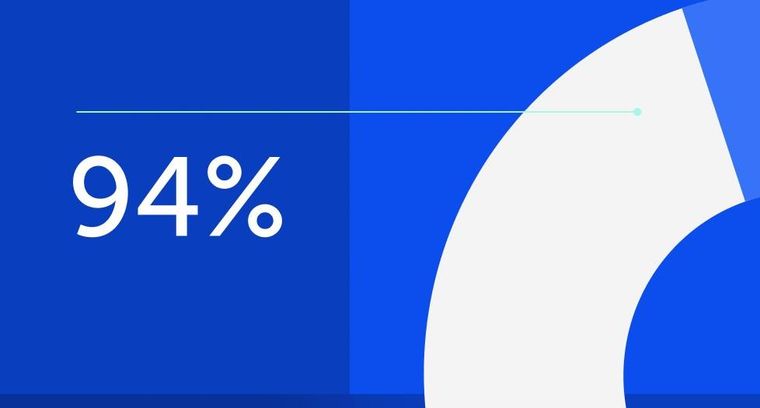
94% of researchers rate our articles as excellent or good
Learn more about the work of our research integrity team to safeguard the quality of each article we publish.
Find out more
MINI REVIEW article
Front. Sustain., 04 May 2022
Sec. Sustainable Consumption
Volume 3 - 2022 | https://doi.org/10.3389/frsus.2022.841106
This article is part of the Research TopicAchieving health equity: Sustainability of plant-based diets for human and planetary healthView all 9 articles
Evidence consistently suggests that plant-based diets promote human and planetary health. Reducing large-scale animal-based food production generates environmental benefits, as the entire livestock agriculture chain plays an outsized role in greenhouse gas emissions, land change and degradation, and scarcity-weighted water use. However, substituting animal products with their plant-based counterparts must come with consideration of the nutritional quality and resource usage of plant-based food production and processing operations. Several policy reforms have been implemented at the national, state, and municipal levels in the United States to support a transition toward more plant-based diets. Federal programs such as the Supplemental Nutrition Assistance Program and the Dietary Guidelines for Americans generally promote the consumption of unprocessed plant-based foods but include little to no information on sustainability and the harmful environmental impact of animal-based foods. National policies are complemented by state efforts aimed at incentivizing produce purchased from local suppliers and encouraging resource-conserving agriculture. At the local level, public schools are implementing programs to promote plant-based protein on their menus, and urban gardens are sprouting across the country to increase access to organic farming. This mini-review examines these policy reforms and behavioral intervention strategies, based on the social-ecological model, and discuss their capacity and limitations to promote a shift toward sustainably produced plant-based diets in the United States. We conclude that transforming the food systems toward plant-based diets in the animal-centered United States requires multi-sector collaboration and context-specific policy solutions to address diet-related climate concerns without neglecting health, social, and financial constraints.
Human nutrition and planetary health are deeply intertwined with food systems. Myriad studies demonstrate the significant potential of diets to both advance human health and environmental sustainability (Tilman and Clark, 2014; Aleksandrowicz et al., 2016; Alsaffar, 2016; Springmann et al., 2018; Clark et al., 2019; Eshel et al., 2019; Fresán and Sabaté, 2019; Hu et al., 2019; Willett et al., 2019; Jarmul et al., 2020; van Vliet et al., 2020). As more research on the stress that the current food system—particularly animal-based food production—places on the global environment comes into focus, food industry leaders, climate activists, and policymakers are driving change to promote diets rich in plant-sourced foods (Tziva et al., 2020).
According to the Academy of Nutrition and Dietetics, plant-based dietary patterns—also referred to as plant-forward diets—entirely exclude or substantially decrease animal product consumption while maximizing fruits, vegetables, whole grains, legumes, nuts, and seeds intake (Melina et al., 2016). Extensive research has associated plant-based diets with a lower risk of chronic diseases (Melina et al., 2016; Yokoyama et al., 2017; Johannesen et al., 2020; Tran et al., 2020). The potential biological mechanisms underlying those health effects are mainly associated with the lower energy density, appropriate saturated/unsaturated fat ratio, and high fiber and antioxidant content (particularly polyphenols, carotenoids, vitamin C, and vitamin E) of minimally processed plant-based diets (Satija and Hu, 2018). However, limited availability of healthy, plant-based foods in some latitudes, together with processing and manufacturing techniques that can strip plant-derived foods of nutritional quality, present barriers to increasing access to quality plant-based diets. The regular consumption of highly processed plant-based products, including sugar-sweetened beverages; snacks high in added sugars, refined starches, sodium, and/or saturated fats; and other energy-dense, hyper-palatable products is one example (Gallagher et al., 2021).
The well-established environmental benefits of decreasing animal-sourced food consumption have led to a rise in popularity of plant-based diets, particularly in developed nations (Fresán and Sabaté, 2019). Animal production and consumption are responsible for a wide variety of environmental burdens that threaten sustainability, including greenhouse gas emissions (GHGEs), land use and degradation, scarcity-weighted water use, nutrient pollution (e.g., acidification and eutrophication), use of fertilizers and pesticides, and consumer-level food waste through the entire product food chain (Magkos et al., 2020). Among these, GHGEs, land degradation, and water use are large-scale and upstream environmental impacts that exacerbate climate change and ultimately determine subsequent environmental deterioration (e.g., natural disaster propensity, habitat and biodiversity loss, freshwater scarcity) in food systems (Aleksandrowicz et al., 2016). As such, we selected these three impact indicators to succinctly capture system-wide threats to the sustainability of global food production, which itself necessitates the mitigation of climate change and efficient natural resource use (García-Oliveira et al., 2022). Conscious that agriculture (primarily for animal-based food production) is responsible for ~30% of GHGEs, 70% of water use, and exceeds the one-third occupation of potentially cultivatable land (Herrero et al., 2013; Smith et al., 2015; Whitmee et al., 2015), this review examines the sustainability of the United States (US) food system by discussing GHGEs, land degradation and implications in water supply–widely regarded as the leading dietary-related indicators of environmental impact.
The environmental analysis draws upon research from global contexts, as the implications of dietary behavior and the food system in the US has far-reaching climate implications. In light of the extensive evidence available, this narrative review aimed to summarize the most influential environmental effects of a food system reliant on animal-based food products, contextualize examples of public health policies supporting production and consumption of plant-based foods using the social-ecological model, and discuss factors that accelerate and hinder a shift toward sustainable plant-based diets for US consumers.
Sustainable diets are protective and respectful of biodiversity and ecosystems, culturally acceptable, accessible, economically fair and affordable, and nutritionally adequate. Land degradation, GHGs, and water use for food production are critical proxy metrics to evaluate dietary sustainability, as depicted in the following sections (Fanzo et al., 2012).
Extensive literature suggests that current levels of animal production are unsustainable in the global food system, resulting in irresponsible natural resource use and substantial environmental harms (Alsaffar, 2016; Fresán and Sabaté, 2019; Jarmul et al., 2020). The EAT-Lancet Commission estimated that animal-derived food production, especially red meat, is responsible for a large proportion of GHGEs in the food system, including carbon dioxide, methane, and nitrous oxide (Willett et al., 2019). An estimated 88% of emissions in the life cycle of animal products are generated on-farm, with only 12% derived from food system activities after agricultural production (e.g., processing, refrigeration, transport, retail, waste). Feed crop production activities, including synthetic fertilizer production, irresponsible land and soil management, and non-renewable energy use, are major sources of these emissions (Woods et al., 2010; Vibart et al., 2021). On-farm emissions produced by activities during livestock rearing include enteric fermentation and manure management. Together, environmental livestock production alone accounts for 14–51% of total anthropogenic GHGEs (Soret et al., 2014). Differences in the impact of certain animal products, however, do exist. Lamb production, for example, emits an estimated 39 kg of carbon dioxide equivalents per kilogram of food (kgCO2eq/kg) compared to lower levels for beef (27 kgCO2eq/kg), cheese (14 kgCO2eq/kg), and eggs (4.8 kgCO2eq/kg). Plant-based foods generally produce less GHGEs across their life cycles, including rice (4.1 kgCO2eq/kg), dried fruit (3.6 kgCO2eq/kg), almonds (2.1 kgCO2eq/kg), tofu (2.0 kgCO2eq/kg), avocados (0.9 kgCO2eq/kg), tomatoes (1.1 kgCO2eq/kg), and peanuts (0.6 kgCO2eq/kg) (Environmental Working Group, 2011; Mejia et al., 2018; Willett et al., 2019; Seltenrich, 2020).
Of note, relative differences in the total GHGEs of plant-based products are largely influenced by production, processing, and other post-farm-gate activities. For example, air-freighted fruits and vegetables significantly contribute to total energy use and CO2 emissions in food supply chains (Saunders and Hayes, 2007). Certain rice cultivation strategies (e.g., continuous flooding, nitrogen fertilizer use) and low-yielding varieties also emit extensive quantities of methane (Jiang et al., 2017; Wang et al., 2021). The farming and processing of nuts, seeds, and dried fruit sometimes requires greater energy inputs (e.g., fuel for irrigation, dehulling, and drying), material (e.g., fertilizer) and water resources than equivalent volumes of animal-derived foods (Eshel et al., 2019; Seltenrich, 2020). Thus, a reduction in meat consumption by itself may not necessarily lead to improvements in sustainability. Replacement of animal foods in the diet must be thoroughly evaluated according to the nature of the substituted product. This approach encompasses public and private initiatives to shift food consumption toward a sustainable diet with foods consistently associated with low environmental impacts (Hyland et al., 2017).
Land used as cropland or pastureland reaches about half of the ice-free land area of Earth, contributing to habitat clearance and species extinction (Tilman and Clark, 2014). Animal foods have particularly high ecological footprints, including milk and dairy products, and animal fat (Rosi et al., 2017). Estimations suggest that 0.85 ha/person (8,500 m2/person) are required annually to support an average global diet, with animal products accounting for as high as 87% of land use. Meat and fish alone account for almost half (44%) of land deterioration, largely due to terrestrial feed crop production for large-scale livestock and aquaculture operations (Davis et al., 2016; Rosi et al., 2017; Froehlich et al., 2018).
The US, a major global agricultural producer, contains approximately 391.5 M acres (1.58 M kilometers) of cropland, about a fifth of its total landmass and only 20% of which is used to grow food intended for direct human consumption; up to 42%, in contrast, is used for livestock feed or exports (Merrill and Leatherby, 2018). The high-intensity use of land for monoculture crop production results in a rapid rate of soil erosion and degradation, with about 90% of cropland losing soil at a rate 13 times above sustainable levels. US pastureland is similarly losing soil, with approximately 60% being overgrazed and subject to accelerated erosion (Baden et al., 2019). Using land for staple crops and livestock feed also implies carbon stock loss, with forest conversion (a proxy of deforestation) accounting for an estimated 2.9 Gt CO2 emissions (metric system) globally every year (Food Agriculture Organization of the United Nations, 2021).
Freshwater use refers to the consumption of surface water (e.g., lakes, rivers, streams, reservoirs) and groundwater (e.g., aquifers) (Springmann et al., 2018). US food production uses about 80% of the country's available freshwater supply, more than any other activity (Clark et al., 2019). The water required to produce various foods and forage crops ranges from 500 to 2,000 L of water per kilogram of food produced, with animal protein production requiring about 100 times more water than that of grain. Although livestock watering directly uses only 1.3% of total agricultural water use, the water required for forage and grain production dramatically increase water requirements (Pimentel and Pimentel, 2003).
In the US, critical water issues exacerbated by agricultural practices include the pollution of surface and groundwater sources, over-drafting of aquifers, waterlogging and salinization of soils, wetlands loss and runoff, evaporation, and leakage from irrigation systems (Marlow et al., 2009). Studies suggest that progressively replacing animal products with plant-based foods could increase freshwater use up to 16% due to water-demanding crops, such as legumes, vegetables, and fruits (Aleksandrowicz et al., 2016; Springmann et al., 2018). Specific foods, such as nuts, also result in higher scarcity-weighted water use compared to dairy, eggs, processed meat, and fish (Clark et al., 2019). Transitions to plant-based diets in the US will thus require careful consideration of freshwater availability as well as innovations to improve irrigation efficiencies and reduce water pollution in plant-based food production.
With an abundance of research demonstrating the harmful environmental impact of the current animal agriculture-based food systems, protein alternatives to large-scale animal food production continue to emerge. Substituting animal products with their plant-based counterparts must come with consideration of the adopted food's nutritional quality and resource requirements used in production and processing. Plant-based meat alternatives (PBMAs), or meat analogs, are designed to imitate properties of traditional animal meat products such as texture, taste, and appearance. Ingredients commonly found in PBMAs include soy, peas, coconut oil, and wheat gluten (Michel et al., 2021). A recent review suggests that PBMAs can potentially reduce global meat consumption, deliver adequate nutritional value, and confer environmental benefit as compared with its animal-meat counterpart (van Vliet et al., 2020). While improvements to flavor and appearance of PBMAs have bolstered demand for these products in recent years (Narayanan Nair, 2021), ambiguity surrounding the nutritional benefit of PBMAs, ultra-processed in particular, persists (Hu et al., 2019; He et al., 2020). Consumer acceptance of these products among the general population is mixed. Culture, taste, texture, convenience, and social and financial constraints may hinder the likelihood of consumers sampling and regularly incorporating PBMAs into the diet (Wyker and Davison, 2010). Availability of PBMAs is another consideration, as access to these products may be limited in lower- and middle-income communities due to marketing strategies of placement and distribution, costs, and customers' perception. This challenge underscores the need for a fundamental change in the food system that requires policies and actions to create a culture in which healthy and sustainable food choices are accessible and affordable to everyone (Hu et al., 2019; Jiang et al., 2020).
Though choosing to consume sustainably produced PBMAs in place of animal meat has potential for significant environmental preservation (Khan et al., 2019), more research is needed not only to produce impartial reports on environmental sustainability of production for emerging and established PBMA companies, but to recommend nutritional improvements. Indeed, given their highly processed nature and use of purified plant proteins that may lead to excess energy intake, the healthfulness of these products should be called into question. PBMAs are also typically elevated in sodium and saturated fat and lower in some micronutrients naturally present in plant foods (e.g., phytochemicals), which does not support a healthy diet (Hu et al., 2019).
Beyond PBMAs, improved access to and demand for nutritionally-sound and minimally processed plant protein sources (e.g., nuts, legumes, whole grains) must accompany a shift toward plant-based diets to improve human health and environmental sustainability. Importantly, all plant-based diets are not necessarily healthy. Studies show much variety in diet quality among non-meat eaters in the US; replacing animal-derived protein with unhealthy plant-based foods from refined grains, for example, may worsen diet-related health outcomes (Neff et al., 2018). Plant-based diet index scores had been developed to characterize the overall dietary quality according to evidence-based recommendations for disease prevention. The healthful plant-based diet index is based on whole grains, fruits, vegetables, nuts, legumes, vegetable oils, and tea/coffee, whereas the unhealthful plant-based diet index includes fruit juices, sugar-sweetened beverages, refined grains, potatoes, and sweets/desserts consistently associated with the risk of health impairment (Satija et al., 2016).
Policies to accelerate environmentally sustainable production of plant-based foods have emerged in recent years at the federal, state, and municipal level. Strategies including incentivizing production and consumption of sustainable plant foods, prohibiting excessive use of natural resources in production, and funding conservation agriculture technology are needed to address the environmental impacts of the current food system. No-till farming techniques, for example, promote carbon sequestration in the soil (Islam and Reeder, 2014), enriching the nutrient quality of harvests (Montgomery and Biklé, 2021) while preventing eutrophication through runoff (Kleinman et al., 2015). Investments in low-resource crops such as legumes promote water conservation and preserve farmland, as they occupy minimal arable land; as such, production of these crops, consumed directly as food for humans instead of animal feed, can be combined with decreases in livestock grazing land to limit land use changes and preserve natural environments. Many species' root systems also efficiently facilitate water retention in the soil, diminishing excess water use and preserving soil health (Rubiales and Mikic, 2015; Stagnari et al., 2017; Ferreira et al., 2021).
Much evidence indicates that solutions to reduce the environmental impact of food production and augment sustainability require multi-sector collaboration, and context-specific policy solutions are needed across food systems sectors to adequately address diet-related climate concerns. While this review contextualizes intervention strategies in the US, there is great need to align domestic food systems initiatives with global frameworks. The United Nations Sustainable Development Goals are a collection of 17 goals that address interrelated global challenges including poverty, inequality, climate change, and environmental degradation (United Nations Department of Economic Social Affairs, 2015). This urgent appeal has spurred nations into action around climate-smart agriculture and advancing plant-based, sustainable diets (Food Agriculture Organization of the United Nations, 2019; European Commission, 2020). This review discusses existing national, state, and municipal policies in the US around food systems and sustainability using the social-ecological framework (Figure 1). Public health professionals must consider the range of interdependent policies that impact our food system and work together to improve human and planetary health.
Figure 1. National, state, and municipal policies in the US addressing food systems and sustainability according to the social-ecological model.
Several US federal programs, such as the Supplemental Nutrition Assistance Program (SNAP), SNAP-Ed (an accompanying educational program with cooking, grocery shopping, and nutritional literacy curricula), and Eat Smart, Live Strong (a nutrition education program for older adults) generally promote fruit and vegetable consumption to their more than 40 million participants (Committee on Examination of the Adequacy of Food Resources SNAP Allotments, 2013). However, these federal nutrition programs do not explicitly discuss benefits of sustainable plant foods, nor the environmental concerns that arise with current reliance on animal products. Further, despite mounting evidence of the link between diet and environmental sustainability (Willett et al., 2019), the Dietary Guidelines for Americans Advisory Committee excluded sustainability as a topic of interest in the 2020 report (U.S. Department of Agriculture, 2017). A 2021 executive order establishing a National Climate Task Force to “increase resilience to the impacts of climate change, protect public health, conserve our lands, waters, and biodiversity” failed to mention the negative impact animal agriculture has on the planet and the positive impact that diets rich in plants can have on the environment (The White House, 2021). Omitted discussion on the sustainability of foods are a missed opportunity to empower consumers with education about environmentally responsible food choices.
Given the nutrition community's recommendations of a greater emphasis on plant foods in our diets (Intergovernmental Panel on Climate Change, 2018), the intersection of nutrition and sustainability is exceedingly relevant information to include in population-level dietary guidelines and education. However, we cannot rely solely on reframing federal nutrition education to fix our food system, as these policy reforms alone may not inspire change that reflects the urgency of environmental and human health crises (National Academies of Sciences, 2019; Bowman, 2020). It has been suggested to the United States Department of Agriculture Farm Bill to increase investment in resource-conserving agriculture and to limit the expansion of large-scale livestock operations (Dewey et al., 2017; Miller, 2017). Bold messaging is needed to explicitly promote sustainable, plant-based diets and must be brought to the forefront in these federal food programs with a multifaceted agricultural and consumer educational approach.
While food systems reform on the federal level has moved at a slower pace (Muller et al., 2009), state and municipal governance typically have more agility to enact climate- and food-related policies.
The US state of Massachusetts, for example, offers the Healthy Incentives Program (HIP) which reimburses SNAP participants when they purchase fruits and vegetables from local retailers including farmers' markets, community-supported agriculture programs, farm stands, and mobile markets (Commonwealth of Massachusetts, 2021). Program evaluation for the Massachusetts HIP show that more than 165,000 individuals increased their fruit and vegetable intake by one serving per day in between 2017 and 2021 (Commonwealth of Massachusetts, 2021). Of note, this increase does not necessarily imply a direct reduction in animal food consumption; however, this policy encourages behavior change that decreases demand for resource-intensive agriculture practices while increasing demand of healthy foods from local producers, representing a strong investment in health and nutrition for low-income consumers and local producers. Furthermore, local retail operations that provide food for programs like HIP are better positioned than conventional agriculture to mitigate the effects of climate change as they motivate positive behavioral changes and, thus, reduce demand for intensive agricultural practices (Goh, 2011).
The US state of California has also demonstrated innovation in promoting environmentally responsible plant food production in rural agricultural settings. Assembly Bill 1289, entitled “Smart Climate Agriculture Program: Plant-Based Agriculture,” aims to support small and mid-sized farmers who wish to transition their lands from livestock farming to low-resource plant-based agriculture, excluding crop production for livestock feed. If passed, the bill will provide technical assistance and best practices for transitioning farmland to participating farmers (California Legislature, 2010). While this initiative does not explicitly promote the consumption of plant foods, it represents a substantial commitment to sustainable diets by incentivizing the production of low-resource plant foods instead of high-resource animal food farming.
The C40 Good Food Cities Declaration is a coalition of cities worldwide to increase planetary health diets in the next 20 years (C40 CITIES, 2019). Los Angeles, California, the only US city to adopt this climate leadership initiative, has promised to leverage public property for plant-based, urban agriculture by increasing the number of produce gardens in parks and public libraries by 50% by 2021 (C40 CITIES, 2019). While urban farming is limited in scale and is not the sole solution to ensuring availability of nutritious and environmentally-sound food, this demonstrates an innovative and promising commitment to sustainable plant food production.
In efforts to boost the number of nutritious, sustainable lunch options in public schools, Sacramento passed the California School Plant-Based Food and Beverage Program in 2019 that incentivizes plant-based food in schools and provides additional funding to schools serving a plant-based entrée and non-dairy milk (California Legislautre, 2019). Though schools across the country are interested in increasing plant-based options, districts face cost barriers as animal-based foods and cow milk are more heavily federally subsidized than plant-based options (Sewell, 2020). Funding these initiatives will entail large-scale government buy-in for schools to be able to afford the high costs.
Behavior intervention strategies promise to be effective tools to nudge consumers toward plant-based food choices. A study performed in three university cafeterias, for example, showed that doubling the proportion of vegetarian meals offered increased sales of between 41% and 79%, indicating that food availability and substitution may be an effective strategy for increasing consumption of plant-based meals (Garnett et al., 2019). Another study measuring food choices in a university cafeteria demonstrated that taste-focused labeling of vegetables in dining halls increased vegetable consumption by 39%, compared to health-centered labeling of the same dish (Turnwald et al., 2017), adding to a growing body of evidence indicating that menu design and labeling can influence consumer acceptance (Vadiveloo et al., 2017). This compelling research suggests that enhancing the variety and availability of vegetarian dishes and using messaging that emphasizes indulgent flavor and culinary technique may be effective behavioral nudging strategies to increase consumption of plant-based foods in school or food establishment settings.
While these outcomes encourage choice architecture innovation to nudge consumers toward healthier choices (Ensaff et al., 2015; Mikic, 2020), behavioral interventions have limitations. For instance, campaigns promoting environmentally responsible consumption, labeling systems addressing ecological footprint, and other interventions targeting customers' food conduct are generally less effective than fiscal tools, advertising bans, and other restrictive market regulations (National Academies of Sciences, 2019). Focusing on individual-centered interventions rather than population-scale measurements has questioned the social-ecological validity of public strategies based on behavioral theory. The remaining gaps in the chain of evidence from small-scale controlled efficacy trials to population frameworks should be addressed to interpret behavioral interventions' effectiveness and generalizability (Hagger and Weed, 2019).
The environmental implications of large-scale livestock farming are too pressing to ignore. While both the increasing climate-related challenges that farmers face and mounting concerns about diet-related chronic disease, we need multidisciplinary collaboration between public and private sectors. Policy reform at the societal and community levels and behavioral intervention strategies at the interpersonal and individual levels have the potential to reshape our food system and support a shift toward more sustainably produced plant-based diets in the US. Because these layers interact to influence individual behavior, public health strategies must be intersectional and coordinated across multiple sectors. Continued research and development investments in consumer behavior, sustainable agriculture, and nutritional value of diets high in plant foods and low in animal foods are essential to inform policy decisions for a sustainable future.
AE-M, KA, AC-A, and LS conceptualized the manuscript, performed the literature search and manuscript drafting. JM, SB, AM, and MT supervised and revised the manuscript. All authors contributed to the article and approved the submitted version.
This project was supported by scholarships from the Mexican Council of Science and Technology (Spanish acronym: CONACYT), Fundacion Mexico en Harvard, and Harvard University provided to AE-M. Additionally, AC-A received funding from Fundacion Mexico en Harvard. Funders had no role in the study design, interpretation, preparation, review, approval, or decision to submit this review for publication.
The authors declare that the research was conducted in the absence of any commercial or financial relationships that could be construed as a potential conflict of interest.
All claims expressed in this article are solely those of the authors and do not necessarily represent those of their affiliated organizations, or those of the publisher, the editors and the reviewers. Any product that may be evaluated in this article, or claim that may be made by its manufacturer, is not guaranteed or endorsed by the publisher.
The authors wish to thank Rushabh Doshi of the Harvard TH Chan School of Public Health for contributing to this project. The authors also appreciate the comments and feedback from colleagues from the 2020 Introduction to Nutrition in Public Health course at Harvard T.H. Chan School of Public Health.
Aleksandrowicz, L., Green, R., Joy, E. J. M., Smith, P., and Haines, A. (2016). The impacts of dietary change on greenhouse gas emissions, land use, water use, and health: a systematic review. PLoS ONE 11, e0165797. doi: 10.1371/journal.pone.0165797
Alsaffar, A. A. (2016). Sustainable diets: the interaction between food industry, nutrition, health and the environment. Food Sci. Technol. Int. 22, 102–111. doi: 10.1177/1082013215572029
Baden, M. Y., Liu, G., Satija, A., Li, Y., Sun, Q., Fung, T. T., et al. (2019). Changes in plant-based diet quality and total and cause-specific mortality. Circulation 140, 979–991. doi: 10.1161/CIRCULATIONAHA.119.041014
Bowman, S. A. (2020). A vegetarian-style dietary pattern is associated with lower energy, saturated fat, and sodium intakes; and higher whole grains, legumes, nuts, and soy intakes by adults: National Health and Nutrition Examination Surveys 2013–2016. Nutrients 12, 2668. doi: 10.3390/nu12092668
C40 Cities Climate Leadership Group (2019). C40 Good Food Cities Declaration Planned Actions To Deliver Commitments. Available online at: https://www.c40knowledgehub.org/s/article/The-C40-Good-Food-Cities-Declaration-Planned-actions-to-deliver-commitments?language=en_US (accessed December 18, 2021).
California Legislature (2010). Assembly Bill No. 1289. Available online at: http://www.leginfo.ca.gov/pub/09-10/bill/asm/ab_2251-2300/ab_2254_bill_20100218_introduced.pdf (accessed September 6, 2021).
California Legislautre (2019). School Meals: Plant-Based Food and Milk Options: California School Plant-Based Food and Beverage Program. Available online at: http://www.leginfo.legislature.ca.gov/faces/billNavClient.xhtml?bill_id=201920200AB479
Clark, M. A., Springmann, M., Hill, J., and Tilman, D. (2019). Multiple health and environmental impacts of foods. Proc. Natl. Acad. Sci. 116, 23357–23362. doi: 10.1073/pnas.1906908116
Committee on Examination of the Adequacy of Food Resources and SNAP Allotments Food and Nutrition Board, Committee on National Statistics, Institute of Medicine, National Research Council. (2013). “Supplemental Nutrition Assistance Program: Examining the Evidence to Define Benefit Adequacy,” in History, Background, and Goals of the Supplemental Nutrition Assistance Program (Washington, DC). Available online at: www.ncbi.nlm.nih.gov/books/NBK206907/
Commonwealth of Massachusetts (2021). Massachusetts Healthy Incentives Program (HIP). Available online at: https://www.mass.gov/service-details/massachusetts-healthy-incentives-program-hip (accessed October 1, 2021).
Davis, K. F., Gephart, J. A., Emery, K. A., Leach, A. M., Galloway, J. N., and D'Odorico, P. (2016). Meeting future food demand with current agricultural resources. Glob. Environ. Chang. 39, 125-132. doi: 10.1016/j.gloenvcha.2016.05.004
Dewey, S., Hanson, L., and Horan, C. (2017). Opportunities to Address Climate Change in the Next Farm Bill. Available online at: http://environment.law.harvard.edu/emmett-clinic/%0A (accessed October 30, 2021).
Ensaff, H., Homer, M., Sahota, P., Braybrook, D., Coan, S., and McLeod, H. (2015). Food choice architecture: an intervention in a secondary school and its impact on students' plant-based food choices. Nutrients 7, 4426–4437. doi: 10.3390/nu7064426
Environmental Working Group (2011). Meat Eaters Guide, Methodology & Results. Available online at: https://static.ewg.org/reports/2011/meateaters/pdf/methodology_ewg_meat_eaters_guide_to_health_and_climate_2011.pdf?_ga=2.111105989.1198169940.1648162231-538380204.1648162231 (accessed October 13, 2021).
Eshel, G., Stainier, P., Shepon, A., and Swaminathan, A. (2019). Environmentally optimal, nutritionally sound, protein and energy conserving plant based alternatives to U.S. Meat. Sci. Rep. 9, 10345. doi: 10.1038/s41598-019-46590-1
European Commission (2020). Communication From the Commission to the European Parliament, the Council, the European Economic and Social Committee and the Committee of the Regions. Available online at: http://jurnal.globalhealthsciencegroup.com/index.php/JPPP/article/download/83/65%0A (accessed November 11, 2021).
Fanzo, J., Cogill, B., and Mattei, F. (2012). Metrics of Sustainable Diets and Food Systems. Rome: Bioversity International. 1–8.
Ferreira, H., Pinto, E., and Vasconcelos, M. W. (2021). Legumes as a cornerstone of the transition toward more sustainable agri-food systems and diets in Europe. Front. Sustain. Food Syst. 5, 694121. doi: 10.3389/fsufs.2021.694121
Food Agriculture Organization of the United Nations (2019). Climate-Smart Agriculture and the Sustainable Development Goals: Mapping Interlinkages, Synergies and Trade-Offs and Guidelines for Integrated Implementation. Available online at: http://www.fao.org/climate-smart-agriculture/resources/publications/en/ (accessed December 7, 2021).
Food and Agriculture Organization of the United Nations (2021). Forest Land Emissions and Removals. Global, Regional and Country Trends 1990-2019. Rome: FAO.
Fresán, U., and Sabaté, J. (2019). Vegetarian diets: planetary health and its alignment with human health. Adv. Nutr. 10, S380–S388. doi: 10.1093/advances/nmz019
Froehlich, H. E., Runge, C. A., Gentry, R. R., Gaines, S. D., and Halpern, B. S. (2018). Comparative terrestrial feed and land use of an aquaculture-dominant world. Proc. Natl. Acad. Sci. 115, 5295–5300. doi: 10.1073/pnas.1801692115
Gallagher, C. T., Hanley, P., and Lane, K. E. (2021). Pattern analysis of vegan eating reveals healthy and unhealthy patterns within the vegan diet. Public Health Nutr. doi: 10.1017/S136898002100197X. [Epub ahead of print].
García-Oliveira, P., Fraga-Corral, M., Pereira, A. G., Prieto, M. A., and Simal-Gandara, J. (2022). Solutions for the sustainability of the food production and consumption system. Crit. Rev. Food Sci. Nutr. 62, 1765–1781. doi: 10.1080/10408398.2020.1847028
Garnett, E. E., Balmford, A., Sandbrook, C., Pilling, M. A., and Marteau, T. M. (2019). Impact of increasing vegetarian availability on meal selection and sales in cafeterias. Proc. Natl. Acad. Sci. 116, 20923–20929. doi: 10.1073/pnas.1907207116
Goh, K. M. (2011). Greater mitigation of climate change by organic than conventional agriculture: a review. Biol. Agric. Hortic. 27, 205–229. doi: 10.1080/01448765.2011.9756648
Hagger, M. S., and Weed, M. (2019). DEBATE: do interventions based on behavioral theory work in the real world? Int. J. Behav. Nutr. Phys. Act. 16, 36. doi: 10.1186/s12966-019-0795-4
He, J., Evans, N. M., Liu, H., and Shao, S. (2020). A review of research on plant-based meat alternatives: Driving forces, history, manufacturing, and consumer attitudes. Compr. Rev. Food Sci. Food Saf. 19, 2639–2656. doi: 10.1111/1541-4337.12610
Herrero, M., Havlík, P., Valin, H., Notenbaert, A., Rufino, M. C., Thornton, P. K., et al. (2013). Biomass use, production, feed efficiencies, and greenhouse gas emissions from global livestock systems. Proc. Natl. Acad. Sci. 110, 20888–20893. doi: 10.1073/pnas.1308149110
Hu, F. B., Otis, B. O., and McCarthy, G. (2019). Can plant-based meat alternatives be part of a healthy and sustainable diet? JAMA 322, 1547. doi: 10.1001/jama.2019.13187
Hyland, J. J., Henchion, M., McCarthy, M., and McCarthy, S. N. (2017). The role of meat in strategies to achieve a sustainable diet lower in greenhouse gas emissions: a review. Meat Sci. 132, 189–195. doi: 10.1016/j.meatsci.2017.04.014
Intergovernmental Panel on Climate Change (2018). Climate Change and Land. Geneva: Intergovernmental Panel on Climate Change.
Islam, R., and Reeder, R. (2014). No-till and conservation agriculture in the United States: an example from the David Brandt farm, Carroll, Ohio. Int. Soil Water Conserv. Res. 2, 97–107. doi: 10.1016/S2095-6339(15)30017-4
Jarmul, S., Dangour, A. D., Green, R., Liew, Z., Haines, A., and Scheelbeek, P. F. (2020). Climate change mitigation through dietary change: a systematic review of empirical and modelling studies on the environmental footprints and health effects of ‘sustainable diets'. Environ. Res. Lett. 15, 123014. doi: 10.1088/1748-9326/abc2f7
Jiang, G., Ameer, K., Kim, H., Lee, E. J., Ramachandraiah, K., and Hong, G. P. (2020). Strategies for sustainable substitution of livestock meat. Foods. 9, 1227. doi: 10.3390/foods9091227
Jiang, Y., van Groenigen, K. J., Huang, S., Hungate, B. A., van Kessel, C., Hu, S., et al. (2017). Higher yields and lower methane emissions with new rice cultivars. Glob. Chang. Biol. 23, 4728–4738. doi: 10.1111/gcb.13737
Johannesen, C. O., Dale, H. F., Jensen, C., and Lied, G. A. (2020). Effects of plant-based diets on outcomes related to glucose metabolism: a systematic review. Diabetes Metab. Syndr. Obes. 13, 2811–2822. doi: 10.2147/DMSO.S265982
Khan, S., Dettling, J., Hester, J., and Moses, R. (2019). Comparative Environmental LCA of the Impossible Burger With Conventional Ground Beef Burger. Available online at: https://impossiblefoods.com/sustainable-food/burger-life-cycle-assessment-2019 (accessed September 16, 2021).
Kleinman, P. J. A., Sharpley, A. N., Withers, P. J. A., Bergström, L., Johnson, L. T., and Doody, D. G. (2015). Implementing agricultural phosphorus science and management to combat eutrophication. Ambio 44, 297–310. doi: 10.1007/s13280-015-0631-2
Magkos, F., Tetens, I., Bügel, S. G., Felby, C., Schacht, S. R., Hill, J. O., et al. (2020). A Perspective on the transition to plant-based diets: a diet change may attenuate climate change, but can it also attenuate obesity and chronic disease risk. Adv. Nutr. 11, 1–9. doi: 10.1093/advances/nmz090
Marlow, H. J., Hayes, W. K., Soret, S., Carter, R. L., Schwab, E. R., and Sabaté, J. (2009). Diet and the environment: does what you eat matter? Am. J. Clin. Nutr. 89, 1699S−1703S. doi: 10.3945/ajcn.2009.26736Z
Mejia, A., Harwatt, H., Jaceldo-Siegl, K., Sranacharoenpong, K., Soret, S., and Sabaté, J. (2018). Greenhouse gas emissions generated by tofu production: a case study. J. Hunger Environ. Nutr. 13, 131–142. doi: 10.1080/19320248.2017.1315323
Melina, V., Craig, W., and Levin, S. (2016). Position of the academy of nutrition and dietetics: vegetarian diets. J. Acad. Nutr. Diet. 116, 1970–1980. doi: 10.1016/j.jand.2016.09.025
Merrill, D., and Leatherby, L. (2018). Here's How America Uses Its Land. Available online at: https://www.bloomberg.com/graphics/2018-us-land-use/ (accessed October 1, 2021).
Michel, F., Hartmann, C., and Siegrist, M. (2021). Consumers' associations, perceptions and acceptance of meat and plant-based meat alternatives. Food Qual. Prefer. 87, 104063. doi: 10.1016/j.foodqual.2020.104063
Mikic, A. (2020). Healthy by design: utilizing choice architecture to improve food environments. Curr. Dev. Nutr. 4, 718–718. doi: 10.1093/cdn/nzaa051_015
Miller, D. A. (2017). Seat at the Table: New Voices Urge Farm Bill Reform, 127 YALE L.J. F. 395. Available online at: http://www.yalelawjournal.org/forum/aseat-at-the-table (accessed November 20, 2021).
Montgomery, D. R., and Biklé, A. (2021). Soil health and nutrient density: beyond organic vs. conventional farming. Front. Sustain. Food Syst. 5, 699147. doi: 10.3389/fsufs.2021.699147
Muller, M., Tagtow, A., Roberts, S. L., and MacDougall, E. (2009). Aligning food systems policies to advance public health. J. Hunger Environ. Nutr. 4, 225–240. doi: 10.1080/19320240903321193
Narayanan Nair, M. (2021). Nutritional composition of novel plant-based meat alternatives and traditional animal-based meats. Food Sci. Nutr. 7, 100109. doi: 10.24966/FSN-1076/100109
National Academies of Sciences (2019). Sustainable Diets, Food, and Nutrition: Proceedings of a Workshop. Washington, DC: National Academies Press (US).
Neff, R. A., Edwards, D., Palmer, A., Ramsing, R., Righter, A., and Wolfson, J. (2018). Reducing meat consumption in the USA: a nationally representative survey of attitudes and behaviours. Public Health Nutr. 21, 1835–1844. doi: 10.1017/S1368980017004190
Pimentel, D., and Pimentel, M. (2003). Sustainability of meat-based and plant-based diets and the environment. Am. J. Clin. Nutr. 78, 660S−663S. doi: 10.1093/ajcn/78.3.660S
Rosi, A., Mena, P., Pellegrini, N., Turroni, S., Neviani, E., Ferrocino, I., et al. (2017). Environmental impact of omnivorous, ovo-lacto-vegetarian, and vegan diet. Sci. Rep. 7, 1–9. doi: 10.1038/s41598-017-06466-8
Rubiales, D., and Mikic, A. (2015). Introduction: legumes in sustainable agriculture. CRC. Crit. Rev. Plant Sci. 34, 2–3. doi: 10.1080/07352689.2014.897896
Satija, A., Bhupathiraju, S. N., Rimm, E. B., Spiegelman, D., Chiuve, S. E., Borgi, L., et al. (2016). Plant-based dietary patterns and incidence of type 2 diabetes in US men and women: results from three prospective cohort studies. PLoS Med. 13, 1–18. doi: 10.1371/journal.pmed.1002039
Satija, A., and Hu, F. B. (2018). Plant-based diets and cardiovascular health. Trends Cardiovasc. Med. 28, 437–441. doi: 10.1016/j.tcm.2018.02.004
Saunders, C., and Hayes, P. (2007). Air Freight Transport of Fresh Fruit and Vegetables. Geneva: International Trade Centre (ITC).
Seltenrich, N. (2020). Diet impacts on climate and health: New Zealand's experience. Environ. Health Perspect. 128, 094005. doi: 10.1289/EHP6957
Sewell, C. (2020). Removing the Meat Subsidy: Our Cognitive Dissonance Around Animal Agriculture. Available online at: https://jia.sipa.columbia.edu/removing-meat-subsidy-our-cognitive-dissonance-around-animal-agriculture#6 (Accessed October 22, 2021).
Smith, P., Bustamante, M., Ahammad, H., Clark, H., Dong, H., and Elsiddig, E. (2015). “Agriculture, Forestry and Other Land Use (AFOLU),” in Climate Change 2014 Mitigation of Climate Change, eds O. Edenhofer, R. Pichs-Madruga, Y. Sokona, E. Farahani, S. Kadner, and K. Seyboth (Cambridge: Cambridge University Press), 811–922.
Soret, S., Mejia, A., Batech, M., Jaceldo-Siegl, K., Harwatt, H., and Sabat,é, J. (2014). Climate change mitigation and health effects of varied dietary patterns in real-life settings throughout North America. Am. J. Clin. Nutr. 100, 490S−495S. doi: 10.3945/ajcn.113.071589
Springmann, M., Wiebe, K., Mason-D'Croz, D., Sulser, T. B., Rayner, M., and Scarborough, P. (2018). Health and nutritional aspects of sustainable diet strategies and their association with environmental impacts: a global modelling analysis with country-level detail. Lancet Planet. Health 2, e451–e461. doi: 10.1016/S2542-5196(18)30206-7
Stagnari, F., Maggio, A., Galieni, A., and Pisante, M. (2017). Multiple benefits of legumes for agriculture sustainability: an overview. Chem. Biol. Technol. Agric. 4, 2. doi: 10.1186/s40538-016-0085-1
The White House (2021). Executive Order on Tackling the Climate Crisis at Home and Abroad. Available online at: https://www.whitehouse.gov/briefing-room/presidential-actions/2021/01/27/executive-order-on-tackling-the-climate-crisis-at-home-and-abroad/ (accessed December 5, 2021).
Tilman, D., and Clark, M. (2014). Global diets link environmental sustainability and human health. Nature. 515, 518–522. doi: 10.1038/nature13959
Tran, E., Dale, H. F., Jensen, C., and Lied, G. A. (2020). Effects of plant-based diets on weight status: a systematic review. Diabetes Metab. Syndr. Obes. Targets Ther. 13, 3433–3448. doi: 10.2147/DMSO.S272802
Turnwald, B. P., Boles, D. Z., and Crum, A. J. (2017). Association between indulgent descriptions and vegetable consumption: twisted carrots and dynamite beets. JAMA Intern. Med. 177, 1216. doi: 10.1001/jamainternmed.2017.1637
Tziva, M., Negro, S. O., Kalfagianni, A., and Hekkert, M. P. (2020). Understanding the protein transition: the rise of plant-based meat substitutes. Environ. Innov. Soc. Transitions 35, 217–231. doi: 10.1016/j.eist.2019.09.004
U.S. Department of Agriculture (2017). 2015 Dietary Guidelines: Giving You the Tools You Need to Make Healthy Choices. Available online at: https://www.usda.gov/media/blog/2015/10/06/2015-dietary-guidelines-giving-you-tools-you-need-make-healthy-choices (accessed November 11, 2021).
United Nations Department of Economic Social Affairs (2015). The 17 Goals. Available online at: https://sdgs.un.org/goals (accessed October 14, 2021]).
Vadiveloo, M. K., Malik, V. S., Spiegelman, D., Willett, W. C., and Mattei, J. (2017). Does a grill menu redesign influence sales, nutrients purchased, and consumer acceptance in a worksite cafeteria? Prev. Med. Reports 8, 140–147. doi: 10.1016/j.pmedr.2017.09.001
van Vliet, S., Kronberg, S. L., and Provenza, F. D. (2020). Plant-based meats, human health, and climate change. Front. Sustain. Food Syst. 4, 128. doi: 10.3389/fsufs.2020.00128
Vibart, R., de Klein, C., Jonker, A., van der Weerden, T., Bannink, A., Bayat, A. R., et al. (2021). Challenges and opportunities to capture dietary effects in on-farm greenhouse gas emissions models of ruminant systems. Sci. Total Environ. 769, 144989. doi: 10.1016/j.scitotenv.2021.144989
Wang, Z.-H., Wang, L.-H., Liang, H., Peng, T., Xia, G.-P., Zhang, J., et al. (2021). Methane and nitrous oxide emission characteristics of high-yielding rice field. Environ. Sci. Pollut. Res. 28, 15021–15031. doi: 10.1007/s11356-020-11641-y
Whitmee, S., Haines, A., Beyrer, C., Boltz, F., Capon, A. G., de Souza Dias, B. F., et al. (2015). Safeguarding human health in the Anthropocene epoch: report of The Rockefeller Foundation–Lancet Commission on planetary health. Lancet 386, 1973–2028. doi: 10.1016/S0140-6736(15)60901-1
Willett, W., Rockström, J., Loken, B., Springmann, M., Lang, T., Vermeulen, S., et al. (2019). Food in the Anthropocene: the EAT–Lancet Commission on healthy diets from sustainable food systems. Lancet 393, 447–492. doi: 10.1016/S0140-6736(18)31788-4
Woods, J., Williams, A., Hughes, J. K., Black, M., and Murphy, R. (2010). Energy and the food system. Philos. Trans. R. Soc. B Biol. Sci. 365, 2991–3006. doi: 10.1098/rstb.2010.0172
Wyker, B. A., and Davison, K. K. (2010). Behavioral change theories can inform the prediction of young adults' adoption of a plant-based diet. J. Nutr. Educ. Behav. 42, 168–177. doi: 10.1016/j.jneb.2009.03.124
Keywords: plant-forward diet, plant-based diet, animal-based diet, planetary health, food production, food policy, social-ecological model
Citation: Espinosa-Marrón A, Adams K, Sinno L, Cantu-Aldana A, Tamez M, Marrero A, Bhupathiraju SN and Mattei J (2022) Environmental Impact of Animal-Based Food Production and the Feasibility of a Shift Toward Sustainable Plant-Based Diets in the United States. Front. Sustain. 3:841106. doi: 10.3389/frsus.2022.841106
Received: 21 December 2021; Accepted: 15 March 2022;
Published: 04 May 2022.
Edited by:
Manu V. Mathai, Azim Premji University, IndiaReviewed by:
Michael Martin, Swedish Environmental Research Institute (IVL), SwedenCopyright © 2022 Espinosa-Marrón, Adams, Sinno, Cantu-Aldana, Tamez, Marrero, Bhupathiraju and Mattei. This is an open-access article distributed under the terms of the Creative Commons Attribution License (CC BY). The use, distribution or reproduction in other forums is permitted, provided the original author(s) and the copyright owner(s) are credited and that the original publication in this journal is cited, in accordance with accepted academic practice. No use, distribution or reproduction is permitted which does not comply with these terms.
*Correspondence: Josiemer Mattei, am1hdHRlaUBoc3BoLmhhcnZhcmQuZWR1
Disclaimer: All claims expressed in this article are solely those of the authors and do not necessarily represent those of their affiliated organizations, or those of the publisher, the editors and the reviewers. Any product that may be evaluated in this article or claim that may be made by its manufacturer is not guaranteed or endorsed by the publisher.
Research integrity at Frontiers
Learn more about the work of our research integrity team to safeguard the quality of each article we publish.