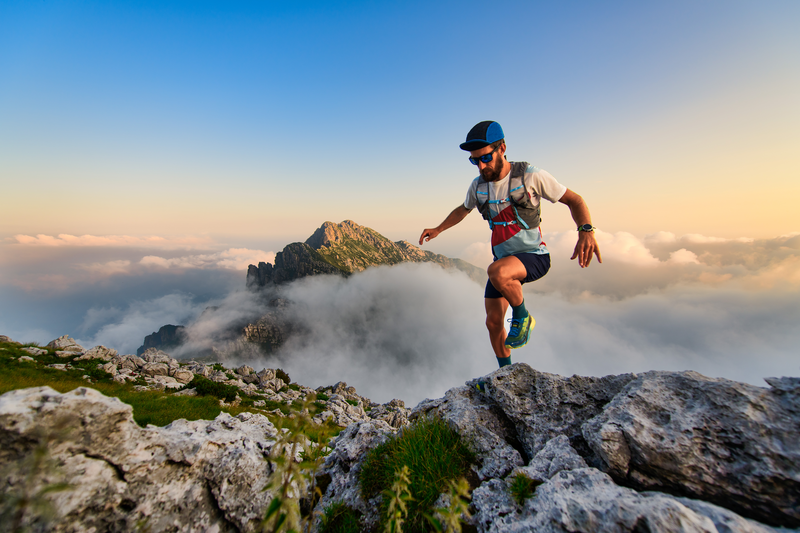
94% of researchers rate our articles as excellent or good
Learn more about the work of our research integrity team to safeguard the quality of each article we publish.
Find out more
ORIGINAL RESEARCH article
Front. Soft Matter , 22 June 2023
Sec. Polymers
Volume 3 - 2023 | https://doi.org/10.3389/frsfm.2023.1197868
This article is part of the Research Topic Dynamic and reconfigurable polymer networks View all 6 articles
Amines as additives in benzoxazines are known to beneficially affect the polymerization temperature and furthermore to allow for partially reversible reaction steps yielding however a non-dynamic polybenzoxazine network. This contribution proves that the polymerization behavior of a two-component polymer of the polyetheramine Jeffamine® ED-600 and a bisphenol-A-based benzoxazine features stress-relaxation and reprocessability usually known from vitrimers. With the aim to gain a deeper understanding of the material properties of this system and the corresponding polymer structure, the reaction mechanism of a monofunctional benzoxazine and monoamine model system was studied revealing at first primary, and then secondary amine induced opening of oxazine rings, leading at first to linear polymer chains and then to covalently crosslinked networks. Both consist of repeated phenolic benzoxazine/amine motifs with permanently incorporated polyetheramine chains that do not impact the mechanical properties, compared to pure polybenzoxazine. Thermal, spectroscopic, and extraction analyses show that the addition of Jeffamine® reduces the polymerization temperature and introduces material properties such as reprocessability at the same time. Stress-relaxation measurements support the assumption that the reprocessability point to vitrimer-like molecular processes. The material shows rapid stress-relaxation of up to 11 s, a corresponding bond-exchange activation energy of 146 kJ/mol, and a topology freezing temperature of 97°C.
Materials research and development in the field of thermosets encounters an almost inexhaustible potential for innovations and improvements in the chemistry of polybenzoxazines. Characterized by design flexibility at the molecular level and thus a broad spectrum of physical and chemical properties at the macroscopic level, benzoxazines form a class of thermosets whose properties outperform those of typical phenols and epoxides. (Ishida and Agag, 2011).
Despite having tremendous advantages in terms of chemical and mechanical stability, the biggest shortcoming of thermosets is their inability to be malleable after polymerization due to their three-dimensionally crosslinked molecular structure. This strongly limits the possibilities of reuse and recycling, especially when they are used in combination with reinforcing fibres. (Witik et al., 2013). To overcome these drawbacks, vitrimers open up new opportunities for the sustainable and smart use of thermosets. Vitrimers are associative covalent adaptive networks and form a new polymer class alongside thermosets and thermoplastics. (Montarnal et al., 2011; Fortman et al., 2018). Covalent adaptive networks are defined by reversible chemical bonds that allow a temporary or permanent change in the network structure through an appropriate stimulus, such as temperature. This reaction is macroscopically associated with a change in the stress-strain state in the plastic region of the material without loss of network integrity or density. (Kloxin et al., 2010). Hence, this ability allows thermosets to overcome their limitation and make them malleable, healable and, depending on the system, recyclable. (Luzuriaga et al., 2016).
Benzoxazines featuring vitrimeric properties pose a challenge due to the high thermal properties of benzoxazines and the temperature range for vitrimeric behavior between the glass transition temperature and the initial degradation of the polymer. (Anagwu et al., 2022). Up to now, covalent adaptive benzoxazine systems are based on boronic ester exchange reaction (Gulyuz et al., 2022; Wang et al., 2022), dioxaborolane metathesis (Bo et al., 2022), disulfide metathesis (Trejo-Machin et al., 2020), transcarbamoylation (Wen et al., 2022) and transesterification (Adjaoud et al., 2022).
A characteristic of vitrimers is their reprocessability, which can be based on the dynamic exchange reactions, but it is well known that such properties are not exclusive to vitrimers. (Arslan et al., 2018). However, the reprocessability is often presented in crushing- or cracking-reforming cycles, evaluated with mechanical tests. For true vitrimers, Wang et al., 2022 show that polybenzoxazines can reprocess via dynamic boronic ester bonds. In tensile tests, strengths at break decreased by up to 15% after three cycles. Ručigaj et al., 2020 developed a diphenolic acid-based benzoxazine. The reprocessability was determined by compact tensile tests. After a single cycle, specimens achieved 40%–50% of the fracture toughness of virgin specimens. Other approaches to develop polybenzoxazine-based reprocessable polymers are realized, for example, via a cardanol-based polybenzoxazine vitrimer (Trejo-Machin et al., 2020), with biobased poly (benzoxazine-co-urethane) (Sriharshita et al., 2020), with isosorbide-based polybenzoxazine (Adjoud et al., 2022), and by means of a catalyst-free trans-esterification in benzoxazines (Adjaoud et al., 2021). In contrast to the chemistry of the systems, the reprocessability of benzoxazine/amine polymers has not been studied, yet.
Polymerization of benzoxazines in presence of amines have been investigated by several groups in the last years, mainly with the aim to lower benzoxazines high polymerization temperatures. (Sun et al., 2015; Zong and Ran, 2019; Zhang et al., 2020). However, the resulting structures are reported to strongly depend on the chemical reactivity of the respective benzoxazine and amine. (Sun et al., 2015; Zong and Ran, 2019; Zhang et al., 2020). Mechanistical studies of benzoxazines with amines suggest the formation of several consecutive reaction steps with increasing reaction temperature that partially can be reversible. The total reaction is, however, reported to be irreversible and non-dynamic. (Sun et al., 2015). Furthermore, oligomeric amines such as the polyetheramine Jeffamine® are used for toughening aside the impact on polymerization temperature. (Yagci et al., 2009; Gonçalves et al., 2022; Gulyuz et al., 2022). The synthesis of a polyetheramine-based main chain polybenzoxazine was developed by Agag et al. (2010). Typically, the polyether diamine is incorporated into the network structure by using the amine as the amine component for the benzoxazine monomer synthesis. The resulting benzoxazine consists of linear oligomers which are liquid at room temperature and can be thermally crosslinked into flexible thermosets. Based on this pioneering work, further research has been conducted. Khan et al., 2018 investigated the gas permeability of main chain polybenzoxazines based on Jeffamines® of different molecular weights. Liu et al., 2016 reported a polyetheramine-bearing polybenzoxazine with thermally induced shape memory properties. Various responsive Jeffamine®-based polybenzoxazines have been reported. However, the responsive effects, such as self-healing, did not result from the presence of Jeffamine®, but from additional functional groups allowing for, e.g., light-induced oxoketene formation (Arslan et al., 2018), boronic ester exchange reactions (Gulyuz et al., 2022), photoinduced dimerization of coumarin (Kiskan and Yagci, 2014) or supramolecular hydrogen-bond formation (Ručigaj et al., 2020). Gonçalves et al. (2022) described a benzoxazine and cardanol-doped polyaniline coating for corrosion protection. In their work, Jeffamine® acts as catalytic curing agent and flexibilizer. Puozzo et al. (Puozzo et al., 2022) used the flexibilizing properties of high molecular weight Jeffamine® to prepare an elastomeric benzoxazine for pressure-sensitive applications. So far, a neat benzoxazine/polyetheramine copolymer has not yet been described to yield a covalent adaptive network.
Against this background, this contribution presents a benzoxazine/polyetheramine based polymer network resulting from bisphenol-A and aniline-based benzoxazine (BA-a) and Jeffamine® as a polyetheramine that exhibits vitrimeric properties. Stress-relaxation measurements and multiple reprocessability proved the dynamic character of the benzoxazine/amine copolymer. The impact of the polymerization parameters was studied on overall but in particular vitrimeric properties. With the aim to elucidate structure-property relation, monofunctional benzoxazine monomer with primary monoamine was studied as a model reaction. In a second step, polymer networks resulting from bifunctional benzoxazines and diamines were characterized in terms of crosslinking densities and the state of polyetheramine incorporation into the polybenzoxazine network. Insights regarding the molecular structure were then correlated to derive a reaction mechanism and the corresponding polymer structure of the vitrimer-like benzoxazine/amine network.
Bisphenol-A and aniline-based benzoxazine, Araldite® MT 35600 (BA-a) was provided by Huntsman Advanced Material (Huntsman International LLC, Texas, United States). para-cresol, aniline, Jeffamine® M-600 and Jeffamine® ED-600 were purchased from Merck KGaA (Darmstadt, Germany). Paraformaldehyde, Na2SO4, ethanol and butan-2-one were purchased from Carl Roth GmbH & Co. KG (Karlsruhe, Germany). Chloroform and NaOH were supplied from VWR International GmbH (Darmstadt, Germany). CDCl3 and DMSO-d6 were purchased from Deutero GmbH (Kastellaun, Germany). All chemicals were used without further purification. Casting moulds for the sample manufacturing were coated with the release agent ACMOScoat 82-9101 (ACMOS CHEMIE KG, Bremen, Germany).
pC-a was synthesized according to Takeichi et al. (Takeichi et al., 2004) para-cresol (25.0 g, 231 mmol), aniline (19.6 g, 210 mmol), and paraformaldehyde (13.9 g, 462 mmol) were placed in a round bottom flask under an atmosphere of N2, slowly heated to 100°C and kept at that temperature for 1 h. The resulting pale-brown liquid was cooled to room temperature, dissolved in 200 mL of chloroform, and washed three times with 150 mL of aqueous NaOH (1 mol L-1). The organic phase was dried over Na2SO4, and the solvent was removed under reduced pressure at 60°C. The resulting pale-brown oil that can solidify with time, was dissolved in 400 mL boiling ethanol, and stored for 24 h at −20°C for recrystallization. The resulting white crystals were melted at 75°C and dried under high vacuum for 30 min.
pC-a was obtained as a pale-yellow solid, 32.6 g (yield = 69%) 1H-NMR (600 MHz, DMSO-d6, 296 K): δ/ppm = 7.23 (t, J = 8.0 Hz, 2H, Har), 7.11 (d, J = 7.7 Hz, 2H, Har), 6.91 (s, 1H, Har), 6.88 (d, J = 8.2 Hz, 1H, Har), 6.85 (t, J = 7.3 Hz, 1H, Har), 6.62 (d, J = 8.2 Hz, 1H, Har), 5.40 (s, 2H, HOCH2N), 4.60 (s, 2H, HCCH2N), 2.19 (s, 1H, HCH3). 13C{1H}-NMR (151 MHz, DMSO-d6, 296 K): δ/ppm = 151.7, 147.9, 129.1, 128.1, 127.3, 120.9, 120.4, 117.3, 115.9 (Car), 78.6 (COCH2N), 48.9(CCCH2N), 20.2 (CCH3). IR-ATR: wavenumber/cm-1: 3042 (CarH), 3027 (CarH), 3013 (CarH), 2914, (CH2/CH3), 1600, 1579, 1493 (PhR3), 1454, 1446, 1356, 1220 (CarOCH2), 1204, 1168, 1141, 1119, 1042, 942 (oxazine ring), 915.
An equimolar mixture of pC-a and Jeffamine® M-600 in a glass vial closed with a PP lid was mixed and stirred at 50°C in an oil bath for 13 days. For the following high-temperature cascade reactions (120°C, 150°C, 180°C) new mixtures of unreacted pC-a and Jeffamine® M-600 were heated in open glass vials and stored in a convection oven for 2 h for each single temperature step (Supplementary Scheme S1).
BA-a and Jeffamine® ED-600 were mixed at molar ratios of 1:0.25, 1:0.50 or 1:1 (BAa:Jeffamine®), liquefied and homogenized at 120°C in a convection oven (Heraeus 19 Function Line, UT 6 P/UT 20 P, 250°C, Thermo Fisher Scientific Inc., Waltham, United States). The mixture was degassed for 30 min in a vacuum oven (Heraeus Vacutherm VT6025, Thermo Fisher Scientific Inc., Waltham, United States). The BA-a/Jeffamine® mixtures were filled in casting molds and polymerized in a convection oven at 120, 150, and 180°C, respectively. The neat BA-a was polymerized according to the manufacturer information at 180 and 200°C. For the further course of this work, the polymerization conditions, as shown in Table 1, will be indicated in roman numbers I–V. The sample composition will be abbreviated as BA-a/J for mixtures of BA-a and Jeffamine® ED-600 and pC-a/J for mixtures of pC-a with Jeffamine® M-600. The appropriate molar ratio (benzoxazine:amine) will be indicated in brackets after the abbreviation. Specimens of the resulting polymers were cut using a wet cut-off sander (Conrad Apparatebau GmbH, Clausthal-Zellerfeld, Germany).
TABLE 1. Heating temperatures and durations in the polymerization of benzoxazine/amine mixtures and neat benzoxazine. The table shows three formulations (column) and the used polymerization program (row). The individual samples are defined by the row. A missing formulation in the row means that the sample was not examined.
NMR experiments were performed on an AVANCE NEO 600 MHz spectrometer (Bruker Corporation, Massachusetts, United States). All spectra were referenced against the residual solvent signal of the respective NMR solvent. IR-ATR spectra were recorded on an ALPHA II (Bruker Corporation, Massachusetts, United States) with a platinum-ATR unit. Recording range: 400–4000 cm−1; resolution: ±2 cm−1; 32 scans.
Polymerized samples of BA-a/Jeffamine® ED-600 copolymers, as well as reference samples of neat BA-a were subjected to Soxhlet extraction. Prior to the experiments, the Soxhlet sleeves were dried for 24 h at 80°C in a convection oven. Crushed polymer samples were extracted for 24 h with butan-2-one (MEK, 100 mL) (oil bath temperature 100°C). Afterwards, the solvent was removed under reduced pressure and the extracts as well as the residual polymer samples were dried at 60°C to mass constancy in a vacuum oven. The gel contents were determined (Eq. 1) and the mass-losses in the sleeves were related to the masses of amine originally placed in the polymer mixture (Eq. 2) from mass
Polymerization behavior was characterized with differential scanning calorimetry (DSC), using a Discovery DSC (TA Instruments, New Castle, Delaware, United States). The sample weight was between 1.5 mg and 3 mg, the heating rate was 10 K/min from −20 to 350°C. Thermogravimetric analysis (TGA) measurements were conducted under ambient atmosphere, using a TGA Q5000 V3.17 Build 265 calorimeter (TA Instruments, New Castle, Delaware, United States). The sample weight was between 1.2 and 3 mg and the measurements were performed with a heating rate of 10 K/min from 34 to 800°C.
Thermo-mechanical behaviour was characterized by dynamic mechanical analysis (DMA) with a RSA-G2 Solid Analyzer (TA Instruments, New Castle, Delaware, United States). The samples were tested in the dual cantilever mode, at a frequency of 1 Hz, from 25 to 250°C, using a heating rate of 2 K/min. The specimen size was 50 mm × 10 mm × 3 mm.
Stress-relaxation measurements were performed on rectangular specimens (50 mm × 10 mm × 3 mm), polymerized at 120°C for 2 h and 150°C for 4 h (BA-a/J-III), with a Discovery Hybrid Rheometer (TA Instruments, New Castle, Delaware, United States) in torsion-mode. The specimens were selected because they showed the best mechanical properties in preliminary tests and parametric studies. The samples were heated to the respective temperature (BA-a/J: 120°C, 130°C, 140°C, 150°C, 160°C, 170°C) and were kept at that temperature for 5 min. Then, a strain of 1.5% for BA-a/J was applied and the decrease in tension was recorded as a function of time. Relaxation times
To investigate the reprocessability and remoulding ability of the material, specimens were pressed multiple times in a manual, hydraulic bench-top laboratory press (Paul-Otto Weber GmbH, Remshalden, Germany). The two-part mould was made of aluminium alloy and five samples, each 50 mm × 10 mm × 3 mm, were produced in one operation. The semi-permeable release agent was refreshed after three press-cycles. In the press, original or healed materials were crushed into 1–3 mm sized particles. The open mould was heated to 155°C for 20 min, before the upper mould was inserted. The samples were pressed for 30 min at 155°C with 15 kN (approximately 6 MPa at each sample). Three series of tests were carried out: one, three and five crushing-pressing cycles. To evaluate the mechanical recycling or the healing ability, the original and pressed samples were tested in a three-point bending test in accordance with DIN EN ISO 604, using a Zwick/Roell Z020, 20 kN (ZwickRoell GmbH & Co. KG, Ulm, Germany).
The presence of amines during the ring opening reaction of benzoxazines is known to affect the polymerization progress in particular to decrease the polymerization temperature. However, the resulting structures are reported to strongly depend on the chemical reactivity of the respective benzoxazine and amine. Furthermore, it is not understood if the amines act as catalyst, or get incorporated into the polymer network. (Sun et al., 2015; Zong and Ran, 2019; Zhang et al., 2020). It is assumed that the benzoxazine/amine reaction follows the formation of several consecutive reaction steps with increasing reaction temperature. However, type of starting materials and applied reaction conditions affect the resulting molecular structure and reversibility of the single reaction steps. With the aim to understand the mechanism of the polymerization reaction a model system was studied. The insight in reaction pathways was then transferred to the bifunctional benzoxazine/amine system.
A monofunctional benzoxazine based on para-cresol and aniline (pC-a) was used as a model system with monoamine Jeffamine® M-600 to elucidate the reaction mechanism and to unveil the resulting molecular structures. Due to the monofunctional nature of the benzoxazine and the single primary amine group of Jeffamine®, the formation of low molecular weight products was observed that were soluble in common solvents and that were thus accessible to conventional 2D-NMR spectroscopy. At the same time, the system was assumed to inherit similar chemical reactivity to the insoluble, high molecular weight product resulting from bifunctional BA-a and diamine Jeffamine® ED-600.
The reaction of pC-a and monoamine was carried out in bulk to avoid influences from a solvent. Previous mechanistic studies in the literature suggest that several consecutive reaction steps occur with increasing reaction temperature. (Sun et al., 2015). To be able to observe as many of these steps as possible, the equimolar reaction of pC-a and Jeffamine® M-600 was first investigated at 50°C, the empirical lowest temperature at which a homogeneous mixture could be obtained. The reaction was monitored in regular intervals via NMR spectroscopy to ensure high conversion of the reactants. The integrals of the signals in the 1H-NMR spectra were normalized by the sum of the integrals of signals corresponding to all methyl groups 1 and 1′ (and 1″) present. The low reaction temperature resulted in a very slow reaction rate with saturation of conversions at 92% for pC-a and of 85% for Jeffamine® M-600 after 13 days, respectively (Figure 1). A continuous integral decrease of the characteristic signals that were assigned to pC-a (methylene bridge-signals 3 and 4) and Jeffamine® M-600 (single-proton signal 2) indicated the opening of the oxazine ring and the consumption of Jeffamine®. At the same time new signals appeared that suggested the formation of a main product A (74% yield of pC-a) and a side product B (10% yield of pC-a).
FIGURE 1. 1H-NMR (600 MHz, DMSO-d6, 296 K): Equimolar mixture of pC-a and Jeffamine® M-600 (red), and reaction products A and B after 312 h at 50°C (blue) with assigned signals and indicated characteristic 2D-NMR correlations for A.
The structure of A was solved using 1D- and 2D-NMR spectroscopy. The characteristic 1H-NMR 1,2,4-trisubstitution pattern of the phenolic benzene ring in pC-a relative to the oxygen atom (doublets 5, 6, singlet 7) changed into a tetrasubstitution pattern (singlets 6′, and 7′). Signals 3′ and 4′ correspond to the newly formed methylene bridges that formerly formed the oxazine ring and 2′ corresponds to the single proton in the former Jeffamine®. A multitude of 2D-correlations prove the constitution of the ring-opened phenolic benzoxazine/amine structure (benzoxazine:amine = 1:1), in which the former amine is attached via methylene bridge 4′ in ortho-position to the hydroxyl group and which thus has a 1, 2, 4, 6-tetrasubstituted phenolic benzene ring. Signals 8′ and 9′ show the formation of two secondary amine groups in the product. Signals 10’ (doublet 2 H), 11′ (triplet 1 H) and 12′ (triplet 2 H) with corresponding COSY correlations indicate the intact monosubstituted N-phenyl moiety attached to 9′.
Due to its low quantity in the product mixture, the structure of side product B could not be fully described. However, chemical shifts, multiplicities, and relative integrals of signals 3’’ (doublet 2 H) and 4’’ (singlet 2 H) suggest the presence of molecule B that was formed in a reaction of A with a second equivalent of pC-a (benzoxazine:amine = 2:1). This assumption is further supported by the fact that a higher conversion was detected for pC-a than for Jeffamine® M-600. This secondary amine induced opening of oxazine-rings has also been recently reported in literature. (Zhang et al., 2020).
Sun et al. (2015) reported a mechanism for the reaction of benzoxazines with amines. A simplified version of this mechanism is shown in Scheme 1. In a first step, the lone pair of the amine attacks the electrophilic carbon atom of the OCH2N moiety of the benzoxazine, inducing opening of the oxazine ring and leading to a covalently bonded benzoxazine/amine adduct. This adduct thermally decomposes at higher temperatures into ionic pair of phenolate and iminium ion. Two potential different final products are reported. On the one hand, the reaction of phenolate oxygen with the iminium ion can lead to a phenoxy structure. On the other hand, electrophilic aromatic substitution of the aromatic ortho-hydrogen of the phenolic benzene ring with the iminium ion can lead to a phenolic benzoxazine/amine structure. In literature, it is assumed that the reversible reactions of the adduct and intermediate product result a reaction equilibrium that breaks down by further heating with a subsequent crosslinking process. (Wang et al., 2016).
SCHEME 1. Simplified reaction mechanism of benzoxazine and amine proposed in previous report. Adapted from Sun et al. (2015), with permission from RSC Publishing.
In contrast to the mechanism suggested by Sun et al. and Wang et al., solely the formation of A and B was observed, which are analogous to the reported phenolic structures. Neither the benzoxazine/amine adduct, nor the ionic intermediates were observed at any stage of the reaction, despite the very low reaction temperature at 50°C. However, they can be assumed as logical intermediates or transition states. The evidence of analogous benzoxazine/amine structures in the literature was gathered in solution-based reactions. (Sun et al., 2015; Zong and Ran, 2019). It is assumed that potential intermediates resulting from the pC-a/amine reaction possess a lower thermodynamic stability in absence of a solvent favouring the formation of product A. The observed effects might also be explained by electronic influences of the polyether chain on the stability of the intermediates. The proposed phenoxy structure was not observed here, either. The formation of this structure was recently reported to be favoured in ortho blocked benzoxazines. (Zhang et al., 2019). The absence of such ortho-blocking substituents in pC-a led to an exclusive formation of the phenolic structure. The newly formed secondary amine group 8′ itself can induce opening of further oxazine rings of unreacted pC-a, leading to phenolic benzoxazine/amine structure B. This has also been reported by Zhang et al., 2020. A is formed in higher amounts than B, which can probably be explained by steric hinderance of secondary amine 8’ through the polyether chain. At higher temperatures and at different stoichiometric ratios of benzoxazine and amine the secondary amine induced reaction may play a more important role.
The reaction was also studied at higher reaction temperatures in a cascade of three successive stages at 120, 150, and 180°C for 2 hours, respectively. At the first stage (120°C), phenolic products A and B are formed as determined at the low temperature experiment, as observed by the presence of all the characteristic signals in 1H-NMR spectra (Supplementary Figure S1). At higher temperatures (up to 180°C), however, these signals decrease in magnitude and a multitude of further, broad signals are observed. These indicate the formation of various oligomers through a complex set of reactions. At the same time, the relative sums of integrals corresponding to aromatic protons decrease from 8 H (unreacted mixture of pC-a and Jeffamine® M-600) to 5 H at the last stage of the reaction. This indicates the occurrence of further substitution reactions, probably in the N-phenyl moieties of the former benzoxazine. (Martos et al., 2020). Another possible explanation for the observed effect is thermal degradation of the polymer accompanied by oxidation reactions in aromatic structures or evaporation of volatile aromatic fragmentation products like aniline. (Zhao et al., 2018). The reformation of free primary Jeffamine®, as described in the literature for different amines (Zhang et al., 2020), was not observed, indicating the permanent incorporation of Jeffamine® into the reaction product.
Based on the results of the model reaction (pC-a and Jeffamine® M-600), a general reaction polymerization mechanism and a polymer structure are proposed for the reaction between BA-a and Jeffamine® ED-600 (Scheme 2). In a first step, the primary amine groups in Jeffamine® ED-600 probably induce opening of the oxazine rings of BA-a, which leads to linear polymer chains, showing repeated phenolic benzoxazine/amine motives, analogous to A. The interaction between benzoxazine and primary amine reduces the overall polymerization temperature compared to the neat benzoxazine. The polymer chains are probably then crosslinked via secondary amine induced opening of oxazine rings. The crosslinking density presumably depends on the polymerization temperature and the stoichiometric ratio of benzoxazine and amine. Higher relative benzoxazine contents probably lead to increased crosslinking density. Further crosslinking reactions assumedly occur, for example, via substitution reactions in the N-phenyl moieties (Martos et al., 2020) or other complex reactions, yielding a highly crosslinked three-dimensional polymer network.
SCHEME 2. Proposed reaction mechanism and polymer structure for polymerization reaction of BA-a (black) and Jeffamine® ED-600 (red).
Samples of BA-a/Jeffamine® ED-600 mixtures with stoichiometric benzoxazine:amine ratios of 1:1, 1:0.5 and 1:0.25 were polymerized at 120, 150°C for 2 h (BA-a/J-II) respectively, or at 120, 150, 180°C for 2 h (BA-a/J-IV), respectively. The corresponding IR spectra of the BA-a/Jeffamine® ED-600 reaction products are qualitatively similar to those of the pC-a/Jeffamine® M-600 mixtures, thermally treated at the same conditions (Figure 2, Supplementary Figure S2). Especially the presence of the absorption band 1477 cm-1, corresponding to the tetrasubstituted benzene ring, indicates the formation of similar phenolic benzoxazine/amine structures as observed in the model reaction. This further proves that the reaction of BA-a and Jeffamine® ED-600 and the model reaction of pC-a and Jeffamine® M-600 follow a similar reaction mechanism.
FIGURE 2. IR-ATR: Mixtures of BA-a with Jeffamine® ED-600 or pC-a with Jeffamine® M-600 in different stoichiometric ratios polymerized at different temperatures.
Abovementioned polymerized samples of BA-a/Jeffamine® ED-600 mixtures, as well as reference samples of neat BA-a, polymerized at either the same conditions (BA-a-II, BA-a-IV) or 180, 200°C for 2 h (BA-a-V), respectively, were subjected to Soxhlet extractions. The gel contents were calculated and the determined mass-losses during extraction were related to the masses of amine, Δm/mamine, that were initially added to the respective polymer mixtures (Figure 3).
FIGURE 3. Gel content (red), and Δm/mamine ratios (blue) determined via Soxhlet extraction experiments for BA-a/Jeffamine® ED-600 mixtures of different stoichiometries (X:Y) (crossed pattern), and pure BA-a (no pattern) polymerized at different temperatures.
For the neat polymerized benzoxazine BA-a-II, a gel content of 0% is determined, indicating that the applied polymerization temperatures were too low to initiate polymerization. In contrast, all samples that included Jeffamine® (BA-a/J-II) show an unexpected high gel content of 86%–95%, indicating that polymerization takes place at low polymerization temperatures and includes already the secondary amine induced ring opening and by this crosslinking reaction at a significant degree. The sample with a benzoxazine:amine ratio of 1:1 shows the lowest gel content, which can probably be explained by a lower crosslinking density and a higher content of flexible polyether chains. Increasing the reaction temperature up to 180°C (BA-a/J-IV) leads to high gel contents of 93%–97% for all benzoxazine:amine ratios, indicating further progress of the polymerization reaction at these conditions. The reference samples of pure BA-a also showed high gel contents at polymerization temperatures above 180°C (BA-a-IV, BA-a-V). It can therefore be assumed that the observed gel content of the BA-a/J-IV samples at these high temperatures results from both, the network formation via benzoxazine/amine copolymerization and homopolymerization of residual unreacted oxazine rings of BA-a. With the aim to elucidate the role of the amine, the determined mass losses during extraction were related to the masses of amine that were initially placed in the respective reaction mixtures. The obtained Δm/mamine values reach up to 0.25 indicating that at least 75% of the amine remains in the polymer network, even in an assumed extreme case that the extracted material was pure Jeffamine®. However, spectroscopic analyses (Supplementary Figures S3, S4) show that all extracts did not contain intact Jeffamine® ED-600 chains. They contained a multitude of fragments of both the polyether chain and the benzoxazine backbone that were probably abstracted from the polymer scaffold during the extraction process. This, again, suggests that the amine stays covalently incorporated into the polymer network, even at elevated reaction temperatures.
The polymerization and thermo-mechanical behavior of BA-a was studied in presence of the amine bearing polyether Jeffamine®, in a stoichiometric ratio of 1:0.25 (BA-a: Jeffamine® ED-600). Basically, the presence of an amine significantly reduces the polymerization temperature of BA-a. Comparison of the DSC of the non-polymerized BA-a monomer and the BA-a/J mixture shows that two endothermic peaks occur in the presence of Jeffamine®. The first peak at about 120°C is associated with the ring opening reaction with the amine. The second peak at about 220°C is attributed to the opening reaction of the remaining closed oxazine rings. (Gonçalves et al., 2022). Moreover, the absolute residual enthalpy ΔHR is already relatively low for BA-a/J-IV: ΔHR = 16.0 ± 2.7 J/g, compared to BA-a/-IV: ΔHR = 56.7 ± 2.1 J/g (Figure 4). The thermal behavior of the polymerization of BA-a/Jeffamine® mixture compared to neat BA-a is in agreement with the results of Sun et al., 2015, who investigated different benzoxazine/amine polymers and showed comparable DSC results. Consistent with Sun et al., BA-a/Jeffamine® mixture did not melt after being thermally treated at 120°C for 2 hours (BA-a/J-I) as can be seen in Figure 4, by the absence of any endothermic peak. This can be explained with the progressed polymerization and crosslinking process at that low temperature. Furthermore, the residual reaction enthalpy between 200 and 250°C is much lower compared to neat BA-a after heating at 120 and 150°C for 2 hours, respectively.
FIGURE 4. DSC and TGA thermograms for the BA-a/Jeffamine® ED-600 system and pure BA-a at different polymerization stages. The addition of Jeffamine® influences the polymerization behavior of the benzoxazine. The ring-opening reaction induced by Jeffamine® already occurs at 120°C and therefore generally reduces the residual enthalpy of the BA-a between 200°C and 250°C.
The various polymerization steps affected the thermal stability of the resulting BA-a/Jeffamine® polymers as was shown by TGA experiments. The first step, sample BA-a/J-I polymerized at 120°C, shows a higher thermal stability compared to BA-a-I, which is probably due to the higher crosslink density respective a higher polymerization degree as a result of the lowering of the polymerization temperature by Jeffamine®. A higher polymerization temperature reverses the effect as ring opening polymerization of BA-a takes place at 150°C to a significant extent. Up to a polymerization temperature of 150°C, the BA-a/J-II exhibits with T5% = 256.6 ± 6.1°C a lower thermal stability, in comparison to the corresponding BA-a-II with T5% = 304.6 ± 5.6°C. Sun et al., 2015 reported similar results for benzoxazine/amine mixtures with aliphatic amines. They have attributed the lower degradation temperatures of the resulting benzoxazine polymer networks to the presence of thermally less stable aliphatic chains.
As expected, polyether chains impact thermo-mechanical properties determined by DMA experiments. Figure 5 shows a strong decreases of the glass transition temperatures (Tg) of the BA-a/J polymers. Compared to the fully polymerized BA-a, (Tg = 188.2 ± 2.34°C), the polymers BA-a/J-II and–III exhibit Tg = 97.2 ± 0.4°C and Tg = 108.1 ± 0.4°C, respectively. The aliphatic polyether chains of the Jeffamine® cause a decrease in polymer network density lowering the Tg accordingly, which is in agreement with TGA results. These results are also in an agreement with studies performed by Ni et al., 2002 on the influence of different isomeric cyclohexane diacids in polyurethanes.
FIGURE 5. DMA results for different polymerization stages of the 1:0.25 BA-a/Jeffamine® polymer compared to pure BA-a, polymerized according to manufacturer information (BA-a-V). The comparison of the different stages of the BA-a/Jeffamine® illustrates the effect of the polymerization conditions and gives an insight into the flexibility of the properties adjusted by process parameters.
Despite the strong impact of Jeffamine on the thermal behavior, the storage modulus did not change significantly. For BA-a/J-II (E´ = 2280 ± 350 MPa) the storage modulus is in the same range as for the polymerized BA-a (E´ = 2300 ± 300 MPa).
In contrast to BA-a/J-IV, the increase in storage modulus for sample BA-a/J-II between 140°C and 250°C is likely due to post-curing processes and/or further aromatic substitutions. This indicates the complexity and temperature dependency of the polymer network and is in line with the molecular studies on polymer network formation.
Rheological tests proved that BA-a/Jeffamine® copolymers feature vitrimeric properties such as stress relaxation and reprocessing ability. All BAa/J-III specimens (BAa:Jeffamine® ratio of 1:0.25 and 1:0.5) were heated to the respective temperature and kept at that temperature for 5 minutes before applying the strain and stress relaxation measurement. Figure 6 shows exemplarily the stress relaxation modulus in dependence of time and temperature treatment for sample BAa/J-III (1:0.25) (Figure 6, left). The initial modulus varies slightly for the studied temperatures due to standard deviation determined on three independent samples and rheology measurements (Supplementary Figure S6). All samples show similar initial relaxation moduli at temperatures above the glass transition temperatures (Tg = 97.2 ± 0.4°C for a ratio of 1:0.25 and Tg = 50.3 ± 0.2°C for 1:0.5), followed by quick relaxation of stress (Figures 6A, B). The relaxation times for the BA-a:Jeffamine® ratio of 1:0.25 vary with test-temperature, from 11 s at 170°C to 1895 s at 120°C, and follow the Arrhenius law. For the mixture-ratio of 1:0.5, the relaxation times range from 9 s at 170°C to 256 s at 120°C; the higher the amine content, the faster stress-relaxation. Based on the linear dependency of the temperature and relaxation time, the activation energy for the 1:0.25 samples was determined with Ea = 145.8 kJ/mol. The activation energy depends on several parameters, like the glass transition temperature and the bond exchange mechanism. Values in the range of 46 kJ/mol to 181 kJ/mol have been reported. (Anagwu et al., 2022; Adjaoud et al., 2023). In comparison to other vitrimeric bond exchange activation energies, the Ea of the BA-a/Jeffamine® copolymers is relatively high. This could be due to the stoichiometric BAa:Jeffamine® ratio of only 1:0.25. An increase of Jeffamine® by varying the stoichiometry yields a decrease in Ea. The activation energy for the 1:0.5 samples is 88.0 kJ/mol. If the molar ratio of Jeffamine® increases, the concentration of covalent adaptive bonds increases, leading to a decrease in Ea.
FIGURE 6. Stress-relaxation measurements at different temperatures. BA-a/J-III: a) Relaxation modulus over time (exemplary for 1:0.25), b): Arrhenius plot based on the relaxation times calculated from the measurements for 1:0.25 filled square and 1:0.5 unfilled square.
Based on the Arrhenius plot, the topology freezing temperature Tv can be calculated using Maxwell´s equation (Supplementary Eqs 1–5). (Dhers et al., 2019). The Tv for the BA-a/Jeffamine® system 1:0.25 is 94°C, which is in the range of Tg. A similar Tv-Tg relationship is also shown for a BA-a/Jeffamine® ratio of 1:0.5, where the Tv = 39°C is also in the range of the Tg. In general, vitrimers are distinguished into two classes. Those with Tv above Tg and vice versa. (Anagwu et al., 2022). A material with a similar relationship of Tv and Tg was reported by Adjaoud et al. (Adjaoud et al., 2022) for a lignin-based benzoxazine exhibiting Tv and Tg in the same range of about 182°C.
The reaction mechanism, gel content and thermo-mechanical experiments prove that the benzoxazine/amine reaction yields a phenolic aminomethyl structure in a reaction equilibrium at low temperatures. Temperature treatments at 150 and 180°C result a crosslinked structure originating from an amine induced ring-opening reaction. The resulting vitrimeric behavior was not expected as the subsequent curing at temperatures higher than 120°C were reported to be irreversible. The deviating observations could result from two facts: 1) the reaction was performed without the use of solvents and 2) the applied amine bears oxypropylene units in contrast to the studied aromatic and aliphatic hydrocarbon-based amines. As Sun et al., 2015 we assume that the electrophile substitution reaction leading to phenolic structure A is irreversible. Based on the Arrhenius-like stress-relaxation behavior, we propose a possible dynamic reaction which is based on an associative mechanism that involves exchange reactions between primary and secondary amines in an addition elimination reaction involving methylene units with positive partial charges. However, further extensive research is needed to prove this hypothesis and to reveal the nature of the dynamic reaction that is responsible for the vitrimer-like behavior of this benzoxazine/Jeffamine® system.
The BA-a/Jeffamine® ED-600 polymer was shown to exhibit vitrimer-like behavior in stress-relaxation measurements. Hence, it should be possible to crush and heal the polymer multiple times, similar to a mechanical recycling process of a thermoplastic material. The results of the three-point bending test after the cyclic crushing-pressing process are shown in Figure 7. Crushing-pressing cycles do not seem to have any effect on the flexural modulus and the values for all crushing-pressing cycles are in the same range of 2300–2700 MPa for the specimens with polymerization condition 120, 150°C each 2 h (BA-a/J-II) and 1600–2000 MPa for 120, 150, 180°C each 2 h (BA-a/J-IV). In general, the high temperature conditions at 120, 150, 180°C for 2 h each, negatively influence the flexural modulus, which is also observed in the storage modulus in the DMA.
FIGURE 7. Results of the three-point bending tests for the mechanically recycled samples with Flexural Modulus (left) and Bending Strength (right). Different polymerization states were tested and recycled up to 5 times each (0–5 x pr.).
In contrast to the modulus, the strength is strongly affected by the mechanical recycling process. After the first crushing-pressing step, the flexural strength decreases, which could be due to defects in the material, such as particle boundaries. Residues of the release agent could also have a significant influence on the bonding process. It adheres to the specimen after the pressing process, and thus also to the interfaces of the pressed particles. After the pressing cycles, the flexural strength of the specimens polymerized at 180°C (BA-a/J-IV) is reduced by about 30% compared to the specimens polymerized at 120°C and 150°C for 2 h each (BA-a/J-II). This could be explained by the occurrence of a temperature-dependent molecular structure or initial degradation processes in Jeffamine® at high temperatures.
Material characterization and structure elucidation have been merged for the first time for a dynamic benzoxazine/polyetheramine using Jeffamine® ED-600 to gain better understanding of the resulting polymer network. Mechanistical studies on a model system based on a monofunctional benzoxazine and monoamine proved that the initial step corresponds to the amine mediated opening of the oxazine rings of BA-a. Bifunctional benzoxazine and diamines result linear polymer chains with repeated phenolic benzoxazine/amine motifs. Moreover, the polymer chains are crosslinked by secondary amine-induced opening of oxazine rings and the introduced amine remains covalently incorporated into the polymer network, as proven by extraction experiments. The mechanical properties of the polymers are similar to those of pure polymerized BA-a, but thermal properties are strongly affected by the Jeffamine® as the glass transition temperature is reduced significantly. The addition of Jeffamine® and by this the presence of amines reduce the polymerization temperature and introduce vitrimeric behavior as proven by stress-relaxation tests; the latter enables reprocessability. The activation energy of the bond exchange reaction for a BA-a:Jeffamine® ratio of 1:0.25 was calculated to be 146 kJ/mol, and the topology freezing temperature was determined to be 97°C. The high Ea in comparison to other vitrimers could be explained by the very low amine ratio. At a higher Jeffamine® ratio (1:0.5), the activation energy and topology freezing temperature decrease to 88.0 kJ/mol and 39°C, respectively. Thus, Ea and Tv as well as general material properties could be adjusted with ratio of benzoxazine and amine. Further research should address the effect of the polymerization parameters and the stoichiometry on the thermo-mechanical properties, to gain a better understanding of the structure-property-relationship of this vitrimer. The dynamic reaction is possibly based on an associative addition elimination mechanism involving primary and secondary amines as well low field shifted methylene units. However, additional research is needed to fully reveal the nature of the dynamic reaction enabling the vitrimeric properties.
The original contributions presented in the study are included in the article/Supplementary Material, further inquiries can be directed to the corresponding author.
LP and AW planned, performed, and evaluated the experiments; prepared the figures; wrote the manuscript. LP was responsible for the characterization of the thermal properties, the vitrimer behavior and the reprocessing characteristics. AW was responsible for determination of the reaction mechanism and the development of the structure model. Both have contributed equally in first authorship to this work. KK and TU guided the research. KK was responsible for the funding and supervision of the project and edited the manuscript. All authors contributed to the article and approved the submitted version.
We acknowledge the financial support from the Bundesministerium für Bildung und Forschung (BMBF) through the NanoMatFutur award (DuroCycleFVK 03XP0001) and Bundesministerium für Wirtschaft und Klima (BMWK) through the ZIM fiamtec network (CFKadapt 16KN102021).
The authors declare that the research was conducted in the absence of any commercial or financial relationships that could be construed as a potential conflict of interest.
All claims expressed in this article are solely those of the authors and do not necessarily represent those of their affiliated organizations, or those of the publisher, the editors and the reviewers. Any product that may be evaluated in this article, or claim that may be made by its manufacturer, is not guaranteed or endorsed by the publisher.
The Supplementary Material for this article can be found online at: https://www.frontiersin.org/articles/10.3389/frsfm.2023.1197868/full#supplementary-material
Adjaoud, A., Puchot, L., Federico, C. E., Das, R., and Verge, P. (2023). Lignin-based benzoxazines: A tunable key-precursor for the design of hydrophobic coatings, fire resistant materials and catalyst-free vitrimers. Chem. Eng. J. 453, 139895. doi:10.1016/j.cej.2022.139895
Adjaoud, A., Puchot, L., and Verge, P. (2022). High-tg and degradable isosorbide-based polybenzoxazine vitrimer. ACS Sustain. Chem. Eng. 10, 594–602. doi:10.1021/acssuschemeng.1c07093
Adjaoud, A., Trejo-Machin, L., and Verge, P. (2021). Polybenzoxazines: a sustainable platform for the design of fast responsive and catalyst-free vitrimers based on trans-esterification exchanges. Polym. Chem. 12, 3276. doi:10.1039/D1PY00324K
Agag, T., Geiger, S., Alhassan, S. M., Qutubuddin, S., and Ishida, H. (2010). Low-viscosity polyether-based main-chain benzoxazine polymers: Precursors for flexible thermosetting polymers. Macromolecules 43, 7122–7127. doi:10.1021/ma1014337
Anagwu, F. I., Thakur, V. K., and Skordos, A. A. (2022). High-Performance vitrimeric benzoxazines for sustainable advanced materials: Design, synthesis, and applications. Macromol. Mat. Eng. 308, 2200534. doi:10.1002/mame.202200534
Arslan, A., Motallebzadeh, A., Kiskan, B., Demirel, A. L., Kumbaracia, V., and Yagci, Y. (2018). Combining benzoxazine and ketene chemistries for self-healing of high performance thermoset surfaces. Polym. Chem. 9, 2031–2039. doi:10.1039/c8py00293b
Bo, C., Sha, Y., Song, F., Zhang, M., Hu, L., Jia, P., et al. (2022). Renewable benzoxazine-based thermosets from cashew nut: Investigating the self-healing, shape memory, recyclability and antibacterial activity. J. Clean. Prod. 341, 130898. doi:10.1016/j.jclepro.2022.130898
Dhers, S., Vantomme, G., and Avérous, L. (2019). A fully bio-based polyimine vitrimer derived from fructose. Green Chem. 21, 1596–1601. doi:10.1039/c9gc00540d
Fortman, D. J., Brutman, J. P., De Hoe, G. X., Snyder, R. L., Dichtel, W. R., and Hillmyer, M. A. (2018). Approaches to sustainable and continually recyclable cross-linked polymers. ACS Sustain. Chem. Eng. 6, 11145–11159. doi:10.1021/acssuschemeng.8b02355
Gonçalves, R. V., Liposki, I. Q., Weber Dias, L., Baldissera, A. F., da Silva Silveira, M. R., Ferreira, C. A., et al. (2022). Corrosion protection of benzoxazine and cardanol-doped polyaniline coatings. J. Coat. Technol. Res. 19, 575–586. doi:10.1007/s11998-021-00545-2
Gulyuz, S., Yagci, Y., and Kiskan, B. (2022). Exploiting the reversible covalent bonding of boronic acids for self-healing/recycling of main chain polybenzoxazines. Polym. Chem. 13, 3631–3638. doi:10.1039/d2py00068g
Khan, M. M., Halder, K., Shishatskiy, S., and Filiz, V. (2018). Synthesis and crosslinking of polyether-based main chain benzoxazine polymers and their gas separation performance. Polymers 10, 221. doi:10.3390/polym10020221
Kiskan, B., and Yagci, Y. Self-healing of poly(propylene oxide)-polybenzoxazine thermosets by photoinduced coumarine dimerization. Jou. Pol. Sci (2014) Part A Polym. Chem. 52:2911–2918. doi:10.1002/pola.27323
Kloxin, C. J., Scott, T. F., Adzima, B. J., and Bowman, C. N. (2010). Covalent adaptable networks (CANs): A unique paradigm in cross-linked polymers. Macromolecules 43, 2643–2653. doi:10.1021/ma902596s
Liu, Y., Huang, J., Su, X., Han, M., Li, H., Run, M., et al. (2016). Shape memory polybenzoxazines based on polyetheramine. React. Funct. Polym. 102, 62–69. doi:10.1016/j.reactfunctpolym.2016.03.010
Luzuriaga, A. R., Martin, R., Markaide, N., Rekondo, A., Cabanero, G., Rodrıguez, J., et al. (2016). Epoxy resin with exchangeable disulfide crosslinks to obtain reprocessable, repairable and recyclable fiber-reinforced thermoset composites. Mat. Horiz. 3, 241–247. doi:10.1039/c6mh00029k
Martos, A., Soto, M., Schäfer, H., Koschek, K., Marquet, J., and Sebastián, R. M. (2020). Highly crosslinked polybenzoxazines from monobenzoxazines: The effect of meta-substitution in the phenol ring. Polymers 12, 254. doi:10.3390/polym12020254
Montarnal, D., Capelot, M., Tournilhac, F., and Leibler, L. (2011). Silica-like malleable materials from permanent organic networks. Science 334, 965–968. doi:10.1126/science.1212648
Ni, H., Dauma, J. L., Thiltgen, P. R., Soucek, M. D., Simonsick, W. J., Zhong, W., et al. (2002). Cycloaliphatic polyester-based high-solids polyurethane coatings. Prog. Org. Coatings 45, 49–58. doi:10.1016/S0300-9440(02)00100-5
Puozzo, H., Saiev, S., Bonnaud, L., De Winter, J., Lazzaroni, R., and Beljonne, D. (2022). Robust and direct route for the development of elastomeric benzoxazine resins by copolymerization with amines. Macromolecules 55, 10831–10841. doi:10.1021/acs.macromol.2c01593
Ručigaj, A., Ambrožič, R., and Krajnc, M. (2020). Thermally assisted self-healing and shape memory behavior of diphenolic acid-based benzoxazines. Macromol. Mat. Eng. 305, 2000463. doi:10.1002/mame.202000463
Sriharshitha, S., Krishnadevi, K., Devaraju, S., Srinivasadesikan, V., and Lee, S. (2020). Eco-friendly sustainable poly(benzoxazine-co-urethane) with room-temperature-assisted self-healing based on supramolecular interactions. ACS Omega 5, 33178–33185. doi:10.1021/acsomega.0c04840
Sun, J., Wei, W., Xu, Y., Qu, J., Liu, X., and Endo, T. (2015). A curing system of benzoxazine with amine: Reactivity, reaction mechanism and material properties. RSC Adv. 5, 19048–19057. doi:10.1039/c4ra16582a
Takeichi, T., Nakamura, K., Agag, T., and Muto, H. (2004). Synthesis of cresol-based benzoxazine monomers containing allyl groups and the properties of the polymers therefrom. Des. Monomers Polym. 7, 727–740. doi:10.1163/1568555042474121
Trejo-Machin, A., Puchot, L., and Verge, P. (2020). A cardanol-based polybenzoxazine vitrimer: Recycling, reshaping and reversible adhesion. Polym. Chem. 11, 7026–7034. doi:10.1039/d0py01239d
Wang, J., Zhen, X. Y., Fu, F. Y., and Liu, X. D. (2016). Latent curing systems stabilized by reaction equilibrium in homogeneous mixtures of benzoxazine and amine. Sci. Rep. 6, 38584. doi:10.1038/srep38584
Wang, X., Zhang, S., He, Y., Guo, W., and Lu, Z. (2022). Reprocessable polybenzoxazine thermosets with high tgs and mechanical strength retentions using boronic ester bonds as crosslinkages. Polymers 14, 2234. doi:10.3390/polym14112234
Wen, Z., Bonnaud, L., Dubois, P., and Raquez, J. (2022). Catalyst-free reprocessable crosslinked biobased polybenzoxazine-polyurethane based on dynamic carbamate chemistry. J. Appl. Polym. Sci. 139, e52120. doi:10.1002/app.52120
Witik, R. A., Teuscher, R., Michaud, V., Ludwig, C., and Månson, J. E. (2013). Carbon fibre reinforced composite waste: An environmental assessment of recycling, energy recovery and landfilling. Compos. Part A 49, 89–99. doi:10.1016/j.compositesa.2013.02.009
Yagci, Y., Kiskan, B., and Ghosh, N. N. (2009). Recent advancement on polybenzoxazine-A newly developed high performance thermoset: Highlight. Pol. Sci. Part A Polym. Chem. 47, 5565–5576. doi:10.1002/pola.23597
Zhang, L., Zhao, Z., Dai, Z., Xu, L., Fu, F., Endo, T., et al. (2019). Unexpected healability of an ortho-blocked polybenzoxazine resin. ACS Macro Lett. 8, 506–511. doi:10.1021/acsmacrolett.9b00083
Zhang, S., Zong, J., Ran, Q., and Gu, Y. (2020). Facile preparation of lightweight and robust polybenzoxazine foams. Ind. Eng. Chem. Res. 59, 7575–7583. doi:10.1021/acs.iecr.0c00313
Zhao, Y., Xu, Y., Xu, Q., Fu, F., Thang, Y., Endo, T., et al. (2018). Significant improvement on polybenzoxazine toughness achieved by amine/benzoxazine copolymerization-induced phase separation. Macromol. Chem. Phys. 219, 1700517. doi:10.1002/macp.201700517
Keywords: benzoxazine, vitrimer network, polyetheramine (PEA), stress-relaxation behavior, reprocessibility
Citation: Pursche L, Wolf A, Urbaniak T and Koschek K (2023) Benzoxazine/amine-based polymer networks featuring stress-relaxation and reprocessability. Front. Soft. Matter 3:1197868. doi: 10.3389/frsfm.2023.1197868
Received: 31 March 2023; Accepted: 07 June 2023;
Published: 22 June 2023.
Edited by:
Kay Saalwächter, Martin-Luther-University Halle-Wittenberg, GermanyReviewed by:
Martin Hager, Friedrich Schiller University Jena, GermanyCopyright © 2023 Pursche, Wolf, Urbaniak and Koschek. This is an open-access article distributed under the terms of the Creative Commons Attribution License (CC BY). The use, distribution or reproduction in other forums is permitted, provided the original author(s) and the copyright owner(s) are credited and that the original publication in this journal is cited, in accordance with accepted academic practice. No use, distribution or reproduction is permitted which does not comply with these terms.
*Correspondence: Katharina Koschek, S2F0aGFyaW5hLktvc2NoZWtAaWZhbS5mcmF1bmhvZmVyLmRl
†These authors have contributed equally to this work
Disclaimer: All claims expressed in this article are solely those of the authors and do not necessarily represent those of their affiliated organizations, or those of the publisher, the editors and the reviewers. Any product that may be evaluated in this article or claim that may be made by its manufacturer is not guaranteed or endorsed by the publisher.
Research integrity at Frontiers
Learn more about the work of our research integrity team to safeguard the quality of each article we publish.