- 1Department of Land, Air & Water Resources, University of California, Davis, Davis, CA, United States
- 2Wild Energy Initiative, John Muir Institute of the Environment, University of California, Davis, Davis, CA, United States
- 3School of Advanced International Studies, Johns Hopkins University, Washington, DC, United States
- 4Electric Power Research Institute, Palo Alto, CA, United States
Energy development improves quality of life for humans, but also incurs environmental consequences. A global energy transition from fossil fuels to renewable energy may mitigate climate change but may also undermine the capacity to achieve some or all 17 Sustainable Development Goals (SDGs). In this study, we use an innovation systems approach to construct a comprehensive roadmap for solar and wind energy to anticipate and improve impacts of a transition to a low carbon future in a manner ensuring climate goals and SDGs are mutually reinforcing. Our multidisciplinary approach began with an assessment of public investments in renewable energy followed by a 2-day research prioritization workshop. Fifty-eight expert workshop participants identified six research themes that proactively address the environmental sustainability of renewable energy. Next, we identified linkages between the six research themes and all 17 SDGs. Finally, we conducted a scientiometric analysis to analyze the research maturity of these themes. The results of these efforts elucidated the limits of existing knowledge of renewable energy-SDG interactions, informing the development of a research, development, demonstration, and deployment (RD3) roadmap to a renewable energy future aligned with both climate goals and SDGs. The RD3 roadmap has been designed to systematically develop solutions for diverse actors and organizations. Overall, our findings confer a broad vision for a sustainable transition to renewables to minimize unintended environmental consequences while supporting interoperability among actors particularly poised to influence its magnitude and direction.
Introduction
A just transition to mitigate climate change necessitates a rapid and global diffusion of renewable energy but in a manner that avoids adverse impacts on the environment such that the United Nations (UN) Sustainable Development Goals (SDGs) can be met concomitantly (Healy and Barry, 2017; Nerini et al., 2019; Yeeles, 2019). The potential contribution of renewable energy to decarbonize the power sector is immense: “affordable and clean energy” is the seventh of the 17 SDGs. Scenarios that consider SDGs while holding global average temperatures below 1.5°C above preindustrial levels as set by the Paris Agreement rely heavily on a transition to renewable energy—at least a doubling of annual supply investments for renewable-based power from 2019 to 2050, reaching $607 billion per year (2018 equivalent dollars; IEA, 2019a). An exponential growth of the renewable sector in the near term is inextricably linked to the environment and dependent on the coordination of actors across the system leading appropriate research, development, demonstration, and deployment (RD3). The advancement of renewable technologies offers an unparalleled opportunity to leverage expert knowledge to mitigate environmental risks with foresight, in advance of large-scale deployment.
While many synergies exist where climate mitigation reinforces movement toward SDGs, action toward one goal may not always align naturally with the other (Nerini et al., 2018, 2019); the environmental aspects of renewable energy is a prime example of the latter. For example, SDG 12 (Responsible Consumption and Production) primarily centers on waste minimization, sustainable natural resource use, and recycling. Potential trade-offs between SDG 12 and high penetration of solar and wind have already been noted; for example, solar e-waste is named by the UN as among the highest potential contributors to growth of the already 50 million metric tons of the annual global e-waste. To date, most solar panels are installed and operating, thus they have not reached their end-of-life, underscoring an imminent necessity (Parajuly et al., 2019). The challenge requires urgent solutions: global solar PV waste alone has the potential to reach 78 million metric tons cumulatively by 2050 (Chowdhury et al., 2020). Bolstering synergies and minimizing incompatibilities of solar and wind power—e.g., waste without adequate recycling, adverse wildlife interactions, and public nuisance—requires a systematic approach that recognizes these technologies' enormous potential for climate and economics benefits while acknowledging environmental risks.
Net impacts of energy development on the environment have been challenging to quantify owing to the diffuse, intractable, and diverse nature of negative impacts–e.g., soil contamination, biodiversity loss, mountaintop removal, and social injustices. Recent studies highlight the exigent need to anticipate linkages between renewable energy and the environment, emphasizing the avoidance of Achilles' heels and reinforcement of reciprocities in this transition (Moore-O'Leary et al., 2017; Nerini et al., 2018; Xu et al., 2018; Hernandez et al., 2019; Grodsky and Hernandez, 2020; Hao et al., 2020). Within this context, the identification of environmental interactions associated with renewables needs more attention, particularly with the nascence of research on the direction, magnitude, and causes of potential interactions (EPRI, 2019a). For example, environmental impacts of solar energy siting decisions span from reductions in evaporative loss of water in irrigation reservoirs (Redón-Santafé et al., 2014) to enhanced ecosystem services valued by indigenous tribal groups (Grodsky and Hernandez, 2020). Further, a lack of understanding of environmental outcomes directly impacts market entry through diffusion (Kemp et al., 1998; Bergek et al., 2008; Negro et al., 2012).
Energy Technology Innovation Systems (ETIS) have presented a systemic perspective for policy-makers, investors, and other decision-makers to more actively advance the creation and use of new energy technologies (Gallagher et al., 2012; Jordaan et al., 2017). While the perspective notably includes national security and economics (Anadon, 2014), the environmental focus of the framework has been primarily on reducing GHG emissions (Anadon et al., 2017). While the use of ETIS in meeting both climate goals and SDGs has received less attention to date in scholarship (Sagar and Holdren, 2002; Negro et al., 2012), public investments in RD3 have been noted as one opportunity to do so (Anadon et al., 2017). Experts note the need for the development of coherent policy apparatus to ensure that both climate goals and SDGs can be achieved (Yeeles, 2019). ETISs can enable such an apparatus by facilitating the necessary finance and institutions, provided that concerted efforts to align SDGs with climate goals are a key consideration in the widespread diffusion of renewable technologies. Roadmaps can play a crucial role in successful energy innovation, by influencing expectations formation (i.e., legitimation) and sharing (i.e., guidance); such benefits increase with stakeholder involvement (Bento and Fontes, 2018).
Knowledge systems—a critical component of ETISs—are complex networks of actors, organizations, and objects that perform knowledge-related functions, most notably linking knowledge with action, which may include research, invention, development, demonstration, deployment, and adoption (Cash et al., 2003; McCullough and Matson, 2016). Coordination across the knowledge systems can be achieved, in part, through roadmaps that identify the requirements of systems intended for growth (McDowall, 2012; Rip, 2012). Several studies find the role of coordination and identification of priorities across knowledge systems to be a determining factor in transition success (Tawney and Weischer, 2011; Cornell et al., 2013; Clar and Sautter, 2014). Despite their potential to contribute solutions, roadmaps have yet to be developed for aligning climate goals and SDGs directing a sustainable transition to renewables (Moore-O'Leary et al., 2017; Bento and Fontes, 2018).
In this study, we sought to construct a comprehensive roadmap to anticipate and improve adverse impacts of a transition to a low carbon future with high penetration of renewables. The roadmap provides a critical, solutions-oriented approach to ensure that both climate goals and SDGs are clearly delineated, recognized, and proactively managed for the renewable power sector. Specifically, our goal was to use an innovation systems approach to construct a comprehensive roadmap for solar and wind energy to anticipate and improve impacts of a transition to a low carbon future in a manner ensuring climate goals and SDGs are mutually reinforcing. Our approach was multidisciplinary, beginning with an investment assessment and then employing a 2-day, in-person research prioritization workshop of 58 expert stakeholders who identified six research themes that seek to improve the environmental sustainability of renewable energy development. We then evaluated instances of linkages between the six research themes and all 17 SDGs that have been confirmed by literature. Next, we conducted a scientiometric analysis to analyze the research maturity of these themes, providing insight into how decision makers may choose to allocate resources, plan RD&D (Research, Development, and Demonstration), and improve the communication of both decisions and research output (Leong et al., 2011; Liggett, 2014). The results of these efforts were integrated to elucidate the limits of existing knowledge of renewable energy-SDG interactions (including potential Achilles' heels and reciprocities) and inform a RD3 roadmap to a renewable energy future aligned with both climate goals and SDGs. Overall, our findings provide the necessary programmatic elements for a roadmap that enables the sustainable, widespread scaling-up of renewable energy, aligning both climate goals and SDGs.
Materials and Methods
We employed a multidisciplinary approach with a structured workflow of research activities to inform the RD3 roadmap (Figure 1). First, we conducted an investment assessment to compare investments across energy types, notably for solar energy, wind energy, and fossil fuels. Next, we conducted a 2-day, in-person research prioritization workshop of 58 expert stakeholders who identified six research themes that address incongruence between environmental sustainability and renewable energy development. We then demonstrated the linkages between the six research themes and all 17 SDGs, with a qualitative evaluation of literature. Next, we conducted a scientiometric analysis and systematic review to analyze the maturity of these themes, including identifying understudied themes. The goal of the RD3 roadmap is to provide a structured approach to address knowledge gaps and align goals. The architecture of the research roadmap was informed by the culmination of results from the RD&D investment analysis, stakeholder workshop, literature review, and scientiometric analysis.
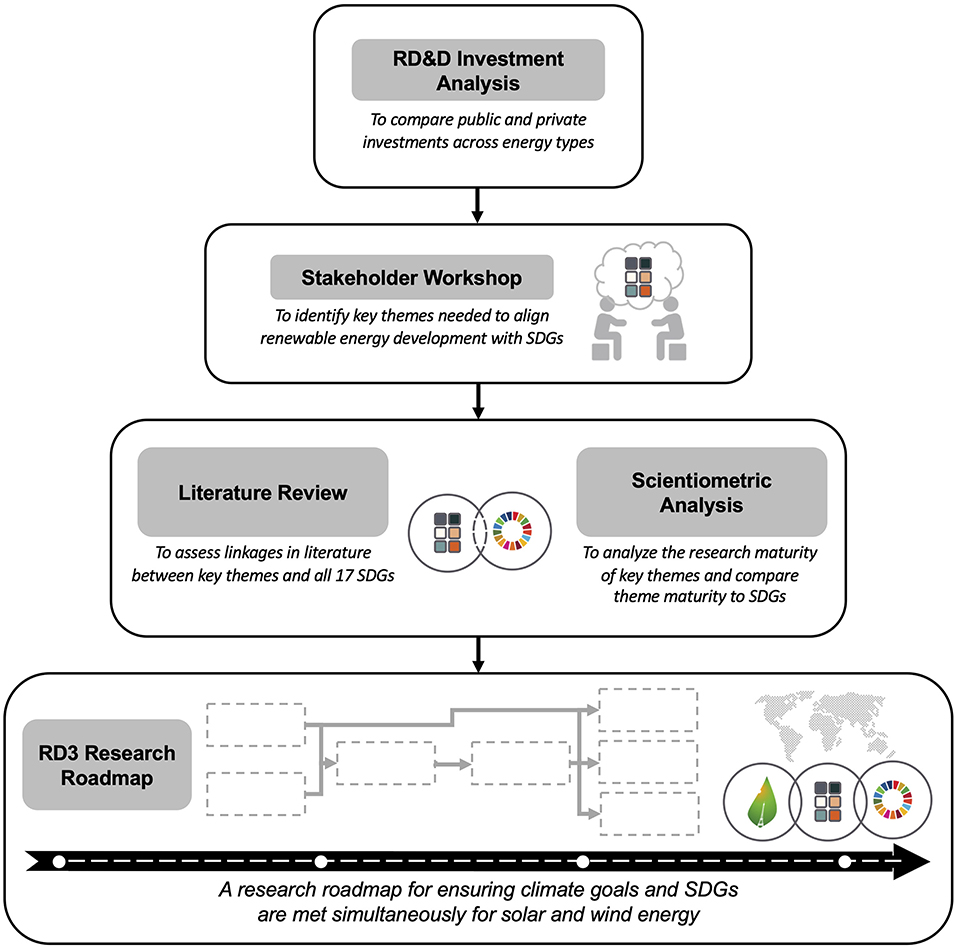
Figure 1. Research work flow showing multi-disciplinary, hierarchical research activities. We led an RD&D investment analysis, stakeholder workshop leading to the development of key research themes (represented by the six squares), literature review, and scientiometric analysis, which collectively informed the development of a research roadmap for ensuring climate goals (represented by the Paris Agreement “green leaf” icon) and the United Nations Sustainable Development Goals (SDGs, represented by the color wheel icon) are mutually reinforcing for the renewable power sector. Graphics use icons reproduced from the Noun Project (https://thenounproject.com).
RD&D Investment Analysis: Technology Innovation
We compiled and evaluated recent data on public and private investments in renewable technologies, solar and wind technologies, as well as the full suite of energy technologies for which data are reported (where consistent data were available) to compare investments in RD&D among solar energy, wind energy, and fossil fuels, which facilitate innovation and knowledge creation. Data collection included government, non-government and industry investments to capture the influence of a range of actors across the innovation process. We examined statistics on national research, development, and demonstration (RD&D) budgets for all countries that submit data that are reported by the International Energy Agency (IEA) from 1974 to 2018 (IEA, 2019b). The IEA data are publicly available; research institutes, scholars, industry, and other entities regularly use the information when facing decisions about innovation, such as those related to investments and policies. Private investment data were also reviewed and compiled, confirming a focus on technology investments and excluding or aggregating environmental expenditures (BNEF, 2019).
Stakeholder Workshop and Literature Review: Development of Research Priorities
Workshops are a tool to engage different stakeholders (academics, scientists, civil society, and government) and develop real world solutions to environmental challenges (Patel et al., 2007; Lang et al., 2012). Stakeholders across the world have initiated various energy innovation workshops with the aim of driving intersectoral and interdisciplinary collaboration (with emphasis on academia-industry dialogue) to identify innovation gaps and shape the directions of future research (CEC, 2013). While limitations of workshops do exist (e.g., difficult to plan and coordinate, time commitment may preclude certain stakeholders, potential for emergence of divergent and/or unreconciled views, outcomes represent a snapshot in time), the strength of a workshop lies in its capacity to identify overarching themes and corroborate the comprehensiveness and accuracy of research priorities generated with other methods (O'Haire et al., 2011). Overall, workshops are critical research tools that can leverage knowledge systems to gather and create knowledge, increase awareness about key issues, better communicate scientific results from producers to users, resolve conflicts, pursue new ideas and insights in a non-hierarchical setting, and network with people who hold complementary knowledge and expertise (Dufva and Ahlqvst, 2015; Scherhaufer et al., 2018).
A 2-day expert workshop was held by the Electric Power Research Institute (EPRI) in Chicago, Illinois (United States [US]) from 29 to 30 April 2019. The goal of the workshop was to develop a comprehensive research agenda, leading to the development of six research themes representing areas where the deployment of solar and wind projects may conflict with the environmental sustainability of the sector (EPRI, 2019a,b). The research agenda was created for the broad stakeholder community to proactively manage and solve environmental issues that may arise for these technologies.
Themes were initially curated by a review of literature (see EPRI, 2019b for the review) and input from seven subject matter experts and EPRI technical staff from various sectors who also acted as session leaders for the workshop (see Supplementary Table 1 for roles; EPRI, 2019a). Beginning on November 9, 2018, a series of five planning calls were hosted by EPRI leaders in coordination with the workshop facilitators/session leaders (n = 15 total) leading up to the workshop on April 29–30, 2019. In total, external session leaders included representatives of industry (four experts), government (three experts), academia (one expert), and non-profit organizations (one expert). Initially, a list of topics to be covered during the workshop was developed by EPRI staff based on the literature review and shared with the expert panel during the first planning call. Over the course of various follow-on calls, eight themes arose that were considered to be the most critical for the workshop. Eventually, some of the themes were consolidated to allow for sufficient time for discussions during the two days of the workshop, and six themes were ultimately selected by the expert panel by consensus to represent the most pressing concerns associated with wind and solar energy project development, operation, and end-of-life.
The workshop was designed to facilitate knowledge transfer among a diverse group of participants (n = 58). All of the guest speaker presentations and three breakout sessions (wind and solar siting, wind wildlife interactions, solar wildlife interactions) were held on Day 1 followed by three breakout sessions (wind end-of-life, solar end-of-life, public acceptance) during the morning of Day 2. Workshop objectives included the development of (1) background information and research needs, and (2) the top five research priorities (also called “research gaps” and “project ideas” during the workshop) for each theme. Guest speaker presentations (15–20 min each; 1–3 presentations per theme) served to meet the first objective by providing background information in length, including an overview of the current state of the science, current research activities, and research gaps. These presentations served to stimulate activities within the breakout sessions. Example presentation titles included “Wind and Solar Siting Tools,” “Noise and Shadow Flicker,” and “Overview of the PV Waste Problem.”
Breakout sessions began with brainstorming activities guided by the session facilitators who subsequently led the group to develop and categorize ideas into ten research priorities for each of the six themes. Final priorities were selected through a voting process: each participant selected five out of ten research priorities under each theme (see Supplementary Table 2). As written and verbal contributions from attendees were guided by session facilitators to be topics representing research priorities, critical research gaps and/or ideas, peripheral and tangential topics often received relatively less votes. Votes were then tallied, and results were used to identify five priorities from each session with a final list of thirty research priorities in need of further development. While the results represent the priorities of the workshop attendees during the time in which the activities were held, they provide an important snapshot of research needs from the perspective of a knowledge system composed of diverse experts.
We then confirmed existing linkages in the literature between themes and SDGs (see Supplementary Tables 3, 4). We caution that a lack of a published relationship between themes and SDGs does not necessarily indicate the absence of a relationship but instead may indicate the lack of evidence of a relationship. We combined outcomes from the workshop and information from the reviews to develop cohesive definitions for each of the six prominent research themes–siting, solar-wildlife interactions, wind-wildlife interactions, public acceptance, solar end-of-life, and wind end-of-life (EPRI, 2019a).
Scientiometric Analysis: Maturity of Research Themes
Research production can be measured quantitatively and effectively using bibliometric data as a relative indicator of scientific activity as well as elucidating the temporal evolution of knowledge domains (Gatto and Drago, 2020; Machado et al., 2020; Xie et al., 2020). A bibliometric analysis performed solely on scientific articles, known as a scientiometric analysis, can serve to measure research progress, including its scope and breadth, and provide planning guidance (Leong et al., 2011). To assess and compare the state and progress of each of the six research themes, we analyzed the number of (1) peer-reviewed journal articles, (2) book chapters, (3) meeting abstracts, and (4) proceedings in Web of Science. Web of Science is a subscription-based (i.e., non-public) collection of databases and indexing service with an indexing mechanism distinct from others (e.g., Google Scholar) and including only English journals. We selected the Web of Science Core Collection database of the Institute for Science Information, which includes ~61 million records across journals, conference proceedings, and books in the sciences, social sciences, arts, and humanities.
For each theme, we developed a controlled, structured set of two to three paired terms, non-randomly selected to encompass and compare publications within and across themes, respectively. Paired terms were developed through an iterative process that ranked and weighted terms hierarchically. Paired terms included one energy and one environment term (e.g., “solar energy” “public acceptance”). In February of 2020, we performed a “Basic Search” for a paired set of terms across the Web of Science Core Collection across all years (1900–2019). We searched for records using the paired term with an “AND” operator. We used the Topic Search Field that searches for the term(s) in any order within the record's title, abstract, author keywords, and Keywords Plus®. After completing the two to three paired term searches for a single theme, we used the “OR” operator (to avoid counting a single publication twice) to determine the total records for the theme across all years (1900–2019). To (1) compare research productivity between the (A) six themes and documents emphasizing (B) UN SDGs, and (2) to determine the number of documents found across both corpora (A and B), we performed a search for the term “sustainable development goal” and six additional unique searches that explored the number of documents shared across this corpus and each of the six themes. We did not weight any publication by impact (e.g., number of citations). We used the count of publications as empirical evidence of (1) relationships between individual themes and SDGs, and (2) research productivity of individual themes, all themes, and SDGs. We did not apply weights to any publications based on attributes (e.g., impact factor of journal, citations), but recognize that all publications may not be equivalent.
Results
Trends in RD&D Investments: Technology Innovation
Improvements in innovation systems necessitate an understanding of the investments being made on the technologies of interest. Our review of prominent investment reports reveals important trends. First, public investments in fossil fuel RD&D continue to far outpace those in renewable technologies in countries reported by the IEA (IEA, 2019b). However, growth in renewables since 1974 is notable. In 2018, such governments reported investing 14.9, 4.4, and 3.4% of the $17.5 billion of RD&D, respectively, for renewable, solar, and wind technologies. In 1974, their fraction was below 1% of the $8.1 billion reported for these countries for Energy RD&D. Second, wind and solar combined have captured just over 50% of RD&D in renewable energy. A close inspection of the IEA reporting brings to light a potential gap in environmental finance for wind and solar in particular; for example, the IEA explicitly notes impacts to ecosystems for large scale hydropower (Figure 2).
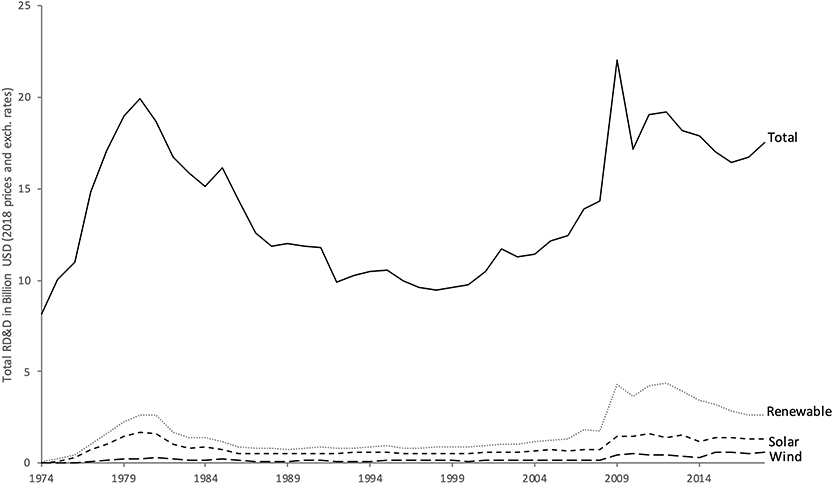
Figure 2. Government research, development and demonstration (RD&D) investments in energy technologies for countries reported by the International Energy Agency. While ecosystems are explicitly noted within the investments for large hydropower, interactions between wind, solar, and the environment remain unmentioned.
We found that, while government investments represented just under 2% of total renewable finance, global investments (public and private) have experienced similar trends with subtle but more pronounced growth (Figure 2, BNEF, 2019). These findings are mirrored in capacity growth: renewable power now contributes a third of global capacity with solar and wind accounting for 84% of the growth in 2018 (IRENA, 2019). Like government RD&D, these finance reports focus on technology: environmental aspects are neither explicitly addressed nor disaggregated. With the focus of innovation systems leaning toward climate mitigation where solar and wind present an attractive part of the solution, interactions with SDGs have largely been overlooked in comparison.
Research Priorities for the Roadmap
A stakeholder workshop led by EPRI was held to identify research priorities under each theme in support of the development of solutions-oriented roadmaps. The intended outcome of the workshop was direction for the broader research community to address environmental issues proactively by ensuring that growth in wind and solar energy does not compromise environmental sustainability (EPRI, 2019a). While single issues or technologies have previously been examined, to the best of the authors' knowledge, this initiative was the first of its kind to develop a comprehensive research roadmap specific to both solar and wind energy. Each theme is established below, a clear definition of each theme is provided in Figure 3, and its capacity to facilitate or inhibit SDGs confirmed with literature (see Supplementary Materials for a full table describing the specific linkages and the literature identified to support the linkages). The content below serves to supplement the theme definitions with additional background on each theme's role and linkages with SDGs.
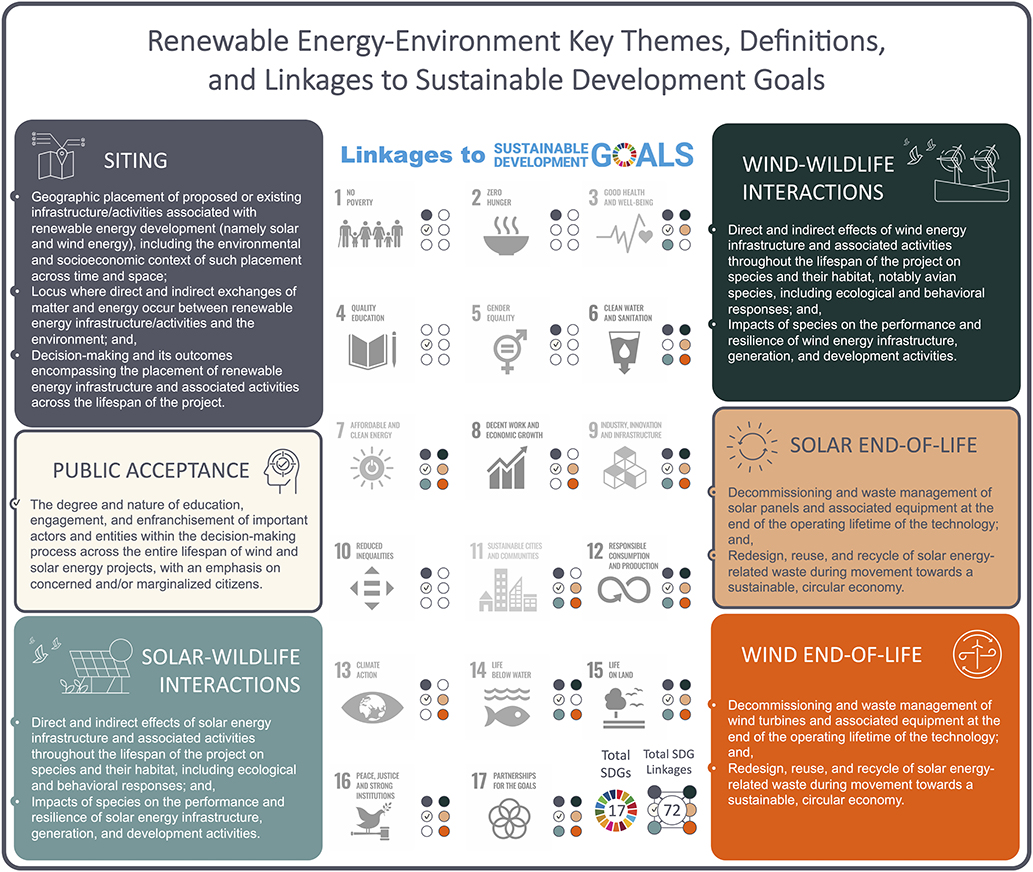
Figure 3. Formal definitions of environmental research themes for wind and solar power and their linkages to United Nations Sustainable Development Goals (SDGs). Filled circles represent one of six themes that have potential to reinforce and/or inhibit progress toward SDGs. A total of 17 SDGs are represented across all six themes owing to 72 total unique linkages confirmed with supporting literature (see Supplementary Material for methods and references). Graphics use icons reproduced from the Noun Project (https://thenounproject.com).
Siting
Site selection for solar and wind energy infrastructure is arguably the most critical determining factor as it directs innumerable effects on key design considerations; the type, severity, and frequency of environmental impacts; the degree of community acceptance that impacts SDG 16 (Peace, Justice and Strong Institutions); and other factors, including the need for decommissioning activities. Effective siting tools, technologies, and collaboration across the full knowledge system of siting decision-makers, including concerned citizens, can safeguard the development of wind and solar energy projects against unforeseen negative ecological and environmental justice impacts in an era of looming land scarcity.
Public Acceptance
Public concerns about wind and solar development are being raised globally that may conflict with SDGs. Related concerns to wind generation are turbine-associated noise, which has been linked to annoyance and sleep disturbance (as well as perceived adverse health effects impacting SDG 3, Good Health and Well-Being), impacts on landscape aesthetics and cultural/religious viewsheds (SDG 16), and shadow flicker. Solar generation (glass PV modules and CSP mirrors) is also associated with glint and glare and may cause visual impacts. There is an increasing need to understand and engage with all modalities of public acceptance, including real and perceived conflicts with SDGs in co-located and nearby communities, as well as the development of optimal tools to realize energy democracy, beyond the communication of risks to residents and other stakeholders.
Solar-Wildlife Interactions
Solar energy infrastructure spans terrestrial and aquatic settings (e.g., floatovoltaics; Armstrong et al., 2020; Cagle et al., 2020) that encompass various levels of human development. The integration of this infrastructure in the built and other human-dominated environments can reduce or eliminate direct and indirect conflicts with biodiversity in alignment with SDGs 14 (Life Below Water) and 15 (Life on Land). In many places, solar energy is pre-existing or is still being emphasized in natural and/or semi-natural environments that wildlife species use and occupy. Infrastructure may serve as a barrier to the movement of species (e.g., desert tortoises may be excluded from fenced areas); however, relatively adaptive species may use and occupy infrastructure in novel ways when introduced into their environment. For example, in residential areas on rooftops, pigeons and other avian species may roost under PV panels.
Wind-Wildlife Interactions
Unlike solar energy, the integration of wind energy infrastructure in human-dominated environments may not necessarily reduce adverse interactions with biodiversity. The integration of wind energy infrastructure into vertical strata can lead to injury or mortality for avian species from direct collisions as well as indirect consequences (e.g., barotrauma, habitat loss) for species, notably bats and birds. Vulnerability due to impacts from these interactions may also lead to habitat loss, thus increasing research is needed to develop solutions such as operational curtailment, deterrent, and other innovations for wind energy development to avoid incompatibilities with SDGs 14 and 15.
Solar End-of-Life Management
With a typical operating life of 25–30 years, viable waste management solutions and logistics must be developed proactively to handle the substantial waste volume: it is expected to reach 7.5–10 million tons cumulatively by 2050 in the US alone (Weckend et al., 2016), with global estimates reaching an order of magnitude higher. And the waste may be hazardous—PV and CSP contain heavy metals such as lead and cadmium—possibly leading to higher disposal costs and risks to the environment and human health. As demand increases, solar end-of-life management outcomes that avoid dismantling and recycling will augment environmental risks associated with raw materials and rare earth mineral extraction and solar energy manufacturing supply chains broadly (Xu et al., 2018). We note the importance of the resulting incompatibilities with SDG 12, but also that disassembly in poor nations with improper handling methods raise important questions about the health and well-being of potential workers (e.g., SDG 3).
Wind End-of-Life
Like solar technologies, wind turbine blades installed over the past decade are set to reach their end-of-life in the late 2020s to mid-2030s. Wind technologies face further challenges since the recovered materials lack high value products. There is a dearth of economically attractive recycling and regulatory options, meaning landfilling has become the most viable alternative to date (Liu and Barlow, 2017; EPRI, 2019a). Exponential growth in waste is expected globally with estimates ranging up to 43 billion tons of cumulative blade waste alone by 2050 (Liu and Barlow, 2017). The wind sector thus faces incompatibilities most notably with SDG 12 from decommissioning onwards.
Maturity of Research Themes
To determine their relative maturity, we conducted a scientometric analysis to compare research productivity across the six themes. We found 1,391 articles, book chapters, meeting abstracts, and proceedings that met our criteria of search terms across all six themes from 1978 to 2019 (Figure 4, Supplementary Figure 1). The year with the greatest research-related productivity across all themes was in 2018 (201) followed by 2019 (183). Siting had the largest cumulative number of publications (560), followed by public acceptance (340), wind-wildlife interactions (289), solar end-of-life (130), solar wildlife interactions (108), and wind end-of-life (40). Our scientometric analysis of the term “sustainable development goal” revealed 898 publications beginning in 1999. Notably, the resulting corpus including publications emphasizing SDGs did not overlap with any publications from the corpus spanning all energy-environment research themes. Over 50% of the SDGs corpus (56.8%) was not published until 2018, representing a recent escalation of productivity compared with 50% productivity milestones across the six themes (2015, 2016, 2016, 2014, 2017, and 2016, respectively; Figure 4). Across all themes, the US was associated with the plurality of theme-related publications (n = 479, 31.6%, Figure 4). Across all themes, the United Kingdom, Germany, China, Canada, Italy, and Greece were also well represented with 168, 113, 100, 66, 58, and 56 publications, respectively. By individual theme, the US had the greatest percentage of publications for siting, wind-wildlife interactions, solar-wildlife interactions, and wind end-of-life interactions, while publications for public acceptance and solar end-of-life were greatest for Germany and China, respectively (Supplementary Figure 2). Recognizing that these themes are at different stages of maturity, an innovation systems approach can support the intended proactive management of the impacts (Figure 5).
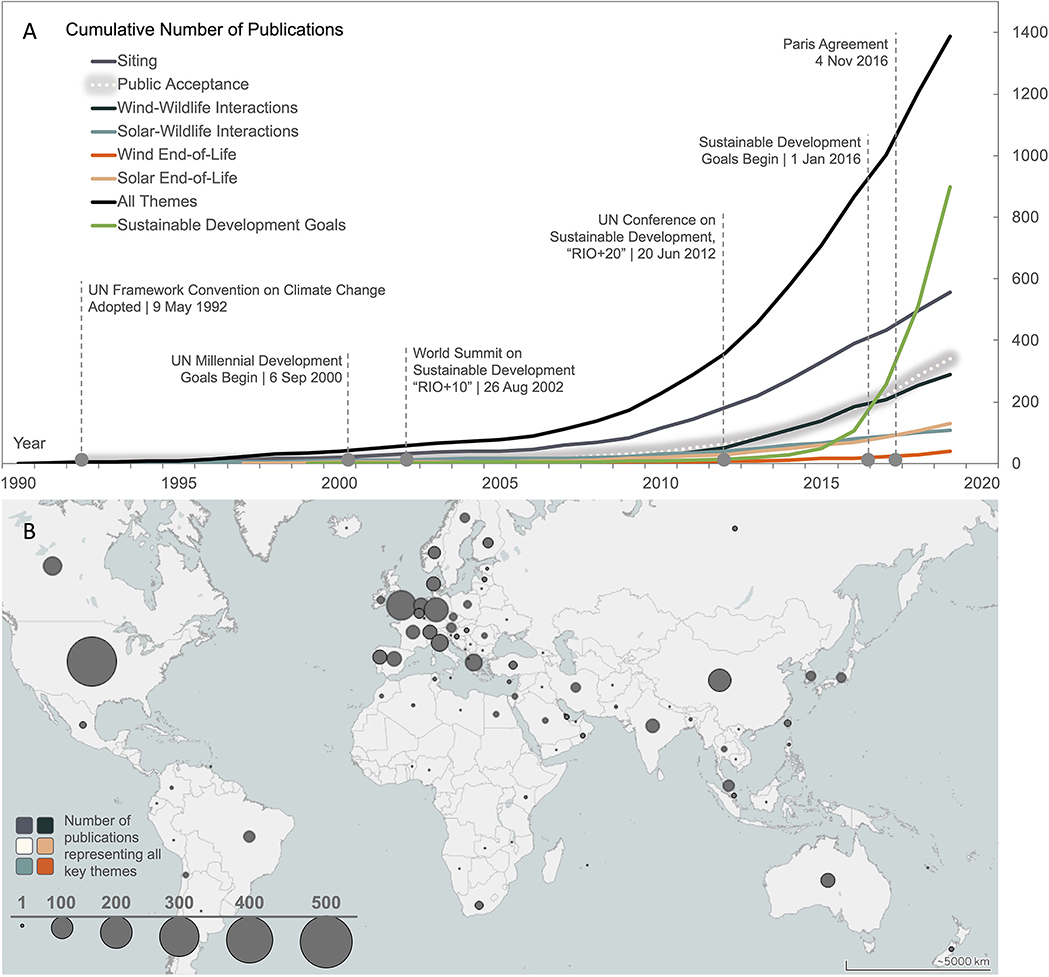
Figure 4. Comparison of research productivity on energy-environment interactions across time and space. (A) The cumulative number of publications across siting, public acceptance, wind-wildlife interactions, solar-wildlife interactions, wind end-of-life, and solar end-of-life research themes, all key themes combined, and publications emphasizing United Nations Sustainable Development Goals from 1990 to 2019. (B) Global comparison of research productivity across all six themes by country from 1990 to 2019. Four siting publications published prior to 1990 are not shown. Data from: Web of Science (2020). Graphics use icons reproduced from the Noun Project (https://thenounproject.com).
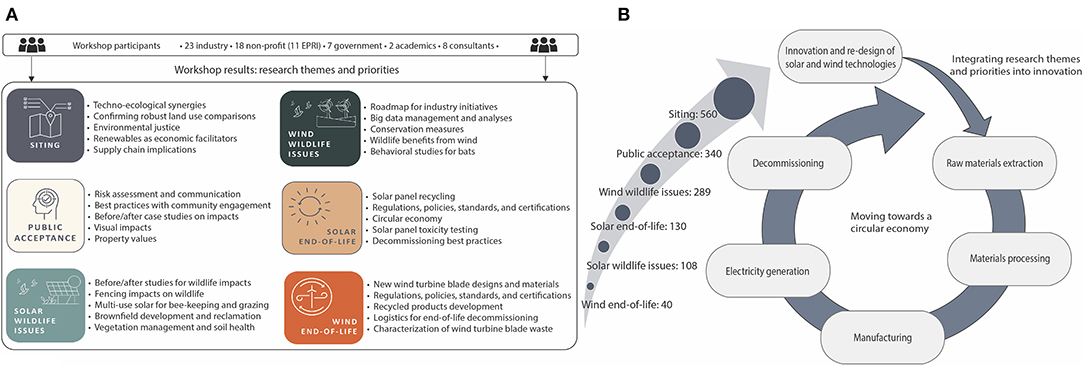
Figure 5. (A,B) Integration of research themes into innovation across the life cycle of wind and solar technologies. (A) Summarizes the results from the 58-expert stakeholder workshop developed using brainstorming and prioritization exercises (see Supplementary Material for additional details about the research priorities). Fifty-eight experts attended, spanning representatives from government, industry and non-profits (seven, 23, and 15 experts, respectively) and two academics; eleven EPRI staff were also in attendance and categorized as non-profit attendees. The numbers reported in the figure represent the cumulative corpus of publications on each individual theme from Web of Science (2020). (B) Presents a circular economy approach to align United Nations Sustainable Development Goals (SDGs) with climate goals in a transition to the widespread diffusion of solar and wind technologies. Graphics use icons reproduced from the Noun Project (https://thenounproject.com).
A Roadmap for an Environmentally Sustainable, Renewable Future
While the feasibility of widespread diffusion of solar and wind technologies has been questioned due to their economic viability and intermittency (Notton et al., 2018), the Intergovernmental Panel on Climate Change (IPCC) suggests that few technological limits exist toward reaching 77% of supply for renewable energy by 2050. The IEA has recently developed a Sustainable Development Scenario (SDS), unique in considering both SDGs and climate goals, yet broadly focused on the energy sector rather than specifically on solar and wind technologies. In this scenario, solar and wind provide 40% of global electricity supply, with their intermittency balanced by flexible gas-fired power (with carbon capture) and dispatchable renewable options (e.g., hydro and bioenergy) (IEA, 2019c).
Motivated efforts will be required to achieve large-scale deployment of solar and wind technologies by 2050 proposed by the SDS without challenges associated with meeting the aforementioned SDGs. The renewable sector, international agencies, as well as national and subnational policy-makers should be active participants in support of such efforts. Using the workshop findings as a starting point, solutions can be actively developed in research priorities under each theme – including performance monitoring and evaluation throughout implementation – within time horizons that honor the respective goals (Figure 6). Solutions-focused research will address environmental challenges across the life of the solar and wind projects, including technological advancements during the design phase, to operational impacts, and end-of-life management.
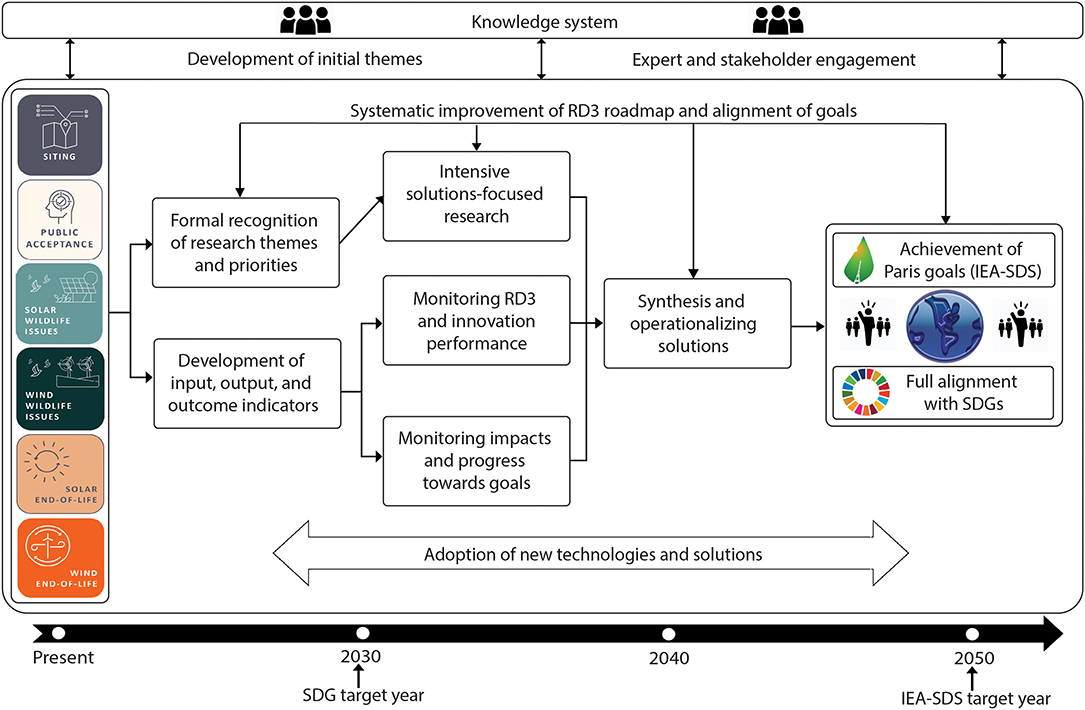
Figure 6. A RD3 roadmap for ensuring climate goals and UN Sustainable Development Goals (SDGs) are met simultaneously for solar and wind energy. The roadmap encompasses not only research, development, and demonstration but also deployment (i.e., RD3) to ensure solutions are being systematically adopted throughout the duration of roadmap implementation. The International Energy Agency's Sustainable Development Scenario (IEA-SDS) is used as an illustrative example for meeting climate goals in alignment with SDGs. Graphics use icons reproduced from the Noun Project (https://thenounproject.com).
We acknowledge that the priorities identified here represent the 2019 state of the field in the US: only a small proprotion of the future global knowledge system for the renewable sector was involved in their development. The present themes and research roadmap should be formalized with active engagement of key global stakeholders and with broad applicability across spatial scales, from local governments to international agencies who engage nations on important questions about sustainable development and the renewable energy sector. A systematic, dynamic approach should thus be employed with active engagement of the growing global knowledge system throughout the alignment of climate goals and SDGs to ensure that key issues continue to be proactively addressed.
A critical insight beyond the development of themes and identification of SDG-climate interactions is the misalignment between the time horizons associated with SDGs and climate goals. This misalignment illuminates the need for concerted efforts in developing not only goals with synergistic, reinforcing frameworks, but also to ensure time horizons enable achievable goals within consistent timescales using robust systems of accountability. The roadmap itself does not promise all SDGs and climate goals will be met but rather provides a structured framework through which governments and other stakeholders can accountably work toward doing so while illustrating the importance of transition to a low carbon society in line with the SDGs even after 2030.
In support of recent findings, we highlight the importance of versatility in applying the roadmap to local contexts (de Leon Barido et al., 2020): engagement of knowledge systems at national and subnational levels will enable the development of support mechanisms necessary for success. Localized impacts within specific nations and localities may be under- or over-studied relative to their importance; the development of more focused solutions should be developed with the understanding of the heterogeneity of specific impacts across regions. Priorities of the themes and interactions - a critical factor in initiating the roadmap - will be variable across geographic scales, depending on the relative urgency of the environmental conditions within specified contexts for implementation.
Discussion
The themes and priorities identified during the workshop exhibit the tensions between climate goals and SDGs: while several items serve to reinforce synergies, others present solutions to tensions. For example, techno-ecological synergies, a priority under the siting theme, present a framework for engineering mutually beneficial relationships between technological and ecological systems; the approach has already been proposed to enhance the sustainability of solar power (Hernandez et al., 2019). Conversely, an example of addressing an incompatibility would be behavioral studies of bats that can support better deterrence and mitigation for mortality caused by wind turbines. While tensions are increasingly acknowledged and can be perceived as detrimental to the growth of renewables, they can be proactively managed with careful planning.
To enable a more sustainable transition – this time to a decarbonized society with a high contribution from renewables – the building blocks necessary to support a policy apparatus must be put in place. Our results suggest (1) a gap in research on the six energy-environment themes that emphasize SDGs, and (2) a gap in the global representation of such research efforts. The innovation systems RD3 roadmap for renewable power, proposed here, is a solutions-focused, adaptive, systematic approach to active engagement of global and local knowledge systems. Leveraging expertise at global, national, regional, and local scales will enable broader uptake of new solutions and technologies.
Innovation systems have been broadly promoted as means to manage the environmental impacts of fossil fuels and can likewise play an important role for the renewable sector. While ETISs engage broad knowledge systems, public RD&D is primarily managed by national governments through the allocation of budgetary support. Both funding and programmatic efforts should be placed toward aligning climate goals with SDGs by actively engaging national governments through international agencies such as the IEA, the UN, and the International Renewable Energy Agency.
A dynamic approach should be employed with active engagement of growing global and local knowledge systems throughout the alignment of climate goals and SDGs to ensure solutions are proactively developed, adopted, and implemented. The themes and priorities represent the 2019 state of the field with engagement of only a small portion of the future global knowledge system for the renewable sector. The RD3 roadmap and themes should be systematically and iteratively formalized with coordination of global and local stakeholders. As decision-makers seek to implement the roadmap, critical misalignments such as the different time horizons become increasingly relevant to address. Critically, achieving the 2050 climate goals should not come at the sacrifice of SDG post-2030, regardless of whether they have all been achieved. In making the concerted efforts toward the other recommendations in the roadmap, a system of accountability will be developed to ensure that goals are addressed concurrently.
The innovation systems RD3 roadmap for renewable power has broad applicability and versatility across spatial scales; local contexts should play a critical role throughout its implementation. Advancements in both science and technology through the RD3 roadmap will more likely be adopted with local acceptance, engagement, and political support. Widespread adoption of solutions plays the most critical role in minimizing trade-offs stemming from the large-scale technological transition required to meet climate goals with sustainable growth of renewable power.
Data Availability Statement
The raw data supporting the conclusions of this article will be made available by the authors, without undue reservation.
Author Contributions
RH, SJ, BK, and NK collected the data. RH and SJ analyzed the data. RH and SJ co-wrote the manuscript with significant input from NK. All authors contributed to the article and approved the submitted version.
Funding
Funding for RH by grants from the Electric Power Research Institute (EPRI), California Energy Commission EPC-15-060 (94-6036494), Department of Energy Solar Energy Technologies Office (DE-EE0008746), Agricultural Experiment Station Hatch projects (CA-R-A-6689- H; CA-D-LAW-2352-H), and a cooperative agreement with the Bureau of Land Management (L19AC00279) and for SJ by EPRI and a Catalyst Award from Johns Hopkins University.
Conflict of Interest
The authors declare that the research was conducted in the absence of any commercial or financial relationships that could be construed as a potential conflict of interest.
Acknowledgments
The authors are thankful to Ashley Bennett, Wally Erickson, Tim Hayes, Garvin Heath, Ihor Hlorowskyj, Cliff Ho, Marcus Krembs, James Kuiper, Ken Ladwig, Cara Libby, Chuck Ludwig, Gavin Meinschein, Kaitlin Monaghan, Christian Newman, Rob O'Neal, Ed Pilep, Annette Rohr, Stephanie Shaw, Michael Speerschneider, Lee Walston, and Stu Webster who contributed to the Environmental Aspects of Renewable Energy Workshop. We thank Alex Kessler, Linxin Gu, and Shreya Rangarajan at Johns Hopkins University for data collection assistance.
Supplementary Material
The Supplementary Material for this article can be found online at: https://www.frontiersin.org/articles/10.3389/frsus.2020.583090/full#supplementary-material
References
Anadon, L. D., Baker, E., and Bosetti, V. (2017). Integrating uncertainty into public energy research and development decisions. Nat. Energy 2, 1–14. doi: 10.1038/nenergy.2017.71
Armstrong, A., Page, T., Thackeray, S. J., Hernandez, R. R., and Jones, I. D. (2020). Integrating environmental understanding into freshwater floatovoltaic deployment using an effects hierarchy and decision trees. Environ. Res. Lett. 14:114055.
Bento, N., and Fontes, M. (2018). Legitimation and Guidance in Scaling Up Energy Innovation Systems Manchester, UK: 9th International Sustainability Transitions Conference; University of Manchester.
Bergek, A., Jacobsson, S., Carlsson, B., Lindmark, S., and Rickne, A. (2008). Analyzing the functional dynamics of technological innovation systems: a scheme of analysis. Res. Policy 37, 407–429. doi: 10.1016/j.respol.2007.12.003
BNEF (2019). Global Trends in Renewable Energy Investment. Frankfurt School-UNEP Centre. Available online at: http://www.fs-unep-centre.org (accessed January 1, 2020).
Cagle, A. E., Armstrong, A., Exley, G., Grodsky, S. M., Macknick, J., Sherwin, J., et al. (2020). The land sparing, water surface use efficiency, and water surface transformation of floating photovoltaic solar Energy installations. Sustainability 12:8154. doi: 10.3390/su12198154
Cash, D. W., Clark, W. C., Alcock, F., Dickson, N. M., Eckley, N., Guston, D. H., et al. (2003). Knowledge systems for sustainable development. Proc. Natl. Acad. Sci. U. S. A. 100, 8086–8091. doi: 10.1073/pnas.1231332100
CEC (2013). CEC Workshop: Roadmap to Identify Future Research for Energy Efficient Natural Gas Use in Buildings. California Energy Commission. Available online at: https://www.etcc-ca.com/news/cec-workshop-roadmap-identify-future-research-energy-efficient-natural-gas-use-buildings (accessed January 1, 2020).
Chowdhury, M. S., Rahman, K. S., Chowdhury, T., Nuthammachot, N., Techato, K., Akhtaruzzaman, M., et al. (2020). An overview of solar photovoltaic panels' end-of-life material recycling. Energy Strateg. Rev. 27:v100431. doi: 10.1016/j.esr.2019.100431
Clar, G., and Sautter, B. (2014). Research driven clusters at the heart of (trans-) regional learning and priority-setting processes. J. Knowl. Econ. 5, 156–180. doi: 10.1007/s13132-014-0180-0
Cornell, S., Berkhout, F., Tuinstra, W., Tàbara, J. D., Jäger, J., Chabay, I., et al. (2013). Opening up knowledge systems for better responses to global environmental change. Environ. Sci. Policy 28, 60–70. doi: 10.1016/j.envsci.2012.11.008
de Leon Barido, D. P., Avila, N., and Kammen, D. M. (2020). Exploring the enabling environments, inherent characteristics and intrinsic motivations fostering global electricity decarbonization. Energy Res. Soc. Sci. 61:101343. doi: 10.1016/j.erss.2019.101343
Dufva, M., and Ahlqvst, T. (2015). Knowledge creation dynamics in foresight: a knowledge typology and exploratory method to analyze foresight workshops. Technol. Forecast. Soc. 94, 251–268. doi: 10.1016/j.techfore.2014.10.007
EPRI (2019a). Environmental Aspects of Renewables Workshop: 2019 Workshop Summary and Research Needs. Electric Power Research Institute. Report 3002016553. Palo Alto, CA: EPRI.
EPRI (2019b). EPRI- Environmental Aspects of Renewables: Current State of Science, Knowledge Gaps and Research Opportunities. Electric Power Research Institute. Report 3002012946. Palo Alto, CA: EPRI.
Gallagher, K. S., Grübler, A., Kuhl, L., Nemet, G., and Wilson, C. (2012). The energy technology innovation system. Annu. Rev. Env. Resour. 37, 137–162. doi: 10.1146/annurev-environ-060311-133915
Gatto, A., and Drago, C. (2020). A taxonomy of energy resilience. Energy Pol. 136:111007. doi: 10.1016/j.enpol.2019.111007
Grodsky, S. M., and Hernandez, R. R. (2020). Reduced ecosystem services of desert plants from ground-mounted solar energy development. Nat. Sustain. 1–8. doi: 10.1038/s41893-020-0574-x
Hao, S., Kuah, A. T., Rudd, C. D., Wong, K. H., Lai, N. Y. G., Mao, J., et al. (2020). A circular economy approach to green energy: wind turbine, waste, and material recovery. Sci. Total Environ. 702:135054. doi: 10.1016/j.scitotenv.2019.135054
Healy, N., and Barry, J. (2017). Politicizing energy justice and energy system transitions: fossil fuel divestment and a “just transition”. Energ. Policy. 108, v451–459. doi: 10.1016/j.enpol.2017.06.014
Hernandez, R. R., Armstrong, A., Burney, J., Ryan, G., Moore-O'Leary, K., Diédhiou, I., et al. (2019). Techno–ecological synergies of solar energy for global sustainability. Nat. Sustain. 2, 560–568. doi: 10.1038/s41893-019-0309-z
IEA (2019a). Sustainable Development Scenario, World Energy Outlook. International Energy Agency. Available online at: https://www.iea.org/reports/world-energy-model/sustainable-development-scenario (accessed March, 3, 2020).
IEA (2019b). Energy RD& D Online Data Service. International Energy Agency. Available online at: http://www.iea.org/statistics/RDDonlinedataservice/ (accessed December, 1, 2019).
IRENA (2019). Renewable Capacity Statistics 2019. International Renewable Energy Agency Available online at: http://irena.org/-/media/Files/IRENA/Agency/Publication/2018/Mar/IRENA_RE_Capacity_Statistics_2018.pdf. (accessed October 4, 2019).
Jordaan, S. M., Romo-Rabago, E., McLeary, R., Reidy, L., Nazari, J., and Herremans, I. M. (2017). The role of energy technology innovation in reducing greenhouse gas emissions: a case study of Canada. Renew. Sust. Energ. Rev. 78, 1397–1409. doi: 10.1016/j.rser.2017.05.162
Kemp, R., Schot, J., and Hoogma, R. (1998). Regime shifts to sustainability through processes of niche formation: the approach of strategic niche management. Technol. Anal. Strateg. 10, 175–198. doi: 10.1080/09537329808524310
Lang, D. J., Wiek, A., Bergmann, M., Stauffacher, M., Martens, P., Moll, P., et al. (2012). Transdisciplinary research in sustainability science: practice, principles and challenges. Sustain. Sci. 7, 25–43. doi: 10.1007/s11625-011-0149-x
Leong, M., Bazoune, A., Wallace, D. R., Tang, V., and Seering, W. P. (2011). “Towards a tool for characterizing the progression of academic research,” in International Design Engineering Technical Conferences and Computers and Information in Engineering Conference 54860 (Washington, DC), 405–414.
Liggett, M. L. (2014). Research Maturity Levels: Implementation and Refinement of an Online Tool for Characterizing the Progression of Research (Doctoral dissertation). Cambridge, MA: Massachusetts Institute of Technology.
Liu, P., and Barlow, C. Y. (2017). Wind turbine blade waste in 2050. Waste Manage. 62, 229–240. doi: 10.1016/j.wasman.2017.02.007
Machado, P. G., Mouette, D., Rathmann, R., dos Santos, E., and Peyerl, D. (2020). “Is energy planning moving towards sustainable development? A review of energy systems modeling and their focus on sustainability,” in International Business, Trade and Institutional Sustainability (Cham: Springer), 629–644.
McCullough, E. B., and Matson, P. A. (2016). Evolution of the knowledge system for agricultural development in the Yaqui Valley, Sonora, Mexico. Proc. Natl. Acad. Sci. U. S. A. 113, 4609–4614. doi: 10.1073/pnas.1011602108
McDowall, W. (2012). Technology roadmaps for transition management: the case of hydrogen energy. Technol. Forecast. Soc. Change 79, 530–542. doi: 10.1016/j.techfore.2011.10.002
Moore-O'Leary, K. A., Hernandez, R. R., Johnston, D. S., Abella, S. R., Tanner, K. E., Swanson, A. C., et al. (2017). Sustainability of utility-scale solar energy–critical ecological concepts. Front. Ecol. Environ. 15, 385–394. doi: 10.1002/fee.1517
Negro, S. O., Alkemade, F., and Hekkert, M. P. (2012). Why does renewable energy diffuse so slowly? A review of innovation system problems. Renew. Sust. Energ. Rev. 16, 3836–3846. doi: 10.1016/j.rser.2012.03.043
Nerini, F. F., Sovacool, B., Hughes, N., Cozzi, L., Cosgrave, E., Howells, M., et al. (2019). Connecting climate action with other sustainable development goals. Nat. Sustain. 2, v674–680. doi: 10.1038/s41893-019-0334-y
Nerini, F. F., Tomei, J., To, L. S., Bisaga, I., Parikh, P., Black, M., et al. (2018). Mapping synergies and trade-offs between energy and the sustainable development goals. Nat. Energy 3, 10–15. doi: 10.1038/s41560-017-0036-5
Notton, G., Nivet, M. L., Voyant, C., Paoli, C., Darras, C., Motte, F., and Fouilloy, A. (2018). Intermittent and stochastic character of renewable energy sources: consequences, cost of intermittence and benefit of forecasting. Renew. Sust. Energ. Rev. 87, 96–105. doi: 10.1016/j.rser.2018.02.007
O'Haire, C., McPheeters, M., Nakamoto, E., LaBrant, L., Most, C., Lee, K., et al. (2011). Engaging Stakeholders to Identify and Prioritize Future Research Needs. Rockville, MD: Agency for Healthcare Research and Quality.
Parajuly, K., Kuehr, R., Awasthi, A. K., Fitzpatrick, C., Lepawsky, J., Smith, E., et al. (2019). Future E-Waste Scenarios. United Nations Environment Programme. Available online at: http://hdl.handle.net/20.500.11822/30809 (accessed January 1, 2020).
Patel, M., Kok, K., and Rothman, D. S. (2007). Participatory scenario construction in land use analysis: an insight into the experiences created by stakeholder involvement in Northern Mediterranean. Land Use Policy 24, 546–561. doi: 10.1016/j.landusepol.2006.02.005
Redón-Santafé, M., Ferrer-Gisbert, P. S., Sánchez-Romero, F. J., Torregrosa Soler, J. B., Ferran Gozalvez, J. J., and Ferrer Gisbert, C. M. (2014). Implementation of a photovoltaic floating cover for irrigation reservoirs. J. Clean. Prod. 66, 568–570. doi: 10.1016/j.jclepro.2013.11.006
Rip, A. (2012). The context of innovation journeys. Creat. Innov. Manag. 21, 158–170. doi: 10.1111/j.1467-8691.2012.00640.x
Sagar, A. D., and Holdren, J. P. (2002). Assessing the global energy innovation system: some key issues. Energy Policy 30, 465–469. doi: 10.1016/S0301-4215(01)00117-3
Scherhaufer, P., Höltinger, S., Salak, B., Schauppenlehner, T., and Schmidt, J. (2018). A participatory integrated assessment of the social acceptance of wind energy. Energy Res. Soc. Sci. 45, 164–172. doi: 10.1016/j.erss.2018.06.022
Tawney, L., and Weischer, L. (2011). “Innovation and technology transfer: supporting low carbon development with climate finance,” in World Resources Institute Working Paper. Available online at: http://www.wri.org/publication/innovation-and-technology-transfer (accessed January 1, 2020).
Weckend, S., Wade, A., and Heath, G. A. (2016). End of Life Management: Solar Photovoltaic Panels. Golden, CO, National Renewable Energy Lab (NREL). No. NREL/TP-6A20-73852.
Xie, H., Zhang, Y., and Duan, K. (2020). Evolutionary overview of urban expansion based on bibliometric analysis in Web of Science from 1990 to 2019. Habit. Int. 95:102100. doi: 10.1016/j.habitatint.2019.102100
Xu, Y., Li, J., Tan, Q., Peters, A. L., and Yang, C. (2018). Global status of recycling waste solar panels: a review. Waste Manage. 75, 450–458. doi: 10.1016/j.wasman.2018.01.036
Keywords: circular economy, climate change mitigation, just transition, land-use and land-cover change, public acceptance, recycling, sustainability, wildlife conservation
Citation: Hernandez RR, Jordaan SM, Kaldunski B and Kumar N (2020) Aligning Climate Change and Sustainable Development Goals With an Innovation Systems Roadmap for Renewable Power. Front. Sustain. 1:583090. doi: 10.3389/frsus.2020.583090
Received: 14 July 2020; Accepted: 12 November 2020;
Published: 18 December 2020.
Edited by:
Christopher Mutel, Paul Scherrer Institut (PSI), SwitzerlandReviewed by:
Mikołaj Owsianiak, Technical University of Denmark, DenmarkMichael Patrick Tsang, Other, Oman
Copyright © 2020 Hernandez, Jordaan, Kaldunski and Kumar. This is an open-access article distributed under the terms of the Creative Commons Attribution License (CC BY). The use, distribution or reproduction in other forums is permitted, provided the original author(s) and the copyright owner(s) are credited and that the original publication in this journal is cited, in accordance with accepted academic practice. No use, distribution or reproduction is permitted which does not comply with these terms.
*Correspondence: Rebecca R. Hernandez, cnJoZXJuYW5kZXomI3gwMDA0MDt1Y2RhdmlzLmVkdQ==
†These authors share first authorship