- School of Computing and Communications, Lancaster University, Lancaster, United Kingdom
Today's data centers use substantial amounts of the world's electrical supply. However, in line with circular economy concepts, much of this energy can be reused. Such reuse includes the heating of buildings, but also commodity dehydration, electricity production and energy storage. This multi-disciplinary paper presents several novel applications for data center waste heat. Next, the paper accounts for three case studies, taken from three different societal contexts: urban Malaysia, rural Costa Rica and semi-urban Sweden. A discussion on data center energy metrics leads to the development of a new metric, Datacenter Energy Sustainability Score (DESS), which is evaluated within the three use cases. Last, it is shown how a material social view on metrics provides a way past a problem that has haunted the data center industry for the last 15 years, whilst benefitting both data center owners who want to compete through sustainability as well as stakeholders from governments on local, regional and national levels. The paper makes clear that a sustainability strategy should be based on a material social view and stretch beyond the building itself. In fact, and as demonstrated by the relevance of DESS, modern data centers are so energy-efficient that data center sustainability is no longer mainly an engineering issue, but a matter requiring multi-disciplinary insights, approaches and collaboration.
1. Introduction
In these environmentally uncertain times, many activities move online, often making everyday life more efficient and offsetting parts of the local and global travel carbon footprint. However, few activities—be they online or in-person—come without an environmental cost. Consequently, the data centers that enable services such as Google Maps, Spotify or health care systems consume about 1% of the world's available electricity (Masanet et al., 2020), and it is likely this figure will increase (Freitag et al., 2021). The purpose of the project described in the rest of this paper has been to alleviate this consumption. Contrary to the majority of research on data center sustainability, this has been done using a holistic, material social point of view, in which engineering queries are equally integrated with societal (and environmental) concerns.
Data centers' high energy needs mainly originate from processor-intensive computations on the computers (called “servers”). Server cooling typically results in 15–100% overhead, where the very large (“hyperscale”) data centers achieve the lowest figures (Ascierto and Lawrence, 2020). Supplied electricity ultimately transforms into waste heat, which is usually vented out (Barroso et al., 2019). Before reaching the servers, the airflow is filtered and often dehumidified, to protect the electrical components. At only 30–40°C, a data center's airflow is much cooler than other industrial waste heat. However, it should be noted that high-performance data centers may approach 45°C (Oltmanns et al., 2020) and that servers submerged in non-conductive liquids—“liquid immersion cooling” systems—may run at higher temperatures since liquids transport heat much better than air does (Bansode et al., 2018).
Any data center has three points of concern for energy sustainability in daily activities: (a) source of and stable access to ingoing (and backup) power, (b) energy use for computation and cooling, and (c) potential waste heat use (Figure 1). Much has been written about renewable energy and, as seen in Section 4.4, about energy saving procedures within the data center. Fewer works address data center waste heat, which is why this has been the project's focus.
Waste heat use is linked to the concept of a circular economy, founded on the three Rs: reduce, reuse and recycle (Murray et al., 2017). Industrial collaboration is at the heart of this concept (Gregson et al., 2015). Of special interest here is the sub-concept industrial symbiosis, where undesired output from one industry serves as input to another (Murray et al., 2017). To enable industrial symbiosis, and so, the circular economy, a holistic and high-level view is required, of industry processes, of societal governance, legislation, needs and visions, of environmental concerns, and of how industry, society and the environment connect. In other words, the project calls for a multi-disciplinary systems engineering view of the entities and their relationships (Figure 2).
Sovacool et al. (2022), who have studied data center waste heat use in the Nordics, note that a holistic strategy is necessary to successfully address data center energetics. More than that, they argue it is an opportunity for society and environment:
Datacenter operators, and policymakers and planners, need to promote a broader, more holistic notion of sustainability that extends beyond servers and computers to encompass the whole system. Although this broadens the challenge of datacenter sustainability, it also enables the identification of a multitude of options to ensure future digital services are more affordable and resilient but also more energy-efficient, more climate friendly, less wasteful, and more optimized. (Sovacool et al., 2022)
Today, data centers placed in the Swedish capitol heat tens of thousands of homes by directing the waste heat airflow to the district heating network (Wahlroos et al., 2018; Koronen et al., 2020; Oltmanns et al., 2020). The financial and environmental gains from such a strategy can be substantial (Davies et al., 2016; Ruch et al., 2017; Wahlroos et al., 2017, 2018; Huang et al., 2020; Oltmanns et al., 2020). Reversing the district heating process, district cooling is potentially obtainable. However, since cooling and heating techniques feed on temperature differentials, and data center waste heat is often not radically different from ambient temperatures, heat pumps or absorption refrigerators are needed to reach adequate temperatures.
In other words, data center waste heat is not entirely reclaimable for a district heating/cooling network, and when other industrial waste heat is available, the comparable value goes down further (Widman, Falu Energi & Vatten AB, meeting August 17, 2022). The low temperature further makes converting heat into electricity a non-viable option. Hence, to avoid conversion losses and investments in added infrastructure, this paper focuses on secondary uses for this dry, clean, lukewarm air.
The paper first discusses existing and proposed applications for this heat energy (Section 3.1). Section 3.2 provides an overview of three case studies, from contexts with widely differing preconditions for waste heat reclamation. A text on data center energy efficiency metrics follows in Section 3.3, and a new metric is proposed, so that the applications and case studies can be evaluated. The evaluation is carried out in Section 3.4. A discussion closes the paper.
2. Materials and methods
There is a constant interplay between the material and the social. As noted by Huber (2015), environmental and economic historians now recognize that in addition to technical systems, fossil fuels have reshaped our political, cultural, and environmental relations for the last two centuries. Evans et al. (2020) see similar patterns for plastics. In the material social perspective proposed in this paper, data center energy is subject to the same concerns. Consequently, this multi-disciplinary project follows the tradition (e.g., Swyngedouw, 2009; Blackwell, 2021) in how to approach societal perspectives on ICT and other infrastructure. In other words, the paper has required a plurality of methods and a multifaceted view of data center energetics. Fortuitously, increasing the complexity beyond engineering queries helps forming new ideas. Indeed, “the goal of epistemological pluralism is … to think more creatively and widely about how to imagine … new development pathways” (Nightingale, 2016).
Finding relevant, up-to-date and accurate information about the data center industry characteristics is challenging. It is a big and much exposed field that changes rapidly, and academia has trouble keeping up with marketing departments of Big Tech (Google, Facebook, Microsoft, Amazon and Apple or the Chinese equivalent ecosystem). For example, large-scale data center developments in the USA and Europe have been followed by issues relating to electricity and water issues (McCammon, 2017; Judge, 2022). Also, there are questions pertaining to metrology—the scientific field of metrics—as the most used energy efficiency metric has come to represent all sustainability-related matters (see Section 3.3.1).
The social sciences, in particular, have been absent from data center research (Hu, 2015), meaning engineering inquiries have dominated the topic. This lack of interest is notable, as inside an often unspectacular and understated physical appearance, a large-scale data center is an entity with a great deal of societal connotations. Ipsen concludes that the
…academic data center literature is not nearly fully developed, and forward progress is slow. The very nature of data centers as both real and virtual spaces requires that research crosses disciplinary boundaries. Their impacts are neither just physical or social, nor spatial or temporal, but a mix of everything at once. Much of the existing literature is technical, where scholars note the intensive energy use of data centers, but fail to engage with the associated social or political dimensions this infrastructure can bring about… (Ipsen, 2018)
Investigating the problematics of the data center industry through a material social lens has required a multi-disciplinary investigation. Systems engineering's strong focus on problem-solving and of making connections between entities (Watson, 2019) has facilitated the investigation. To embrace cross-disciplinarity and give equal weights to engineering and societal concerns, the mixed methods paradigm has been helpful. Stressing the use of both qualitative and quantitative approaches, mixed methods is suiting when one needs a “large toolkit of methods and designs to address complex, interdisciplinary research problems” (Creswell and Garrett, 2008).
Uses for the waste heat are dependent on a multitude of factors. Some of those are globally relevant, such as the willingness or incentives for the adoption of sustainable business strategies, and the expertise needed to challenge the status-quo in a way that would not jeopardize uptime or security of the data center. Others are specific to location, such as temperature, humidity, presence of commodities suitable for drying (and if so, the length of their drying season) political stability, infrastructure, ICT (information and communications technology) needs—for low-income nations, a strong tourism sector may facilitate—and not least the financial strength of the region. Many more factors are charted in the analytical framework (Figure 3), itself an outcome from research visits and literature studies, and a potential checklist for future data center establishments worldwide.
For validation purposes, the project uses triangulation, providing a multi-faceted perspective on one specific issue (Yin, 2012). In this case, the research problem has been approached through an extensive and cross-disciplinary literature review, research visits, interviews, participation in data center industry hearings, and the development of a new metric for data center energetics measurement.
The literature review comprises papers, books, and gray literature such as industry reports. These relate to data centers, energy, commodity production, drivers for commercialization, societal progression, country statistics and environmental sustainability. In addition, newsletters from industry associations, media and data center infrastructure specialists have been monitored throughout the project. This reading deepened the understanding for the computer science, engineering, societal, environmental and managerial dimensions of the project, and provided insights in possibilities and barriers for successful data center energy improvements in specific societal and environmental contexts.
The paper includes three case studies, to obtain an understanding of the value of proposed applications and new metrics in various contexts. Malaysia was chosen because of its strategic location (see Section 3.2.1) and the challenges of reclaiming waste heat in an equatorial nation. Sweden is a leader in data center waste heat recovery (see Section 3.2.3), and features a relatively cold climate. Consequently, Swedish and Malaysian preconditions would drastically differ. The paper identifies coffee production as a beneficiary of data center waste heat. Among the 15 largest coffee producers, Costa Rica has the lowest ICRI (Infrastructure/Consumer Readiness Index; see Terenius et al., 2021a). This indicates Costa Rica has an attractive market space for added ICT infrastructure, and so, it is well positioned for implementing the ideas outlined in Section 3.2.2.
Ethnographic research has been needed for this project, since waste heat reclamation has both material and social connotations and “research that seeks solutions to environmental problems often lacks a deeper understanding of social relations and power structures” (Kaijser and Kronsell, 2014). Research visits have involved travel to Luleå and Boden in northern Sweden, October 26–29, 2020, Falun in mid Sweden August 23, 2021 and August 17, 2022, and Kuala Lumpur, Johor Bahru, Port Dickson and Cameron Highlands in Malaysia, April 30–June 2, 2022. Visiting Luleå and Falun highlighted the importance of cultural factors for the establishment of the sustainable data center industry in the Nordics. Exploring Kuala Lumpur and the more rural areas of Peninsular Malaysia's west coast mainly by foot and public transport provided many insights in everyday life, legislation and cultural factors.
A formal interview specific to this project was held on May 7, 2022 with the former vice-president of the Malaysian Academy of Science, who has served as an advisor to the president of Malaysia and as the Director General of the Malaysian Institute of Microelectronic Systems. In addition to a number of visits to Malaysian academic researchers, a meeting was held with WWF Malaysia on May 30, 2022, to discuss data centers' role for social and environmental sustainability during the coming three decades.
The project has meant actively partaking in many industry hearings and academic conferences on data center sustainability, data center future design and marine energy prospects.
3. Results
This section begins by presenting results relating to applications for data center waste heat. Next follow three studied societal contexts, where these ideas could potentially be implemented: urban Malaysia, rural Costa Rica and semi-urban Sweden. Section 3.3 relates to metrics used and misused in the data center industry, and introduces a new, holistic metric. Finally, Section 3.4 discusses the earlier use cases in light of improved data center sustainability metrics.
3.1. Applications
Industrial waste heat has many uses, and is often used to supply district heating networks with heat. Heat reuse is attractive, as it may offset an equal portion of energy gathered from other sources. The major obstacle in reclaiming data center waste heat is its low temperature, which is not much hotter than outside temperatures in the tropics. As a consequence, within the data center industry, waste heat use is quite undeveloped. Still, the high amount of heat energy—today mostly wasted—makes it worth trying to reclaim it.
There are some more known uses for data center waste heat besides district heating. Site-specific uses include heating water for swimming pools or fish and lobster farming,1 and heating greenhouses to prolong the growing season in cold regions (for data centers located within city limits, urban farming is an option). For city-based small-scale data centers, heating a nearby facility directly is often feasible. A merit of these uses is that heat pumps can be avoided; to avoid conversion losses, need for supplementary power and expensive investments, it is always beneficial to use energy as-is.
But how can the heat be utilized where these applications are not appropriate, such as in hot climates? This section presents some suggested applications for data centers' outgoing heat.
3.1.1. Commodity dehydration
First presented to the research community in 2020 (Terenius, 2020), commodity dehydration using data center waste heat would not decrease energy use, but give an ability to easily repurpose heat to the benefit of high-, mid-, and low-income countries around the Globe. Furthermore, as mentioned above, using the heat as-is would avoid the need for heat exchangers and their associated energy conversion losses.
One suitable commodity is wooden pellets from forestry industry leftovers. Once the powdered wood has been dehydrated by the waste heat, the powder is pressed into pellets (Figure 4). The pellets are then used for heating during winter. Heat for wood powder dehydration is currently supplied to the Falun municipality by EcoDataCenter in Sweden to become “the world's first climate-positive data center”.2 Many other commodities can potentially be dehydrated using data center waste heat, such as fruit, fish or tea leaves for human consumption, fodder for cattle, and seaweed for biofuel production. In so doing, data centers can substitute massive amounts of electricity and fossil fuel used for dehydration today. It is true that in the tropics, many of these commodities are sun-dried. However, industrial dehydration in warehouses increases food security since rodents, insects and birds cannot access the commodity and since the air is filtered. Moreover, the produce can be dehydrated in a controllable fashion and more evenly than through sun-drying, and also at night, when sun is set and ambient temperature decreases. The relatively cool waste heat maps particularly well to commodities risking scorching (fruit, coffee beans) as well as to those where hotter air causes fuel volatilization (seaweed is one example thereof, see Skoglund et al., 2017).
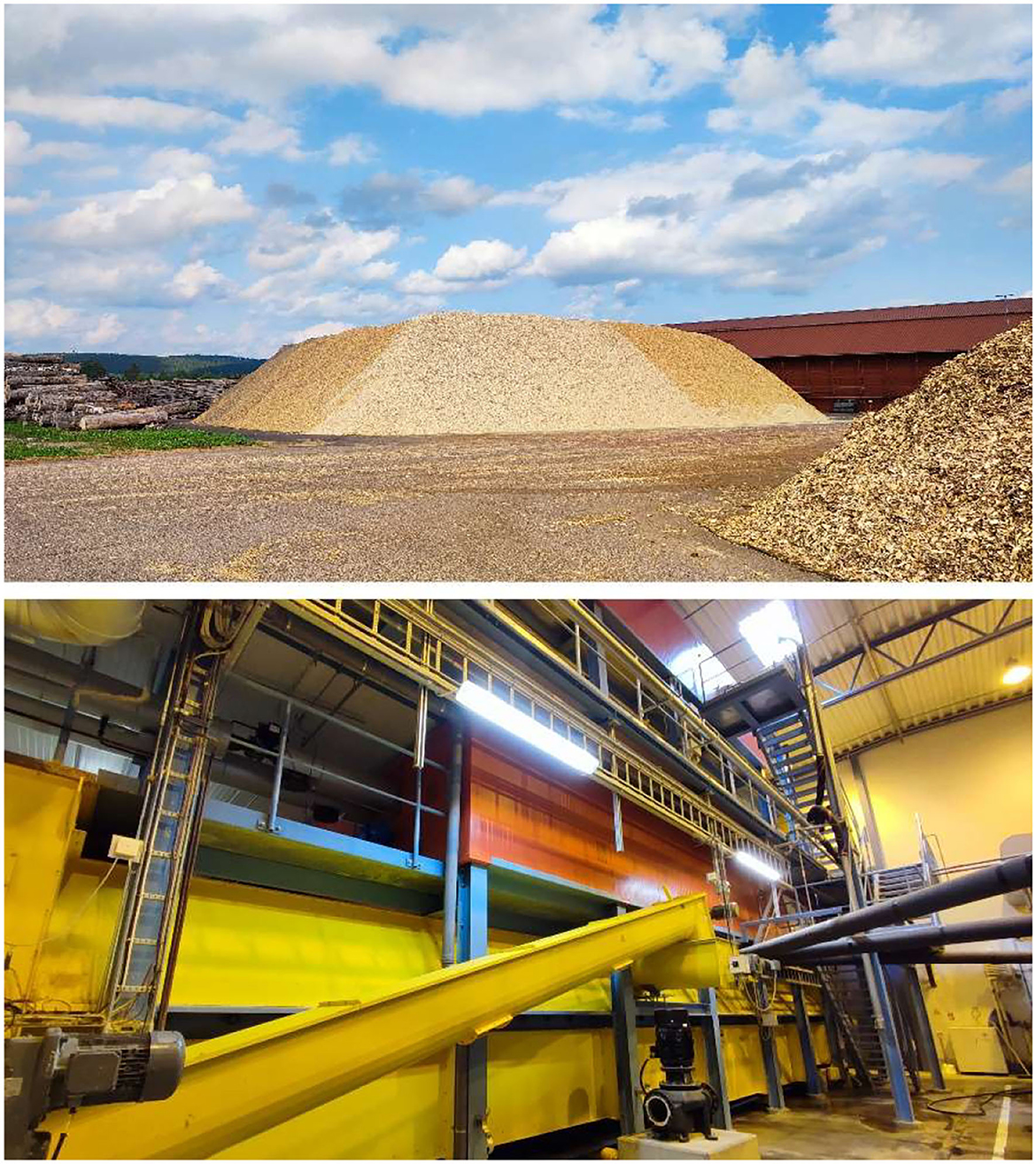
Figure 4. Large-scale wooden pellet dehydration, partially carried out with data center waste heat from EcoDataCenter, at Falu Energi and Vatten AB. The upper photo shows timber deemed unusable by the forestry industry (left) and therefore destined to become pellets (the two gigantic piles). Photo: P. Terenius.
In addition to addressing the world's electricity use, data access and commodity dehydration are enablers for building local sustainable communities. One particularly suitable commodity for dehydration is coffee, one of the world's most traded agricultural commodities. Coffee beans need drying to achieve moisture levels low enough for extended storage, and to avoid taste deficiencies, the airflow should preferably not exceed 45°C (Phillips, 1963). Moreover, coffee is often produced in humid areas, where sun-drying for partial dehydration is not possible. Therefore, drying is commonly (partly or wholly) carried out using diesel-, wood- or electricity-powered machinery.
For an agricultural community away from major cities, the prospects of using data center waste heat to dry coffee beans or other commodities are appealing. Conversely, suppose an existing drying facility in a community may be powered by data center waste heat. That may attract international data center builders for both financial and CSR (corporate social responsibility) reasons, in turn increasing ICT availability locally or regionally.
This vision—illustrated in Terenius et al. (2021a)—empowers local coffee producers: drying coffee beans close to source and then playing a more active role in supply chains increases profits for local farmers or collective efforts. Bridging political ecology and engineering, this strategy can be used in many countries, with many commodities, on different scales and in various societal contexts.
3.1.2. Energy storage solutions
In an energy supply chain, heat is usually considered the least desirable form of energy, as it is challenging to distribute and use. In addition, heat is regarded difficult to store. However, there are ways to store waste heat energy economically, safely and reliably. Dehydration of wooden pellets serves as one example, biofuel production from seaweed as another.
Another option worth further investigation is dehydration of salt hydrates. This technique—suggested for data center waste heat purposes in 2008 (Harman, 2008) but not further explored—involves running a warm airflow over the salt hydrate, to remove the water and leave the salt behind. The salt is then packed and transported to wherever there is use for heat. Here, humid air is introduced to the salt and rehydrates it. Doing so releases energy, and a building can be heated. “Charging” thermo-chemical materials such as salt hydrates has several benefits: salt hydrates are inexpensive, easily stored for prolonged times, and non-toxic. Importantly, some of them work at the low-temperate heat provided by data centers (Onder and Sarier, 2015; Noël et al., 2022), avoiding the need for heat pumps.
3.1.3. Marine energy
Both large (Microsoft, Google) and small actors are currently expressing interest in placing data centers near the coastline, or even as floating (Clidaras et al., 2009) or submerged3 facilities, to take advantage of seawater for free server cooling. However, the waste heat—in this case, the heated seawater—goes wasted. One option is connecting a data center and an ocean thermal energy conversion (OTEC) plant via a working fluid such as ammonia or seawater and a dedicated heat exchanger (Terenius et al., 2021b). In OTEC, tropical surface water and cold deep ocean water (DOW) interplay to evaporate a working fluid which in turn drives a turbine to generate electricity. Optionally, the plant can be designed to produce drinking water as a by-product. One barrier to commercial success for OTEC plants is the low energy conversion potential, about 4% (Vega, 2013). The amount of energy converted is directly corresponding to the temperature differential, so if this can be increased, OTEC plants will become more profitable.
In this proposition, heat energy is transferred via heat exchangers from used server cooling fluid to the OTEC plant. The working fluid then cools the multi-megawatt data center. The data center's electricity is thus reused as heat in the OTEC plant, and the retrieved heat energy helps to power the data center as electricity. As before, the OTEC plant can then potentially supply a dehydration plant with heat, and in any case serve the community with data access (Figure 5). A less intricate option is to provide the data center with seawater air conditioning (SWAC) from the DOW, and then use the waste heat for the dehydration facility.
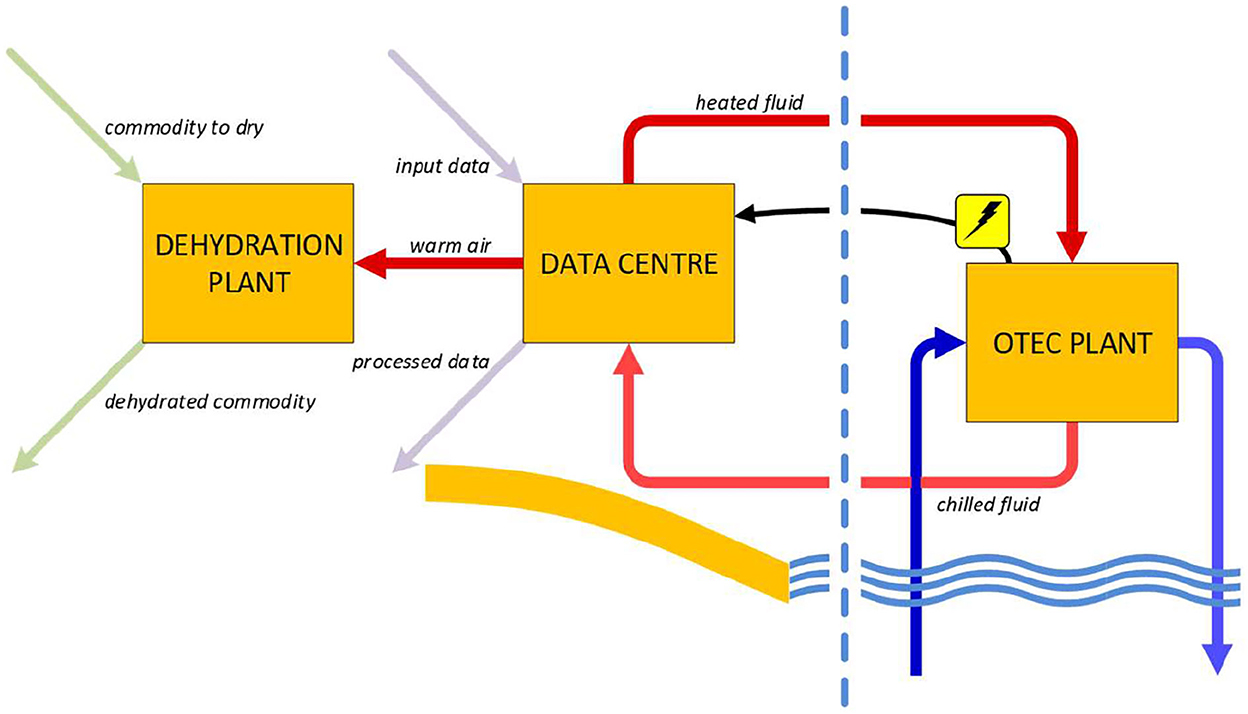
Figure 5. A tropical large-scale data center, and its industrial symbiosis with an OTEC plant and a dehydration plant.
OTEC plants can produce hydrogen gas through electrolysis of the sea water, and data centers can provide these plants with heat energy. Incidentally, it has been suggested that future data centers should rely on hydrogen fuel cells for backup power, to replace today's diesel backup solutions (Enterprise Ireland, 2022). Thus, not only is there a symbiotic relationship regarding electricity between data centers and OTEC plants, but also a supply- and demand-relationship regarding auxiliary power. Here, it should be noted that an ocean-based (possibly submerged) OTEC-powered data center can take advantage of the hydrogen gas as a backup when the plant needs maintenance.
3.2. Societies
In both low- and high-income regions, a little more than 30% of all produce goes to waste, adding up to millions of tons of food each year. The difference is that whereas in high-income countries a vast majority of the waste comes from the end-user (the consumer), in many low-income countries about 30% of the crops never reach a distribution network. Instead, they are either never harvested, or harvested but eaten by rodents or insects due to poor storing capabilities (Berners-Lee, 2021). With a data center's warm and dry airflow, a substantial amount of this produce can be dehydrated. Once dried, it is easier to store and transport the produce because of the substantial decrease in size and weight. Long term storage is also made possible this way, which is beneficial to homes lacking access to a refrigerator. A walkthrough of practical steps needed to be taken to enable this process is found in Terenius et al. (2021a).
As mentioned above, data center waste heat is currently being utilized, especially in the Nordics. It is not an expensive technology, but rather a way to increase the sustainability of the data center, and simultaneously provide the operator with a second revenue channel. The tropics face more challenges, not least southeast Asia. Here, at the center of the world (more than half of the world population lives in Southeast Asia, India or China), large data centers are built without reuse capabilities. They also suffer from much higher energy use than data centers in colder climates. With fast economic growth, growing populations and a high energy footprint, waste heat use would be especially attractive—and especially important.
3.2.1. Building a sustainable future for Malaysia
Malaysia is home to 33 million people and on the brink to achieve high-income status, much thanks to oil export. A quite hot country, where day temperatures seldom go below 30°C, can be regarded a challenge for waste heat use. Still, many of the ideas proposed in the previous section can be utilized also in this context.
Malaysia has a long tradition of semiconductor manufacturing. This industry started out as a grassroot initiative to bring workers back to a de-populating region of the country (Azzman Shariffadeen, interview May 7, 2022). In the late 1980s, ideas were presented to transform Malaysia into a knowledge-based nation and to achieve prosperity through ICT, thus expanding on knowledge acquired from the semiconductor manufacturing industries (Azzman Shariffadeen, 1988). Not long after, the president Mahathir bin Mohamad built the “Multimedia Super Corridor” in a new town called Cyberjaya, alongside the new governmental town Putrajaya. Cyberjaya has now become home to many data center operators. The town is large and spacious (see Figure 6). Hence, adding warehouses for commodity dehydration would be much easier here than in, say, Singapore, London or Amsterdam, where data centers are abundant, but available land severely limited and prohibitively costly.
A major agricultural producer, Malaysia has a number of commodities that could be dehydrated this way:
• Forestry products: Malaysia is a substantial timber producer, even when excluding Borneo (overseas transportation makes drying forestry products from Borneo in Cyberjaya less favorable). With about 7 million m3 produced wooden goods per annum in Peninsular Malaysia (Miyamoto et al., 2014), Cyberjayan waste heat may well be fully utilized solely to dry wooden products such as pellets and construction boards.
• Fish, fruit and vegetables: Malaysian commodities of these types often require dehydration, and a warehouse solution increases food security, whilst providing more evenly dried commodities. Dried food is also easier to store and export than fresh, and may therefore increase commercial opportunities for local producers.
• Seaweed: There is ongoing research at several Malaysian universities on seaweed farming. Thus, seaweed may, with time, become an important industry. If some of the seaweed could be dried in Cyberjaya to become biofuel, Malaysia's oil dependency would decrease.
The dehumidified air of a data center makes dehydration faster and more efficient. Still, with the high outdoor heat of the region in which Cyberjaya is placed, the efficiency gains may not be as favorable as in, for example, Japan, Europe or North America. Things may change soon, though. With liquid immersion cooling implemented, outgoing heat can reach 50°C or more. Increasing temperatures, in turn, increases the number of use cases for tropical data center waste heat, especially as liquids transport heat much better than air does.
Energy storage, in particular hydrogen gas production, is an important key to the future Malaysian economy (WWF Malaysia and Boston Consulting Group, 2021). As mentioned above, ocean-based OTEC is designed to support this scenario, since bringing electricity to shore from a floating plant far away is problematic. OTEC happens to be a technology that works well in the waters north of Malaysia, and important OTEC research is carried out in the nation. However, due to the shallow and warm waters in the Malaccan Strait, OTEC does not work closer to Cyberjaya and to the majority of the Malaysian population. This fact pushes the question of the suitability of an OTEC–data center industrial symbiosis to questions about geographical practicalities and the geopolitical worries about conflicts over Malaysian territory in the Chinese Sea.
Using the heat energy to “charge” salt hydrates is also viable for large and small Malaysian data centers. Still, this implies the salt hydrates must be shipped to a location having a demand for the salt hydrates, such as northern Europe. In turn, Europe has a large share of the global data center market, so provided this strategy pays off, Europeans could avoid shipping from southeast Asia and easily supply their own salt hydrates.
As expressed in the Green Technology Master Plan Malaysia 2017–2030, “a sectoral target does not only produce a single isolated outcome within the sector but also has concurrent outcomes in other sectors” (Ministry of Energy Green Technology Water Malaysia, 2017). This holistic attitude to industries' contribution to Malaysian prosperity gives hope for waste heat use in Cyberjaya.
3.2.2. Drying coffee beans in Costa Rica
Coffee is the world's most traded agricultural commodity in need of dehydration. An industry employing 60 million people (Sachs et al., 2019) and delivering almost 10 million tons of produce to the global market yearly, coffee production can have a substantial impact on consumption, values and climate, thus responding to the demands of many of the UN Sustainable Development Goals (SDGs). This is particularly valid if lessons learned from coffee production are used in other parts of the commodity sector, such as tea and cocoa production, and thereby multiplying the societal and environmental impacts. The fact that sustainable coffee connects to several of the SDGs has not gone unnoticed by the International Coffee Organization:
Higher coffee prices are associated with more rural employment, higher contribution of agriculture to GDP, lower levels of poverty (SDG 1), increased food security (SDG 2), reduced inequality (SDG 10), and higher political stability (SDG 16). Hence, policies that help to increase and stabilize income levels of coffee-producing households can have a significant impact on economic and social development … (International Coffee Organization, 2019)
Sachs et al. (2019) connect 14 of the SDGs to the coffee production sector, omitting only SDGs 10, 11, and 14. Terenius et al. (2021a), in turn, estimate that sustainable coffee production in low- and mid-income countries has high or very high contributions to 11 of the UN SDGs (all but 3, 6, and 14–17). In other words, with some incitement, the coffee production sector can become an enabler for sustainable communities. Still, that requires a catalyst—and the data center industry has the potential to be it.
Like Malaysia, Costa Rica is an upper-middle income country (International Monetary Fund, 2022). However, many of its five million inhabitants (and migrant coffee plantation workers from neighboring countries) live under the poverty line of USD 1.90 per day. The annual coffee export is 90,000 tons (International Coffee Organization, 2020). To dry these beans, roughly 25,000 MWh of electrical energy and up to 140,000 m3 of wood are needed (Terenius et al., 2021a).
As argued by Terenius et al. (2021a), it may be that for semi-rural areas in many low- and mid-income countries, the future lies in container-sized data centers. In the paper, it is shown that in theory, if these data centers could run at max capacity, fewer than 60 container-sized data centers would dry the entire coffee production. In reality, more would be needed, since a data center should not be run at full capacity. Moreover, the season drying length for coffee beans in Costa Rica is only five months. That said, during the rest of the year the data centers could help dry other commodities for small-scale industries, thus offsetting the same amount of electrical energy from those processes. Also, it is quite possible that with new technologies such as liquid immersion cooling, the available heat would be hotter.
If fully implemented, this idea would also bring an additional estimated 10–20% of possible data storage and computing to Costa Rica, increasing network capacity and stability. More importantly for the local community, the idea would enable computation closer to the source (“edge” computing). That, in turn, would increase data speed locally, and lessen the amounts of data in need of shuffling to the capital San José as well as abroad.
3.2.3. Sweden and the new Klondike
The use cases above involved tropical, mid-income countries. However, the material social concerns of the data center industry also stretches to the wealthier regions of the far north.
Sweden, like its Nordic neighbors Norway and Finland, has some excellent conditions for data center establishment: a climate that enables free cooling, plenty of renewable, stable energy (mainly hydropower), populations with excellent foreign language-speaking skills and a deeply rooted concern for the environment, political stability and practically no censorship, tectonically safe land, a history of practical, innovative solutions to technical problems, well-established road, railway and Internet infrastructure, a collaborative spirit between municipalities and local industries and—like some other countries (Skatteverket, 2015)—discounted energy tax for energy-intensive industries. On top of this, Sweden is built around district heating, making waste heat recovery possible.
The reasons above speak for Sweden—again, together with Norway and Finland—as a leading future data center nexus, and a glance at Figure 3 confirms this notion. Interviews among decision-makers (Dybdal Christensen et al., 2018; Korhonen, 2018) further support this argumentation, pointing to many of the factors mentioned above. These countries are also actively researching future prospects for data center waste heat: fish and lobster farming is tried in Norway, Luleå Technical University researches commodity dehydration in Sweden, and Finnish industry experts have developed ISO standards for data center waste heat recovery (Suomen Standardisoimisliitto SFS RY, 2021).
Sweden is to 70% covered with forests. The main rivers used for hydropower are found in the north, a relatively low-populated region. The last half century has seen a migration from the north to the southern part of Sweden, the result of reduced employment opportunities. For the Swedish government, establishing large-scale data centers was a part of a strategy to stop this gradual depopulation (Harding, 2015).
Against this background, it is not surprising that the data center industry suited both the Swedish government and foreign heavy data users well. In 2011, Facebook built their first European data center outside Luleå in northern Sweden, and Google and Microsoft followed suit. Nowadays, operators in Stockholm sell their waste heat for district heating (Velkova, 2016). In this way, data centers transform into tax-relieved electric heaters with the ability to store and compute data. On top of monetary savings, this method has both societal and environmental benefits. The technical manager at the city-owned energy company Stockholm Exergi explains: “Increased digitization gives the city's residents new opportunities while the heat from the data centers is taken care of and contributes to a more sustainable energy system. You can say that the electricity is used twice—first in the servers, then for heating” (Conapto, 2022).
A decade after Facebook's establishment, the data center industry has brought employment opportunities to Sweden, and the once depopulating northern counties are now repopulated, turning northern Sweden into what's been referred to as a new “Klondike” (Myrén, 2021). Clearly, despite a per capita wealth four-five times higher than Malaysia's and Costa Rica's (International Monetary Fund, 2022), Sweden was subjected to the same migratory patterns that haunted Malaysia. And in both cases, the IT industry helped to turn the tide.
3.3. Metrics
An old saying in business is “you can't manage what you can't measure”. But what about occasions when metrics are not being used the way they were designed to? In the data center industry, energy efficiency is primarily measured in Power Usage Effectiveness (PUE). As shown in this section, the industry needs better metrics to capture the value of waste heat.
3.3.1. Power usage effectiveness
Proposed in 2006 (Malone and Belady, 2006) to deal with the high energy costs associated with data center cooling, PUE was soon adopted by the data center industry and by other stakeholders (The Green Grid, 2010a). Fifteen years after PUE's inception, it is still the most used data center energy use metric. It formalizes energy efficiency according to this formula4 (The Green Grid, 2007):
PUE soon became an ISO standard (ISO/IEC 30134-2:2016), thus establishing its conclusive definition: “ratio of the data center total energy consumption to information technology equipment energy consumption, calculated, measured or assessed across the same period” (International Organization for Standardization, 2016a).
The way the formula is defined means that the lower PUE, the less energy is needed for auxiliary processes. When all incoming energy is used solely by servers, that is, when no energy is needed for cooling or other supporting uses, PUE becomes one. Conversely, everything above PUE=1 is overhead. This overhead is what has primarily been addressed by data center constructors and engineers—and for some years, this focus made sense as the overhead was, at the time, quite high in the industry, often exceeding 100%.
One striking drawback of the formula is that it works as a seesaw: efficiency gains in cooling reduces PUE, but efficiency gains in IT equipment (more efficient servers, fewer idle servers, better memory management in server software and so forth) reverse the dynamic and instead increase PUE proportionally, as shown in Table 1. Therefore, “PUE corresponds poorly with energy and carbon efficiency” (Horner, 2016). Further, as Uptime Institute puts it: to rely “too heavily on PUE as the industry's key efficiency metric may reduce operators' motivation to pursue IT efficiency improvements” (Davis et al., 2022).
In other words, PUE cannot be used as an all-encompassing metric for data center energy efficiency. Yet, that is exactly how it has been used for the last 15 years, by managers, climate-conscious clients and politicians alike (Horner, 2016). There are several other drawbacks to using PUE as an overall metric (paper in preparation). Some of them are not primarily engineering problems, which may be why they—and PUE itself—have been able to persist in this engineering-heavy industry.
Some metrics see to more holistic energy utilization within the data center (e.g., Vasques et al., 2019). A few others aspire to look beyond it instead. Since this paper is mostly concerned with waste heat reuse, one metric of special interest is Energy Reuse Effectiveness (ERE). Introduced by the industry organization The Green Grid (2010b), ERE is formulated this way:
Thus, ERE extends PUE by reducing reused energy from the nominator. Introduced together with ERE, the Energy Reuse Factor (ERF; ISO/IEC 30134-6) is the amount of reused energy divided by the data center's total energy. Similar metrics exist, such as Carbon Usage Effectiveness (CUE; ISO/IEC 30134-8), which is the ratio between the data center's and its IT equipment's CO2 emissions.
The Renewable Energy Factor (REF; ISO/IEC 30134-3), finally, is the “ratio of the renewable energy … owned and controlled by a data center to the total data center energy consumption” (International Organization for Standardization, 2016b). A problem recognized by the ISO is the underlying definition of renewable energy, stating—with reason—that criteria to “categorize an energy source as renewable can differ among jurisdictions based on local environmental or other reasons” (International Organization for Standardization, 2016b). In other words, REF can fluctuate depending on policy decisions. When the EU in 2022 declared fossil gas sustainable power (Hancock, 2022), data centers powered in such a manner would wrongfully have enjoyed substantially improved REFs.
3.3.2. The quest for new data center energy efficiency metrics
Despite its usefulness for minimizing overhead power, PUE was never designed for making holistic measurements. Nevertheless, throughout the industry and among other stakeholders, PUE has unfortunately often come to represent overall data center sustainability. As a matter of fact, Amsterdam, one of the most important European data center hubs (Brocklehurst, 2021) placed a cap on data centers already in 2008 based on their PUE ratings (Laan, 2008). Singapore, home to many data center providers in southeast Asia, followed suit a few years after (Swinhoe, 2022). China too bases planning permissions on PUE (Industry Information Division, 2021).
Just how problematic PUE is can be illustrated by Google's sustainability reports, which use yearly PUE rating improvements as a token of successful sustainability efforts (Google, 2020). With a stronger emphasis on power-hungry AI computation, a higher IT equipment use is to be expected, in turn lowering the PUE because of the increased energy use. This is especially concerning since web advertisement services are at the core of Google's business model, indicating that their AI efforts drive consumption more efficiently than before.
Over the years, many suggested replacement metrics for PUE have been put forward (see Reddy et al., 2017 for a large collection of sustainability-related metrics). Since each data center is different and will have a different power profile, it is difficult to accurately describe adequate power and energy use. For instance, a data center mainly used for long time data storage, with reliability, safety and security as primary concerns, would have other demands and opportunities than one dedicated to power-hungry cryptocurrency mining. In this case, a stakeholder using PUE as a sustainability indicator would likely incorrectly report the cryptocurrency mining facility as more sustainable, due to its high power usage. Neither can ERE and ERF provide a complete picture of energy reuse options. For example, ERE and ERF do not specify transportation losses and conversion losses, and from a financial perspective they fail to acknowledge that the heat energy retrieved could have been gathered from somewhere else, say, from other industrial waste heat.
From a holistic perspective, it should also be noted that neither PUE nor ERE/ERF incorporate the quality of the electrical power going to the data center. How sustainable is it? And even when this power is based on 100% renewable energy—giving an impressive REF of 1—it may be asked whether there could have been better societal use for it. In worst case, highly profitable data center operators may set up operations in Sub-Saharan countries, use scarce renewable energy resources to provide targeted ads to customers, claim that the operations are 100% sustainable, and use ISO standards to support their claim.
3.3.3. Introducing the datacenter energy sustainability score
Reddy et al. conclude that none of the more than hundred metrics they investigated allow for just comparisons between data centers (Reddy et al., 2017): there are simply too many variables to consider. One solution to the problem is to grade data center energy concerns (see Figure 1) individually, and add them together. This is done in the following working metric, Datacenter Energy Sustainability Score (DESS), which this work proposes as a complement to existing energy efficiency metrics:
The three constituents of DESS all have values between 0 and 1, and are
• EnergyIn—this is a ratio, not to be confused with renewable energy per se. As explained above, a data center operator using up a society's available renewable energy cannot be regarded sustainable. When sustainable energy is plenty, the ratio is the amount of energy derived from renewable energy sources divided by total energy. It should be noted though that also sustainable energy sources come with different environmental and societal footprints.
• EnergyCompute—this part of the formula is 1 minus PUE overhead. PUE is certainly not the best way to show energy use within a data center, or the usefulness of that work. Future endeavors will hopefully complement PUE with a metric that instead specifies the amount of useful work carried out per energy used (though a challenge would be to define “useful work”; a computer engineer might think of floating point operations per second, a business person of computation/dollar profit and a sociologist of computation that somehow benefits a society).
• EnergyReuse—this unit indicates the amount and degree of usefulness of heat reclaimed outside of the data center for a given purpose, such as commodity dehydration in a certain societal context. ERF is similar, but omits the comparable usefulness of EnergyReuse (see below).
Each constituent has a few members, as shown in Table 2. The exact constituents of the metric will need further investigation and collaboration between different parties. Indeed, the goal is not precision: rather than advocating for exactness, DESS is an early attempt to transform a highly engineer-centric worldview into a material social. This transformation, in turn, necessitates interventions with actors from disciplines outside engineering.
The equation shows that the constituents are not graded equally. This is because of adherence to the circular economy principles. As a starting point, the incoming resource (electricity in this case) should be sustainably obtained. Restricted energy use relates to the Reduce principle, and waste heat reuse to Recycle and Reuse. Of these, Energy use is seen as least important, since if all energy is reused, the climate impact is low. That being said, an energy-inefficient data center puts strain on the climate due to unnecessary hardware consumption. The factors 3−1−2 provide a crude but efficient way to represent these concerns, as evidenced by Table 3 and Figure 7.
It is possible to rewrite the DESS formula with a combination of existing metrics, but it is introduced here to mirror the simplicity of Figure 1, to enforce a perspective that stretches beyond engineering concerns, and to retain flexibility as the metric evolves. Like PUE, DESS relates to energy use only. Therefore, similar metrics may be needed, not least for carbon emissions (Datacenter Carbon Emission Sustainability Score, DCESS), land use (Datacenter Land Use Sustainability Score, DLUSS), water use (Datacenter Water Use Sustainability Score, DWUSS), and social issues (Datacenter Social Impact Sustainability Score, DSISS). Weighed together, they would create a scorecard solution (Datacenter Overall Sustainability Score, DOSS).
The following section compares DESS to PUE for the earlier use cases.
3.4. Connecting the perspectives
Table 2 and Figure 7 show 16 scenarios, spanning a variety of climates, economies and access to renewable resources.
To facilitate comparisons between the data, all share TotalPower and most share sustainability coefficients (0.9 is used rather than 1, even in cases where 100% renewable energy is utilized, to compensate for the fact that energy could have served other purposes, and that most energy sources are limited).
To represent future installations, PUE values is set to represent current leading endeavors:
• 1.60 is the reported value of NTT's flagship Cyberjaya Data Center 5 (NTT, 2022).
• 1.60 is also used for the container-sized data centers of Costa Rica. This value would differ based on data center configuration and computational load. The climate is colder than in Cyberjaya, indicating the possibility of lower PUEs, but it is more difficult to attain low PUEs in small data centers, pushing PUE up again.
• 1.25 is used for data centers in the UK and North America. To facilitate comparison, so is the coal-powered data center.
• The reported PUE for EcoDataCenter, 1.15,5 is used for the Swedish and Finnish examples.
• The reported average value for Google's data center fleet is 1.12 (Google, 2020), and for Microsoft's submerged Natick containers it is 1.07.6
EnergyReuse estimates are based on the many factors of the analytical framework, the literature study and the research visits carried out during the project. They should be read as follows:
• In Cyberjaya, 80% of the waste heat is reused, and the usefulness of that heat is 70%.
• In Costa Rica, 65% is reused to dry coffee, but also leather, fruit etc. The usefulness of the heat is higher than in Cyberjaya's case, since Costa Rican coffee is grown on high altitudes, featuring less sunshine, a colder climate and weaker transportation infrastructure.
• In one of the examples from the Nordics, district heating is only used in winter (no district cooling is accounted for), thus 50%. The heat is seen as quite valuable.
• In the other example, since the heat is reused all year around, its reuse is set to 90%. However, drying pellets is (here) less efficient than district heating, thereby lowering overall yearly usefulness.
• The Californian data center lacks waste heat reuse, and is powered through an energy mix, consisting of the statewide average 40% fossil fuel dependency (Nyberg, 2022).
• Google's data centers are sustainably powered (Google, 2020). Since Google's data centers are found in many parts of the world, reuse options are unrelated to specific uses and geographical and societal contexts.
• In 2022, the Fiber Blackpool Cooperative Alliance announced a sustainable data center nexus on England's western coast. The data centers will be powered by an ocean-based wind farm.7 Since Blackpool is a beach resort that hosts a number of swimming pools, data center waste heat can potentially be used as-is, heating these pools. Other heat would be substituted in the process, which is why reuse and usefulness values are high.
• The Natick tests have not included waste heat use. However, should the outgoing heat be connected to an enclosed environment, it is expected that submerged data centers can enhance fish or shellfish production in some locations.
• The coal data center in this example fares well in terms of PUE but not in DESS.
• To clearly illuminate the differences between PUE and DESS, two unrealistic coal-powered use cases are added, one with 100% reuse and one with no reuse but PUE = 1.
Figure 7 highlights the discrepancies even more clearly than Table 3 does, showing PUE and DESS side by side. PUE and DESS are not truly compatible, as PUE has no upper limit. In this figure, however, PUE = 2 is set as the maximum tolerated number; anything higher would be unacceptable in a modern data center.
The differences between the resulting PUE and DESS values are telling. Unsurprisingly, accounting for renewable energy and reused heat energy makes—correctly—a big difference for DESS. For example, the coal-powered data centers perform very well as far as PUE is concerned, but terribly in terms of DESS. Tables 2, 3 also provides several reasons why DESS should interest the industry, clients and legislators:
1. The advantage PUE erroneously gives Google over the “Nordics” and “Blackpool” data centers (which reuse their heat) is corrected with DESS. In other words, the new metric makes it easier for EcoDataCenter and similar enterprises to compete with sustainability arguments.
2. Just as importantly, DESS provides governments on national, regional and local levels with a more valid key metric for their regulations. Set correctly, DESS-based regulations should result in newbuilds with higher sustainability foci.
3. With a more accurate metric in use among decision-makers, data center owners get an incitement to invest wiser. DESS is designed to encourage savings and even earnings from the three parts of the energy chain, rather than solely point to advanced and expensive cooling systems as the main strategy to improve ratings. On that note, it should be stressed that at least for the time being, what's inside the data center will be measured with PUE also in DESS, so no additional work needs to be carried out for data center managers.
4. Discussion
Data centers are Janus-faced creatures. On the one hand, they are industry buildings, in need of power, water, roads, competent operating personnel and so forth. On the other hand, they possess a mythical aura, filled with surveillance data, credit card details, and people's innermost thoughts.
Waste heat, too, is two-faced, or rather, elusive. From a distance, data center waste heat evades the senses. However, it can be quite tangible, especially when one stands in front of the waste heat airflow in a snowy landscape (Figure 8). This paper aims to bridge the gap between these two different personas, and between the different worlds of social scientists and engineers.
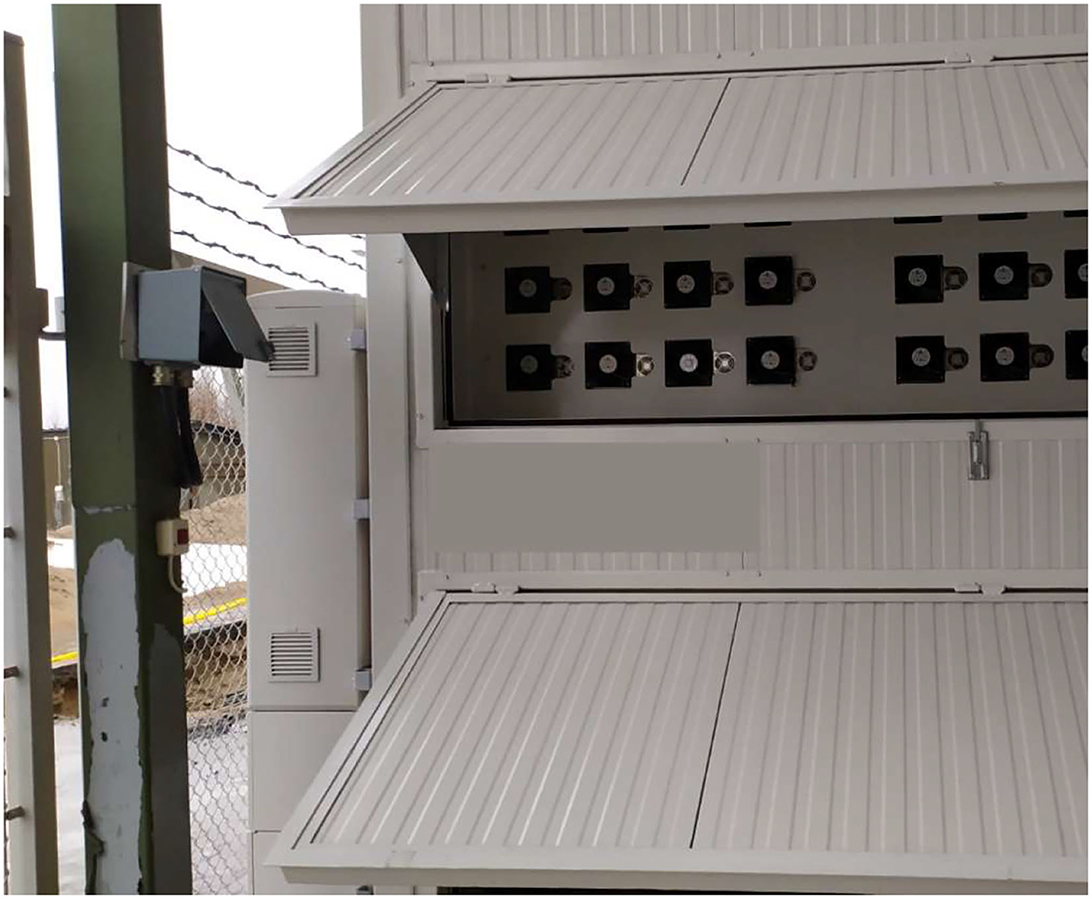
Figure 8. An outdoor data center in northern Sweden. It may be a cold and snowy landscape, but seemingly the paint has been flaked off the pole from the waste heat of the crypto-mining servers. Photo: P. Terenius.
4.1. Key findings
This study set out to view data center energy problematics through a material social lens, starting with the premise that waste heat use may benefit the data center industry, society and the environment more than additional energy-savings within the building. Then, the feasibility of a few novel applications for data center waste heat reclamation was shown, and a new (working) metric, DESS, was introduced. Designed for holistic views on data center energetics, DESS provided a more accurate picture of data center energy sustainability matters than what PUE can ever do. In Table 3 and Figure 7, DESS helped to reveal the difference between an internally efficient coal-fired plant without heat reclamation and data centers served by renewable energy and with a high degree of energy reuse. Further, DESS illustrated how tropical data centers can make sense from both environmental and societal perspectives.
Some of the more significant findings to emerge from this cross-disciplinary study are:
• Data center waste heat is a promising resource regardless of location, data center size and societal structure (that is, some of the dimensions of concern found in Figure 3).
• Since many societal and engineering factors pertain to data centers, their optimal placement must be defined on a case-by-case basis.
• To avoid conversion losses, waste heat should preferably be used as-is.
• A reasonably energy-efficient data center with proper waste heat reclamation can be more sustainable than data centers approaching PUE = 1 but lacking waste heat reclamation.
• It is clear that a successful data center sustainability strategy stretches far beyond the building itself. In fact, modern data centers are so efficient that data center sustainability is not any longer primarily an engineering issue, but a combination of questions that requires a multi-disciplinary approach.
The use of mixed methods and triangulation have benefitted the project. Brought together, the different research approaches point to—to quote Yin (2012)—“the same set of events, facts, or interpretations”: again, data center waste heat use is possible in both cold and warm climates and regardless of societal structures and local financial situation.
4.2. Interpreting the results
Based on the analytical framework, it was found that the data center industry can benefit the three studied nations in different manners. In Sweden, the data center industry provides some employment opportunities. But, more importantly, it brings technical expertise, future industry opportunities and hopefully a spin-off effect on academia—Luleå University, a stone's throw away from Europe's premier Facebook data center, is one example. In sparsely populated areas, far away from the largest Swedish universities, this makes political sense.
For millisecond-critical applications, the Arctic region of the Nordics is not the best choice due to the time it takes for light to travel from the user to the data center; Cyberjaya would make more sense for a large part of the world's population. However, for massive time-indifferent computation, streaming services, long-term storage or more common response times, the Nordics (and possibly Canada) seem to have unequaled capabilities. The greenhouse effect does not recognize territorial borders, so to limit global greenhouse gas emissions, Sweden, Norway and Finland should be among the most suitable locations for data center establishment—provided outgoing heat is reused.
Malaysia is in a good position to supply future generations of southeast Asia with high-speed computation. In the late 1980s, Dr. Azzman Shariffadeen envisioned that an established data center industry would propel Malaysia—its rural areas included—into a knowledge-based nation (Azzman Shariffadeen, 1988). Whilst the data center industry has now been built, it is yet to be seen whether the final step of this transformation will come to fruition. As a complement to Cyberjaya, placing data centers in Johor Bahru, on the border to Singapore and home to Malaysia's technical university, may be beneficial.
Compared to continued growth in these two data center-important nations, placing rurally positioned small-scale data centers on the Costa Rican map, with the prospects of local community empowerment, may seem less straight-forward. On the other hand, with small-scale visions follows small-scale investment. Even for purely experimental reasons, trying this idea would be relatively inexpensive for data center providers or Big Tech. And should that project pay off, a whole new market segment opens.
Although the three use cases show many differences, not least in ambient temperature, there are also important similarities. One is the possibility of energy reuse. Another similarity is the hope for know-how and industrial and/or academic spin-off effects, thus the belief in the data centers' end product: data. These effects are enablers for achieving more knowledge-based nations, in turn providing hope for sustainable futures, locally and globally.
4.3. Limitations
The scope of this study was limited in terms of access to real data. This is a general problem for data center industry research, as such information is not readily published on regular basis by data center providers. However, even with detailed data provided, the many types of data center configurations, of server models used and of computation workloads run on these servers give very different results, so those data would still have limited value. And in any case, this paper views the data center as somewhat of a black box, since much research has been carried out on energy savings within the data center (see Section 4.4), and since, as shown in this paper, PUE improvements is not where the majority of future energy savings can be made.
Another limitation is that this paper only relates to energy use. Thus, it is not concerned with all aspects of a data center, such as building concerns accredited through LEED certifications (though many leading industry players use these too—see, e.g., Equinix, 2021). Likewise, it is not concerned with carbon emissions, or for that matter, with the life-cycle of servers—itself an important topic. Hopefully, this paper can inspire to such future work.
Finally, more cases would provide a more detailed view of the problems addressed, and hopefully indicate patterns for future strategies. Although the current study is based on a small example set, the findings make a strong argument for waste heat use as well as for material social solutions—in turn relying on systems engineering and systems science—to engineering problems. Hence, to keep climate change at bay and lower the data center industry's strain on regional and national energy grids, further cross-disciplinary investigation and experimentation into this matter are strongly recommended.
4.4. Prior research
There is a wealth of literature on renewable energy, and in that respect, the data center industry is not that different from other high-energy users. The various constituents of the data center itself—server racks, cooling systems, and so forth—have been studied individually, often as part of other disciplines, mainly engineering. However, their specific integration within a data center has been studied much less. Some researchers, such as Ebrahimi et al. (2014), Guitart (2017), Li et al. (2018), Levy (2019), Cheng et al. (2021), and Manganelli et al. (2021), have studied the relationships between data centers' internal components. Even at an early stage, Jamalzadeh and Behravan sought to establish a holistic framework of data center-related metrics (Jamalzadeh and Behravan, 2012). Some followed, such as Zakarya (2018). Today, a decade after Jamalzadeh and Behravan, holistic ways to lower data center energy use are still investigated, but now using AI (Mahbod et al., 2022). In addition to the academic literature, industry recommendations exist, from Google (Barroso et al., 2019) and from accreditation bodies such as the Uptime Institute. However, more research is needed here, not least with regards to “future” technologies now being deployed, such as liquid immersion cooling. Lei and Masanet (2020) have explored energy use for free cooling scenarios, and Wan et al. (2021) waste heat integration, but again, from an engineering viewpoint.
Some researchers have investigated the societal impact of data centers. The anonymity of data centers has been studied by Hu (2015) and Vonderau (2021), and how they underpin AI activities, with a slew of ethical problems, was recently dealt with by Crawford (2021). A similar approach was taken by Lucivero (2019). Broader society-related connotations have been investigated by Velkova (2016), Sovacool et al. (2022), and again, Vonderau (2019).
Using OTEC in conjunction with industrial waste heat is not an entirely new idea. For example, researchers have examined the use of heated water from nuclear power plants or gas plants (Kim et al., 2009; Soto and Vergara, 2014). It has been found that with the elevated warm water temperatures, not only can the OTEC process be more efficient, but also used in waters that are typically too cold for the technology to be economically feasible. Incidentally, industrial and cultural factors enabling marine technologies such as OTEC in low- and mid-income countries (Göthberg et al., 2021) resemble those that have worked in favor for the Nordic data center industry (see Dybdal Christensen et al., 2018).
4.5. The path forward
This research project can hopefully inspire research groups in academia and in the data center industry to increase much needed work efforts regarding data center sustainability. Due to its holistic approach, this forward-looking paper has many facets. Identified needs for further research relate primarily to success factors, use case data, possible uses and metrics.
Much disciplinary-specific as well as multi-disciplinary research is needed to investigate the suitability of various solutions for commodity dehydration, electricity production and energy storage applications in various global contexts. Hopefully, the analytical framework may help in these endeavors. For many nations, it would help studying the success factors of the Nordics (see Dybdal Christensen et al., 2018; Brocklehurst, 2021 as starting points) and note what political, financial, technical and cultural factors may play to their own specific advantage.
However, defining success factors is just a start. It is equally—if not even more—important to obtain additional data from existing and modeled data center waste heat use cases. The industry and academic institutions need to collaborate in this work.
There are more possible uses for data center waste heat than those discussed in this paper. To avoid additional investments and energy conversion losses, waste heat should preferably be used as-is, so identifying such uses needs further work.
Finally, practitioners, academia and government representatives must work together on holistic metrics for evaluation of societal and environmental impacts of planned data centers.
5. Conclusion
For the last decade, engineers from academia and the data center industry have tried to find an appropriate successor to PUE. That the work has largely been unsuccessful is not strange—this is not merely an engineering problem. Indeed, there exists a bond between metrics, applications and societal context: what works in one society may not work well in another, and new metrics must be designed for stakeholders to make better decisions. Thus, to become more sustainable, future data center placement must be more carefully chosen, and rely on a multitude of factors.
Using a material social approach, this work contributes to existing knowledge of data center energetics by providing a truly holistic perspective on the matter at hand. Whilst the paper does not provide answers for every specific location or data center size, its three use cases indicate that data center waste heat use has societal and environmental value under very different conditions. In fact, DESS shows that reusing heat energy can have much greater benefit than larger and larger investments in gradually smaller energy savings within the data center. Furthermore, an energy-efficient data center powered with coal fares very well in terms of PUE, but is properly punished using DESS. In other words, where PUE falls short, DESS correctly shows that a data center in agricultural communities in, say, Costa Rica, can make more environmental, social and financial sense than yet another template data center in the United States, and even that sustainably powered data centers with heat reclamation in Cyberjaya may become more sustainable than data centers sunk in the ocean.
It is clear that a more holistic understanding of data and energy is required: after all, the data center is only one piece of the societal puzzle. Therefore, optimizing sustainability work requires both a site-specific understanding and a holistic approach to the capabilities of technology, of people, of nature and of energy itself.
Data availability statement
Publicly available datasets were analyzed in this study. This data can be found here: https://www.imf.org/en/Publications/WEO/weo-database/2022/April/weo-report.
Ethics statement
The studies involving human participants were reviewed and approved by the Faculty of Science and Technology Research Ethics Committee (FSTREC) at Lancaster University. The patients/participants provided their written informed consent to participate in this study.
Author contributions
PT: conceptualization, methodology, investigation, original draft, and review and editing. PG and RH: supervision and review and editing. All authors contributed to the article and approved the submitted version.
Funding
This work was supported by the Leverhulme Trust Doctoral Scholarship Program (DS-2017-036) and the Engineering and Physical Sciences Research Council (EP/P031617/1).
Acknowledgments
The authors would like to thank Dr. Sathiabama T. Thirugnana and others at Universiti Teknologi Malaysia for their expertise and for help arranging meetings in peninsular Malaysia; Dr. Mattias Vesterlund and colleagues at Research Institutes of Sweden for insights in their current research; Dr. Andy Lawrence at the Uptime Institute for views on metrics; Dr. Lars G. Golmen from the Norwegian Institute for Water Research for OTEC discussions; the International Coffee Organization for sharing working data; Dr. Drew Lilley at UC Berkeley for pointing to salt hydrates; staff at EcoDataCenter for showing the premises; Reviewer JS for ISO metrics discussions, CEO Ulf Lindvall at Lindvalls Kaffe for sharing his experiences from the international coffee industry; Dr. Tangku Mohd Azzman Shariffadeen for sharing the story of Malaysia's transformation; Operations Manager Daniel Widman at Falu Energi & Vatten AB for presenting their pellet dehydration work; Prof. Phang and Dr. Yeong at Universiti Malaya for discussions on seaweed farming; WWF Malaysia for the conversation regarding their report findings; the team and participants at the Material Social Futures program. Last but not least, many thanks to Damian Borowiec at Lancaster University's Experimental Distributed Systems Lab for 3 years of discussions on data center energy metrics.
Conflict of interest
The authors declare that the research was conducted in the absence of any commercial or financial relationships that could be construed as a potential conflict of interest.
Publisher's note
All claims expressed in this article are solely those of the authors and do not necessarily represent those of their affiliated organizations, or those of the publisher, the editors and the reviewers. Any product that may be evaluated in this article, or claim that may be made by its manufacturer, is not guaranteed or endorsed by the publisher.
Footnotes
1. ^https://greenmountain.no/2021/06/22/data-center-heat-reuse/ (accessed September 26, 2022).
2. ^https://ecodatacenter.se/sustainability/ (accessed October 14, 2022).
3. ^https://natick.research.microsoft.com/ (accessed September 26, 2022).
4. ^The original version uses “power” rather than “energy”, in line with “Power Usage Effectiveness”.
5. ^https://ecodatacenter.se/sustainability/ (accessed October 14, 2022).
6. ^https://natick.research.microsoft.com/ (accessed September 26, 2022).
7. ^https://ethicallypowereddata.com (accessed October 14, 2022).
References
Ascierto, R., and Lawrence, A. (2020). Global Data Center Survey 2020. New York, NY: Uptime Institute.
Azzman Shariffadeen, T. M. (1988). Microelectronics, Information Technology and Society. Kuala Lumpur: Malaysian Institute of Microelectronic Systems [formally not published; only surviving copy is at National Library of Malaysia].
Bansode, P. V., Shah, J. M., Gupta, G., Agonafer, D., Patel, H., Roe, D., et al. (2018). Measurement of the thermal performance of a single-phase immersion cooled server at elevated temperatures for prolonged time. International electronic packaging technical conference and exhibition. Am. Soc. Mech. Eng. doi: 10.1115/IPACK2018-8432
Barroso, L. A., Hölzle, U., and Ranganathan, P. (2019). The Datacenter as a Computer: Designing Warehouse-Scale Machines, Third Ed. San Rafael, CA: Morgan and Claypool.
Berners-Lee, M. (2021). There Is No Planet B: A Handbook for the Make or Break Years Updated Edition. Cambridge: Cambridge University Press.
Blackwell, A. F. (2021). Ethnographic artificial intelligence. Interdiscip. Sci. Rev. 46, 1840226. doi: 10.1080/03080188.2020.1840226
Brocklehurst, F. (2021). International Review of Energy Efficiency in Data Centres for the Australian Department of Industry, Science, Energy and Resources. Oxford.
Cheng, H., Liu, B., Lin, W., Ma, Z., Li, K., and Hsu, C. H. (2021). A survey of energy-saving technologies in cloud data centers. J. Supercomput. 77, 13385–13420. doi: 10.1007/s11227-021-03805-5
Clidaras, J., Stiver, D. W., and Hamburgen, W. (2009). Water-Based Data Center. USA Patent Application 11/679,013. Mountain View, CA: Google.
Crawford, K. (2021). Atlas of AI: Power, Politics, and the Planetary Costs of Artificial Intelligence, New Haven, CT; London: Yale University Press.
Creswell, J. W., and Garrett, A. L. (2008). The “movement” of mixed methods research and the role of educators. South Afr. J. Educ. 28, 321–333. doi: 10.15700/saje.v28n3a176
Davies, G., Maidment, G., and Tozer, R. (2016). Using data centres for combined heating and cooling: an investigation for London. Appl. Thermal Eng. 94, 296–304. doi: 10.1016/j.applthermaleng.2015.09.111
Davis, J., Bizo, D., Lawrence, A., Rogers, O., and Smolaks, M. (2022). Global Data Center Survey 2022. New York, NY: Uptime Institute.
Dybdal Christensen, J., Therkelsen, J., Georgiev, I., and Sand, H. (2018). Data Centre Opportunities in the Nordics: An Analysis of the Competitive Advantages. Copenhagen: The Nordic Council.
Ebrahimi, K., Jones, G. F., and Fleischer, A. S. (2014). A review of data center cooling technology, operating conditions and the corresponding low-grade waste heat recovery opportunities. Renew. Sustain. Energy Rev. 31, 622–638. doi: 10.1016/j.rser.2013.12.007
Enterprise Ireland (2022). Towards Net-zero Construction in Energy Intensive Sectors. Experiences from the Decarbonisation of Mission-Critical Facilities. Dublin: Enterprise Ireland.
Evans, D. M., Parsons, R., Jackson, P., Greenwood, S., and Ryan, A. (2020). Understanding plastic packaging: the co-evolution of materials and society. Global Environ. Change 65, 102166. doi: 10.1016/j.gloenvcha.2020.102166
Freitag, C., Berners-Lee, M., Widdicks, K., Knowles, B., Blair, G. S., and Friday, A. (2021). The real climate and transformative impact of ICT: a critique of estimates, trends, and regulations. Patterns 2, 100340. doi: 10.1016/j.patter.2021.100340
Göthberg, M., Hammar, L., Carneiro, G., Mattsson, Y., and Sundman, M.-L. (2021). Enabling Local Blue Growth in Developing Countries. A Thematic Review. Gothenburg: Göteborg.
Gregson, N., Crang, M., Fuller, S., and Holmes, H. (2015). Interrogating the circular economy: the moral economy of resource recovery in the EU. Econ. Soc. 44, 218–243. doi: 10.1080/03085147.2015.1013353
Guitart, J. (2017). Toward sustainable data centers: a comprehensive energy management strategy. Computing 99, 597–615. doi: 10.1007/s00607-016-0501-1
Hancock, A. (2022). EU Parliament Votes to Designate Gas and Nuclear as Sustainable. London: Financial Times.
Harding, L. (2015). The Node Pole: Inside Facebook's Swedish Hub Near the Arctic Circle. London: The Guardian.
Harman, T. D. (2008). Waste Heat Recovery in Datacenters: Ejector Heat Pump Analysis. Atlanta: Georgia Institute of Technology.
Horner, N. C. (2016). Powering the Information Age: Metrics, Social Cost Optimization Strategies, and Indirect Effects Related to Data Center Energy Use. Pittsburgh, PA: Carnegie Mellon University.
Huang, P., Copertaro, B., Zhang, X., Shen, J., Löfgren, I., Rönnelid, M., et al. (2020). A review of data centers as prosumers in district energy systems: renewable energy integration and waste heat reuse for district heating. Appl. Energy 258, 114109. doi: 10.1016/j.apenergy.2019.114109
Industry and Information Division (2021). New Data Center Development Three-Year Action Plan (2021-2023). Beijing: Industry and Information Division.
International Coffee Organization (2019). Coffee Development Report 2019: Growing for prosperity. London: International Coffee Organization.
International Coffee Organization (2020). Coffee Production Report April 2020. Available online at: http://www.ico.org/trade_statistics.asp?section=Statistics (accessed December 28, 2022).
International Monetary Fund (2022). World Economic Outlook Database: April 2022, in Fund, I. M. (ed.). Washington, DC: International Monetary Fund.
International Organization for Standardization (2016a). ISO/IEC 30134-2:2016(en). Geneva: International Organization for Standardization.
International Organization for Standardization (2016b). ISO/IEC 30134-3:2016(en) Information technology - Data centres - Key performance indicators - Part 3: Renewable energy factor (REF) Geneva: International Organization for Standardization.
Ipsen, H. A. (2018). Catching the Cloud and Pinning It Down: the Social and Environmental Impacts of Data Centers. Syracuse, NY: Syracuse University.
Jamalzadeh, M., and Behravan, N. (2012). An exhaustive framework for better data centers' energy efficiency and greenness by using metrics. Indian J. Comput. Sci. Eng. 2, 813–822.
Judge, P. (2022). Drought-Stricken Holland Discovers Microsoft Data Center Slurped 84m Liters of Drinking Water Last Year. Data Centre Dynamics. Available online at: https://www.datacenterdynamics.com/en/news/drought-stricken-holland-discovers-microsoft-data-center-slurped-84m-liters-of-drinking-water-last-year/ (accessed August 23, 2022).
Kaijser, A., and Kronsell, A. (2014). Climate change through the lens of intersectionality. Environ. Polit. 23, 417–433. doi: 10.1080/09644016.2013.835203
Kim, N. J., Ng, K. C., and Chun, W. (2009). Using the condenser effluent from a nuclear power plant for Ocean Thermal Energy Conversion (OTEC). Int. Commun. Heat Mass Trans. 36, 1008–1013. doi: 10.1016/j.icheatmasstransfer.2009.08.001
Koronen, C., Åhman, M., and Nilsson, L. J. (2020). Data centres in future European energy systems—energy efficiency, integration and policy. Energy Effic. 13, 129–144. doi: 10.1007/s12053-019-09833-8
Lei, N., and Masanet, E. (2020). Statistical analysis for predicting location-specific data center PUE and its improvement potential. Energy 201, 117556. doi: 10.1016/j.energy.2020.117556
Levy, M. (2019). New Family of Data Center Metrics Using a Multidimensional Approach for a Holistic Understanding. Boca Raton, FL: Florida Atlantic University.
Li, X., Garraghan, P., Jiang, X., Wu, Z., and Xu, J. (2018). Holistic virtual machine scheduling in cloud datacenters towards minimizing total energy. IEEE Trans. Parallel Distrib. Syst. 29, 2688445. doi: 10.1109/TPDS.2017.2688445
Lucivero, F. (2019). Big data, big waste? A reflection on the environmental sustainability of big data initiatives. Sci. Eng. Ethics. 26, 1009–1030. doi: 10.1007/s11948-019-00171-7
Mahbod, M. H. B., Chng, C. B., Lee, P. S., and Chui, C. K. (2022). Energy saving evaluation of an energy efficient data center using a model-free reinforcement learning approach. Appl. Energy 322, 119392. doi: 10.1016/j.apenergy.2022.119392
Malone, C., and Belady, C. (2006). “Metrics to characterize data center and IT equipment energy use,” in Proceedings of the Digital Power Forum. Dallas, TX.
Manganelli, M., Soldati, A., Martirano, L., and Ramakrishna, S. (2021). Strategies for improving the sustainability of data centers via energy mix. Energy Conserv. Circ. Energy Sustain. 13, 114. doi: 10.3390/su13116114
Masanet, E., Shehabi, A., Lei, N., Smith, S., and Koomey, J. (2020). Recalibrating global data center energy-use estimates. Science 367, 984–986. doi: 10.1126/science.aba3758
McCammon, S. (2017). Google Moves in and Wants to Pump 1.5 Million Gallons of Water per Day. Washington, DC: National Public Radio.
Ministry of Energy Green Technology and Water Malaysia Kettha. (2017). Green Technology Master Plan Malaysia 2017-2030. Putrajaya: Ministry of Energy Green Technology and Water Malaysia, Kettha.
Miyamoto, M., Parid, M. M., Aini, Z. N., and Michinaka, T. (2014). Proximate and underlying causes of forest cover change in Peninsular Malaysia. Forest Policy Econ. 44, 7. doi: 10.1016/j.forpol.2014.05.007
Murray, A., Skene, K., and Haynes, K. (2017). The circular economy: an interdisciplinary exploration of the concept and application in a global context. J. Bus. Ethics 140, 369–380. doi: 10.1007/s10551-015-2693-2
Myrén, K. (2021). Här är Sveriges nya Klondike - men därför kan jobben stoppas. Stockholm: Tidningen Näringslivet.
Nightingale, A. J. (2016). Adaptive scholarship and situated knowledges? Hybrid methodologies and plural epistemologies in climate change adaptation research. Area. 48, 12195. doi: 10.1111/area.12195
Noël, J. A., Kahwaji, S., Desgrosseilliers, L., Groulx, D., and White, M. A. (2022). “Phase change materials,” in Storing Energy, 2nd Edn, ed T. M. Letcher (Elseivier), 503–535. doi: 10.1016/B978-0-12-824510-1.00005-2
Oltmanns, J., Sauerwein, D., Dammel, F., Stephan, P., and Kuhn, C. (2020). Potential for waste heat utilization of hot-water-cooled data centers: a case study. Energy Sci. Eng. 8, 1793–1810. doi: 10.1002/ese3.633
Onder, E., and Sarier, N. (2015). “Thermal regulation finishes for textiles,” in Woodhead Publishing Series in Textiles, Functional Finishes for Textiles, ed R. Paul (Woodhead Publishing), 17–98.
Phillips, A. L. (1963). A solar-energy method for reducing coffee-drying costs. J. Agric. Univ. Puerto Rico 47, 226–235. doi: 10.46429/jaupr.v47i4.12958
Reddy, V. D., Setz, B., Rao, G. S. V., Gangadharan, G., and Aiello, M. (2017). Metrics for sustainable data centers. IEEE Trans. Sustain. Comput. 2, 290–303. doi: 10.1109/TSUSC.2017.2701883
Ruch, P., Ammann, J., Paredes, S., Wiik, N., Lörtscher, E., Meijer, G. I., et al. (2017). “Sustainable data centers and energy conversion technologies,” in 12th IEA Heat Pump Conference. Rotterdam: IEA Energy Technology Network.
Sachs, J. D., Cordes, K. Y., Rising, J., Toledano, P., and Maennling, N. W. (2019). Ensuring Economic Viability and Sustainability of Coffee Production. New York, NY: Columbia Center on Sustainable Investment.
Skatteverket (2015). Betänkandet Energiskatt på el – En översyn av det nuvarande systemet (SOU 2015:87). Stockholm: Skatteverket. Available online at: https://www.skatteverket.se/omoss/varverksamhet/rapporterremissvarochskrivelser/remissvar/2015/remissvar2015/13157527715112.5.3810a01c150939e893f14c5c.html?q=datacenter (accessed November 19, 2021).
Skoglund, N., Werner, K., M, N. G., Pavia, H., Eva, A., and Broström, M. (2017). Combustion of seaweed - A fuel design strategy. Fuel Proc. Technol. 165, 17. doi: 10.1016/j.fuproc.2017.04.017
Soto, R., and Vergara, J. (2014). Thermal power plant efficiency enhancement with Ocean Thermal Energy Conversion. Appl. Therm. Eng. 62, 105–112. doi: 10.1016/j.applthermaleng.2013.09.025
Sovacool, B. K., Upham, P., and Monyei, C. G. (2022). The “whole systems” energy sustainability of digitalization: humanizing the community risks and benefits of Nordic datacenter development. Energy Res. Soc. Sci. 88, 102493. doi: 10.1016/j.erss.2022.102493
Suomen Standardisoimisliitto SFS RY (2021). Harnessing Waste Heat - A New Standard Improves Energy Efficiency of Data Centres. Helsinki: Suomen standardisoimisliitto SFS RY. Available online at: https://sfs.fi/en/harnessing-waste-heat-a-new-standard-improves-energy-efficiency-of-data-centres/ (accessed August 29, 2022).
Swinhoe, D. (2022). Singapore Enters Pilot Phase to Restart Data Center Development; Will Accept Some New Applications. London: Data Centre Dynamics. Available online at: https://www.datacenterdynamics.com/en/news/singapore-enters-pilot-phase-to-restart-data-center-development-will-accept-some-new-applications/ (accessed March 7, 2022).
Swyngedouw, E. (2009). The political economy and political ecology of the hydro-social cycle. J. Contemp. Water Res. Educ. 142, 56–60. doi: 10.1111/j.1936-704X.2009.00054.x
Terenius, P. (2020). “Using data centre waste heat to dry coffee whilst supplying small-scale farmers with ICT,” in International Conference for Sustainable Development, 21-22 September. New York, NY.
Terenius, P., Garraghan, P., and Harper, R. (2021a). Using data centre waste heat to dry coffee whilst supplying small-scale farmers with ICT: a novel idea and a case study based on a systems approach. J. Strat. Innov. Sustain. 16, 4297. doi: 10.33423/jsis.v16i2.4297
Terenius, P., Golmen, L. G., Garraghan, P., and Harper, R. (2021b). “Heat energy from datacenters: an opportunity for marine energy,” in International Conference on Ocean Energy 2021. Washington, DC.
The Green Grid (2007). The Green Grid Data Center Power Efficiency Metrics: PUE and DCiE. Green Grid Report. Beaverton, OR: The Green Grid.
The Green Grid (2010a). Carbon Usage Effectiveness (CUE): A Green Grid Data Center Sustainability Metric. Beaverton, OR: The Green Grid.
The Green Grid (2010b). ERE: A Metric for Measuring the Benefit of Reuse Energy from a Data Center. The Green Grid.
Vasques, T. L., Moura, P., and De Almeida, A. (2019). A review on energy efficiency and demand response with focus on small and medium data centers. Energy Effic. 12, 1399–1428. doi: 10.1007/s12053-018-9753-2
Vega, L. A. (2013). “Ocean thermal energy conversion,” in Renewable Energy Systems, eds M. Kaltschmitt, N. J. Themelis, L. Y. Bronicki, L. Söder, and L. A. Vega (New York, NY: Springer). doi: 10.1007/978-1-4614-5820-3_695
Velkova, J. (2016). Data that warms: waste heat, infrastructural convergence and the computation traffic commodity. Big Data Soc. 3, 2053951716684144. doi: 10.1177/2053951716684144
Vonderau, A. (2019). Scaling the cloud: making state and infrastructure in Sweden. Ethnos 84, 698–718. doi: 10.1080/00141844.2018.1471513
Vonderau, A. (2021). “Att få molnet att växa: En studie av den digitala framtidens megaprojekt i Sverige,” in Megaprojekt - Kritiska perspektiv på storskalig infrastruktur. Svenska sällskapet för antropologi och geografi, eds G. Körling and S. Baez Ullberg (Stockholm: Ethnos).
Wahlroos, M., Pärssinen, M., Manner, J., and Syri, S. (2017). Utilizing data center waste heat in district heating–Impacts on energy efficiency and prospects for low-temperature district heating networks. Energy 140, 1228–1238. doi: 10.1016/j.energy.2017.08.078
Wahlroos, M., Pärssinen, M., Rinne, S., Syri, S., and Manner, J. (2018). Future views on waste heat utilization: case of data centers in Northern Europe. Renew. Sustain. Energy Rev. 82, 1749–1764. doi: 10.1016/j.rser.2017.10.058
Wan, J., Zhou, J., and Gui, X. (2021). Sustainability Analysis of Green Data Centers with CCHP and Waste Heat Reuse Systems. IEEE Trans. Sustain. Comput. 6, 473. doi: 10.1109/TSUSC.2020.2979473
Watson, M. D. (2019). Systems engineering principles and hypotheses. Insight 22, 12233. doi: 10.1002/inst.12233
WWF Malaysia and Boston Consulting Group (2021). Securing our Future: Net Zero Pathways for Malaysia. Klang: WWF Malaysia and Boston Consulting Group.
Keywords: sustainability, renewable energy, society, waste heat, commodity dehydration, metrics, circular economy, data center
Citation: Terenius P, Garraghan P and Harper R (2023) A material social view on data center waste heat: Novel uses and metrics. Front. Sustain. 3:1008583. doi: 10.3389/frsus.2022.1008583
Received: 01 August 2022; Accepted: 19 December 2022;
Published: 25 January 2023.
Edited by:
Deborah Andrews, London South Bank University, United KingdomReviewed by:
Jon Summers, Research Institutes of Sweden (RISE), SwedenDuygu Erten, Istanbul Medipol University, Turkey
Copyright © 2023 Terenius, Garraghan and Harper. This is an open-access article distributed under the terms of the Creative Commons Attribution License (CC BY). The use, distribution or reproduction in other forums is permitted, provided the original author(s) and the copyright owner(s) are credited and that the original publication in this journal is cited, in accordance with accepted academic practice. No use, distribution or reproduction is permitted which does not comply with these terms.
*Correspondence: Petter Terenius, cC50ZXJlbml1cyYjeDAwMDQwO2xhbmNhc3Rlci5hYy51aw==