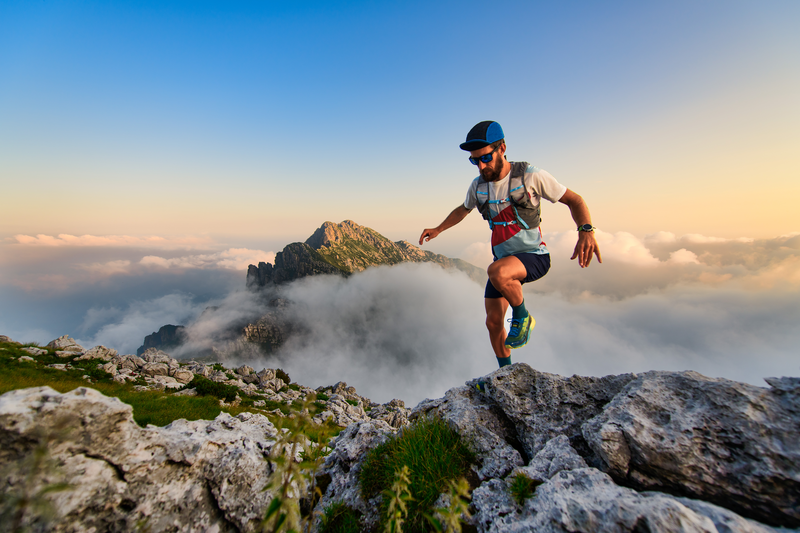
95% of researchers rate our articles as excellent or good
Learn more about the work of our research integrity team to safeguard the quality of each article we publish.
Find out more
PERSPECTIVE article
Front. Sustain. , 15 March 2022
Sec. Circular Economy
Volume 3 - 2022 | https://doi.org/10.3389/frsus.2022.789435
This article is part of the Research Topic Integrated Resource Network Optimization for Sustainable Development View all 7 articles
The biobased business model, which initiated the era of improved use of the biological resources, upgrading resources otherwise wasted or downgraded, started with the “biomass to biofuel” biorefinery: economy by scale, requiring large investments, and stable supply of high volume, low-cost feedstock. A high-profiled business model for pioneering biorefineries was a joint venture, often formed by two already mature businesses, joining two sets of competence, skills, and experience; with opportunity for later merger or acquisition. The biofuel biorefinery was challenged from two sides, its commercial viability (caused by a low-priced end product) and sustainability issues, as it utilizes only the energy content and not the biomass structures. A new era of biobased industries was started, focusing on unlocking the full potential of biomass, by cascading optimized valorization of all (or at least more parts of) the biomass components. This dual objective, opens a spectrum of new types of bioeconomy business models, suitable for more complex biobased industries, including higher-value products in the biobased portfolio, being more complex, handling several more process steps and streams, and producing several types of biobased products. Five examples of such business models are described: (1) Biobased industry, upgrading in-house production sidestreams. (2) Biomass-specialized biorefinery. (3) Cooperatively owned biobased valorization of crop residues and processing sidestreams. (4) Industry clusters as the preferred biobased business model. (5) Local public/private consortium-owned production of biobased products. Furthermore, foresight analysis is presented on possible new business models, suitable for the expected wide variety of new types of biobased industries.
The biobased-business model, which initiated the era of improved use of the biological resources, started with the “biomass to biofuel” biorefinery (Sönnichsen, 2021), which is large in scale, likewise requiring large investments and stable supply of high volume and low-cost feedstock (primarily wheat straw or corn cobs, but also wood chips). The term biorefinery associates 1:1 the biofuel biorefinery to an oil refinery, signaling clearly the objective of substituting fossil-based fuel with biobased fuels. The business model relied on, and was in many instances dependent on: (a) policies and incentive structures, creating and growing the market (e.g., blend-in directives), and (b) public funding supporting development of biomass conversion technologies. The need for biofuel biorefineries to be large in scale and requiring huge investments is caused by fuels being low in price and the profit margin narrow, hereby creating a need for economy-by-scale, in order to ensure commercial viability.
The need for large-scale investments and ditto the size of the production plant and feedstock volume was also a reality for a large part of the biorefineries, established for producing building blocks for biobased chemicals and materials. Notably, the biofuel frontrunner country, the United States, supported, through public funding, the early development of biorefinery technologies for producing biobased fuel, chemicals, and materials, partially due to being seen as a welcomed support for the farmers, producing large volumes of wheat and corn. Public funding for developing biorefinery technologies, incentive structures, and market-creating directives impacted the business and the business model. The number of biofuel biorefineries in the United States was rising in its startup period (50 in 1999, 191 in 2009, and 213 in 2014), but recently, some have gone out of business (in total, 205 in 2019) (Sönnichsen, 2021).
Forming joint ventures for production of biobased substitutes for fossil-based products is another business model used for establishing such sugar platform biorefineries. Case: Production of biobased substitutes for fossil-based polymer or chemistry building blocks can be made by enzyme treatment of the feedstock, creating a sugar platform, suitable for growing bacteria, which have been modified to produce such building blocks. However, making a business in developing the high-quality finished product will also require expertise, competence, and skills, as well as knowledge about product characteristics and customer “care-abouts” within the chemical and polymer business. Leaps forward for the biobased industries have occurred through such strategic joint ventures, often formed by two already mature businesses, joining two sets of competence, skills, and experience, often, later on, leading to a merger or acquisition. Similarly, in the future, it is to be expected that joint ventures, acquisition, and mergers will happen when the biorefinery technologies will expand into making pharmaceutical drugs derived from plant, algae, or fungal biomass components. First-hand knowledge and experience with regard to regulatory approval [e.g., US Food and Drug Administration (FDA)] of the production plant, the process, and the final product, are here crucial to have onboard. Biorefinery technology alone is not sufficient to make such types of specialized products (GreenCarConference, 2008).
The biofuel biorefinery was challenged from two sides, its commercial viability (caused by low-priced end product) and sustainability issues, as it utilizes only the energy content and not exploiting and valorizing the biomass structures. In parallel, a new era of the “Waste2Value” business activities and public research efforts were started. Here, the focus was on unlocking the full potential of the biomass, with a cascading utilization of all (or at least more parts of) the biomass and on striving for producing also higher-value products from the biomass. This dual objective, opens a spectrum of new types of bioeconomy business models, more complex, handling several more process steps and streams, and producing several types of biobased products. This included often products belonging to many types of industrial business segments, for meeting several kinds of customer needs, and regulated by several types of authority approval systems (of products, process, and production plants) (Sierra et al., 2021).
This new type of biobased production, with complexity in process and product, customer needs, and markets open a spectrum of different business models. Notably and importantly, producing several types of products can give robustness to fluctuations in market and price of specific products. Furthermore, getting more value from the biomass conversion, by using several components, not just the energy content, and producing products of significantly higher value than fuels (e.g., new functional materials and chemicals, cosmetics, feed additives, and food ingredients), with potentially larger profit margin, opens improved commercial viability, as well as improved sustainability, documented by strengthened LCA analysis, due to a more efficient and responsible use of the bioresources. This was a game changer for the biobased industry sector at large. The requirement for building biobased business by economy at scale was no longer a general condition (Lange et al., 2021b).
This discontinuity expanded the biobased business vision. Establishing smaller-scale and even local biobased production plants became a realistic opportunity, of relevance for industrial, emerging economy, and developing countries, and using more costly product recovery and product development methods and processes, as well as investing in product approval could be a realistic business strategy, all due to higher profit margin. Interestingly, biobased food and feed ingredients, and even health-improving food supplements and feed additives were leveraged by progress in microbiome sequencing analysis. By improved understanding of microbiomes, it was possible to document changes in gut microbiome organismal composition and function, hereby opening evidence-based demonstration of the effect of new biobased products, not only on gut health but also for skin health and plant health (Berg et al., 2020). Moreover, future opening for those biobased products, made from hitherto wasted or downgraded resources, could be instrumental for improved public health and animal welfare due to improved gut health.
Exploiting the new “biobased” complexity, described above, created a need for development of a range of different business models. In an attempt for a systematic overview of this business diversity, descriptions of six different types of bioeconomy business models are given below.
Classical biological-processing companies, within both food and non-food segments, create several organic sidestreams, which are now downgraded to biogas or soil-improvement products or to bulk, low-priced animal feed. Notably, many of the sidestreams represent a significant (in weight and volume) part of the primary product. This is, for example, the case in the broad spectrum of food processing industries, and in the cotton (textile and fashion) business, as well as in forestry and fishery processing. Inspiring state-of-the-art examples are upgraded use of plant and animal food-processing sidestreams exemplified by a dairy industry case: Pioneering efforts of upgrading in-house industrial sidestreams were made in the dairy industry, upgrading whey to higher-value products (Bolwig et al., 2019). From whey being a very low-priced downgraded product, or even a waste stream you have to pay for having disposed of, the sidestreams can be turned into products with a significantly higher profit margin than the primary product, the milk and the cheese, so much so that upgrading of own sidestreams can contribute significantly to the competitiveness of the (even internationally positioned) business as a whole. The reason for the success of this business model is quite simple. Using the raw materials more efficiently adds disproportionally to both width of the product portfolio and net profit at large. In this way, a food-processing business in reality is turned into being a biorefinery, producing products from all parts of its feedstock, the milk, and all components of the milk being valorized. Notably, beyond successful valorization of whey, the frontrunner industries in the dairy sector have also valorized other sidestreams as well as the organic content of industrial wastewater streams.
Another approach to valorizing own sidestreams, developed over the last decades, is for the food processing company (e.g., slaughterhouse) to get income from upgrading some of the most precious components of their sidestreams and selling business-to-business (B2B) the other sidestreams to specialized waste handlers (Gregg et al., 2020). Notably, calculated according to difference in profit margin, the upgrading-waste handler could acquire slaughterhouses, hereby getting control of the entire value chain, with optimized circularity as a business concept.
Capital expenditures (CAPEX) for upgrading in-house industrial sidestreams is, in general, significantly lower than for a standalone biorefinery. Harvesting synergy as part of an existing production facility can be used for the new biobased value chain processing. Similarly, also the operating expenses (OPEX) are typically lower, due to feedstock logistics already covered by primary production, while marketing and sales can be impacted both positively (more products sold to the same already established customers) or negatively if product diversification involves new types of customers. Based on this overall favorable CAPEX and OPEX scenarios, it is to be expected that the two new trends, resource efficiency via circularity and more plant-based food, will result in widespread practice of upgrading of own sidestreams within the food-processing industries, e.g., brewing, cereal milling, fish processing, and plant-based dairy and meat, as well as starch, juice, and oil extraction. Such improved resource efficiency can strengthen significantly both the competitiveness and the LCA of food processing and biobased industries.
This type of biorefinery characteristically converts a specific type of biomass, aims at unlocking a large part or even the full potential of its feedstock, and strives for strengthening competitiveness by including also higher-value products in the portfolio of biobased products. A series of such biorefineries can be grouped and classified by a color code, reflecting the type of biomass and type of biomass processing (Lange et al., 2021b) (see also Figure 1).
• The green biorefinery, extracting protein from the green parts of the plant, e.g., grass or leaves and stems of sugar beet, and the residual pulp after protein recovery can be sold as bulk cattle field. The driver for the green biorefinery is to develop animal feed from local sources of protein, to substitute for the import of soy from, e.g., South America, produced on deforested land (Gregg et al., 2020). The potential of the business model for this type of biorefinery is not yet fully exploited. Improved commercial viability can be achieved, e.g., upgrading the hemicellulose of the protein extraction pulp can provide basis for producing gut health-promoting food ingredients and feed additives (Fehlenberg et al., 2017).
• The yellow biorefinery, converting and valorizing cereal straw, corn cobs, or wood chips, after pretreatment (including enzyme processing), making biobased products from the cellulose (functional cellulose or a sugar platform for materials, chemicals, or fuels); the hemicellulose (e.g., converted to gut health animal feed (Fehlenberg et al., 2017); and the lignin, by conversion into a range of higher-value products) (Dotsenko et al., 2018).
• The red biorefinery, upgrading, e.g., blood from slaughter houses to medicine for iron deficiency in women and children (Fache et al., 2015), or upgrading chicken feather, converting by microbial/enzymatic conversion, into protein-rich animal feed (Jimenez and Gasche, 2015; Lange et al., 2016).
• The blue biorefinery, upgrading fish cutoffs as well as seaweeds (macroalgae) to a range of higher value, e.g., food and health-promoting products as well as skin treatment and wound healing products; in the future, possibly including also an upgrade of bycatch (Huang et al., 2020; Alfio et al., 2021).
• The brown biorefinery, a new suggested term for valorization of organic materials in sludge and wastewater treatment plants. Of special interest is the production of phosphorous soil improvement products from the microbial biomass of wastewater treatment plants (Lange et al., 2020b) and producing new types of materials (substituting for animal-based products) from the glycoprotein of the bacterial biomass of the wastewater treatment plant.
Figure 1. A color code of the basic six types of biomass conversion is here illustrated. Notably, the color code is not just an easy overview; it groups together different types of biorefinery processing, reflecting the recalcitrance of the biomass, yellow very recalcitrant, plant cell wall rich; green, lignocellulosic but more accessible, needing less pretreatment; blue and red include primarily animal, wall-less biomass (viz. no lignocellulosic recalcitrance). The gray (or white) is often food compatible as it is residue from a food processing, and brown is mixed and wet, with special precautions with regard to contaminants.
Notably, the biorefineries here described (Figure 1) are highly complex, in processing and product development and in marketing and sales, due to the integrated production of several types of end products. Unlocking the full potential of the biomass opens higher profit margins; this more than compensates the add-on expenses expected for such integrated operations. Notably, improved use of the organic resources could be the best approach to prepare for future competition under climate change-derived scenarios, with higher regulatory demands to standards in resource efficiency.
Cooperatively owned food-processing companies represent a highly specialized and successful business model, which at the same time has a lot in common with both in-house upgrading of own sidestreams and sustainable, biomass-specialized biorefineries (see sections Business Model 1: Upgrade of in-house production sidestreams and Business Model 2: Biomass-specialized biorefineries, optimized for sustainability and higher value products above). Cooperatively owned food-processing companies are found in many countries, the owners, typically being the circle of farmers delivering their primary production to the slaughterhouse, the dairy, the olive oil mill, or the potato- or cassava starch-processing plant. The prominent characteristic of such cooperative food-processing plants is that the farmers producing the primary product are getting their share of the revenues, created along the entire value chain. Extending such cooperatively owned food processing to cooperatively owned biorefineries can be a very strong business model. The cooperatively owned biobased production is the only business model that inherently integrates the primary producers, not only being feedstock suppliers but part of the value chain. Interestingly, in, e.g., Denmark, the cooperatively owned food-processing companies are the pioneers and the fastest movers in developing and using new biorefinery technologies for increased resource efficiency and higher income from upgrading sidestreams to higher-value products (Osei-Owusu, 2020). Three business model-relevant features can explain this success: First, the owners are farmers, who know about biology and business, and can see an opportunity for increased income when all parts of their crop are valorized. The second feature is that cooperatively owned food-processing/sidestream biorefineries have control over the entire value chain, from initial harvest to sidestream valorization. The third feature is that this type of ownership provides basis for long-term business planning as no one in the circle of owners is typically advocating for exit strategies or short-term capitalization by selling or merging or being diluted by forming joint ventures.
A biorefinery needs resources in the form of feedstock, enzymes, microorganisms, energy, and water, and a biorefinery produces one or several biobased products, as well as organic sidestreams, processing water, and surplus heat/energy. Thus, value (of mutual benefit) can be harvested through establishing an industrial cluster, among which resources can be exchanged and shared (including also SMEs), together utilizing the resources with optimized efficiency, wasting less, and cutting operational costs (Kalundborg Symbiosis, http://www.symbiosis.dk/en/). In such a cluster, specialized startups and/or divisions of established industries can be integrated: Typically, e.g., an SME (small or medium-sized enterprise) can receive a feedstock component (B2B cluster exchange) from the biorefinery for further specialized product development, e.g., to a specialized chemical or a skin care product. By building a carefully thought thorough clustering of businesses (big and small), the business model for the biorefinery cluster could be established to produce a set of value chains (e.g., biobased food ingredients, feed additives, or soil-improving products) and a profitable B2B company, accomplished by selling interim products to specialized SMEs or industrial divisions in the cluster. In this way, each part of the cluster is both contributing and receiving; together optimizing biobased business of the cluster at large; together having the needed technical expertise, regulatory experience, and knowledge about customer care abouts.
Availability of significant amounts of underutilized local bioresources, combined with, e.g., vacant production facility, entrepreneurial engineers, and bioprocessing specialists, proactive skillful farmers with leftover crop residues, public business development planners, and local industries with knowledge about biobased production, maybe having a sidestream they want to have upgraded—all this can be the starting point for establishing a local versatile biorefinery. In such a local business consortium, the straightforward business model is to establish a new bioprocessing business and sharing both costs and value chain revenues. Different from most biorefineries, here, the feedstock suppliers will typically benefit also from the value generated through the entire value chain. By opening a positive feedback loop, wherein biorefinery business can be improved by optimization of the specialized feedstock, stable in supply and quality (Stegmann et al., 2020), while the cooperatively owned business model, structured by the value chain in focus, from primary production to end product, the consortium-owned business model takes point of departure in a mix of local resources: entrepreneurial skills, vacant buildings, a forward-looking municipality, seeking economic and social development (by job creation), and joining forces in exploiting business opportunities in upgrading of available and accessible underutilized bioresources.
Highly interesting technologies for new types of biobased food production by fermentation are being developed as we speak. Yeast biomass, produced efficiently by fermentation and applied as nutritious and healthy food and feed ingredients (for both large- and small-scale food processing), is already commercialized (Gasser et al., 2008; Sharma et al., 2018). Next in line is to grow yeast on sidestream-derived nutrients, under contained conditions and to grow types of yeast, genetically modified to produce milk and meat proteins and, notably, where the genetically modified yeast organisms themselves are not a part of the final food product. The optimal or preferred choice of business model for this exciting new type of low in emission and land-use food production has not yet been settled. Established biotech companies, with experience in large-scale fermentation and access to huge fermentation tank volume infrastructures, now dominating the global markets in the field of industrial enzymes, could be growing into being food producers as well. For example, the advanced, highly optimized technologies developed for (heterologous) production of enzymes (supplied and sold in bulk) could provide basis for competitively priced food proteins, where milk, meat, and fungal proteins are produced by the fungal yeasts.
Research efforts to convert, e.g., macroalgae, fish innards, crustaceans, or slaughterhouse sidestreams into higher value products have resulted in the discovery of a number of different candidates for new types of pharmaceutical drugs (Lange et al., 2021a). It is to be expected that such drug-lead candidates (e.g., shrimp-based product for control of human blood cholesterol level) will be passing clinical test and regulatory approval and end up as new commercialized biobased drugs. For such high-value, biobased production, joint ventures, mergers, and acquisitions are to be expected as the upscaling, quality testing, and regulatory approval may require double competences, both bioprocessing of biomass (conversion and product recovery) technologies as well as pharma-related upscaled production, formulation, regulatory approval, marketing, and sale of pharmaceuticals. Similarly, investments needed for bringing pharmaceuticals through testing, approval, marketing, and sales are out of the league compared with industrial biobased products.
As a direct result of striving for decreased emission footprint of food production, plant-based food is in focus all over the world [IPCC recommendations (link)], with the purpose of substituting for the import of soy protein for feed and/or to reduce high emissions from animal-based food, meat, milk, and cheese. However, the turnaround, switching toward having plant-based food as the preferred choice, is going only slowly, one reason being that much of the plant-based food does not appeal to people who are used to having meat as a major part of their daily diet. An obvious and very promising trend to make plant-based food the preferred choice is to make plant-based food more attractive, by deliciousness, achieved by adding fungal-based ingredients. Fungi are closely related to animals than to plants, and fungal proteins have the inherent potential (as also glutamate-rich meat protein has), to give the “Umami” flavor, taste, and mouthfeel. Industrial production of sustainable “Umami” -flavored plant-based food can be achieved by making fungal-derived “Umami” flavoring food ingredients, sold business-to-business to plant-based food industries (or sold directly to end users as “Umami” sauce, fond/buillion, or spreading). Such sustainable “Umami” flavoring food ingredients can be made either by growing fungi on sidestreams or by enzyme treatment of glutamate-rich plant-derived sidestreams.
Some of the biorefinery concepts, already developed and established in commercial scale, have an inherent potential of being implemented as a mobile unit. By being mobile, feedstock also from larger areas can be served by low-investment production units. This opens the opportunity of delivering freshly made biobased products (such as food and feed ingredients and soil-improving products) to the neighborhood. Thus, mobile bioprocessing production units can enable a new type of business model.
In a society undergoing a transmission into higher degrees of circularity and resource efficiency, the wastewater treatment plants should be recognized as an asset, representing a potentially valuable source of organic feedstock, available and accessible for biorefinery upgrading. New business models can be developed for valorizing of bioresources previously going wasted or used only for downgrading (e.g., biogas production). Right now, wastewater treatment plants have, in many countries, quite strict regulations for which type of commercial business (if any) they can be part of. This kind of restrictions (found also for handling of municipality waste) will have to be revisited in order to optimize for a growing need for increased resource circularity.
Some biorefinery technologies for advanced and complex processing, optimized for producing specialized (IP-protected) high-value products from specialized feedstocks found in high volume in several parts of the world could call for a globally distributed business model. Daughter companies established on several continents, in close proximity to where the feedstock is produced, benefitted by a globally protected technology, with the opportunity to sell the products without being burdened by the CO2 from transcontinental transport.
Carbon capture technologies, combined with access to low-cost surplus of renewable electricity and pyrolysis are within reach, developing as we speak, using, e.g., atmospheric CO2 as feedstock and Power2X as the enabling technology producing, e.g., renewable jet fuel and naphta (for alternative, non-fossil-based textile fibers). However, another and even more readily at hand opportunity is to use biogenic CO2 and/or biogenic methane, CH4, derived from biogas, as basis for Power2X technologies, or similarly using manure from pig production as feedstock for pyrolysis, making new types of low-emission fertilizers. For such highly promising negative emission technologies, the business models of choice have not yet been identified; however, it seems likely that most of such initiatives will be developed, aiming at large-scale production plants, requiring huge upfront investments, exactly as the initial biomass to biofuel biorefineries. Notably, with a high probability that after some years, also smaller-scale applicable carbon capture units (“end of chimney,” producing also higher-value products) will be established using negative emission technologies, possibly even accommodating use of gentle and environmentally friendly bioprocessing instead of only focusing on large-scale pyrolysis for negative emission processes. An interesting example is the production of bacterial single-cell protein for animal feed, based on growing methane-digestive bacteria on methane, derived from biogas (Ritala et al., 2017).
The biobased era has been developing, continent by continent more than in a globalized manner. For the United States, Canada, Russia, and to some extent Australia, biobased focus has been on domestic markets for biofuel and biobased chemicals and materials. South America was pioneering the biofuel era by the early development of sugarcane–ethanol with extended local use. China focused, in the last 5-year plan, primarily on producing biofuel, e.g., from corn stover. Developing the European Union biobased economy focused on converting European feedstocks into a broader variety of biobased products, to be sold primarily in the European Union, starting with the sugar platform-based fuels, chemicals, and materials. However, in the last 5–7 years, the European Union has, in their common Horizon2020 R&D BioBased Industries program (BBI-JU), developed bioprocessing value chains, creating higher value by recovering also more complex plant components (proteins, oligomers, and metabolites) turned into higher-value products (Lange et al., 2021b). European biotech companies produce enzymes, food ingredients, alternative food proteins, and starter cultures, by daughter companies, operating and commercializing in many continents. It remains to be seen if the most successful biobased products will, in turn, also be produced by globalized companies, being present in many continents or the trend will go toward establishing (also) many local industries.
A different biobased business model could be developed for valorizing underexploited biomass, present in high amounts in tropical and subtropical countries, in developing countries, as well as in emerging market countries. The need for improved use of the African biological resources is a burning platform. Climate change-challenged agriculture in Africa is posing a threat to food security, locally as well as regionally. This happens at the same time as ~33% is lost as food waste (Verma et al., 2020). Another 10–15% of available crop residues and sidestreams are downgraded or going to waste; notably, these huge amounts of wasted biomass hold potential to be developed into nutritious food as well as feed or phosphorous and nitrogen soil-improving products (Yogev et al., 2020). This crucial fact is continuing even though biomass conversion technologies exist, by which microbes and microbial enzymes can be used to open the biomass structures and to enrich its protein content, e.g., for nutritious and healthy food for people or feed for, e.g., chicken, pigs, and fish.
A clever and timely move for a more sustainable world (contributing to meeting the UN SDGs, mitigating climate change, adaptation to climate change-derived lower yields, and stopping biodiversity loss) by using the harvest efficiently instead of wasting almost half, can be waiting just around the corner. Europe could collaborate more with African scientists, engineers, young entrepreneurs, and startups for developing, using, and optimizing open-access bioprocessing technologies to upgrade local resources, crop residues, sidestreams, sludge, and household waste to healthy nutritious food, animal feed, locally produced fertilizer, biobased materials, chemicals, or fuels, hereby, building improved societal resilience by gaining stable food security (substituting for food-aid programs, which risks to harm local food-processing companies and start-ups), creating jobs, stimulating rural livelihood, and diminishing the urge to emigrates because life is good where home is. This cross-continent collaboration and knowledge sharing can be turned into one of the most important business models for the biobased era.
For this to happen, business models must include more than what is described above plus market forces: Corporate social responsibility efforts, CSR, international green funds, and philanthropy should work hand in hand with local (also many young) entrepreneurs (see the successful BioInnovate Africa, https://bioinnovate-africa.org/), and skilled work force, based on local biomass resources producing much needed biobased products, marketed in a growing market, including for export, hereby providing for improved food security for a growing population (Lange et al., 2012, 2020a).
The original contributions presented in the study are included in the article/supplementary material, further inquiries can be directed to the corresponding author/s.
The author confirms being the sole contributor of this work and has approved it for publication.
The author declares that the research was conducted in the absence of any commercial or financial relationships that could be construed as a potential conflict of interest.
All claims expressed in this article are solely those of the authors and do not necessarily represent those of their affiliated organizations, or those of the publisher, the editors and the reviewers. Any product that may be evaluated in this article, or claim that may be made by its manufacturer, is not guaranteed or endorsed by the publisher.
The author hereby appreciates the inspiring discussions/shared experience with colleagues in both public and private R&D/R&D-based businesses in the industry and SMEs, and colleagues among committee members, being bioeconomy advisory committees, in the EU, Nordic Countries, and Denmark, or members of advisory boards or boards of directors for small and large industries, academia, NGOs, or public research council committees.
Alfio, V. G., Manzo, C., and Micillo, R. (2021). From fish waste to value -an overview of the sustainable recovery of omega-3 for food supplements. Molecules 26, 1002. doi: 10.3390/molecules26041002
Berg, G., Rybakova, D., Fischer, D., Cernava, T., Vergès, M. C., Charles, T., et al. (2020). Microbiome definition re-visited: old concepts and new challenges. Microbiome 8, 103. doi: 10.1186/s40168-020-00875-0
Bolwig, S., Brekke, A., Strange, L., and Strøm-Andersen, N. (2019). Valorisation of Whey: A Tale of Two Nordic dairies. From Waste to Value: Valorisation Pathways for Organic Waste Streams in Circular Bioeconomies. London: Taylor & Francis. Available online at: https://www.taylorfrancis.com/books/e/9780429460289
Dotsenko, G., Meyer, A. S., Canibe, N., Thygesen, A., Nielsen, M. K., and Lange, L. (2018). Enzymatic production of wheat and ryegrass derived Xylo-oligosaccharides and evaluation of their in vitro effect on pig gut microbiota. Biomass Convers. Biorefinery 8, 497–507. doi: 10.1007/s13399-017-0298-y
Fache, M., Boutevin, B., and Caillol, S. (2015). Vanillin, a key-intermediate of biobased polymers. Eur. Polym. J. 68, 488–502 doi: 10.1016/j.eurpolymj.2015.03.050
Fehlenberg, V., Baumanna, M., Gasparrib, N. I., Piquer-Rodrigueza, M., Gavier-Pizarro, G., and Kuemmerlea, T. (2017). The role of soybean production as an underlying driver of deforestation in the South American Chaco. Global Environ. Change 45, 24–34. doi: 10.1016/j.gloenvcha.2017.05.001
Gasser, B., Saloheimo, M., Rinas, U., Dragosits, M., Rodríguez-Carmona, E., Baumann, K., et al. (2008). Protein folding and conformational stress in microbial cells producing recombinant proteins: a host comparative overview. Microb. Cell Fact 7, 11. doi: 10.1186/1475-2859-7-11
GreenCarConference (2008). DuPont and Genencor, a Division of Danisco A/S, Forming a 50-50 Global Joint Venture. Itasca, IL: DuPont Danisco Cellulosic Ethanol LLC. Available online at: https://www.greencarcongress.com/2008/05/dupont-and-gene.html
Gregg, J. S., Jürgens, J., Happel, M. K., Strøm-Andersen Tanner, A. N., Bolwig, S., and Klitkou, A. (2020). Valorization of bio-residuals in the food and forestry sectors in support of a circular bioeconomy: a review. J. Cleaner Prod. 267, 122093. doi: 10.1016/j.jclepro.2020.122093
Huang, Y., Łezyk, M., Herbst, F.-A., Busk, P. K., and Lange, L. (2020). Novel keratinolytic enzymes, discovered from a talented and efficient bacterial keratin degrader. Sci. Rep. 10, 10033. doi: 10.1038/s41598-020-66792-2
Jimenez, K, Kulnigg-Dabsch, S., and Gasche, C. (2015). Management of iron deficiency anemia. Gastroenterol. Hepatol. (N Y) 11, 241–250.
Lange, L., Agger, J. W., and Meyer, A. S. (2020a). “fungal biotechnology, unlocking the full potential of fungi for a more sustainable world,” in Grand Challenges in Biology and Biotechnology, ed H. Nevalainen (Cham: Springer), 3–32. doi: 10.1007/978-3-030-29541-7_1
Lange, L., Bak, U. G., Mikkelsen, M. D., Meyer, A. S., Karlsson, E. N., Polymenakou, P., et al. (2021a). The Blue Bioeconomy - A Significant Contribution to Sustainable Development, Locally as Well as Globally. Copenhagen.
Lange, L., Bech, L., Busk, P. K., Grell, M. K., Huang, Y., Lange, M., et al. (2012). The importance of fungi and of mycology for a global development of the bioeconomy. IMA Fungus 3, 87–92. doi: 10.5598/imafungus.2012.03.01.09
Lange, L., Grandorf-Bak, U., Hansen, S. C. B., Gregersen, O., Harmsen, P., Nordberg-Karlsson, E., et al. (2020b). “Sustainable seaweed technologies,” in Cultivation, Biorefinery, and Applications, Advances in Green Chemistry, Chapter 1 - Opportunities for Seaweed Biorefinery, eds Torres MD, Kraan S, and Dominguez H (Amsterdam: Elseiver), 3-31. doi: 10.1016/B978-0-12-817943-7.00001-9
Lange, L., Huang, Y., and Busk, P. K. (2016). Mirobial decomposition of keratin in nature—a new hypothesis of industrial relevance. Appl. Microbiol. Biotechnol. 100, 2083–2096. doi: 10.1007/s00253-015-7262-1
Lange, L., O‘Connor, K., Arason, S., Bundgård-Jørgensen, U., Canalis, A., Carrez, D., et al. (2021b). Developing a sustainable and circular bio-based economy in EU: by partnering across sectors, upscaling and using new knowledge faster, and for the benefit of climate, environment & biodiversity, and people & business. Front. Bioeng. Biotechnol. 8, 619066. doi: 10.3389/fbioe.2020.619066
Osei-Owusu, A. K. (2020). Assessing Denmark's global food traderelated environmental impacts (PhD dissertation). Department of Environmental Science, Faculty of Technical Sciences, Aarhus University, Aarhus, Denmark.
Ritala, A., Häkkinen, S. T., Toivari, M., and Wiebe, M. G. (2017). Single cell protein–state-of-the-art, industrial landscape and patents. Front. Microbiol. 13, 2009. doi: 10.3389/fmicb.2017.02009
Sharma, S., Hansen, L. D., Hansen, J. Ø., Mydland, L. T., Horn, S. J., Øverland, M., et al. (2018). Microbial protein produced from brown seaweed and spruce wood as a feed ingredient. J. Agric. Food Chem. 66, 8328–8335. doi: 10.1021/acs.jafc.8b01835
Sierra, A., Zika, E., Lange, L., Llorente, P., Canalis, A., Esteban, P. M., et al. (2021). The bio-based industries joint undertaking: a high impact initiative that is transforming the bio-based industries in Europe. New Biotechnol. 60, 105–112. doi: 10.1016/j.nbt.2020.09.003
Sönnichsen, N. (2021). U.S. Number of Installed Ethanol Biorefineries 1999-2019. Available online at: https://www.statista.com/statistics/1106352/number-installed-biorefineries-us/
Stegmann, P., Londob, M., and Junginger, M. (2020). The circular bioeconomy: its elements and role in European bioeconomy clusters. Resour. Conserv. Recycl. 6, 100029. doi: 10.1016/j.rcrx.2019.100029
Verma, M. v. d. B., de Vreede, L., Achterbosch, T., and Rutten, M. M. (2020). Consumers discard a lot more food than widely believed: estimates of global food waste using an energy gap approach and affluence elasticity of food waste. PLoS ONE 15, e0228369. doi: 10.1371/journal.pone.0228369
Keywords: stand-alone biorefineries, cascading biorefineries, biorefinery business clusters, cooperative biorefineries, joint Ventures
Citation: Lange L (2022) Business Models, Including Higher Value Products for the New Circular, Resource-Efficient Biobased Industry. Front. Sustain. 3:789435. doi: 10.3389/frsus.2022.789435
Received: 04 October 2021; Accepted: 21 January 2022;
Published: 15 March 2022.
Edited by:
Rodrigo Salvador, Universidade Tecnológica Federal do Paraná, BrazilReviewed by:
S. Venkata Mohan, Indian Institute of Chemical Technology (CSIR), IndiaCopyright © 2022 Lange. This is an open-access article distributed under the terms of the Creative Commons Attribution License (CC BY). The use, distribution or reproduction in other forums is permitted, provided the original author(s) and the copyright owner(s) are credited and that the original publication in this journal is cited, in accordance with accepted academic practice. No use, distribution or reproduction is permitted which does not comply with these terms.
*Correspondence: Lene Lange, bGVuZS5sYW5nZTJAZ21haWwuY29t
Disclaimer: All claims expressed in this article are solely those of the authors and do not necessarily represent those of their affiliated organizations, or those of the publisher, the editors and the reviewers. Any product that may be evaluated in this article or claim that may be made by its manufacturer is not guaranteed or endorsed by the publisher.
Research integrity at Frontiers
Learn more about the work of our research integrity team to safeguard the quality of each article we publish.