- 1Advanced Navigation and Control Systems (ANCS) Laboratory, Department of Mechanical and Aerospace Engineering, University at Buffalo, Buffalo, NY, United States
- 2Center for Multisource Information Fusion (CMIF), University at Buffalo, Buffalo, NY, United States
- 3Center for Space Cyber Strategy and Cyber Security, School of Engineering and Applied Sciences, University at Buffalo, Buffalo, NY, United States
Kessler’s Syndrome is a global phenomenon characterized by the presence of tens of millions of debris pieces of various sizes that disrupt satellite operations. This article delves into the potential outcomes of a Kessler’s Syndrome occurrence and its implications on satellite operations. The potential threats posed by this scenario are discussed, including the implications of minimal to no satellite function on human impacts, including crashes and minimized or disrupted functions in essential utility services, as these sectors heavily rely on satellites. In addition, we discuss how the loss of satellite communications could gradually or rapidly affect global affairs. As humans are a dominant force on Earth, their endangerment would reverberate throughout the ecological system, potentially leading to the demise of other species. It is crucial for policymakers and relevant stakeholders to recognize these implications and work towards safeguarding satellite communication to mitigate potential negative outcomes for humanity’s wellbeing and progress. The recycling of space debris emerges as a promising and long-term sustainable solution to the mitigation of space debris. By repurposing decommissioned satellites and debris into useful materials to support other space missions, this recycling strategy presents a win-win scenario, promoting environmental sustainability and resource efficiency in space exploration.
1 Introduction
Space has emerged as a key domain since the historic launch of Sputnik 1, the first man-made satellite, in 1957 Dickson (2011). Since then, there has been an exponential proliferation of artificial objects in space Anz-Meador (2020) and Bastida Virgili et al. (2016). These satellites serve diverse purposes, from military and commercial applications to navigation and communication systems. Satellite communication has revolutionized global connectivity, becoming indispensable in the modern world. With more than 8,000 active and defunct satellites orbiting Earth Cowardin (2022), satellite systems have become integral to the daily lives of individuals, businesses, and organizations around the world. The significance of satellite communication is evident from the fact that, as of April 2022, there were 5.03 billion internet users, representing 63.1% of the world’s population, highlighting the massive dependence on satellite communication for various activities Petrosyan, (2023).
However, this escalating presence of satellites in space has led to a growing concern: space debris. In particular, the collision of the Iridium 33 and Kosmos-2251 satellites in 2009 generated a cloud of debris that continues to pose a threat to other satellites Oleksyn (2009) and Garcia (2021). Additionally, India’s antisatellite missile test in 2019 resulted in approximately 400 pieces of orbital debris, increasing the risk to the International Space Station (ISS) Molayath and Sanal Kumar (2010) and Mariappan et al. (Mariappan et al., 2019; Mariappan et al., 2020). Low Earth and geostationary (GEO) orbits, where many operational satellites are located, have become cluttered with space debris, some traveling at hypervelocity speeds of 7 km/s to 15 km/s Sample (2016). Even minuscule debris particles possess significant kinetic energy, posing threats to satellites and spacecraft. The risk of collision-induced chain reactions, as theorized by Donald J. Kessler in 1978, has gained traction and is now known as “Kessler’s Syndrome” Kessler and Cour-Palais (1978). This syndrome predicts an escalating space debris population that leads to an increased likelihood of collisions and further debris creation, resulting in a cascade of detrimental impacts. Such collisions, even minor ones, can set off a catastrophic chain reaction, jeopardizing all existing satellites and filling orbits with high-velocity debris. Accessing space orbits would become immensely challenging, and the prospect of exploring outer space might be compromised. Computer-generated future scenarios indicate that the space debris population may reach saturation and collisions will persist even without new spacecraft launches Liou and Johnson (2006) and National Research Council (2011).
This article delves into the potential outcomes of the occurrence of Kessler’s Syndrome. While space exploration has propelled humanity’s understanding of the cosmos, it is essential to recognize and address the potential threats posed by space debris. Collaborative efforts, innovative mitigation techniques, and proactive measures are essential to safeguard the future of satellite communication and space exploration. The responsibility falls on all nations to work together, akin to global agreements addressing environmental issues, to protect our collective interests and ensure the long-term sustainability of space activities. As we navigate the complexities of space, it is vital to preserve the integrity of our endeavors and protect against the adverse impacts of space debris on humanity’s future.
2 Impact on major sectors without satellites
In the contemporary era, the accessibility of an array of conveniences within arm’s reach has become a hallmark of modern civilization, primarily enabled by the vast network of thousands of Earth-orbiting satellites facilitating real-time data collection and transmission. However, it is imperative to consider the potential consequences should we encounter a sudden loss of control or communication with all satellite systems within a compressed time frame Hollingham (2013). In this article, “satellite communication” pertains to the retrieval of satellite data from ground stations in the event of satellite damage, whether partial or complete. This section delves into the prospective ramifications of Kessler’s Syndrome, envisioning a scenario where satellite communication is entirely compromised over the span of two to three decades, and explores its profound implications on humans. The authors highlight the increasing risk of collisions between active space vehicles and space debris, an effect attributed to the increase in the affordability of satellite launches and the commercialization of the space industry Tao et al. (2022). Understanding the gravity of such a prospect is vital for informed decision-making and robust contingency planning.
2.1 Space industry
The present investigation examines the potential threats posed by Kessler’s Syndrome to the space industry and its implications on satellite operations and access to space. Current data suggest that, at this point, the probability of an abrupt occurrence of this syndrome over a short period remains relatively low Kessler (2009). It is estimated that destructive collisions below 1,000 km altitude occur approximately every 3.9 years over the span of 1,000 years, considering certain assumptions such as debris size being greater than or equal to 10 cm, controlled launch rates, and absence of explosions Lewis (2020). The amount of space debris in orbit by size is shown in Table 1. However, it is essential to recognize that unforeseen factors, such as the launch of additional satellites in the coming years, anti-satellite missile tests, and satellite collisions, could escalate these risks. Even tiny debris measuring 1 mm, carrying an energy equivalent to 71 J or 0.0003 TNT/kg, could trigger catastrophic collisions and generate more fragments The Aerospace Corporation (2023). A collision with a 10 cm single of debris, possessing high kinetic energy, can further exacerbate the situation. Additionally, the presence of tens of millions of tiny debris pieces, below or equal to 1 cm in size, presents tracking challenges, hindering the efficacy of current mitigation techniques that involve maneuvering spacecraft to avoid potential impacts.
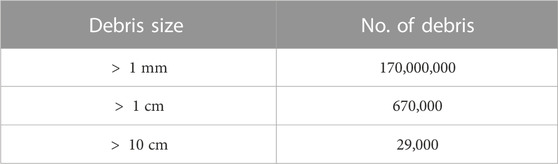
TABLE 1. Size distribution of man-made space debris. Based on data from The Aerospace Corporation (2023).
The lack of an active removal system in space could lead to an exponential increase in objects in low-Earth orbit (LEO), impeding spacecraft launches and maneuvering. Consequently, loss of control within LEO or significant communication disruptions with satellites could severely limit access to space for future missions. Furthermore, with the appearance of Kessler’s Syndrome, the launch of satellites would be a higher risk, as the orbits could be densely populated with debris clouds exhibiting varying kinetic energies The Aerospace Corporation (2023). Such an eventuality may necessitate a shutdown of global space programs until viable solutions are devised, potentially leading to economic imbalances and job losses within the space industry. The ensuing section delves into the cascading effects on other industries resulting from space program limitations due to the inaccessibility of space.
2.2 Impact of GNSS disruption on various industries
The Global Navigation Satellite Systems (GNSS) is a critical satellite-based navigation system that provides essential guidance to humans and vehicles navigating various terrains. Its uses are widespread, with essential industries such as defense, aviation, space exploration, maritime, rail transportation, and road networks benefiting from it Pistoia (2009) and The Aerospace Corporation (2021). GNSS satellites operate primarily in Medium Earth Orbit (MEO), at altitudes ranging from 2,000 km to 31,570 km above the Earth’s surface. MEO, which is located between GEO and LEO, has the advantage of having a lower population of space junk than its counterparts, LEO and GEO European Space Agency (ESA) (2023). Because of the increasing worldwide competitiveness in space activities, the LEO, an ever-increasing concentration of space objects, is attained the saturation. The increase in debris in LEO poses a serious threat to current and future space operations, compromising our ability to launch and maintain spacecraft in this important region Colvin et al. (2023). If LEO becomes overcrowded with orbital debris and effective mitigation techniques remain undeveloped, the chances of putting a spacecraft into orbit will diminish dramatically. It is important to think on long-term scales, which would take LEO problems to other orbits, such as MEO and GEO. Thus, looking at the effect of GPS operations in MEO orbits is crucial for future debris mitigation. Figure 1 shows the number of trackable objects in each orbital domain.
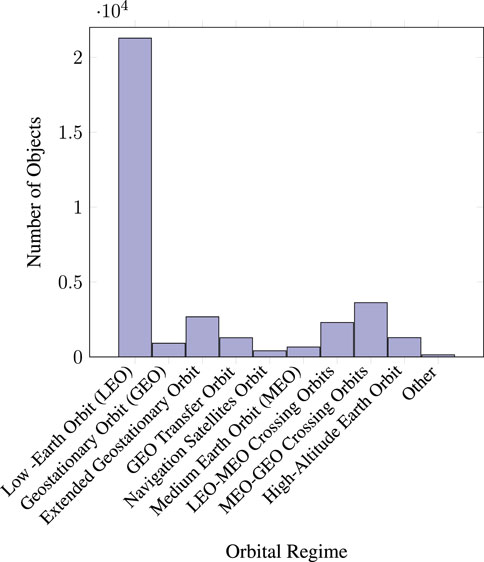
FIGURE 1. Count of objects in various orbital regimes. Based on data from European Space Agency (ESA) (2023).
This section explores the potential consequences of a GNSS disruption on many sectors, particularly if our ability to launch new satellites into orbit is jeopardized. It is critical to fully appreciate the implications in order to identify vulnerabilities and build contingency plans to reduce the impact of GNSS unavailability across these varied businesses. At the moment, Earth’s orbit is home to a constellation of 31 active Global Positioning Satellites (GPS) United States Space Force (2022a), 35 active BeiDou satellites, 24+ Galileo satellites, 24+ GLONASS (Globalnaya Navigazionnaya Sputnikovaya Sistema, or Global Navigation Satellite System), 7 Indian Regional Navigation Satellite System (IRNSS)/Navigation Indian Constellation (NavIC), and 7 Quasi-Zenith Satellite System (QZSS) United States Space Force (2021d).
2.2.1 Impact on the defense sector
The defense sector is heavily dependent on satellite communication (SATCOM) for critical military operations, including communication, missile guidance, search and rescue missions, and the piloting of unmanned aerial vehicles (UAVs), among other essential functions United States Space Force (United States Space Force, 2021b; United States Space Force, 2022a). Loss of satellite communication systems would significantly impede the sector’s ability to operate at full capacity. Without reliable communication systems, transmitting messages would become difficult for military personnel. Although an analog system serves as a backup mode of communication United States Space Force (2019), its limited operational range poses challenges for effective communication. Relying solely on analog systems for extensive military operations would prove impractical. Currently, the defense sector worldwide exhibits an average reliance of 70%–80% on satellite communication, and this figure is expected to increase in the future, although the exact percentage remains classified for security reasons Weeden and Samson (2018).
2.2.2 Airline industry
The airline industry relies extensively on the Flight Management System (FMS) to automate various in-flight operations that were previously performed manually, thus facilitating smoother flight operations Spitzer (2018). FMS uses multiple sensors, including GPS, to calculate the position of the aircraft and fuel levels, among other critical parameters Stössel (2015). A breakdown in the satellite communication system would disrupt the transmission of GPS data, potentially compromising the FMS functionality. Although modern aircrafts are equipped with backup sensors to facilitate landing in case of technical failures Stössel (2015), relying solely on analog communication for flight operations midst the multitude of flights poses significant risks. In such an event, communication between Air Traffic Control (ATC) and flights could be severely hampered, affecting effective flight management and response to emergencies European Space Agency (2022) and Federal Aviation Administration (FAA) (2022).
Without access to satellite communication systems, pilots and ATC would not be able to receive real-time weather updates, leading to potential risks of navigate under hazardous weather conditions Norton and Omberg (1947). Given the projected future increase in global air passenger traffic Statista Research Department (2022a), the implications of disrupted satellite communication in the airline industry could be far-reaching. The industry plays a vital role in various sectors, including medical tourism, vaccine distribution, and air cargo delivery International Air Transport Association (2022) and Dalen and Alpert (2019). A loss of effective satellite communication could hinder the seamless functioning of these critical services, potentially jeopardizing lives and putting off urgent medical care.
In addition, the airline industry maintains a considerable workforce, with nearly 87.7 million people employed directly and indirectly Salas (2022a). In the event of reduced or unavailable satellite communication, the industry may face temporary or permanent downsizing, leading to job losses and economic instability for numerous individuals and families.
2.2.3 Marine industry
Modern ships rely heavily on sophisticated systems, such as Automatic Identification Systems (AIS) and GNSS, among others, to ensure safe navigation and collision avoidance during their voyages Tetreault (2005) and Coast Guard—Office of Navigation Systems and Code of Federal Regulations (2019). Satellite communication systems play a crucial role in facilitating seamless communication and weather monitoring, helping ships reach their destinations safely. In the event of satellite communication failure, ships would be forced to resort to radar and analog communication systems. However, the maximum range of a ship radar for object detection is limited to 200 nautical miles Jenn (2019). Without proper communication with the Vessel Traffic Services, ships can be forced to suspend shipping operations until a viable communication solution is established or resort to navigating through analog means.
As ocean shipping serves as the primary mode of transport for global trade Placek (2022), disruptions in satellite communication would have far-reaching effects on various industries relying on shipping services. The shipping industry provides essential logistical support for numerous sectors, and any disruption could lead to supply chain disruptions and economic repercussions. According to estimates for 2011, the global marine fishery industry employs more than 260 million people Teh and Sumaila (2011). The potential reduction in shipping operations due to communication system failures would put these jobs at risk, leading to economic challenges for the workforce and affecting local communities dependent on the maritime sector.
2.2.4 Railway industry
The global railway network, which comprises an extensive route length of more than 1.3 million kilometers, is heavily dependent on GNSS technology for numerous indispensable functions Salas (2022b). Modern railway systems around the world are equipped with GNSS-based Positive Train Control systems, which play a critical role in preventing train collisions and ensuring safe operations United States Space Force (2021a). GNSS serves as a crucial tool for tracking locomotives, rail cars and wayside equipment, facilitating the synchronization of communication systems and enabling seamless communication among locomotive engineers, dispatchers, trains, airports, rail stations, and ports. Passengers also benefit from accurate train location information, allowing them to predict arrival times with precision.
Given the paramount importance of GNSS for navigation and signaling, the uninterrupted operation of current rails depends on this indispensable technology. A potential reduction in GNSS availability could lead to the curtailment of railway operations, affecting millions of passengers who rely heavily on train commutes for transportation Hughes (2020). By 2022, the rail freight industry in the United States provided employment to approximately 135,000 individuals Statista Research Department (2022c). Similarly, Indian railways employ an estimated 1.54 million individuals, while the Chinese railway industry boasts nearly 2 million employees The Economic Times (ET) (2017) and Statista Research Department (2021b). These statistics underscore the significant contribution of the railway sector to the economy on a global scale, and any potential minimization of the railways due to GNSS disruptions would jeopardize these jobs.
In addition, the railway industry plays a crucial role in transporting cargo to various locations. In the United States, rail freight volumes reached 2.53 trillion tonnes kilometer in 2018, but the impact of the COVID-19 pandemic saw this figure decrease to 2.1 trillion tonnes kilometer by 2020 Statista Research Department (2022b). In India, Indian Railways transported more than 1.42 billion metric tons of freight in fiscal year 2022 Sun (2023). In China, the rail industry transported an impressive 4.77 billion tons of rail cargo in 2021 Statista Research Department (2023c).
2.2.5 Roadways
In 2015, global road freight activity reached approximately 19,000 billion tonne-kilometers, and projections indicate that this figure will more than double by 2050. It is expected that the volume of road freight will increase by 91% in 2050 compared to 2020 Statista Research Department (2021c). However, the efficient functioning of road transport is highly dependent on GNSS technology. GNSS plays an essential role in improving vehicle efficiency and safety on roads, streets and mass transit networks by mitigating routing and dispatch challenges for commercial vehicles, as well as for mass transit, road maintenance, and emergency vehicles United States Space Force (2021e). The global installed base of Global Navigation Satellite System devices was 6.5 billion units in 2021, with forecasts predicting an increase to 10.6 billion devices by 2031 Alsop (2022).
In addition, numerous nations use GNSS to inspect highway and road networks, enabling the detection of various features such as service stations, emergency services, entry and exit ramps, and road damage. The data collected feed into Geographic Information Systems, contributing to reduced maintenance and repair expenses and improved driver safety. GNSS technology also facilitates the automation of traffic lights Munoz-Organero et al. (2018).
Taking into account the immense scale of road travel, statistics indicate that Americans engage in 1.1 billion travel activities daily, each person taking approximately four trips within the country Bureau of Transportation Statistics (2017). Additionally, an average of 236 billion vehicles travel on the road in the United States every month Bureau of Transportation Statistics (2022). With such high vehicle traffic, GNSS monitoring is crucial for accident prevention, especially as we move towards automated driving. The absence of GNSS would present significant challenges to vehicle monitoring and navigation in a world increasingly dependent on transportation systems. This disruption would profoundly affect various industries, as nearly every sector depends on efficient transportation systems to take care of the commute of workers and deliver products to customers.
2.2.6 GNSS timing
GNSS plays a critical role in providing accurate determination of time, longitude, latitude and altitude, making it a crucial fourth dimension for various industries and economic activities United States Space Force (2022b). GNSS satellites are equipped with highly precise atomic clocks, enabling the dissemination of real-time GNSS signals with exceptional accuracy. Access to time within 100 billionths of a second eliminates the need for costly investments in maintaining atomic clocks, benefiting industries that heavily rely on precise timing.
Numerous sectors, such as electrical power grids, financial networks, and communication systems, depend on precise timing to achieve synchronization and operational efficiency. GNSS time enables companies to optimize operations, reduce costs, and improve capabilities. In wireless telephone and data networks, perfectly synchronized base stations improve spectrum usage and improve mobile phone performance. Digital radio broadcasting services also take advantage of GNSS time to ensure simultaneous bit transmission from all radio stations, minimizing listener delays during station switching.
The financial sector benefits from GNSS time for accurate time-stamping of transactions, ensuring precise record-keeping and traceability. Furthermore, integration of GNSS time into seismic monitoring networks enables rapid pinpointing of epicenters of earthquakes and other seismic phenomena.
The utility and power industries have specific time and frequency requirements to ensure reliable power transmission and distribution. GNSS time plays a crucial role in meeting these demands United States Space Force (2022b). However, the potential risks to satellite communication systems pose threats to the global economy, potentially leading to crashes and minimized or disrupted functions in essential utility services, as these sectors heavily rely on GNSS timing. Such economic downturns could result in widespread job losses that affect billions of people worldwide. Further discussions on the implications of losing GNSS time in the energy and utility sectors are presented later in this article.
As technology continues to advance rapidly, the reliance on GNSS timing is expected to grow significantly in the future. Therefore, protecting and fortifying the GNSS infrastructure becomes imperative to support global economic stability and ensure the continued operation of essential utility services.
2.2.7 Agriculture
From 1990 to 2012, agricultural activities occupied a significant proportion of the global land area, accounting for 35.79% in 2011 Statista Research Department (2015). The implementation of precision agriculture has revolutionized agricultural practices through the utilization of GNSS-based applications United States Space Force (2021c). Precision agriculture encompasses a diverse range of tasks, including farm planning, tractor guidance, variable rate applications, and yield mapping, among others. The integration of GNSS technology has proven invaluable to farmers, allowing seamless operations even in challenging conditions such as poor visibility caused by rain, dust, fog or darkness.
Precision agriculture, powered by GNSS, facilitates the application and distribution of precise pesticides, herbicides, and fertilizers, leading to cost reduction, increased production, and enhanced ecological sustainability. Manufacturers of GNSS equipment have developed various tools to improve productivity and efficiency in precision agricultural practices. Farmers worldwide use GNSS services to optimize their operations Katz and Murphy (1997), and weather forecasts, accessible through satellite communication systems Ritchie (2021), play an essential role in guiding their farming decisions.
The minimization or loss of satellite communication systems would profoundly impact agriculture, directly affecting food production. The indispensable role of GNSS in modern farming practices makes it challenging for farmers to revert to traditional methods without its support. Ensuring the continued operation of satellite communication systems is crucial for maintaining efficient and sustainable agricultural practices and securing global food production. Further discussions on the implications of losing satellite communication on the agricultural sector are presented in subsequent sections of this article.
2.3 Energy and utility sector
The energy and utility sector plays a vital role in society and is highly dependent on GNSS time to efficiently distribute and transmit power United States Space Force (2022b). However, the sector faces significant risks when satellite communication systems are reduced or completely lost. Additionally, the energy and utility sector’s operations depend on various interlinked services, including banking for financial transactions, the transportation sector for the delivery of essential goods and the distribution of utilities such as gas.
The global usage of electricity is substantial, with nearly 7 billion out of 7.67 billion people worldwide using electricity daily Ritchie (2021). In 2019, global electricity consumption reached approximately 23,900 TWh Statista Research Department (2022d). The availability of electricity is crucial for individuals, communities, and organizations alike, and any disruption would significantly impact their functioning. In a testimonial report released by a congressional committee in 2015 House and Senate (2015), it was stated that the absence of electricity could potentially lead to a 90% mortality rate among Americans. This assertion may hold true not only for Americans but for a significant portion of the global population, given the indispensable role that electricity plays in everyday survival for people worldwide.
The energy sector relies on various sources, such as renewable energy, nuclear reactors, coal, natural gas, and liquid fuels, for electricity generation Statista Research Department (2021a). Figure 2 displays the distribution of energy sources used to generate electricity. However, this sector is highly dependent on the transportation sector to ensure the supply of resources and the distribution of electricity. In the absence of proper transportation functioning, sectors depending on energy and utility, including banking and hospitality, would stop working or face significant limitations.
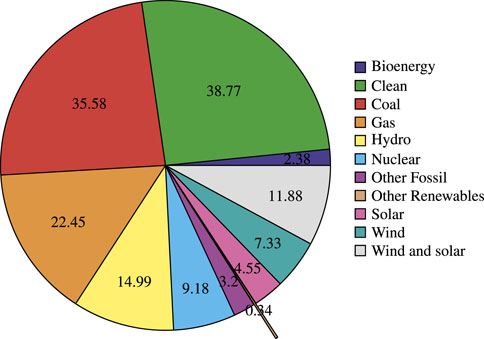
FIGURE 2. Worldwide Power Generation in 2022 by energy source. Based on data from Statista Research Department (2021a).
As satellite communication systems contribute substantially to the efficiency and reliability of the energy and utility sector, any potential loss of this technology could have far-reaching consequences. Further discussions on the implications of the loss of satellite communication systems on the energy and utility sector and associated industries will be discussed in later sections of this article. The protection of satellite communication stability is imperative to ensure uninterrupted energy and utility services and the smooth functioning of numerous interconnected sectors in the modern world.
2.3.1 Renewable energy
Renewable energy plays a significant role in global electricity generation, contributing approximately 6.99 TW-hours Statista Research Department (2021a). Among various renewable energy sources, biomass, hydroelectric, geothermal, wind, and solar energy are the main contributors United States Energy Information Administration (U.S. Energy Information Administration, 2023b and U.S. Energy Information Administration, 2022).
Hydropower is based on the water cycle, and electricity is generated through dams utilizing elevation differences. During major power outages, many countries depend heavily on this energy source. Large dams employ numerous sensors, which rely on satellite communication, for crucial tasks such as opening and closing gates, dam assessment, power generation and monitoring Coombs and Braithwaite (2021). Although manual gate operation is possible, efficiently controlling the water allowance without an effective satellite communication system may prove challenging.
Geothermal energy Heasler et al. (2009) requires precise location mapping, a task facilitated by GNSS technology. Satellite communication enables complete monitoring of entire geothermal plants with fewer human resources, ensuring timely reporting of any failures Morgan (2020). The development and management of solar and wind farms also benefit from satellite technology. Consequently, the dependence on satellite communication becomes crucial for the efficient operation of these renewable energy facilities.
Countries that rely on other nations for resources to generate electricity, such as biomass and fuel, may face significant challenges, as importation of goods would be hindered without a properly functioning transportation sector. The interconnected nature of the generation and distribution of renewable energy underscores the importance of preserving satellite communication systems to ensure a stable and sustainable global energy landscape.
2.3.2 Coal
Coal is the dominant resource for electricity generation, with approximately 35.8% of global coal usage dedicated to energy production Statista Research Department (2023d). In 2022, worldwide coal power generation reached approximately 10,191 TWh Statista Research Department (2023a). The coal industry is highly dependent on satellite technology, covering various stages from mining Xie et al. (2018) to coal processing for fuel and gas production. The modern equipment utilized in these processes benefits from the support of satellite technology. In particular, China and India are the largest exporters of coal. Any disruption in transportation means for exporting coal would significantly impact countries that rely on coal for electricity generation. The interconnectedness of the coal industry with satellite communication highlights the critical role that this technology plays in the smooth functioning of coal-related operations and its importance in supporting electricity generation worldwide.
Further exploration of the potential implications of minimal to no satellite communication on the coal industry and the subsequent impact on electricity generation will be elaborated upon in subsequent sections of this article. Safeguarding satellite communication systems becomes imperative to ensure the continued stability and reliability of coal-related processes and power generation in an increasingly energy-dependent global landscape.
2.3.3 Nuclear reactors, natural gas and liquid fuels
The combined production of energy from nuclear reactors, natural gas and liquid fuels represents a significant portion of total energy, approximately 33.8% Statista Research Department (2023d). Nuclear reactors heavily rely on computer systems to monitor crucial parameters, such as pressure levels, especially in the event of a failure. These monitoring systems depend on satellite communication systems to process and store critical data. Given the potential risks associated with nuclear reactors, including scenarios such as coolant loss and water supply failure Nuclear Energy Agency (2020), effective communication systems are essential for timely response and mitigation efforts in nuclear emergencies. The absence of reliable satellite communication could pose challenges in responding to such emergencies, and in severe cases, it could necessitate permanent reactor shutdowns to prevent meltdowns.
Currently, the world has over 400 nuclear reactors Statista Research Department (2023b), making their safety and efficient operation paramount to global energy stability. In addition to nuclear power, natural gas and liquid fuels are crucial energy resources, with numerous countries relying on their import for electricity generation and various other applications. These resources also depend on satellite communication systems for tasks such as mining, efficient operations, and transportation. Any disruption in satellite communication would hinder the prompt and smooth transportation of these gases and fuels, potentially leading to electrical generation constraints in various regions. The interdependence of nuclear reactors, natural gas, and liquid fuels with satellite communication systems underscores the critical role that this technology plays in ensuring reliable energy production and supply.
2.3.3.1 Ensuring nuclear reactor Safety and preparedness
The possibility of a nuclear meltdown due to the minimization or loss of satellite function is an exceptionally rare occurrence. It is vital to emphasize that the nuclear power industry is committed to ensuring the utmost safety and adheres to strict disaster prevention measures. Although we are considering potential risks in this article, it is equally essential to acknowledge the continuous efforts made by the nuclear industry to improve safety and prevent catastrophic events.
In the case of a nuclear meltdown, where water supply and essential systems might be affected by a scenario such as Kessler’s Syndrome, nuclear power plants are equipped with 30-day emergency water supplies known as Ultimate Heat Sinks (UHS). These UHS play a critical role in cooling the reactor, even after it has been turned off Union of Concerned Scientists (2010). Although the absence of electricity and communication could make implementing these emergency measures challenging, it is essential to remember that the occurrence of Kessler’s Syndrome is an unlikely event with a low probability of impacting multiple nuclear reactors simultaneously.
If, hypothetically, multiple reactors collapse in a short time frame, the release of radiation into the environment could potentially affect a significant portion of the Earth’s surface Lelieveld et al. (2012). However, it is crucial to reiterate that such a scenario is extremely improbable and is based on multiple simultaneous nuclear accidents.
In the event of an emergency, nuclear reactors have two systems in place: active and passive Xing et al. (2016). While the active system requires electricity to operate, the passive system operates independently, providing a safety measure even in situations of power loss. The passive system would eventually shut down within a specified time frame, typically between 72 h and 10 days, depending on the facility Giges (2014). As a last resort, submerging nuclear rods in seawater might be considered to cool the reactor. However, such a measure is expensive and would render the nuclear facility temporarily unusable, requiring significant financial investment to rebuild World Nuclear Association (2022).
To reiterate, the risk of a global nuclear meltdown simultaneously affecting multiple reactors is remarkably low due to the stringent safety protocols and disaster prevention measures upheld by the nuclear power industry. This section has been included to raise awareness of potential risks and to underscore the industry’s ongoing commitment to safety and the pursuit of alternative forms of energy that could be less hazardous in the event of rare occurrences.
2.3.4 Other utilities
The essential services of water, gas and waste disposal are vital for the maintenance of human life and the maintenance of a healthy living environment American Geosciences Institute (2016), U.S. Energy Information Administration (2023a) and The World Counts (2022). Water is a fundamental necessity for every living being on Earth, while gas serves crucial functions in cooking, heating, and various other activities. Water and gas are generally delivered through pipelines to homes, businesses, and industries, while in remote areas, gas can be supplied using containers transported by vehicles American Geosciences Institute (2016) and U.S. Energy Information Administration (2023a). Delivery systems often rely on electric pumps, which, in turn, require electricity to operate efficiently United States Space Force (2022b).
Some regions also utilize GNSS time to optimize water and gas distribution to ensure equitable access United States Space Force (2022b). However, in the absence of electricity and a reliable satellite communication system, the supply of water and gas to many households would be severely disrupted. Global statistics indicate that approximately 71% of the population has access to clean water, while approximately 60% has access to clean fuel for cooking Ritchie and Roser (2019) and Ritchie et al. (2022). The loss of supply would significantly affect people’s daily lives and wellbeing.
The waste disposal industry is highly dependent on the transportation sector to collect waste from homes and industries and transfer it to waste processing sites. Electricity is essential for waste processing, and in 2022 alone more than 1 billion tons of waste were collected worldwide The World Counts (2022). Without adequate transportation facilities, many households would face difficulties disposing of waste, leading to potential health hazards and environmental concerns Ann (2023). The interruption of these critical services due to the loss of satellite communication systems and electricity highlights the need to protect these technological infrastructures to ensure the smooth functioning of essential utilities.
2.4 Importance of other types of satellites
Aside from navigation satellites, there are several other prominent types of satellites, such as communication satellites, Earth observation satellites, and astronomical satellites Sergieieva (2023). Communication satellites aid in long-distance communication by filling the void left by traditional ground-based networks, which primarily support short-distance communication. These communication satellites act as conduits for a variety of media transmissions, including radio, television, phone, and internet services.
Earth observation satellites, on the other hand, are divided into two categories: weather satellites and remote sensing satellites. Weather satellites play an important role in a variety of industries, including agriculture, fisheries, and transportation, by predicting and mitigating the effects of adverse weather conditions. Remote sensing satellites Emery and Camps (2017), in turn, make significant contributions to resource exploration and monitoring of phenomena such as floods, droughts, soil moisture, wildfires, vegetation health, forest degradation, road infrastructure surveillance, etc.,. Remote sensing satellites are used in the military to capture high-resolution images of strategic locations, monitor enemy activities, and assess potential threats.
Astronomical satellites serve a unique purpose in that they aid in the identification of potential threats to Earth, such as asteroid impacts and solar storms.
3 Impact on healthcare
Modern medicine is heavily dependent on satellite communication for efficient storage and retrieval of patient data, electricity to power medical devices, and transportation for patient and drug mobility. The global burden of disease is significant, with more than 95% of the population affected by various diseases and many individuals experiencing multiple chronic or acute conditions. In Australia, low back pain and depression are prevalent health problems with a substantial impact on individuals Vos (2015).
In particular, several major health challenges continue to pose significant threats to global wellbeing. The worldwide prevalence of diabetes is over 500 million people International Diabetes Federation (2021), with approximately 19.3 million people facing cancer Sung (2021) and about 38.4 million living with HIV HIV.gov (2022). Managing these conditions requires meticulous care, and in 2017, more than 50% of patients use health apps to help with their medical management Stewart (2017). Furthermore, an estimated 310 million people undergo surgery annually International Surgical Outcomes Study group (2016). Many other health issues, not mentioned here, can be fatal if not properly treated in a timely manner.
The efficient functioning of medical facilities worldwide requires reliable access to electricity and transportation facilities. Without these essential resources, it would be impossible to provide adequate medical treatment to patients, leading to potentially millions or billions of deaths.
4 Impact on humans and other living beings
The rapid advancement of technology has significantly enriched human life, fostering learning and growth while driving progress and prosperity. In recent decades, technological innovations have brought about transformative changes in how our minds and bodies adapt to the modern world Clark (2007). In particular during the COVID-19 lockdown, people turned to technology for entertainment and information, leading to increased dependence on technological means Verbanas (2021).
In the United States alone, approximately 30% of adults are active online, highlighting the widespread influence of technology on modern society Verbanas (2021). While technology has facilitated various aspects of life, concerns arise regarding the potential addiction to technology, warranting a critical examination of its impact.
It is critical to recognize that the Kessler’s Syndrome has primarily indirect consequences due to modern society’s intricate interdependence. As a result, it is critical to emphasize the potential environmental consequences, including impacts on mankind, if Kessler’s Syndrome event occurs, resulting in partial or complete loss of satellite access. This analysis must also consider how human interventions, or their absence, may alter the environmental landscape and circumstances.
As this article progresses, we will explore the implications of minimal to no satellite function on human lifestyle, delving into how the loss of satellite communication systems could gradually or rapidly affect global affairs.
4.1 Impact on individuals
The impact of minimizing satellite functions on the digital communication system is a critical concern that demands attention. While the exact timeline of the consequences is uncertain, gradual restrictions on communication services can escalate to the point where effective communication becomes impossible. Such restrictions could trigger panic among people as they experience the effects of reduced satellite communication.
Reduction in banking services due to the diminishing capabilities of satellites may exacerbate panic, leading people to rush to stores to buy essential items and stock up with cash, displaying anxiety and panic buying behaviors Leung et al. (2021). The situation could escalate further if complete loss of satellite communication occurs on a global scale. Governments would also face challenges in carrying out special operations effectively without adequate satellite support and might struggle to collaborate with allies to find solutions. Shortfalls in government services and aid could create uncertainty and panic among the population.
With limited resources, essential needs such as food, water, and shelter could become scarce, leading to increased crime and unsafe living conditions. The heavy reliance of the modern world on technology could exacerbate mental health concerns, as a significant number of people experience nomophobia and Internet addiction Humood et al. (2021) and Longstreet and Brooks (2017). The occurrence of mental disorders could increase, given the substantial prevalence of such conditions worldwide World Health Organization (WHO) (2022).
Basic needs, such as water, food, and shelter, could face significant challenges due to the impacts of minimizing satellite functions. The lack or minimized availability of electricity could also be catastrophic, as demonstrated by studies suggesting that a large proportion of people are not prepared with emergency supplies Bedard (2019) and Centers for Disease Control and Prevention (2012). Inadequate healthcare services due to the loss of satellite-dependent technology could lead to a significant increase in fatalities related to poor healthcare quality Kruk (2018).
The exact extent and duration of these effects depend on the speed of loss of satellite communication systems and the potential adoption of alternative systems. It is crucial for policymakers and relevant stakeholders to recognize these implications and work towards safeguarding satellite communication to mitigate potential negative outcomes for humanity’s wellbeing and progress.
4.2 Biodiversity and ecological imbalance
Kessler’s Syndrome, at its extremes, poses a potential threat to humanity and can lead to ecological imbalances in the global ecosystem Weisman (2007). The continuous existence of numerous plant and animal species on Earth is intricately connected to human activities. Human interventions, such as the domestication and genetic modification of various plant species to serve our needs, have shaped the current biodiversity Daru et al. (2021).
However, in the event of severe disruptions caused by Kessler’s Syndrome, certain plant species that rely heavily on artificial herbicides and fertilizers for their survival could face rapid extinction without the assistance of humans in delivering those to plants. The sudden removal of insecticides, which are commonly used in agricultural practices, could also trigger a surge in population among insect species. Consequently, this population increase may lead to a corresponding increase in the numbers of insects that eat in the ecosystem, including birds, rodents, reptiles, and others, causing a cascade effect throughout the food chain.
The potential consequences of these disruptions on biodiversity and ecological balance are concerning. The loss of certain plant species could have far-reaching impacts on the organisms dependent on them for food and habitat. Furthermore, changes in insect populations could alter the dynamics of predator-prey relationships, leading to unforeseen changes in the composition of ecosystems UF Thompson Earth Systems Institute (2020).
5 Preventive measures for a sustainable future
As explored earlier in this article, the possibility that Kessler’s Syndrome will occur within the next few decades is a matter of concern. While it may seem improbable, the potential consequences demand our attention, prompting us to consider the scenarios that could unfold if preventive measures are not taken. This section aims to shed light on the likely outcomes if we lack viable solutions to mitigate space debris and prevent Kessler’s Syndrome.
Drawing insights from “The Beginner’s Guide to Nation-Building,” a 2007 book Dobbins et al. (2007), a hypothetical concept was introduced to estimate the cost of rebuilding a nation from scratch. Conceptual estimation suggested that rebuilding a nation with a population of 5 million and a per capita GDP of $500 would require $15,000 million. However, it is crucial to acknowledge that this estimate was made in 2007, and such figures are subject to change over the subsequent decades. Furthermore, the idea of rebuilding the entire world from scratch appears daunting and nearly insurmountable given the rapid progress in technology and societal advancements.
5.1 Policymakers
As the population of space debris grows, it becomes clear that properly addressing this global concern requires a united effort from all nations. Similarly to the global agreement reached on addressing climate change, governments must put aside their differences and work together to find effective solutions to protect our celestial environment Maizland (2022). It is critical for states to choose experts capable of functioning as unbiased facilitators in international negotiations in order to ensure impartial decision-making and prioritize the protection of lives in our planet and its space assets.
The allocation of specialized funds for space conservation projects is a critical step in this collaborative effort. These financial commitments should be used to develop space debris mitigation techniques and proactive efforts to avoid the uncontrolled spread of space debris. The formation of a specialized council dedicated to space protection, comprised of representatives from various states, can act as a driving force in the development of international regulations that control space activity. Once these regulations are in place, rigorous enforcement procedures should be put in place that are globally applicable to all space agencies, regardless of national origin or affiliation. Strict sanctions for violators will establish responsibility and serve as a deterrent.
The Inter-Agency Space Debris Coordination Committee (IADC) now serves as a policy-making organization aiming to prevent Kessler’s Syndrome. The role and actions of the IADC must be strengthened in order to create worldwide cooperation and collaboration in the reduction of space debris. Nations can actively collaborate with the IADC and other relevant international bodies to adopt more stringent legislation and best practices for space protection. Furthermore, the United Nations (UN) United Nation (UA) (2010) and the 2018 White House Presidential Memorandum The White House (2018) set critical guidelines for space organizations to follow to promote peaceful operation in space and mitigate the problem of rising space debris. These principles include measures such as responsible spacecraft reentry following missions and avoiding space weapons testing, such as Anti-Satellite tests, to mitigate the growing population of space debris.
International cooperation is critical to guaranteeing the long-term viability of space activities and the preservation of our future in space. Countries may establish a harmonious and secure environment for space exploration by transcending geopolitical boundaries and taking the role of responsible global citizens. Only through joint dedication and teamwork will we be able to protect the enormous benefits and opportunities that space provides humanity while also limiting the considerable threats posed by space debris and the approaching threat of Kessler’s Syndrome.
5.2 Mitigation techniques
In addition to policy measures, the implementation of mitigation techniques is crucial to address the current space debris orbiting Earth. These techniques can be categorized into the following.
5.2.1 Short-term solution
In an effort to address the immediate threat posed by space debris, short-term solutions should prioritize the removal of hazardous debris. Various research efforts have proposed the deorbiting of debris into the Earth’s atmosphere, allowing them to burn upon reentry. Although this approach shows promise as a viable mitigation technique in the short term, it cannot be considered a long-term sustainable solution. The widespread use of lightweight aluminum in spacecraft construction poses a significant concern in this context Mariappan et al. (2020). During reentry, aluminum is converted to aluminum oxide, known as alumina, which could accidently trigger an accidental geoengineering experiment, potentially altering regional climate patterns. Additionally, the presence of aluminum oxide in the atmosphere may have detrimental effects on the ozone layer, possibly causing further depletion Delbert (2021). Given the complexities and limitations of de-orbiting techniques, it is evident that a comprehensive long-term solution is imperative to effectively address the challenge of space debris.
5.2.2 Long-term solution
The recycling of space debris emerges as a promising long-term sustainable solution to the mitigation of space debris. A conceptual approach proposed by Mariappan et al. (Mariappan et al,. 2019; Mariappan et al., 2020) suggests the recycling of space debris into functional powder by atomizing water. These powders can serve as valuable resources to produce fuel and artificial soil, facilitating the cultivation of plants in space McDowell (2020). By repurposing decommissioned satellites and debris into useful materials to support other space missions, this recycling strategy presents a win-win scenario, promoting environmental sustainability and resource efficiency in space exploration.
6 Concluding remarks
The scenario of Kessler’s Syndrome, while mostly fictional at this time, represents a potential real threat to humanity if space debris accumulates and disrupts satellite systems in a short time frame. Despite the challenges in accurately predicting the occurrence of such an event, it is essential to consider the potential consequences and the lack of viable solutions at the time. The loss of satellite communication would have far-reaching impacts on various industries, including transportation, banking, energy, and military operations. Furthermore, disruption of the ecological balance, with humans at the top of the food chain, poses serious concerns for our survival. Although humans can attempt to adapt mentally, sudden environmental changes would prove challenging for our physical wellbeing. Therefore, a global effort, similar to the global warming agreement, is necessary to mitigate space debris.
Two categories of solutions emerge: short-term and long-term. In the short term, emphasis is placed on deorbiting and burning debris posing immediate threats. Although this technique may address immediate risks, it is not a long-term sustainable solution. The burning of debris could have unintended consequences, such as depletion of the ozone layer, potentially triggering new threats to humanity and the environment. As such, a long-term approach, focusing on recycling, is crucial. Researchers have proposed innovative methods to recycle space debris into useful powders that serve as fuel, artificial soil, and other resources for space missions. By reusing materials from decommissioned satellites, we can achieve cost-effective and sustainable solutions, avoiding further harm to our environment.
To safeguard humanity’s future and prevent the potentially catastrophic space-debris apocalypse, the launch of the first space-debris mitigation mission should be a priority. Collaboration between countries and the implementation of effective recycling techniques are vital steps toward ensuring the long-term sustainability and safety of our activities in space. Proactive measures today will determine the course of our future in space exploration and will protect the delicate balance of life on Earth.
Data availability statement
The original contributions presented in the study are included in the article/Supplementary Material, further inquiries can be directed to the corresponding author.
Author contributions
AM: Writing–original draft, Conceptualization, Data curation, Formal Analysis, Investigation, Methodology, Software. JC: Project administration, Supervision, Writing–review and editing.
Funding
The author(s) declare that no financial support was received for the research, authorship, and/or publication of this article.
Acknowledgments
The first author would like to thank the management of the University at Buffalo, Buffalo, New York, 14260 for their extensive support of this research work.
Conflict of interest
The authors declare that the research was conducted in the absence of any commercial or financial relationships that could be construed as a potential conflict of interest.
Publisher’s note
All claims expressed in this article are solely those of the authors and do not necessarily represent those of their affiliated organizations, or those of the publisher, the editors and the reviewers. Any product that may be evaluated in this article, or claim that may be made by its manufacturer, is not guaranteed or endorsed by the publisher.
References
Alsop, T. (2022). GNSS device installed base worldwide 2019-2031 (Statista). https://www.statista.com/statistics/1174544/gnss-device-installed-base-worldwide.
American Geosciences Institute (2016). How is water distributed? https://www.americangeosciences.org/education/k5geosource/content/water/how-is-water-distributed.
Anz-Meador, P. (2020). U.S. National space council announces update to the USG ODMSP. JSC-E-DAA-TN77633 (national aeronautics and space administration). https://sma.nasa.gov/news/articles/newsitem/2019/12/09/national-space-council-announces-update-to-usg-odmsp.
Bastida Virgili, B., Dolado, J., Lewis, H., Radtke, J., Krag, H., Revelin, B., et al. (2016). Risk to space sustainability from large constellations of satellites. Acta Astronaut. 126, 154–162. doi:10.1016/j.actaastro.2016.03.034
Bedard, P. (2019). New emp warning: us will ‘cease to exist,’ 90 percent of population will die. Washington, D.C., USA: Washington Examiner.
Bureau of Transportation Statistics, (2017). National household travel survey daily travel quick facts. Washington, D.C., USA: Bureau of Transportation Statistics.
Bureau of Transportation Statistics, (2022). Highway travel - all systems. Washington, D.C., USA: Bureau of Transportation Statistics.
Centers for Disease Control and Prevention (2012). Startling facts you should know about disaster preparedness. Atlanta, Georgia, United States: CDC.
Clark, G. (2007). A farewell to alms: a brief economic history of the world. Princeton, New Jersey, United States: Princeton University Press.
Coast Guard - Office of Navigation Systems and Code of Federal Regulations, (2019). International and U.S. Inland navigation rules (national oceanic and atmospheric administration). https://www.nauticalcharts.noaa.gov/publications/coast-pilot/docs/NavigationRulesStandardSize.pdf.
Colvin, T. J., Karcz, J., and Wusk, G. (2023). Cost and benefit analysis of orbital debris remediation (national aeronautics and space administration). https://www.nasa.gov/wp-content/uploads/2023/03/otps_-_cost_and_benefit_analysis_of_orbital_debris_remediation_-_final.pdf.
Coombs, M., and Braithwaite, C. (2021). Satellite technology enhances dam safety monitoring. Int. J. Hydropower and DAMS 28. https://www.hydropower-dams.com/articles/satellite-technology-enhances-dam-safety-monitoring.
Cowardin, H. (2022). Orbital debris quartely news. Intentional Destr. Cosmos 1408 26. https://orbitaldebris.jsc.nasa.gov/quarterly-news/pdfs/odqnv26i1.pdf.
Dalen, J. E., and Alpert, J. S. (2019). Medical tourists: incoming and outgoing. Am. J. Med. 132, 9–10. doi:10.1016/j.amjmed.2018.06.022
Daru, B. H., Davies, T. J., Willis, C. G., Meineke, E. K., Ronk, A., Zobel, M., et al. (2021). Widespread homogenization of plant communities in the anthropocene. Nat. Commun. 12, 6983. doi:10.1038/s41467-021-27186-8
Delbert, C. (2021). All the satellites in space could crack open the ozone layer. New York City, NY, USA: Popular Mechanics.
Dobbins, J., Crane, K., DeGrasse, B. C., and Jones, S. G. (2007). The Beginner’s Guide to nation-building. Santa Monica, California, USA: RAND National Security Research Division.
Emery, W., and Camps, A. (2017). Introduction to satellite remote sensing. Amsterdam, Netherlands: Elsevier.
European Space Agency (2022). Iris system to digitalise airspace goes global. Paris, France: European Space Agency.
European Space Agency (ESA) (2023). Space environment statistics. Paris, France: European Space Agency.
Federal Aviation Administration (FAA) (2022). Navigation aids. Washington, D.C., United States: Federal Aviation Administration.
Garcia, M. (2021). Space debris and human spacecraft. https://brewminate.com/space-debris-and-human-spacecraft/.
Giges, N. S. (2014). Time for passive safety at nuclear plants. Time Passive Saf. A. T. Nucl. Plants - ASME. https://www.asme.org/topics-resources/content/time-passive-safety-nuclear-plants.
Heasler, H. P., Jaworowski, C., and Foley, D. (2009). Geothermal systems and monitoring hydrothermal features. Geol. Monit., doi:10.1130/2009.monitoring(05)
Hiv.gov, (2022). Hiv and aids epidemic global statistics. https://www.hiv.gov/hiv-basics/overview/data-and-trends/global-statistics/.
Hollingham, R. (2013). What would happen if all satellites stopped working? Future. https://www.bbc.com/future/article/20130609-the-day-without-satellites.
House, , and Senate, (2015). Protecting the electric grid from the potential threats of solar storms and electromagnetic pulse. Scotts Valley, California, USA: CreateSpace.
Hughes, C. (2020). Rail passenger traffic - asia oceania and the Middle East 2019 (Statista). https://www.statista.com/statistics/261172/rail-passenger-activity-in-asia-oceania-and-the-middle-east.
Humood, A., Altooq, N., Altamimi, A., Almoosawi, H., Alzafiri, M., Bragazzi, N. L., et al. (2021). The prevalence of nomophobia by population and by research tool: a systematic review, meta-analysis, and meta-regression. Psych 3, 249–258. doi:10.3390/psych3020019
International Air Transport Association (2022). Value of air cargo. Montreal, Canada: International Air Transport Association.
International Diabetes Federation (2021). Diabetes facts and figures. Montreal, Canada: International Air Transport Association.
International Surgical Outcomes Study group (2016). Global patient outcomes after elective surgery: prospective cohort study in 27 low-, middle- and high-income countries. Br. J. Anaesth. 117 (5), 601–609. Erratum in: Br J Anaesth. 2017 Sep 1;119 (3), 553. doi:10.1093/bja/aew316
Jenn, D. C. (2019). Radar and laser cross section engineering. Sunrise Valley Dr, Virginia, USA: American Institute of Aeronautics and Astronautics.
Katz, R. W., and Murphy, A. H. (1997). Economic value of weather and climate forecasts. Cambridge University Press.
Kessler, D. J., and Cour-Palais, B. G. (1978). Collision frequency of artificial satellites: the creation of a debris belt. J. Geophys. Res. 83, 2637–2646. doi:10.1029/ja083ia06p02637
Kruk, , Gage, A. D., Arsenault, C., Jordan, K., Leslie, H. H., Roder-DeWan, S., et al. (2018). High-quality health systems in the sustainable development goals era: time for a revolution. Lancet Glob. Health 6, e1196–e1252. doi:10.1016/s2214-109x(18)30386-3
Lelieveld, J., Kunkel, D., and Lawrence, M. G. (2012). Global risk of radioactive fallout after major nuclear reactor accidents. Atmos. Chem. Phys. 12, 4245–4258. doi:10.5194/acp-12-4245-2012
Leung, J., Chung, J. Y. C., Tisdale, C., Chiu, V., Lim, C. C. W., and Chan, G. (2021). Anxiety and panic buying behaviour during covid-19 pandemic—a qualitative analysis of toilet paper hoarding contents on twitter. Int. J. Environ. Res. Public Health 18, 1127. doi:10.3390/ijerph18031127
Lewis, H. G. (2020). Understanding long-term orbital debris population dynamics. J. Space Saf. Eng. 7, 164–170. doi:10.1016/j.jsse.2020.06.006
Liou, J.-C., and Johnson, N. L. (2006). Risks in space from orbiting debris. Science 311, 340–341. doi:10.1126/science.1121337
Longstreet, P., and Brooks, S. (2017). Life satisfaction: a key to managing internet and social media addiction. Technol. Soc. 50, 73–77. doi:10.1016/j.techsoc.2017.05.003
Maizland, L. (2022). Global climate agreements: successes and failures. New York, NY, United States: Council on Foreign Relations.
Mariappan, A., Kumar, V. R. S., Weddell, S. J., Muruganandan, V. A., and Jeung, I. (2020). Theoretical studies on space debris recycling and energy conversion system in the international space station. Eng. Rep. 3. doi:10.1002/eng2.12317
Mariappan, A., Sanal Kumar, V., Anand, V., Weddell, S., and Jeung, I.-S. (2019). A conceptual method to recycle space debris into fuels and artificial soil in the iss for numerous applications. AIAA Propuls. Energy 2019 doi:10.2514/6.2019-4157
McDowell, J. C. (2020). The low earth orbit satellite population and impacts of the spacex starlink constellation. Astrophysical J. 892, L36. doi:10.3847/2041-8213/ab8016
Molayath, A., and Sanal Kumar, V. (2010). “Studies on space debris tracking and elimination,” in 46th AIAA/ASME/SAE/ASEE Joint Propulsion Conference and amp; Exhibit, Nashville, TN, USA, July, 2010. doi:10.2514/6.2010-7008
Morgan, C. (2020). How utility companies are using satellite technology - x2nsat. https://x2n.com/blog/how-utility-companies-are-using-satellite-technology/.
Munoz-Organero, M., Ruiz-Blaquez, R., and Sánchez-Fernández, L. (2018). Automatic detection of traffic lights, street crossings and urban roundabouts combining outlier detection and deep learning classification techniques based on gps traces while driving. Comput. Environ. Urban Syst. 68, 1–8. doi:10.1016/j.compenvurbsys.2017.09.005
National Research Council (2011). Limiting future collision risk to spacecraft: an assessment of NASA’s meteoroid and orbital debris programs. Washington, D.C., United States: The National Academies Press. doi:10.17226/13244
Norton, K., and Omberg, A. (1947). The maximum range of a radar set. Proc. IRE 35, 4–24. doi:10.1109/jrproc.1947.231216
Nuclear Energy Agency (2020). Nuclear fuel behaviour in loss-of-coolant accident (LOCA) conditions. Paris, France: Organisation for Economic Co-operation and Development OECD.
Petrosyan, A. (2023). Number of internet and social media users worldwide as of April 2023 (Statista). https://www.statista.com/statistics/617136/digital-population-worldwide/.
Pistoia, G. (2009). Battery operated devices and systems. Amsterdam, Netherlands: Elsevier. doi:10.1016/b978-0-444-53214-5.x0001-5
Placek, M. (2022). United States - international trade tonnage by transportation mode 2021 (Statista). https://www.statista.com/statistics/1341612/us-international-trade-tonnage-by-transportation-mode-direction/.
Ritchie, H. (2021). The number of people without electricity more than halved over the last 20 years. https://ourworldindata.org/number-without-electricity.
Ritchie, H., and Roser, M. (2019). Clean water and sanitation. Our world in data. https://ourworldindata.org/clean-water-sanitation.
Ritchie, H., Roser, M., and Rosado, P. (2022). Access to energy. Our world in data. https://ourworldindata.org/energy-access.
Salas, E. B. (2022a). Air transportation. Statista. https://www.statista.com/topics/1707/air-transportation/.
Salas, E. B. (2022b). Rail industry worldwide. Statista. https://www.statista.com/topics/1088/rail-industry/.
Sample, I. (2016). Rise in space junk could provoke armed conflict, say scientists. Hindu. https://www.thehindu.com/sci-tech/science/Rise-in-space-junk-could-provoke-armed-conflict-say-scientists/article14017691.ece.
Sergieieva, K. (2023). Types of satellites: different orbits and real-world uses. https://eos.com/blog/types-of-satellites/.
Spitzer, C. R. (2018). Avionics: elements, software and functions. Boca Raton, Florida, United States: CRC Press. doi:10.1201/9781315222240
Statista Research Department (2021a). World electricity generation by energy source 2050 (Statista). https://www.statista.com/statistics/859178/projected-world-electricity-generation-capacity-by-energy-source.
Statista Research Department (2015). Agricultural land: percentage of total land area, 2012 (Statista). https://www.statista.com/statistics/268762/agricultural-land-as-a-percentage-of-total-world-land-area-since-1990/.
Statista Research Department (2021b). China: Number of Employees in railway Transport by region (Statista). https://www.statista.com/statistics/278355/employees-in-railway-transport-in-china-by-region.
Statista Research Department (2021c). Road freight activity forecast 2050 (Statista). https://www.statista.com/statistics/1246737/forecast-road-freight-activity.
Statista Research Department (2022a). Airline industry - passenger traffic worldwide 2004-2022 (Statista). https://www.statista.com/statistics/564717/airline-industry-passenger-traffic-globally/.
Statista Research Department (2022b). Rail freight transportation in U.S. 2021 (Statista). https://www.statista.com/statistics/255558/value-added-of-the-us-rail-transportation-industry.
Statista Research Department (2022c). U.S. Class I railroads: number of employees 2014-2020 (Statista). https://www.statista.com/statistics/562887/us-class-one-railroads-number-of-employees.
Statista Research Department (2022d). World electricity consumption 2021 (Statista). https://www.statista.com/statistics/383633/worldwide-consumption-of-electricity-by-country.
Statista Research Department (2023a). Global coal power generation 2022 (Statista). https://www.statista.com/statistics/1082201/coal-fired-electricity-generation-globally.
Statista Research Department (2023b). Nuclear power plants in the world 2023 (Statista). https://www.statista.com/statistics/267158/number-of-nuclear-reactors-in-operation-by-country/.
Statista Research Department (2023c). Transportation volume in China. Statista. https://www.statista.com/topics/5816/transportation-volume-in-china.
Statista Research Department (2023d). World electricity generation shares by source 2022 (Statista). https://www.statista.com/statistics/269811/world-electricity-production-by-energy-source.
Stewart, C. (2017). Health apps usage number amongst familiar patients 2017 (Statista). https://www.statista.com/statistics/809394/health-apps-number-usage-share-by-known-patients/.
Stössel, M. (2015). Brief introduction to flight management systems. http://www.a3xxflightdeck.com/images/PDF/Handout_FMS_AT.pdf.
Sun, S. (2023). India: rail freight volume 2022 (Statista). https://www.statista.com/statistics/741325/india-rail-freight-volume.
Sung, , Ferlay, J., Siegel, R. L., Laversanne, M., Soerjomataram, I., Jemal, A., et al. (2021). Global cancer statistics 2020: globocan estimates of incidence and mortality worldwide for 36 cancers in 185 countries. CA A Cancer J. Clin. 71, 209–249. doi:10.3322/caac.21660
Tao, H., Che, X., Zhu, Q., and Li, X. (2022). Satellite in-orbit secondary collision risk assessment. Int. J. Aerosp. Eng. 2022, 1–18. doi:10.1155/2022/6358188
Teh, L. C. L., and Sumaila, U. R. (2011). Contribution of marine fisheries to worldwide employment. Fish Fish. 14, 77–88. doi:10.1111/j.1467-2979.2011.00450.x
Tetreault, B. (2005). Use of the automatic identification system (ais) for maritime domain awareness (mda). Proc. OCEANS 2005 MTS/IEEE 2, 1590–1594. doi:10.1109/oceans.2005.1639983
The Economic Times (ET) (2017). 10 facts we bet you didn’t know about indian railways - know your railways. Mumbai, India: The Economic Times.
The White House, (2018). Space policy directive-3, national space traffic management policy Washington, DC, United States: The White House.
The World Counts, (2022). The world counts. https://www.theworldcounts.com/.
The Aerospace Corporation (2021). Brief history of gps. El Segundo, California, United States: Aerospace Corporation.
The Aerospace Corporation (2023). Space debris 101. El Segundo, California, United States: Aerospace Corporation.
UF Thompson Earth Systems Institute (2020). The insect effect: insect decline and the future of our planet. Gainesville, FL, USA: Thompson Earth Systems Institute.
Union of Concerned Scientists, (2010). How it works: water for nuclear. Cambridge, Massachusetts, United States: Union of Concerned Scientists.
United Nation (UN) (2010). Space debris mitigation guidelines of the committee on the peaceful uses of outer space. https://www.unoosa.org/pdf/publications/st_space_49E.pdf.
United States Space Force (2019). Loran-c infrastructure and e-loran. Virginia, USA: United States Space Force.
United States Space Force (2021c). Agricultural applications. Virginia, USA: United States Space Force.
United States Space Force (2021d). Other global navigation satellite systems (gnss). Virginia, USA: United States Space Force.
United States Space Force (2021e). Road and highway applications. Virginia, USA: United States Space Force.
U.S. Energy Information Administration (2023a). Delivery and storage of natural gas. Washington, D.C. United States: U.S. Energy Information Administration.
U.S. Energy Information Administration (2022). Biomass explained. Washington, D.C. United States: U.S. Energy Information Administration.
U.S. Energy Information Administration (2023b). Renewable energy explained. Washington, D.C. United States: U.S. Energy Information Administration.
Verbanas, P. (2021). Are you addicted to technology? New Brunswick, NJ, United States: Rutgers University.
Vos, , Barber, R. M., Bell, B., Bertozzi-Villa, A., Biryukov, S., Bolliger, I., et al. (2015). Global, regional, and national incidence, prevalence, and years lived with disability for 301 acute and chronic diseases and injuries in 188 countries, 1990–2013: a systematic analysis for the global burden of disease study 2013. Lancet 386, 743–800. doi:10.1016/s0140-6736(15)60692-4
Weeden, B., and Samson, V. (2018). Global counterspace capabilities: an open source assessment. UG1523. Washington, D.C., USA: Secure World Foundation.
Weisman, A. (2007). The world without us. Flatiron Building, New York, USA: Thomas Dunne Books. doi:10.1604/9780312347291
World Nuclear Association (2022). Nuclear power economics. London, United Kingdom: World Nuclear Association.
Xie, X., Xu, C., Wen, Y., and Li, W. (2018). Monitoring groundwater storage changes in the loess plateau using grace satellite gravity data, hydrological models and coal mining data. Remote Sens. 10, 605. doi:10.3390/rs10040605
Keywords: space debris, Kessler’s syndrome, space debris mitigation, satellite disruption, human impacts, ecological impacts, global effort, long-term sustainability
Citation: Mariappan A and Crassidis JL (2023) Kessler’s syndrome: a challenge to humanity. Front. Space Technol. 4:1309940. doi: 10.3389/frspt.2023.1309940
Received: 09 October 2023; Accepted: 14 November 2023;
Published: 28 November 2023.
Edited by:
Alberto Buzzoni, Astrophysics and Space Science Observatory of Bologna (INAF), ItalyReviewed by:
Dario Spiller, Sapienza University of Rome, ItalyAyodele Periola, Cape Peninsula University of Technology, South Africa
Copyright © 2023 Mariappan and Crassidis. This is an open-access article distributed under the terms of the Creative Commons Attribution License (CC BY). The use, distribution or reproduction in other forums is permitted, provided the original author(s) and the copyright owner(s) are credited and that the original publication in this journal is cited, in accordance with accepted academic practice. No use, distribution or reproduction is permitted which does not comply with these terms.
*Correspondence: Amrith Mariappan, YW1yaXRobWFAYnVmZmFsby5lZHU=