtosis ± 20. Therefore, the data in this study does not satisfy the assumptions of univariate and multivariate normality. Since the data does not meet the multivariate normality of Skewness ± 1; and Kurtosis ± 20 (Mardia, 1970), the researchers decided to use the smart PLS 3.0 for the regression (Hair et al., 2012).
Besides, we also checked the common method variance (CMV) of the data usually caused by self-reported biasness which Conway and Lance (2010) supposed to exaggerate relationships between variables. The researchers performed the “Harman one-factor-test” using SPSS to ascertain the degree of biasness in different proportion distributions of the items (Barker and Ong, 2016). Podsakoff and Organ (1986) infer that CMV is challenging if a single latent factor would account for the majority of explained variance (i.e., 50%), The researchers used un-rotated factor analysis and found that the one-factor accounted for 42.304% of the total variance; hence, the CMV was not a grave risk for the data. Factor analysis here compelled all the measurements into one single factor, and it emerges to be <50%, implying that CMV is not a drawback. This paved the way for the researchers to continue with the development of the measurement model as shown in Figure 1.
Figure 1. Partial least squares (PLS) algorithm showing indicators' loadings, paths' coefficients, and coefficient of determination (R2).
Wong (2016) refers to convergent validity as the model's ability to explain the indicator's variance. It is the extent to which a measure correlates positively with alternative measures of the same construct (Hair et al., 2017). Metrics that include outer loadings of the indicators, the indicators' reliability, and the average variance extracted (AVE) are mostly the considerations in establishing convergent validity.
Indicator loadings are the first metric to be evaluated to ascertain the validity of the model. We verify the indicator loadings and reliability to be certain that the associated indicators have much in common that is encapsulated by the latent construct (Wong, 2016). Hair et al. (2018) suggest that loading below 0.708 should be removed unless its removal will affect the content validity of the linked latent construct, or will not improve the AVE of the construct. Table 1 shows that 37 (75.51%) of the indicators surpassed the more strict cut-off verge (0.708) which means that more than 50% (0.7082) of the variance in the observed latent variable is assigned in the construct, while 12 (24.49%) of the indicators had loadings below 0.708, and the researcher decided to retain them in the study because Hulland (1999) recommends items' loadings of 0.4 and higher for exploratory study, coupled with their conceptual importance in the measurement model.
The AVE remains the only common measure to establish convergent validity on the construct's level. Hair et al. (2014), stated that the AVE is the grand mean value of the squared loadings of a set of indicators and is equivalent to the communality of a construct. Bagozzi and Yi (1988), recommend values of AVE to be 0.50 as a threshold level to provide evidence of convergent validity. An AVE of 0.50 or higher indicates that the construct explains 50% or more of the variance of the indicators that make up the construct (Hair et al., 2018). Table 1 shows the various AVEs values for the exogenous constructs involved in the path model. As can be seen in the table, the exogenous constructs of knowledge, skills, and attitudes have AVE values of 0.577, 0.534, and 0.619, respectively. Furthermore, the target endogenous construct in the model has an AVE value of 0.517. The researchers, therefore, concluded that the measures of all the endogenous constructs in the path model have significant levels of convergent validity.
Composite reliability and rho A values were used to establish the internal consistency reliability in the model. Rho A represents an estimated precise measure of construct's reliability, and mostly lies between Cronbach's alpha and composite reliability. Composite reliability varies between 0 and 1, with values approaching 1 signifying a high-level of reliability and vice-versa. Hair et al. (2017) suggested values of composite reliability (CR) between 0.7 and 0.9 as satisfactory to ascertain internal consistency. Table 1 shows the CR and rho A values of the constructs implying good internal consistency reliability in the constructs. The three exogenous constructs—knowledge skills and attitudes have CR and rho A values of 0.961 and 0.958, 0.966 and 0.964, and 0.890 and 0.848, respectively. Furthermore, the target endogenous construct in the model has CR and rho A values of 0.981 and 0.989, respectively.
Discriminant validity is the extent to which a construct is realistically distinct from another construct in the structural model (Hair et al., 2018). If discriminant validity is proved in a model, it suggests that a construct is distinctive and depicts phenomena that are not depicted by other constructs in the model. The researchers assessed the discriminant validity in the model using HTMT and Foenell-larcker criteria as shown in Tables 2, 3 below.
As shown in Tables 2, 3 above, the discriminant validity of the constructs is established. All the constructs in this study are considered conceptually similar. The researchers used a repeated indicator approach in the model estimation, which undoubtedly, makes some constructs have a value higher than the suggested threshold value.
The fit of the model was established by evaluating the following five metrics:
Hair et al. (2018) suggest that a value of VIF higher than five signifies likely collinearity issues among the predictor (or exogenous) constructs. The researchers assessed the collinearity issues by validating the VIF values of the exogenous constructs as shown in Table 4 below.
Following the running of a PLS algorithm, the path coefficient approximates are made available. These approximate correspond to the hypothesized relationships relating to the constructs. Path coefficient values are standardized on a range from −1 to +1, with coefficients closer to +1 denoting robust positive relationship and coefficients closer to −1 signifying vigorous negative relationship. Hair et al. (2017) stressed that even though, values near +1 or −1 are often statistically relevant, a standard error must be acquired using bootstrapping to test for significance. The bootstrap standard error allows calculating the empirical t-value. If the empirical t-value is greater than the critical value (in this study = 1.96 at p < 0.05, two-tailed), we conclude that the coefficient is significant at a 5% probability error. Figure 2 below shows the results of the PLS algorithm and the bootstrap results using 5,000 samples. Similarly, Table 5 shows the hypotheses testing for all the path relationships in the model.
Figure 2. Bootstrapping results showing the weight of indicators and empirical t-values using 5,000 samples.
Figure 2 and Table 5 show the hypothesized path relationships among the constructs in the model. Specifically, Table 5 reveals that the hypothesized path relationship between TSK and Technical Sustainability (H1) is statistically significant with a t-value of 28.228 and p < 0.05 at 0.376 and 0.431, with 95% confidence intervals for lower and upper levels, respectively. Similarly, the hypothesized path relationship between TSS and Technical Sustainability (H2) is found to be significant statistically. The t-value is 39.400 and p < 0.05 at 0.498, 95% confidence intervals for lower level, and 0.551, 95% confidence intervals for upper level. In the same vein, the path relationship was found to be significant statistically between TSA and Technical Sustainability (H3). The t-value was found to be 28.125 and p < 0.05 at 0.102 and 0.118, with 95% confidence intervals for lower and upper levels, respectively.
A vital element of structural model assessment is the estimation of the coefficient of determination (R2). Hair et al. (2017) affirmed that “this coefficient is a measure of the model's predictive accuracy and is calculated as the squared correlation between a specific endogenous construct's actual and predicted value” (p. 174). Hair et al. (2017) avowed that the R2-value varies from 0 to 1, with 1 implying absolute predictive accuracy and larger explanatory. The coefficient of determination, R2, for the target endogenous construct (Technical sustainability) is 1.00. This implies that the three exogenous constructs (TSK, TSS, and TSA) linked to it, extremely explained 100% of the variance in the context of sustainability.
When a particular exogenous construct is removed from a model and a PLS-SEM algorithm is run, the R2-value for the target endogenous construct changes. This change in R2-value can be used to assess whether the removed construct has a meaningful impact on the endogenous construct (Hair et al., 2017). This measure is termed the f2 effect size and is calculated as f2 = R2included-R2excluded/1–R2included
where: R2included and R2excluded are the R2-values of the endogenous construct when a particular exogenous construct is included in or excluded from the model. The effect sizes of the endogenous constructs were assessed as a result of the PLS algorithm and are shown below in Table 6.
The researchers evaluated the cross-validated redundancy measure, otherwise known as Stone-Geisser's Q2-value, which is evidence of the model's predictive relevance. The Q2-represents a measure of how well-observed values are reconstructed by the model and its parameter estimates (Chin, 1998). The blindfolding technique is used to get the Q2-value for a specified omission distance; D (in this case, D = 9). Table 6 above shows the predictive relevance of the endogenous constructs in the model which are all well above Cohen's suggestions for strong predictive relevance. We noted, as explained by Rigdon (2014) that, while comparing the Q2-value to zero is indicative of whether an endogenous construct can be predicted, it does not say anything about the quality of the prediction. As such, Hair et al. (2018) stated that similar to the f2 effect size method for evaluating R2-values, the relative effect of predictive relevance can be compared by means of the measure to the q2 effect. Table 6 above shows that TSK has the largest q2 effect size of 0.027, TSS has the second largest q2 effect size of 0.041, and lastly, TSA has the least q2 effect size of 0.001.
Consequently, the researchers formulated a validated model of the technical sustainability competences as shown in Figure 3 below.
Figure 3. Proposed model of technical sustainability for integration into HND electrical/electronic engineering curriculum in Nigeria.
Partial Least Square-Sequential Equation Modeling commonly functions upon the belief that the data being examined originate from a single population. However, as Sarstedt et al. (2011) observed, for several practical purposes, this belief of homogeneity is not viable, since people are liable to be heterogeneous in their opinions, understandings, and assessment of latent constructs. The researchers performed a multi-group analysis to uncover if there is a difference between the groups of respondents with respect to hypothesized path relationships in the model as shown below.
The bootstrapping result shown in Table 7 reveals that all the hypothesized path relationships in the model are also significant when data for each group is considered separately. To discover whether there is a significant difference between coefficients, one requires running a PLS-SEM multi-group analysis, which is a set of different methods for comparing PLS model estimates across groups of data. It is generally employed to examine variations between path coefficients in the inner model. The three sets of tests for multi-group analysis are shown below in Table 8.
Table 8 below shows all three tests for the multi-group analysis. The PLS-MGA test reveals that the hypothesized path relationships (H1, H2, and H3) are not significant. The parametric test shows that the hypothesized path relationship between TSA and Technical Sustainability (H1) is significant, while the hypothesized path relationships (H2 and H3) are not significant. Furthermore, the Welch-Satterthwait test confirmed the parametric test indicating a significant path relationship in H1 and no significant path relationships in H2 and H3.
The objective of this study was to validate identified competencies in technical sustainability suitable for incorporation into HND electrical/electronic engineering curriculum in Nigeria. The competencies were investigated in the earlier research conducted by the authors using both qualitative (document analysis and interview), and quantitative (Delphi survey) means, with fewer participants. This study then, administered questionnaires developed from the findings of the earlier research to a larger sample of the population. Consequently, the authors used PLS-SEM to validate the findings and formulate a more robust model of technical sustainability. Hence, the discussions of the findings are presented based on the three domains of learning a competency, i.e., knowledge, skills, and attitudes.
The findings of the study on knowledge of technical sustainability revealed a lot of competencies in this domain for inclusion in HND electrical/electronic engineering curriculum in Nigeria. These are discussed under the following: Eco-design and Life-Cycle Assessment, Research, Modeling, Simulation, and Optimization (MSO), and Recycling/Renewable resources.
The findings indicated that the experts stressed the importance of cognitive competencies in Eco-design and Life-Cycle Assessment for inclusion in the HND electrical/electronic engineering curriculum. Eco-design is the integration of environmental aspects into product design and development, with the aim of reducing adverse environmental impacts throughout the product life cycle (ISO 14006, 2011). This finding is consistent with who stated that eco-design has been recognized as a promising technique that is able to brace and reinforce the knowledge, skills, and attitudes of students in higher education, and therefore, evaluated whether eco-design related topics are fused in higher education courses and degree programs in Germany and some other countries. Furthermore, Favi et al. (2019) stated that eco-design methods needed to be more evident in the academic programs and scientific literature, lamenting the difficulty of its effective implementation within the technical departments in the industry. This finding also agrees with Pigosso and Leroy (2017) who reported that “Educating students (on Eco-design) seems to be more promising than changing industrial habits.” As such, Kattwinkel et al. (2018) concluded that a key success factor in integrating eco-design into the product development practice is the development of related competences among employees and designers through training and education. Romli et al. (2015) also supported this finding by stressing the benefits of competences in eco-design which include understanding of reducing the environmental impact and cost for the whole product life cycle. Konig (2021) also, stated that in the face of civilization's complex and existential sustainability challenges, there are urgent demands on science to be at the service of society.
Life-Cycle Assessment (LCA), on the other hand, is an eco-design tool that is able to assess the product's environmental performance through a life cycle perspective. Our findings matched the findings of Roure et al. (2018) who maintained that LCA is one of the sustainability concepts that can be integrated into many engineering programs to leave the “end of pipe” approach and enter a more pollution-prevention and holistic approach. This finding is concise with Bielefeldt (2013) who viewed LCA as a method of assessing the environmental impacts associated with a product or process from cradle-to-grave including raw material extraction and processing, manufacturing, transportation, use, upkeep, and disposal. In support of this, Corporation and Curran (2006) developed a guiding document to provide an introductory overview of LCA and describe the general uses and major components of LCA for the benefit of academia in learning how to incorporate environmental performance based on the life-cycle concept into their programs. This is good evidence that inculcating cognitive competencies in Eco-design and LCA to HND graduates will get them fully prepared to work in the manufacturing industries, whose focus now is on sustainable design and production. Their contributions as technicians and/or technologists will help in uplifting such industries and will manifest in societal growth and development. This indicates that there is a need for the HND electrical/electronic engineering curriculum developers in Nigeria to incorporate cognitive competencies in Eco-design and LCA in order to accelerate the achievement of sustainable development goals in the country.
The findings also revealed that the respondents agreed with the inclusion of cognitive competencies in modeling, simulation, and optimization (MSO) of electrical/electronic products, processes, and systems. Modeling is the understanding and predicting the functioning and performance of individual components of a system or the overall system behavior. Simulation involves solving the set of equations of mathematical models in order to determine the unknown variables so as to obtain key insights into the system's behavior. While optimization is determining the conditions of optimal system performance with respect to a particular objective which may be economical, environmental, social, or a combination of the three (Subramanian et al., 2018). This finding is in line with the study outcome of Vehmaa's et al. (2018) who emphasize the need to incorporate into engineering education competencies that include the use of certain software and modeling skills, as well as Geographic Information systems. The findings are also in agreement with Bielefeldt (2013) who maintained that the use of specialized software tools can be a valuable way to help students analyze technical sustainability aspects by assisting them in quantifying the various factors that relate to the sustainability of a project. It is with no doubt that acquiring adequate competency in using relevant software tools will enhance the HND electrical/electronic engineering graduates' understanding of modeling, simulation, and optimizing electrical/electronic products, processes, and systems. This will also help in equipping them with the necessary dispositions for working adequately in manufacturing industries and especially electrical energy companies. Effective understanding of modeling, simulation, and optimization of the energy system will aid in the sustainable production, transmission, and distribution of electrical energy to teeming end users and therefore, improve the living standards of the people, and also make society a better place.
Furthermore, the respondents in this study accepted competencies in research for inclusion in the curriculum of HND electrical/electronic engineering. This finding is not coming as a surprise because Carter et al. (2016) discovered that engineering students who engage in undergraduate research tend to report higher skill levels such as academic skills, knowledge of science, and research processes. Consistent with this finding is the study of Seymour et al. (2004) in which they stressed the benefits of undergraduate research as having a positive impact on students' interest in engineering majors, career preparation, critical thinking, self-efficacy, and self-confidence. They describe undergraduate research as “powerful affective, behavioral and personal-discovery experiences whose dimensions have profound significance for their emergent adult identity and sense of direction” (p. 531). The literature is rich with works suggesting that undergraduate research affects cognitive skills, and as such has become a bedrock of academic activity in colleges and universities (Carter et al., 2016). This finding stresses the need for HND electrical/electronic engineering graduates in Nigeria to acquire adequate dispositions in sustainability research and developments to enable them to match the requirements of industries and companies. These are dynamic organizations that rely on research and development for the ever-changing needs of technology, machines, materials, products, processes, and systems.
Moreover, the findings revealed that HND electrical/electronic engineering curriculum needs to incorporate cognitive competencies in the aspect of recycling and renewable resources. This finding confirms the assertion of Gupta et al. (2011) who stated that there are millions of green jobs in the labor market, but there are not enough workers who can design and build green buildings, design renewable energy systems, install and maintain solar panels and windmills, and make processes more efficient. For these reasons, they advocated for greening the engineering curricula. Supporting this finding are Behboodi et al. (2016) who emphasized the need for an accelerated step toward greening the engineering curricula by embedding competencies in renewable resources. This finding is also consistent with the study of Rajan et al. (2019) that found and reported that many higher education institutions have incorporated topics related to recycling and waste management into their curricula at different levels to increase awareness as well as to develop new recycling technologies. This is so important because recycling waste is a vital technique for addressing the crucial concerns associated with environmental pollution arising out of dumping waste into the municipal solid waste stream. Electrical/electronic engineering curriculum developers in Nigeria need to consider reviewing the curriculum to incorporate competencies in aspects of recycling and renewable resources because of the intense global focus on the rising costs of non-renewable energy sources and the negative impacts that our societies have on the global environment. This finding if implemented will help in cutting costs, mitigating risks, opening up new competitive and revenue opportunities, driving innovation, and improving employee development and retention, as well as helping Nigeria in achieving the sustainable development goals.
The findings of the study on skills in technical sustainability showed a lot of competencies are accepted by the research participants for inclusion in HND electrical/electronic engineering curriculum. We, therefore, discuss these competencies under the following: Communication/ICT; Sustainable production; Application of modern engineering software tools; Operating and troubleshooting of electrical engineering machines, devices, processes, and systems; and Skills in waste-to-energy technology.
The findings of the study revealed that communication/ICT competencies are accepted by the respondents for inclusion in HND electrical/electronic engineering curriculum. This finding corroborates the claims of Carter et al. (2016) who affirmed that in engineering, written, oral and graphic communication skills are inevitable because they are regarded as components of students' training for engineering practice. They stressed that technical communication courses should concentrate on the development of these skills and students frequently practice these skills in design courses through presentations to peers, faculty, and industry representatives. The finding is also in conformity with the study outcome of Kolmos et al. (2016) in which they reported that communication skills were highly recommended by engineering employers and graduates for inclusion in the new sustainability curriculum. The employers emphasize the need for engineering graduates to communicate effectively, listen, observe, speak, draw, and write; communicate results qualitatively, quantitatively, graphically, electronically, and textually; communicate processes of thinking and reflection including giving constructive feedback. Vehmaa's et al. (2018) study also justify this finding, because their discovery showed that soft skills, and in particular, communication skills are considered essential in an engineer's early career, and this includes information and communication technology (ICT) skills that make them good communicators. This point to the fact that integrating communication/ICT skills in the HND electrical/electronic engineering curriculum in Nigeria will help in preparing the students to meet up with the job requirements of the industries, and therefore assist in realizing the sustainability goals of the industries, the society and the country in large.
The study also found that competences in sustainable production are unanimously agreed upon by the participants for inclusion in HND electrical/electronic engineering curriculum in Nigeria. This finding is strengthened by the work of Raoufi et al. (2017) who noted that in view of the rising relevance of the need for sustainable production in manufacturing industries, engineering programs must educate students as the next generation of engineers by providing appropriate types of education. In line with this, Raoufi et al. (2018) said that engineering accreditation bodies were challenged to focus on the changes in the undergraduate curricula that would support sustainable production. The finding is also in harmony with the work of Blume et al. (2015) who stressed the significance of acquiring useable competencies in sustainable production engineering, which depends on a deep understanding of the fundamental principles as well as on practical skills. Hence engineering education directed at these complex topics needs adequate didactic measures and learning environments, or else the students barely accumulate factual knowledge. Adequate inclusion of competencies in sustainable production engineering in the HND electrical/electronic engineering curriculum will help the graduates with broad skills in sustainable production engineering to be able to assist manufacturers in achieving appropriate and lasting sustainable products and processes.
Furthermore, the findings of the study indicated that appropriate competencies in the application of engineering software tools are suitable for incorporation into HND electrical/electronic engineering curriculum in Nigeria. This finding is concise with the Networked European Software and Services Initiative (NESSI) that recommends all European universities modernize and meet the demands for basic and advanced software engineering skills and competencies (Bellavista, 2014). The white paper reported that engineering curricula across Europe need to address emerging technology trends, including cloud-based, data-intensive, CPS-oriented, and/or service-oriented systems through adequate software tools. Hussain and Hamid (2017) augmented this finding by maintaining that, for smooth entry into the Malaysian industries, engineering graduates are expected to be conversant with certain fundamental software tools needed in the industries. They stated that competency in using modern engineering tools and software is crucial in the working environment to ensure quality standards and to meet engineering specifications. Sarwar (2018) listed some of the electrical/electronic engineering software for graduates to be acquainted with. This includes MATLAB, Simulink, Pspice, Multisim, ETAP, Power World Simulator, PSCAD, PSS/E, LabVIEW, and Keil uVision to mention but a few. It is obvious that the inclusion of competencies in the application of electrical engineering software tools in the Nigerian HND electrical/electronic engineering curriculum is eminent because software tools have become ubiquitous in today's digital world. Industries and Companies are in dire need of employees with the requisite competencies to embed software in almost all kinds of modern products and services, since it is the key enabler for innovation.
The findings of this study also include the incorporation of competencies in operations and troubleshooting of electrical engineering machines, devices, processes, and systems into the HND electrical/electronic engineering curriculum in Nigeria. This finding is in line with the mission of the National Board for Technical Education NBTE (2001) of promoting the production of skilled technical manpower for the development and sustenance of the national economy. HND electrical/electronic engineering program in the polytechnics was designed to produce higher technicians in electrical/electronic engineering for the manufacturing, assembling, and service industries, instrumentation and control, power generation, transmission, distribution, and utilization industries. This finding is also positively related to the study of Mukhtar (2014) whose findings revealed that psychomotor competencies in constructing, installing, assembling, testing, and maintaining electrical machines, devices, systems, and equipment are approved by industry managers for inclusion in bachelor of engineering electrical/electronic curriculum in Nigeria. If this finding is implemented, the graduates will be conversant with the actual rigors of carrying out practical activities so that they help industries in the development, manufacture, and operations of a wide variety of electrical devices, circuits, systems, products, and equipment. This will, in turn, influence societal and national growth and development technologically, economically, socially, and environmentally.
Competencies in waste-to-energy technology were also identified by the respondents as suitable for inclusion in HND electrical/electronic engineering curriculum. This finding is not coming as a surprise due to Rada's et al. (2017) claim that globally 1.3–1.9 billion tones of solid waste is collected per year, and only 19% is recycled, 11% is used as energy recovery, and the rest ends up in landfills or dumps. Nevertheless, Alawin et al. (2016) identified a lack of awareness and shallow knowledge about renewable energy technologies among senior students in faculties of engineering. As a result, Robinson et al. (2018) called on higher education institutions to increase their effort in playing an influential role in providing technical solutions to climate-related issues, and assessment of the relevance and applicability of carbon management standards. This is an obvious indication that the inclusion of the competencies in waste-to-energy technology into the HND electrical/electronic engineering curriculum in Nigeria is paramount, and will help in preparing the graduates to be well-equipped with state-of-the-art expertise such as modern incineration and anaerobic digestion. This will yield environmental benefits, promotes financial savings, and increases competition.
Attitudes/Values are defined as favorable students' perspectives of sustainability issues (Stubbs, 2013). The findings of the study on attitudes toward technical sustainability demonstrated that values needed for integration into HND electrical/electronic engineering curriculum comprise Engineering ethical awareness, Occupational and safety health (OSH), and Inter-generational equity (Biasutti and Frate, 2017).
The finding of this study disclosed that affective values in engineering ethical awareness need to be captured into the HND electrical/electronic engineering curriculum. This finding is in cognizance with the work of Ali and Sinha (2016) who pointed out that the world presently is in a complete state of conflict and confusion, hatred and violence, war and rebellion, mainly due to the serious negligence of ethical values, which have not been adequately incorporated into education curricula. This is perhaps the reason why the Accreditation Board for Engineering and Technology (ABET) in the US requires engineering students to “acquire an understanding of professional and ethical responsibility” as well as other competences related to appreciating the engineer's role in society, and engineering's impact in the wider world (Newberry et al., 2011). The finding is also consistent with Biasutti and Frate (2017) who realized the importance of sustainability attitudes in higher education, and therefore, developed and validated a quantitative scale for measuring sustainable development attitudes based on four dimensions consisting of environment, economy, society, and education. Engineering ethics is the study of moral issues and decisions confronting individuals and organizations engaged in engineering. Incorporating it appropriately into HND electrical/electronic engineering curriculum will facilitate the transformation of the students into sustainability-literate graduates and contribute to increased professionalism, increased awareness of engineering's societal context, and thus allowing them to make educated, sustainability-informed decisions on the overall welfare of the public.
The findings also revealed that the respondents stressed the importance of embedding competencies in occupational safety and health (OSH) into the HND electrical/electronic engineering curriculum. This is perhaps due to the frequent work-related deaths caused by work place accidents and occupational diseases. Integrating competencies in OSH will save the industry millions of dollars in workers' compensation, medical costs, and work efficiency. In line with this, Tureková and Bagalová (2018) stressed that educational organizers must ensure occupational safety and hygiene, since this has an economic impact and educational attainment, in addition to humanistic nature. This is also consistent with Endroyo et al. (2015) who believed that to guard against industrial accidents, it becomes essential to enhance the knowledge, skills, attitudes, and habits of the people involved by adequately embedding safety education in the school curriculum. Daud et al. (2010) agreed with this finding and saw the need to, and subsequently identified threshold and differentiating competencies needed by OSH professionals for administrating and enforcing legislations related to OSH in Malaysia.
Findings of the study also showed that affective competencies related to intergenerational equity are worthwhile for integration into the HND electrical/electronic engineering curriculum. This finding is strongly supported by the World Commission of Environment Development (1987) report in which the concept of intergenerational equity was popularized: “… without compromising the ability of future generations to meet their own needs…” This implies equitable distribution of bio-spherical compatible improvements in human wellbeing both today and tomorrow (Van der Bank, 2014). This finding also coincides with the view of Stazyk et al. (2016) who stated that organizations (higher education institutions inclusive) striving to be socially and environmentally responsible would be well-served by treating sustainability as a form of intergenerational social equity or fairness. As such, when sustainability is conceptualized in intergenerational social equity, it is easier to determine what socially and environmentally responsible organizations look like in practice. Equity is a vital social notion in sustainable development discussion and is believed to be the nucleus of sustainability. As a result, when HND electrical/electronic engineering curriculum is adequately entrenched with values in intergenerational equity, the students will acquire skills that render them the opportunity to work and help industries in achieving their sustainability goals.
In consideration of the United Nations' appeal to higher institutions of learning to build systems for environmental and development education, provides cross-disciplinary courses to all students, and elevate quality and superiorities in interdisciplinary research and education, this study quantitatively validated technical sustainability competencies for incorporation into HND electrical/electronic engineering curriculum in Nigeria. Appropriate technical sustainability competencies which are adequately validated can undoubtedly be used to formulate a model for incorporation into the engineering curriculum. This will then ensure adequate production of the requisite and vital manpower to hasten the technological, environmental, and socio-economic growth and development of the nation, which will serve as a tool for societal transformation as well as economic development. The study found that cognitive competencies in Eco-design and Life-Cycle Assessment; Research; Modeling, Simulation, and Optimization (MSO); and Recycling/Renewable resources are suitable for incorporation into the curriculum. The study also discovered that skills in Communication/ICT; Sustainable production; Application of modern engineering software tools; Operating and troubleshooting of electrical engineering machines, devices, processes, and systems; and Skills in waste-to-energy technology are suitable for incorporation into the curriculum. Similarly, the study uncovered that affective values related to Engineering ethical awareness, Occupational, and safety health (OSH), and Inter-generational equity are suitable for incorporation into the curriculum. Therefore, the electrical/electronic engineering curriculum developers in Nigerian polytechnics and appropriate accreditation bodies should organize and coordinate the processes of implementing the validated model into the said curriculum. This will help the upcoming twenty-first-century engineers to possess positive sustainability thinking, innovative skills in problem-solving, and the ability for assessing the consequences of their actions outside immediate engineering walls.
The original contributions presented in the study are included in the article/supplementary materials, further inquiries can be directed to the corresponding author/s.
Ethical review and approval was not required for the study on human participants in accordance with the local legislation and institutional requirements. The patients/participants provided their written informed consent to participate in this study. Written informed consent was obtained from the individual(s) for the publication of any potentially identifiable images or data included in this article.
NM was responsible for the development of the introductory and methodology aspects of the articles as well as the actual field collection of the quantitative data. YK was responsible for the data analysis which involves the effective running of the software (SmartPLS), as well as interpretation of the results for both measurement and structural models. MS was responsible for the discussions of the findings as well as organizing and rearranging the references using EndNote. All authors contributed to the article and approved the submitted version.
This work was funded by UTM Encouragement Research Grant Reference No: PY/2021/01612; Cost Center No: Q.J130000.3853.20J37.
The authors declare that the research was conducted in the absence of any commercial or financial relationships that could be construed as a potential conflict of interest.
All claims expressed in this article are solely those of the authors and do not necessarily represent those of their affiliated organizations, or those of the publisher, the editors and the reviewers. Any product that may be evaluated in this article, or claim that may be made by its manufacturer, is not guaranteed or endorsed by the publisher.
The Supplementary Material for this article can be found online at: https://www.frontiersin.org/articles/10.3389/frsus.2022.841643/full#supplementary-material
Alawin, A. A., Rahmeh, T. A., Jaber, J. O., Loubani, S., Dalu, S. A., Awad, W., et al. (2016). Renewable energy education in engineering schools in Jordan: existing courses and level of awareness of senior students. Renew. Sust. Energy Rev. 65, 308–318. doi: 10.1016/j.rser.2016.07.003
Ali, Z., and Sinha, A. R. (2016). Integrating ethics in technical education for sustainable development. Purushartha J. Manage. Ethics Spirit. 9, 85–97.
Bagozzi, R. P., and Yi, Y. (1988). On the evaluation of structural equation models. J. Acad. Market. Sci. 16, 74–94. doi: 10.1007/BF02723327
Barker, R., and Ong, D. (2016). A measurement scale for students' usage of online networks. Perspect. Educ. 34, 1–18. doi: 10.18820/2519593X/pie.v34i2.1
Bauer, M., Rieckmann, M., Niedlich, S., and Bormann, I. (2021). Sustainability governance at higher education institutions: equipped to transform? Front. Sust. 2, 24. doi: 10.3389/frsus.2021.640458
Behboodi, S., Chassin, D. P., Crawford, C., and Djilali, N. (2016). Renewable resources portfolio optimization in the presence of demand response. Appl. Energy 162, 139–148. doi: 10.1016/j.apenergy.2015.10.074
Bellavista, P. (2014). Software Engineering Key Enabler for Innovation. NESSI Working Group: Networked European Software and Services Initiative. Brussels: White Paper.
Biasutti, M., and Frate, S. (2017). A validity and reliability study of the attitudes toward sustainable development scale. Environ. Educ. Res. 23, 214–230. doi: 10.1080/13504622.2016.1146660
Bielefeldt, A. (2013). Pedagogies to achieve sustainability learning outcomes in civil and environmental engineering students. Sustainability 5, 4479–4501. doi: 10.3390/su5104479
Blume, S., Madanchi, N., Böhme, S., Posselt, G., Thiede, S., and Herrmann, C. (2015). Die Lernfabrik–Research-based learning for sustainable production engineering. Proc. CIRP 32, 126–131. doi: 10.1016/j.procir.2015.02.113
Carter, D. F., Ro, H. K., Alcott, B., and Lattuca, L. R. (2016). Co-curricular connections: the role of undergraduate research experiences in promoting engineering students' communication, teamwork, and leadership skills. Res. High. Educ. 57, 363–393. doi: 10.1007/s11162-015-9386-7
Cheah, S.-M., Yang, K., and Sale, D. (2012). “Pedagogical approach to integrate sustainable development into engineering curriculum,” in Proceedings of the 8th International CDIO Conference (Brisbane).
Chin, W. W. (1998). “The partial least squares approach to structural equation modeling,” in Modern Methods for Business Research, ed G. A. Marcoulides (Mahwah, NJ: Erlbaum), 295–358.
Chu, B., Duncan, S., Papachristodoulou, A., and Hepburn, C. (2013).Analysis and control design of sustainable policies for greenhouse gas emission. Appl. Thermal Eng. 53, 420–431. doi: 10.1016/j.applthermaleng.2012.04.022
Collin, A. (1997). “Learning and development,” in Human Resource Management: A Contemporary Perspective, eds I. Beardwell, and L. Holden (Pitman, NJ; London: Pearson Education).
Conway, J. M., and Lance, C. E. (2010). What reviewers should expect from authors regarding common method bias in organisational research. J Bus Psychol. 25, 325–34. doi: 10.1007/s10869-010-9181-6
Corporation, S. A. I., and Curran, M. A. (2006). Life-Cycle Assessment: Principles and Practice: National Risk Management Research Laboratory. Cincinnati, OH: Office of Research and Development; U.S. Environmental Protection Agency.
Daud, R., Ismail, M., and Omar, Z. (2010). Identification of competencies for Malaysian occupational safety and health professionals. Ind. Health 48, 824–834. doi: 10.2486/indhealth.MS1115
Doppelt, B. (2012). The Power of Sustainable Thinking: “How to Create a Positive Future for the Climate, the Planet, Your Organization and Your Life.” London: Routledge.
Elder, J. L. (2009). Higher education and the clean energy, green economy. EDUCAUSE Rev. 44, 108–109. doi: 10.1111/j.2150-1092.2009.tb01483.x
Endroyo, B., Yuwono, B. E., and Mardapi, D. (2015). Model of learning/training of occupational safety & health (OSH) based on industry in the construction industry. Proc. Eng. 125, 83–88. doi: 10.1016/j.proeng.2015.11.013
Favi, C., Marconi, M., and Germani, M. (2019). Teaching eco-design by using LCA analysis of company's product portfolio: the case study of an Italian manufacturing firm. Proc. CIRP 80, 452–457. doi: 10.1016/j.procir.2019.01.032
Gupta, D., Athinarayanan, R., and Deken, B. J. (2011). “Development of green technology curriculum,” in American Society for Engineering Education (Missouri). doi: 10.18260/1-2–17775
Hair, J. F., Hult, G. T. M., Ringle, C. M., and Sarstedt, M. (2017). A Primer on Partial Least Squares Structural Equation Modeling (PLS-SEM), 2nd Edition. Thousand Oaks, CA: Sage.
Hair, J. F., Risher, J. J., Sarstedt, M., and Ringle, C. M. (2018). When to use and how to report the results of PLS-SEM. Euro. Bus. Rev. 31, 2–24. doi: 10.1108/EBR-11-2018-0203
Hair, J. F., Sarstedt, M., Hopkins, L., and Kuppelwieser, V. (2014). Partial least squares structural equation modeling (PLS-SEM) an emerging tool in business research. Euro. Bus. Rev. 26, 106–121. doi: 10.1108/EBR-10-2013-0128
Hair, J. F., Sarstedt, M., Ringle, C. M., and Mena, J. A. (2012). An assessment of the use of partial least squares structural equation modeling in marketing research. J. Acad. Market. Sci. 40, 414–433. doi: 10.1007/s11747-011-0261-6
Hibbard, S. W. (2009). Six key factors for achieving sustainable manufacturing. Foundry Manage. Technol. 137, 40.
Hidalgo, L. A., and Arjona Fuentes, J. M. (2013). The development of basic competencies for sustainability in higher education: An educational model. U. S. China Educ. Rev. 3, 447–458. Available online at: https://files.eric.ed.gov/fulltext/ED544120.pdf
Hulland, J. (1999). Use of partial least squares (PLS) in strategic management research: a review of four recent studies. Strat. Manage. J. 20, 195–204. doi: 10.1002/(SICI)1097-0266(199902)20:2<195::AID-SMJ13>3.0.CO;2-7
Hussain, I. S., and Hamid, F. A. (2017). “Development of technical skills in electrical power engineering students: a case study of power electronics as a key course,” in IOP Conference Series: Materials Science and Engineering (Melaka). doi: 10.1088/1757-899X/226/1/012197
International Society of Sustainability Professionals (2010). Making Sustainability Standard Practice. International Society of Sustainability Professionals. Retrieved from www.sustainabilityprofessionals.org
ISO 14006 (2011). Environmental Management Systems - Guidelines for Incorporating Eco-Design. London: British Standards Institute.
Kattwinkel, D., Song, Y.-W., and Bender, B. (2018). “Analysis of ecodesign and sustainable design in higher education,” in DS92: Proceedings of the DESIGN 2018 15th International Design Conference (Dubrovnik). doi: 10.21278/idc.2018.0305
Kolmos, A., Hadgraft, R. G., and Holgaard, J. E. (2016). Response strategies for curriculum change in engineering. Int. J. Technol. Des. Educ. 26, 391–411. doi: 10.1007/s10798-015-9319-y
Konig, A. (2021). Taking the complex dynamics of human–environment–technology systems seriously: a case study in doctoral education at the university of Luxembourg. Front. Sust. 2, 673033. doi: 10.3389/frsus.2021.673033
Krejcie, R. V., and Morgan, D. W. (1970). Determining sample size for research activities. Educ. Psychol. Measure. 30, 607–610.
Lähtinen, K., and Myllyviita, T. (2015). Cultural sustainability in reference to the global reporting initiative (GRI) guidelines: case forest bioenergy production in North Karelia, Finland. J. Cult. Herit. Manage. Sust. Dev. 5, 290–318. doi: 10.1108/JCHMSD-06-2013-0025
Lotz-Sisitka, H., Hlengwa, A., Ward, M., Salami, A., Ogbuigwe, A., Pradhan, M., et al. (2015). Mainstreaming Environment and Sustainability in African Universities: Stories of Change. Grahamstown: Rhodes University Environmental Learning Research Centre.
Louw, W. P. (2013). Green curriculum: sustainable learning in higher education. Int. Rev. Res. Open Distrib. Learn. 14, 1–15. doi: 10.19173/irrodl.v14i1.1310
Lozano, R., Lukman, R., Lozano, F. J., Huisingh, D., and Lambrechts, W. (2013). Declarations for sustainability in higher education: becoming better leaders, through addressing the university system. J. Clean. Prod. 48, 10–19. doi: 10.1016/j.jclepro.2011.10.006
Mardia, K. V. (1970). Measures of multivariate skewness and kurtosis with applications. Biometrika. 57, 519–530.
Mihelcic, J. R., Crittenden, J. C., Small, M. J., Shonnard, D. R., Hokanson, D. R., Zhang, Q., et al. (2003). Sustainability science and engineering: the emergence of a new meta-discipline. Environ. Sci. Technol. 37, 5314–5324. doi: 10.1021/es034605h
Mukhtar, N. (2014). Occupational skills required by electrical engineering graduates of universities for effective performance in industries of Kano and Kaduna States, Nigeria. (Unpublished M. TECH Thesis), Department of Industrial and Technology Education, Federal University of Technology, Minna (Nigeria).
Mukhtar, N., Kamin, Y. B., Saud, M. S. B., Al Rahmi, W. M., Nordin, M. S. B., Arsat, M. B., et al. (2020). Conceptual model of technical sustainability for integration into electrical/electronic engineering programmes in nigerian polytechnics. IEEE Access 8, 128519–128535. doi: 10.1109/ACCESS.2020.3002579
Nagel, J. K., Nagel, R. L., Pappas, E., and Pierrakos, O. (2011). “Integration of a client-based design project into the sophomore year,” in Paper Presented at the ASME 2012 International Design Engineering Technical Conferences and Computers and Information in Engineering Conference.
Newberry, B., Austin, K., Lawson, W., Gorsuch, G., and Darwin, T. (2011). Acclimating international graduate students to professional engineering ethics. Sci. Eng. Ethics 17, 171–194. doi: 10.1007/s11948-009-9178-6
Pappas, E. (2012). A new systems approach to sustainability: University responsibility for teaching sustainability in contexts. J. Sustain. Educ. 3, 3–18.
Pigosso, D. C. A., and Leroy, Y. (2017). “Follow up from workshop,” in ICED 2017, eds D. Marjanović, M. Štorga, S. Škec, N. Bojčetić, and N. Pavković (Vancouver, CA: Georgia Tech; BTH; DTU; the Design Society).
Podsakoff, P. M., and Organ, D. W. (1986). Self-reports in organizational research: problems and prospects. J. Manage. 12, 531–544. doi: 10.1177/014920638601200408
Rada, E. C., Cioca, L.-I., and Ionescu, G. (2017). “Energy recovery from Municipal solid waste in EU: proposals to assess the management performance under a circular economy perspective,” in MATEC Web of Conferences. doi: 10.1051/matecconf/201712105006
Rajan, K. P., Gopanna, A., and Thomas, S. P. (2019). A project based learning (PBL) approach involving PET recycling in chemical engineering education. Recycling 4, 10. doi: 10.3390/recycling4010010
Raoufi, K., Haapala, K. R., Jackson, K. L., Kim, K.-Y., Kremer, G. E. O., and Psenka, C. E. (2017). Enabling non-expert sustainable manufacturing process and supply chain analysis during the early product design phase. Proc. Manuf. 10, 1097–1108. doi: 10.1016/j.promfg.2017.07.100
Raoufi, K., Raman, A. S., Haapala, K. R., and Paul, B. K. (2018). Benchmarking undergraduate manufacturing engineering curricula in the United States. Proc. Manuf. 26, 1378–1387. doi: 10.1016/j.promfg.2018.07.114
Rigdon, E. E. (2014). Rethinking partial least squares path modeling: breaking chains and forging ahead. Long Range Plan. 47, 161–167. doi: 10.1016/j.lrp.2014.02.003
Robinson, O. J., Tewkesbury, A., Kemp, S., and Williams, I. D. (2018). Towards a universal carbon footprint standard: a case study of carbon management at universities. J. Clean. Product. 172, 4435–4455. doi: 10.1016/j.jclepro.2017.02.147
Romli, A., Prickett, P., Setchi, R., and Soe, S. (2015). Integrated eco-design decision-making for sustainable product development. Int. J. Product. Res. 53, 549–571. doi: 10.1080/00207543.2014.958593
Rosen, M. A. (2012). Engineering sustainability: a technical approach to sustainability. Sustainability 4, 2270–2292 doi: 10.3390/su4092270
Roure, B., Anand, C., Bisaillon, V., and Amor, B. (2018). Systematic curriculum integration of sustainable development using life cycle approaches: the case of the civil engineering department at the Université de Sherbrooke. Int. J. Sust. High. Educ. 19, 589–607 doi: 10.1108/IJSHE-07-2017-0111
Sarstedt, M., Henseler, J., and Ringle, C. M. (2011). “Multi-group analysis in partial least squares (PLS) path modeling: alternative methods and empirical results,” in Measurement and Research Methods in International Marketing, 195–218. doi: 10.1108/S1474-7979(2011)0000022012
Sarwar, M. (2018). Ten Must Learn Electrical Engineering Software for Every Electrical Engineer. Available online at: https://eepowerschool.com/softwares/10-must-learn-electrical-engineering-software (accessed August 6, 2019).
Segalas, J., Ferrer-Balas, D., and Mulder, K. F. (2010). What do engineering students learn in sustainability courses? The effect of the pedagogical approach. J. Clean. Prod. 18, 275–284. doi: 10.1016/j.jclepro.2009.09.012
Seymour, E., Hunter, A. B., Laursen, S. L., and DeAntoni, T. (2004). Establishing the benefits of research experiences for undergraduates in the sciences: first findings from a three-year study. Sci. Educ. 88, 493–534. doi: 10.1002/sce.10131
Sianipar, C. P. M., Kiyoshi, D., Gatot, Y., and Adhiutama, A. (2013). Seven pillars of survivability: appropriate technology with a human face. Euro. J. Sust. Dev. 2, 1–18. doi: 10.14207/ejsd.2013.v2n4p1
Spangenberg, J. H. (2002). Institutional sustainability indicators: an analysis of the institutions in Agenda 21 and a draft set of indicators for monitoring their effectivity. Sust. Dev. 10, 103–115. doi: 10.1002/sd.184
Stazyk, E. C., Moldavanova, A., and Frederickson, H. G. (2016). Sustainability, intergenerational social equity, and the socially responsible organization. Admin. Soc. 48, 655–682. doi: 10.1177/0095399713519094
Stubbs, W. (2013). Addressing the business-sustainability nexus in postgraduate education. Int. J. Sust. High. Educ. 14, 25–41, doi: 10.1108/14676371311288930
Subramanian, A., Gundersen, T., and Adams, T. (2018). Modeling and simulation of energy systems: a review. Processes 6, 238. doi: 10.3390/pr6120238
Tilbury, D. (2011). Higher education for sustainability: a global overview of commitment and progress. High. Educ. World 4, 18–28.
Tureková, I., and Bagalová, T. (2018). Knowledge and experiences of safety and health occupation risks among students. Int. J. Eng. Pedagogy 8, 108–120. doi: 10.3991/ijep.v8i5.8981
United Nations Educational Scientific and Cultural Organization (2006). United Nations Decade of Education for Sustainable Development: Reorienting Programmes. United Nations Educational Scientific and Cultural Organization. Retrieved from http://portal.unesco.org/education
Van der Bank, C. (2014). The fulfilment of South Africa's environmental right: sustainable development the fundamental building block. Mediterranean J. Soc. Sci. 5, 28. doi: 10.5901/mjss.2014.v5n25p28
Vehmaa, A., Karvinen, M., and Keskinen, M. (2018). Building a more sustainable society? A case study on the role of sustainable development in the education and early career of water and environmental engineers. Sustainability 10, 2605. doi: 10.3390/su10082605
Wiek, A., Withycombe, L., and Redman, C. L. (2011). Key competencies in sustainability: a reference framework for academic program development. Sust. Sci. 6, 203–218 doi: 10.1007/s11625-011-0132-6
Wong, K. K.-K. (2016). Mediation analysis, categorical moderation analysis, and higher-order constructs modeling in partial least squares structural equation modeling (PLS-SEM): a B2B example using SmartPLS. Market. Bull. 26, 1–22.
Keywords: Higher National Diploma, incorporation of sustainability, PLS-SEM approach, technical sustainability, competency model
Citation: Mukhtar N, Kamin YB and Saud MSB (2022) Quantitative validation of a proposed technical sustainability competency model: A PLS-SEM approach. Front. Sustain. 3:841643. doi: 10.3389/frsus.2022.841643
Received: 22 December 2021; Accepted: 12 September 2022;
Published: 26 October 2022.
Edited by:
Larisa Ivascu, Politehnica University of Timişoara, RomaniaReviewed by:
Bogdan Fleacă, University POLITEHNICA of Bucharest, RomaniaCopyright © 2022 Mukhtar, Kamin and Saud. This is an open-access article distributed under the terms of the Creative Commons Attribution License (CC BY). The use, distribution or reproduction in other forums is permitted, provided the original author(s) and the copyright owner(s) are credited and that the original publication in this journal is cited, in accordance with accepted academic practice. No use, distribution or reproduction is permitted which does not comply with these terms.
*Correspondence: Yusri Bin Kamin, cC15dXNyaUB1dG0ubXk=
Disclaimer: All claims expressed in this article are solely those of the authors and do not necessarily represent those of their affiliated organizations, or those of the publisher, the editors and the reviewers. Any product that may be evaluated in this article or claim that may be made by its manufacturer is not guaranteed or endorsed by the publisher.
Research integrity at Frontiers
Learn more about the work of our research integrity team to safeguard the quality of each article we publish.