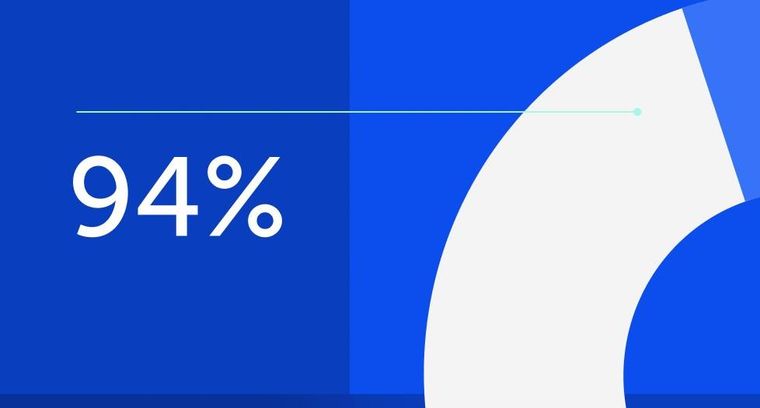
94% of researchers rate our articles as excellent or good
Learn more about the work of our research integrity team to safeguard the quality of each article we publish.
Find out more
REVIEW article
Front. Soft Matter, 15 March 2023
Sec. Self-Assembly and Self-Organisation
Volume 3 - 2023 | https://doi.org/10.3389/frsfm.2023.1109508
The increased interest of the scientific community in lipid nanoparticles has pushed the boundaries of personal medicine and drug delivery. The focus has been set on vesicular nanocarriers, as their structure and functionalities have been well described, but the application of their non-lamellar counterparts, cubosomes and hexosomes, has shown their potency as drug carriers. In addition, the sponge phase dispersion (L3, an intermediate between the lamellar and the bicontinuous cubic) has also been proved effective for the encapsulation of large macromolecules. Their physicochemical characterization has improved in the past decades due to the investigations conducted at high-power synchrotron facilities and the application of surface-sensitive techniques, discovering new connections between physical parameters and biological performance. Several administration routes of cubosomes and hexosomes have been studied, such as intravenous, dermal, transdermal, and oral, to evaluate their cytotoxicity and distribution in biological media. This review aims to summarize the challenges and recent achievements of cubosomes, hexosomes, and sponge nanoparticles as suitable carriers for the administration of bioactives.
Modern nanoparticles useful for applications in nanomedicine are often designed to respond to a multiplicity of tasks (Lammers et al., 2011; Biffi et al., 2015). By always keeping in mind the need for the lowest possible cytotoxicity, the versatility of these nanocarriers toward different administration routes and the possibility of modifying their surface via functionalization/decoration with targeting agents and/or fluorescent dyes are additional examples of desired qualities. In this arena, amphiphilic lipids are considered suitable building blocks (Aleandri and Mezzenga, 2020; Murgia et al., 2020), as they fulfill these prerogatives, simultaneously offering high biodegradability and showing rich polymorphism in water (Sahdev et al., 2014).
Among the various lipid-based self-assembled nanoparticles, lamellar phase dispersions, composed of a lipid bilayer enclosing a water compartment and being known as liposomes or vesicles, are the first and most widely studied, as they resemble the natural structure of cell membranes. Liposomes are largely proposed as nanocarriers for nanomedicine applications, and the first FDA-approved nanodrug was indeed a liposomal formulation (Doxil) (Barenholz, 2012), while scale-up, stability, cost, and reproducibility issues have now been addressed (Crommelin et al., 2020).
The non-lamellar counterparts of lamellar dispersions, namely, cubosomes (Gustafsson et al., 1997) and hexosomes (Yaghmur and Glatter, 2009), are now under investigation and often proposed as a valid alternative. Moreover, in recent years, the “melted” cubic phase known as the sponge phase (Angelov et al., 2011; Zhai et al., 2020a) has gained much interest since it can accommodate quite large macromolecules (e.g., proteins and enzymes) and can be dispersed in water similarly to cubosomes and hexosomes to form sponge nanoparticles, known as spongosomes (Chen et al., 2015; Valldeperas et al., 2019). Figure 1 reports the representation and morphology by an electron microscopy connection for the lipid nanoparticles under discussion.
FIGURE 1. Lipid nanoparticles from vesicles to hexosomes, increasing the curvature of the interface, passing through sponge and bicontinuous inverse phase dispersions. The cry-TEM images were reproduced with permission from Valldeperas et al. (2016) (sponge phase), Fornasier et al. (2021) (hexosomes and vesicles), and Demurtas et al. (2015) (cubosomes).
This review focuses on different aspects of these lipid nanoparticles, cubosomes, hexosomes, and spongosomes, highlighting the advances in physicochemical characterization and biological performance. The most significant findings and studies for the biennium 2020–2022 are presented.
In comparison to the original liquid crystalline phases, cubosome, hexosome, and spongosome dispersions are diluted, non-viscous aqueous materials with an internal nanostructure suitable for nanomedicine applications.
They can usually be obtained via a high-energy emulsification procedure (Murgia et al., 2020), such as ultrasonication, high-pressure homogenization, or high-speed shearing, from their bulk phase or by adding a hydrotrope, an amphiphile incapable of displaying phase behavior by itself, thus providing a salting-in effect on the building blocks otherwise insoluble in water (Barriga et al., 2019; Murgia et al., 2020).
Larsson et al. reported the first case of bicontinuous inverse cubic dispersion in the late 1990s (Longley and McIntosh, 1983; Gustafsson et al., 1997), opening the path to the history and application of cubosomes. As for the lamellar phase, the reconstruction on the nanoscale as dispersion does not affect the inner structure, and cubosomes can present bicontinuous inverse cubic phases of symmetry, where Im3m, Pn3m, and Ia3d are their original bulk phases (Aleandri and Mezzenga, 2020). Generally, two sets of non-connected water channels are formed by a tridimensional arrangement of a lipid bilayer with an average zero curvature, yielding a porous material suitable for the confinement of biomolecules. The L3 or sponge phase is considered an intermediate mesophase between the lamellar and cubic phase, a “melted” cubic phase, in which it has a similar short-range structure of interconnected water channels, but with no long-range order. Spongosomes are obtained by the dispersion of the L3 phase and have been studied extensively by the group of Nylander, especially with regard to how to produce them from bulk phases with low-energy methods (Gilbert et al., 2019; Valldeperas et al., 2019).
Similar to the bicontinuous inverse cubic and sponge phases, the inverse hexagonal bulk phase can be fragmented into nanoparticles called hexosomes. These are composed of cylindrical inverse micelles closely packed in a hexagonal array, providing a network of water channels. Usually, they are obtained using the very same procedure and building blocks that should lead to cubosomes but with the addition of surfactants characterized by small head groups and long tails that affect the curvature of the system making it more negative (Salentinig et al., 2010).
Glycerol monooleate (GMO; monoolein) and phytantriol (PHYT) are the most common lipids employed for the preparation of cubosomes and hexosomes (Zhai et al., 2019; Murgia et al., 2020), and their phase behavior was thoroughly studied. The phase sequence with an increasing water content includes an inverse micellar, a lamellar, and two inverse bicontinuous cubic phases, the Schoen double gyroid (Ia3d space group) and the Schwarz double diamond (Pn3m space group) (Mezzenga et al., 2019). In addition, an inverse hexagonal phase appears at high temperatures.
Scientific interest relies on the fact that above a certain percentage of solvent and water, Pn3m or inverse hexagonal phases can coexist separately (liquid crystal + water excess), indicating the maximum solubilization capacity of that phase for the solvent (Kulkarni et al., 2011).
The sponge phase can be obtained with monoglycerides by adding organic solvents as propylene glycol, polyethylene glycol, or ethanol that disrupt the short-range cubic network, yielding the peculiar melted phase (Angelov et al., 2011). Recently, diglycerol monooleate (DGMO) and a mixture of mono-, di-, and tri-glycerides (Capmul GMO-50) were employed as the main components of the sponge nanoparticle formulation, and its phase behavior was reported (Valldeperas et al., 2016). Figure 2 summarizes the most common components for cubosome, hexosome, and sponge nanoparticles, also referring to the conventional stabilizers employed in their formulation.
FIGURE 2. Molecular structures of the main components of lipid nanoparticles under review and combinations to obtain the desired carriers. (A) GMO or PHYT can be used as the main components to produce cubosomes in water after adding Pluronics as stabilizers; (B) addition of oleic acid (or other long-chain acids) to the same system induces the formation of hexosomes; (C) a mixture of DGMO and GMO-50 in the presence of Tween 80 as the stabilizer leads to the formation of sponge phase dispersion in water under shaking. The hydrophilic and hydrophobic units are highlighted in blue and yellow, respectively. The image of the sponge phase is reproduced from Valldeperas et al. (2016) with permission from ACS publications.
Several studies also appointed polymers as suitable materials for cubosome formulations (Blanazs et al., 2009; Chen and Li, 2021). Ha et al showed how dendritic block copolymers can yield cubosomes by tuning the molecular geometry of the amphiphile (Ha et al., 2020). Chen et al. employed fluorescently labeled PEG-b-PTPEMA as the material for preparing cubosomes and hexosomes with aggregation-induced emissions (Ptissam, 2021). Mesoporous cubosomes (average water channel diameter of ca. 45 nm) formulated using a soft-template strategy were also tested for the controllable encapsulation of albumin (Wu et al., 2022); the tunable surface charge of this carrier allowed the selective adsorption of only albumin, and its release was pH dependent.
Dispersing a lamellar phase in water to obtain vesicles is an easy task. Indeed, the ability of the lamellae to bend into a closed structure that avoids contact between the hydrophobic domain and bulk water permits the breaking of the bulk phase easily. On the contrary, the dispersions of inverse hexagonal and bicontinuous cubic phases are more difficult given the boundary conditions imposed on fragmented crystals (Kulkarni et al., 2011; Murgia et al., 2013; Barriga et al., 2019; Aleandri and Mezzenga, 2020). These aggregates possess limited colloidal stability in aqueous dispersions, and they are prone to flocculation. Therefore, an additional surfactant is required to facilitate the dispersion of these phases, and it acts as a stabilizer.
The non-ionic tri-block copolymers that present poly(ethylene oxide)–poly(propylene oxide)–poly(ethylene oxide) moieties, known as Pluronics, are historically chosen as stabilizers for cubosomes and hexosomes (Meli et al., 2015; Akhlaghi et al., 2016). The hydrophilic corona surrounding the nanoparticles provides steric stabilization, whereas the polymer hydrophobic portion (the poly(propylene oxide) moiety) interacts with the lipid bilayer, partially anchoring to it. To ensure the functionalization of the stabilizer to impart targeting, therapeutical, or imaging properties, poly(propylene oxide) ends can be modified. Our group has been quite active in formulating cubosomes with modified Pluronics and new stabilizers. We investigated the functionalization of both Pluronic F108 and F127 by coupling folic acid and fluorophores, formulating cubosomes and hexosomes suitable for theranostic applications (Caltagirone et al., 2015; Meli et al., 2015; Meli et al., 2017). Moreover, we conjugated a photosensitizer to Pluronic F108 (Jenni et al., 2020), in order to formulate cubosomes suitable for photodynamic therapy.
The design of thermoresponsive nanoparticles can be achieved by the inclusion of thermoresponsive polymers as shown by Balestri et al. They used NIPAM-based block copolymers as stabilizers for GMO cubosomes, indicating that the polymer phase transition is preserved even after inclusion into the formulation (Balestri et al., 2022). The inclusion of the polymer in the formulation yielded Im3m at a low polymer content with a typical morphology of cubosomes, as shown by cryo-TEM investigations. When approaching the polymer critical temperature, the water channel radius of the Im3m phase decreases, stabilizing cubosomes by NIPAM block copolymers suitable for thermoresponsive drug delivery. Notably, increasing the concentration of the polymer in cubosomes induced a phase transition toward vesicles, as already seen in formulations of cubosomes stabilized by Pluronic F127 (Gustafsson et al., 1997).
Although poloxamers are largely employed as stabilizers for lipid nanoparticles, they are not biodegradable in vivo and can exert a significant cytotoxic effect, which is concentration dependent (Azmi et al., 2016). For this reason, investigations on new stabilizers with a biodegradable moiety have been conducted in the past years. A Pluronic F127 analog was synthesized based on polyphosphoesters (PPEs) and used to stabilize GMO cubosomes (Fornasier et al., 2020). The study highlighted that even at high concentrations, PPEs did not affect the inner structure of nanoparticles, and it improved the stability of the formulation.
The biodegradability of natural materials can also be exploited in this regard. Hemicellulose, an abundant material discarded by the wood industry, was still applied as a stabilizer for GMO cubosomes (Naidjonoka et al., 2021). Two purified hemicellulosic extracts were tested, which showed that the content of lignin plays a crucial role in the stabilization of the water–oil interface.
Indeed, non-polymeric stabilizers have been suggested in the past years. A mixture of propylene glycol and phospholipids can ensure the stabilization of GMO cubosomes without any polymeric material being added to the formulation (Bazylińska et al., 2018). Even biosurfactants such as bile salts are able to stabilize the interface of hexosomes suitable for the topical administration of antioxidants (Fornasier et al., 2021).
Tween 80 has been employed mostly in cubosomes suitable for crossing the blood–brain barrier. A recent study by Boyd and coauthors showed that PHYT cubosomes coated by Tween 80 managed to significantly increase the uptake of the model drug rhodamine B in zebrafish as compared to a control suspension of the drug or cubosomes stabilized with Pluronics (Azhari et al., 2021).
Linoleylethanolamide-based cubosomes stabilized with either Pluronic F68, F127, and Tween 80 greatly influenced the size, inner structure, and stability, as the interaction between the stabilizer and the lipid rules the physicochemical properties of nanoparticles. Interestingly, the authors showed that such stabilizers did not impact their cytotoxic effect on cells (Mohammad et al., 2020), even though this effect was highlighted in GMO-based cubosomes stabilized by the same polymers. This finding strongly suggests that the stabilizer–lipid interaction of the material composing nanoparticles plays a significant role in their biological impact.
Scattering and microscopy methods have always been the conventional approaches to characterizing lipid nanoparticles. Given the increased interest in the field and the variety of functionalities that were added to the systems, new different approaches can be employed to investigate the nanoparticle features at the physicochemical and biological level, depending on the aim and application of these formulations.
Mainly, such techniques can be divided into three categories (a schematic flow scheme is reported in Figure 3):
• Bulk techniques, which aim to assess the bulk properties of aggregates such as the size, morphology, structure, and colloidal stability
• Surface techniques, which investigate the surface of particles suggesting how they could interact with other kinds of surfaces
• Bioassays in vitro and in vivo, which evaluate the biological impact of nanoparticles on cells, tissues, and model animals
FIGURE 3. Step-by-step approach for formulating lipid nanoparticles, followed by the key bulk surface and biological characterization.
Spectroscopies such as nuclear magnetic resonance (NMR), UV–Vis, fluorescence, Raman, and Fourier transform infrared (FT-IR) can improve the knowledge of the local structure or the orientation of specific molecules in the lipid bilayer.
The figures obtained by bulk and surface characterization can give a hint about the possible biological impact of aggregates in vitro or in vivo. Indeed, the correlation between all these complementary techniques is crucial to developing the most efficient formulation for drug delivery or imaging purposes.
Dynamic light scattering (DLS) experiments can provide information on the size distribution and hydrodynamic sizes of lipid nanoparticles. Electrophoretic light scattering (ELS) measurements provide information about the zeta potential of the formulation to investigate the colloidal stability.
Generally, cubosomes and hexosomes exhibit an average hydrodynamic size of ca. 150 nm with a low degree of polydispersity. Interestingly, GMO and PHYT cubosomes formulated with Pluronics, as stabilizers, display a quite negative (<20 mV) zeta potential. Although the components are non-ionic and the pH of the formulation is quite far from their pKa, this phenomenon puzzled the scientific community for some time. The answer was found in a common phenomenon occurring at oil/water interfaces, where the water layer surrounding the lipid nanoparticle is highly polarized by the lipid/stabilizer surface, inducing a negative charge on the Stern layer, where the zeta potential is evaluated (Beattie and Djerdjev, 2004; Driever et al., 2013). The higher the electron density of the stabilizer (i.e., the higher the content of high-electronegative atoms), the more negative the zeta potential of nanoparticles (Fornasier et al., 2020).
The same phenomenon is observed for the zeta potential of spongosomes, even though the main components do not exhibit a charge in water at the pH of application. On the other hand, the size distribution of sponge nanoparticles is broader, ranging from around 110 to 200–250 nm (Yaghmur and Glatter, 2009; Valldeperas et al., 2016).
Small-angle scattering of X-rays and neutrons represents the most powerful tool to investigate the local ordering and structure of colloidal systems in the length scale 1–300 nm (Biffi et al., 2015). The angle is typically less than 5°. The advent of large synchrotron facilities and the use of high-energy and monochromatic sources allowed subsequent access to deeper information on the structure of soft materials, which scatter less than silica and metal nanoparticles.
The main parameters acquired by scattering experiments are phase and lattice parameters. In addition to these pieces of information provided by X-ray experiments, small-angle neutron scattering (SANS) methods can also provide information on the thicknesses of the bilayer structure, fluctuations, and topological changes (Glatter, 2018; Valldeperas et al., 2019). The chemical deuteration or solvent contrast matching offers a means of altering the neutron scattering length density of a specific component in the mixture, highlighting peculiar features in the structure. A better and more comprehensive discussion about scattering experiments can be found elsewhere (Glatter, 2018).
The presence of a stabilizer can also affect the native nanostructure of lipid nanoparticles (Muller et al., 2010; Chong et al., 2015; Valldeperas et al., 2016; Valldeperas et al., 2019; Fornasier et al., 2020). Such a phenomenon impacts the drug delivery properties of the carrier (Nakano et al., 2001), as discussed in the following sections. So far, only a limited amount of studies have reported formulations of cubosomes where the stabilizer did not induce a biphasic system or a phase transition (Fornasier et al., 2020; Naidjonoka et al., 2021; Balestri et al., 2022). On the other hand, spongosomes are obtained by exploiting the interaction of the stabilizer polysorbate 80 with the lipid bilayer, yielding the melted cubic phase L3.
Interestingly, GMO cubosomes prepared using PPEs display a phase transition to hexosomes at temperatures higher than 40°C (Fornasier et al., 2020), a much lower temperature than that reported for GMO/W binary systems (ca. 90°C) and for GMO cubosomes stabilized with Pluronic F127 (higher than 95°C). This temperature-dependent phase transition is related to the higher mobility of GMO acyl chains as the temperature increases. However, considering that PPEs were used as stabilizers and that PF127 shares an almost identical hydrophobic block, the anticipated cubic-to-hexagonal phase transition recorded in PPE-stabilized cubosomes can be taken as proof of the strong interaction of PPE hydrophilic blocks with the lipid bilayer.
Commonly, hexosomes are obtained in GMO and PHYT systems on the addition of unsaturated acids with a long acyl chain, since these wedge-shaped molecules promote a more negative inverse curvature of the lipid/water interface, favoring the cubic-to-hexagonal phase transition. In addition, these kinds of molecules can also make the nanostructure tunable to changes in the pH or ionic strength (Nguyen et al., 2011; Fong et al., 2020).
Cryogenic transmission electron microscopy (cryo-TEM), a microscopy variant of TEM in which the sample is vitrified prior to the measurements, represents a powerful tool to investigate both the structure and morphology of soft matter-based systems. During the vitrification process, the sample is deposed on a grid, immersed quickly into liquid ethane (−190°C), and then, stored in liquid nitrogen prior to any measurements (Knapek and Dubochet, 1980). The fast-cooling rate vitrifies the sample, preserving its morphology and structure.
Although cryo-TEM is adopted to study the morphology, size, and size distribution of the aggregates, fast Fourier transforms of cryo-TEM images can be used to precisely determine interplanar distances and angles between crystallographic planes, giving structural information, such as SAXS measurements (Demurtas et al., 2015; Murgia et al., 2020; Naidjonoka et al., 2021).
The advent of cryo-TEM, especially, assisted in understanding the presence of interlamellar attachments and folding on the bilayer in an arrangement between a lamellar and bicontinuous cubic phase, in GMO cubosomes, hexosomes, and spongosomes on increasing the concentration of the stabilizer (Borné et al., 2002; Demurtas et al., 2015). The investigations on interlamellar attachments were fundamental in explaining the fact that they may mediate membrane fusion between the cell membrane and lipid nanoparticles (Siegel et al., 1989). Some cryo-TEM images of nanoparticles under discussion are reported in Figure 1.
Unveiling how nanoparticles interact with cells for their application in nanomedicine requires knowledge of surface and interfacial properties. These features have been recently evaluated via the interaction with a model or complex surfaces characterized by hydrophilic, hydrophobic, or amphiphilic properties (Zhang and Wang, 2012; Ellipsometry, 2015). Among the different techniques employed, quartz crystal microbalance with dissipation monitoring (QCM-D) gives information about the interfacial wet mass adsorbed on a surface, its viscoelastic properties, and the kinetics of adsorption. In a typical experiment, an alternating voltage is applied at a specific frequency to make a quartz crystal oscillate at its resonance frequency (Chen et al., 2016). When a molecule is bound or adsorbed to the crystal surface, a decrease in the resonance frequency (Df) is detected.
Different in terms of physical phenomenon, ellipsometry (EP) is a non-destructive technique based on the analysis of polarization changes caused by the reflection of light, and it allows the detailed optical and microstructural characterization of surfaces, thin films, and multilayers (Tompkins and Irene, 2005).
Both QCM-D and EP have found a large variety of applications; however, they have recently been employed in characterizing the surface and interfacial properties of lipid nanoparticles.
Most studies highlight the formation of a supported lipid bilayer as a model to mimic the nanoparticle surface and to evaluate the interactions.
A limited amount of papers were, however, focused on the adsorption of cubosomes on model surfaces made with phospholipids and biosurfactants (Fraser et al., 2012; Tajik-Ahmadabad et al., 2017; Boge et al., 2019; Dyett et al., 2019). The interaction with cell membranes for therapeutic applications can be mimicked via the formation of a supported lipid bilayer on the QCM-D crystal or EP silica surface (Jackman and Cho, 2020).
Regarding QCM-D measurements, cubosomes usually exhibit high values of dissipation. Such a finding is mostly due to the nature of lipids and their architecture in the bicontinuous cubic phase, leading to a more viscoelastic system (Tajik-Ahmadabad et al., 2017). The adsorption features measured via QCM-D and EP are strongly dependent on the composition, especially on the stabilizer employed. Incredibly, no papers have so far been devoted to the surface characterization of hexosomes and sponge nanoparticles, to the best of our knowledge. Indeed, this represents a new and rich field of investigation, allowing a systematic comparison, in terms of interfacial properties, between cubosomes, hexosomes, and spongosomes.
A recent study by Jabłonowska and collaborators also showed how Langmuir monolayers can be exploited to study the interactions of GMO and PHYT cubosomes with model membranes. By mimicking a single leaflet of the cell membrane, significant differences between the two building blocks were obtained, with PHYT nanoparticles being more effective in disrupting the lipid layer assembly, thus resulting in high cytotoxicity in vitro (Jabłonowska et al., 2021).
The biocompatibility and biodegradability of lipids have attracted great attention in the pharmaceutical field since they can be employed as emulsifiers, solubilizers, and, especially, building blocks for drug delivery platforms (Tan et al., 2022). Indeed, the use of lipid nanoparticles as drug carriers is less invasive in comparison to other methods, and it allows an increase in the selectivity and effectiveness of the therapy (Barriga et al., 2019; Lai et al., 2020; Murgia et al., 2020).
The route chosen directly affects the drug's bioavailability (Suk et al., 2016), thereby determining the onset and duration of pharmacological effects. Such routes are influenced by various factors, e.g., state and age of the patient, nature of the drug, first-pass metabolism, and convenience of application.
Depending on these parameters, administration routes can be divided into local (or topical) and systemic. The former is the simplest mode of administration; the formulation containing the drug is applied where the desired action is required. On the other hand, the systemic administration of a drug or a formulation is a more complex route, since the enteral (via the gastrointestinal system) or the parenteral (bypassing the gastrointestinal system) mode can be adopted.
The enteral route involves drug adsorption via the gastrointestinal tract and includes oral, sublingual, and rectal administration, whereas the parenteral route bypasses the gastrointestinal system, and it exploits injection (e.g., intravenous, intramuscular, and subcutaneous), inhalation, or transdermal delivery. Indeed, each route presents advantages and drawbacks depending on the nature of the drug and the biodistribution necessary to achieve a therapeutic effect. Figure 4 summarizes the administration routes of lipid nanoparticles discussed in the following sections.
FIGURE 4. Schematic representation of the administration routes of non-lamellar lipid nanoparticles for nanomedicine applications. The image was partially created with BioRender.com. The structure of the sponge phase is reproduced from Valldeperas et al. (2016) with permission from ACS publications.
Up to date, lipid nanoparticle formulations exploit both local (Lai et al., 2020) and systemic administration routes (Mulet et al., 2013).
Among all the injectable routes of administration, the intravenous drug infusion or injection provides the most efficient and rapid effect, given the minor limitations in terms of administration volume.
After the injection, nanosized objects interact with physiological fluids (e.g., blood or extracellular matrices) and several kinds of protein-like macromolecules that can be adsorbed on the surface of nanoparticles, thus leading to the formation of the so-called protein corona (Francia et al., 2019). Some of these proteins bind on the nanoparticles’ surface irreversibly and, subsequently, affect their biodistribution, immune system activation, cellular recognition, and final fate.
The protein corona composition is strongly related to the physicochemical properties of the nanoparticles, such as the size, geometry, charge of the interface, and, especially, surface features (Lesniak et al., 2013; Francia et al., 2019). For the latter reason, understanding the surface properties of the nanoparticle is pivotal in predicting the formation of the protein corona with a specific formulation.
Regarding the systemic administration of lipid nanoparticles, anti-cancer treatment is still a pivotal field of application (Varghese et al., 2022), as cubosomes and hexosomes have been quite applied in this regard, showing promising results (Yaghmur and Mu, 2021). Recently, GMO cubosomes encapsulating bedaquiline were tested as excellent inhalable medicine to treat non-small-cell lung cancer (Patil et al., 2021). In order to improve the encapsulation of cisplatin and paclitaxel, GMO cubosomes were coated with poly-Ɛ-lysine (Zhang et al., 2020). The characterization showed this formulation to be effective in reducing the proliferation of HeLa cells with a sustained release of drugs over time. Paclitaxel was also encapsulated in GMO cubosomes stabilized with Pluronic F127 and DSPE-PEG-malemide. In vivo investigations showed a significant reduction of the A431 tumor size (ca. 50%) in the cubosome-treated group (Zhai et al., 2020b). Cytryniak showed that GMO cubosomes could be doped with DOTAGA-OA177Lu as a radiotracer and accommodate doxorubicin for effective cancer treatment (Cytryniak et al., 2020). Icariin, a poorly soluble anti-cancer drug, was loaded into GMO cubosomes stabilized with Pluronic F127, which showed the significant cytotoxic effect of the formulation against SKOV-3 ovarian cancer cells (Fahmy et al., 2021). Mokhtar and collaborators tested GMO cubosomes targeted for breast cancer therapy (Mokhtar et al., 2022); the inclusion of methotrexate conjugated with lactoferrin exhibited a synergistic effect of the two molecules, with a high uptake within 4 and 24 h. The addition of omega-3 polyunsaturated fatty acids such as docosahexaenoic acid to DOPG hexosomes was studied by Yaghmur and collaborators (Bor et al., 2022a). The PEGylation process of such carrier yielded a phase transition to the lamellar phase and the resulting vesicles presented an increased circulation time in vivo. Indeed, aminolipids were also added to the GMO to produce carriers with pH-tunable properties, shifting from cubosomes to hexosomes by playing with the pH of the bulk solution (Rajesh et al., 2021).
Antibiotic resistance represents a current threat to modern medicine, and antimicrobial peptides have been addressed as a powerful solution to this problem. Some studies have highlighted the possibilities of encapsulating these peptides in cubosomes (Boge et al., 2017), as they can allow a high loading of antimicrobials and the intrinsic biocompatibility and biodegradability of lipid components. Shen and collaborators employed PHYT cubosomes loaded with antibiotics and studied their performance against A. baumannii, P. aeruginosa, and K. pneumoniae (Lai et al., 2022), finding that these lipid nanoparticles can be suitable carriers in treating diseases related to superbugs. Meikle and coauthors studied the encapsulation of different antimicrobial peptides within GMO and PHYT cubosomes stabilized with Pluronic F127 (Meikle et al., 2021). The peptides were successfully encapsulated by changing the buffer condition or the lipidic composition, yielding a bicontinuous cubic structure in most cases, except in the case of melittin and indolicidin, where a phase transition to vesicles occurred. The effect of these formulations in vitro was highly variable; PHYT cubosomes exhibited almost an exclusive antimicrobial activity, due to a different possible uptake of PHYT and GMO nanoparticles, as the authors mentioned. The same group also investigated how Gram-positive and -negative bacteria uptake GMO and GMO/DOTAP cubosomes loaded with antibiotics (Dyett et al., 2021). The nanoparticles interact in two stages with Gram-negative bacteria, first presenting their fusion with the outer membranes followed by the diffusion of the inner wall, whereas the cubosomes are slowly internalized after adhering to the outer layer in Gram-positive bacteria.
In order to formulate PHYT cubosomes with antimicrobial features, miltefosine (hexadecylphosphocholine), a broad-spectrum antimicrobial drug, was encapsulated (Malheiros et al., 2022). The study showed how the concentration of the drug can induce a phase transition from Pn3m to Im3m; this is a common phenomenon when the drug loading is high or when the drug interferes with the self-assembly properties of the lipid. Also, the transition from Pn3m to Im3m and vice versa is crucial for the diffusion of hydrophilic compounds through the water channels of both phases (Zabara and Mezzenga, 2014; Aleandri and Mezzenga, 2020), given their different topologies and geometries. Such differences may also impact the diffusion and release of the drug, thereby influencing the efficacy of the therapy. Indeed, further investigations are needed to quantify this parameter for applications in drug delivery in biologically relevant media.
A significant aspect of the systemic administration of lipid nanoparticles is the reduction of the immune response since the body can recognize the material as “non-self” (Rodrigues et al., 2018), destroying the nanoparticles before they reach their target. In this regard, several attempts have been carried out to increase the biocompatibility of the formulations (Yaghmur and Mu, 2021). Our group synthetized PPEs as an analog of Pluronic F127 and used them as stabilizers of GMO cubosomes (Fornasier et al., 2020). The conventional and PPE formulations were then tested in terms of the activation of the complement system, the first line of defense of our body. These results showed how the immune response of the cubosomes is greatly connected to the stabilizer used and that the hydrophilicity is indeed crucial. Another strategy to regulate the response is to actually support the immune system in patients with compromised activity due to immune illnesses. Ovoalmbumin was loaded in cubosomes formulated with CTAB and Polygonatum sibiricum polysaccharide, an immunostimulant and active ingredient of Polygonatum sibiricum (Liu et al., 2020a). Such a formulation was able to activate dendritic cells and promote lymphocyte proliferation, stimulating the immune response.
Given the current attention toward vaccine delivery, cubosomes have been tested for this application as well (Gajda et al., 2020). PHYT cubosomes containing also CTAB were functionalized with a layer-by-layer approach with poly(diallydimethyl ammonium chloride) (PDDAC) and encapsulated ovalbumin as a model antigen. The humoral- and immuno-response after decoration with PDDAC was beneficial, opening a new route of application of bicontinuous cubic lipid nanoparticles (Liu et al., 2020b).
The combination of in vitro and in vivo studies enables a better understanding of the performance of lipid nanoparticles, linking model systems with actual complex environments, where the formulation needs to dispatch its therapeutic effect. Pramanik and collaborators employed click chemistry to decorate the surface of GMO cubosomes with affimers for targeting colorectal cancer cells, given that the affinity of the protein for carcinoembryonic antigens was expressed by this cell line (Pramanik et al., 2022). Their results showed a significant accumulation of cubosomes in the target area and the delivery of copper acetylacetonate as a model drug.
Application of a formulation on the skin represents a non-invasive and safe route of drug administration (Lai et al., 2020). The stratum corneum (SC) is the outermost layer, and it is formed by corneocytes (protein-enriched dead cells) and lipid sheets (Pyo and Maibach, 2019). A closer examination of SC barriers reveals a brick-and-mortar structure as described by Elias (2012), where the bricks represent non-living corneocyte cells rich in cross-linked keratin and the intracellular mortar is a mixture of lipids organized in lamellar arrays. This architecture resembles a gel state characterized by the low mobility of the lipid alkyl chains.
Underneath this layer are the viable epidermis (EP) and the dermis (DE). As the protein corona hinders the application of a drug carrier in intravenous administration, SC represents a challenging barrier in the administration of drugs. The alternation of hydrophilic and hydrophobic layers in the SC strongly restricts the diffusion of drugs through it (Lai et al., 2020).
Indeed, various approaches can be adopted to enhance the penetration of a drug into the skin layers. One way is represented by the use of formulations based on chemical permeation enhancers, e.g., lipids, which disrupt the highly ordered bilayer structures of the intracellular lipids found in SC (Pham et al., 2018).
Phospholipid vesicles were the first carriers tested for such applications, due to their natural resemblance with cell membranes (Lai et al., 2020). On the other hand, non-lamellar liquid crystalline nanoparticles were studied in the last decade as an alternative platform to the vesicles for the dermal/transdermal release of drugs. Even though few examples have been reported so far, hexosomes and cubosomes were reported to deliver antimicrobial peptides (El Zaafarany et al., 2010), drugs (Rapalli et al., 2020), and photosensitizers (Bazylińska et al., 2018).
By overcoming the first line of defense, the repartition coefficient of the drug decides its fate, allowing its therapeutic effect on the skin (dermal release) or reaching the capillaries and entering the systemic route (transdermal release). Chang et al. evaluated the differences between cubosomes and liposomes in terms of the loading of curcumin and of their biological performances in vitro (Chang et al., 2021). The cytotoxicity (against NIH-3T3 and B16-F1 cell lines, suitable for understanding the biocompatibility of a formulation for such application) of curcumin-loaded cubosomes was higher than the one obtained with DSPC liposomes, showing the potency of the bicontinuous cubic phase dispersion as a carrier for poorly water-soluble drugs.
Cubosomes have been quite studied in the past years as suitable nanomedicines for ophthalmology-related diseases. Flak et al. loaded GMO cubosomes stabilized with Pluronic F127 with the anti-cancer drug AT101 to treat glioblastoma multiforme in vitro; their study showed a high loading efficiency of the drug within the formulation with a sustainable release that is able to induce a strong cytotoxic effect against A172 and LN229 cell lines (Flak et al., 2020).
PHYT cubosomes encapsulating latanoprost managed to reduce the intraocular pressure in rabbits in vivo, reaching better performances than a molecular dispersion of the free drug (Bessone et al., 2021). Cubosomal gels containing beclomethasone dipropionate were prepared for the management of uveitis, increasing corneal irrigation after treatment (Gaballa et al., 2020).
The inclusion of edge activators in the formulation can significantly improve the penetration properties. Among them, bile salts have been found to be quite efficient in achieving this result (Lai et al., 2020; Fornasier et al., 2022). We showed that the inclusion of taurocholate in GMO cubosomes shifts the curvature of the interface toward the lamellar phase dispersion (high bile salt content) or the inverse hexagonal phase (low bile salt content), yielding hexosomes suitable for the topical administration of catechins, a natural antioxidant (Fornasier et al., 2021).
Cubosomal gels formed by GMO, Pluronic F127, and CTAB were employed to encapsulate colchicine in order to treat gout, a common inflammatory disease characterized by the deposition of serum urate crystals in synovial fluids and joints. The in vivo studies highlighted that the transdermal application of such nanomedicines improved drug adsorption, with a significant bioavailability of colchicine (Nasr, 2020).
Cubosomes loaded in a hydrogel matrix were formulated using the quality-by-design approach (using software to optimize the formulation) to develop an economically optimized formulation for the topical administration of ketoconazole (Rapalli et al., 2021), a drug having antifungal activity.
A hyaluronic acid-based hydrogel was prepared for accommodating PHYT cubosomes in its matrix and evaluating the drug release profile of diclofenac for topical application (Gradella et al., 2022). The study showed that the cubosomes maintain their inner structure and properties within the hydrogel, opening up new possibilities for complex formulations composed of a polymeric matrix and lipid nanoparticles.
Victorelli et al. prepared GMO/DOTAP cubosomes containing curcumin, a natural antioxidant with anti-inflammatory and anti-cancer properties. Such a formulation was tested against HeLa cell lines in comparison with the free drug formulation and an antiangiogenic effect in vivo by using the CAM model (Damiani et al., 2022).
The application of cubosomes in the field of photodynamic therapy has been very scarcely explored, and only a few papers (all belonging to our group, to the best of our knowledge) are reported in the literature (Bazylińska et al., 2018; Jenni et al., 2020). In our last study, we formulated GMO cubosomes loaded with both inorganic up-converting nanoparticles (UCNPs) and daunorubicin. In this formulation, the UCNPs were the NaYF4 nanoparticles co-doped with Yb3+ and Er3+ rare earth materials, and they served as energy harvesters and diagnostic agents, while daunorubicin was the photosensitizer. Since cubosomes typically possess a negative zeta potential, we used the layer-by-layer technique to cover the cubosomes' surface with subsequent layers of chitosan, a positively charged polymer, DNA, as a model genetic material, and folate-conjugated chitosan to impart these nanoparticles an active targeting for tumor tissues. As a result of the partial superimposition of the daunorubicin absorption spectrum with the visible emission by the UCNPs after NIR excitation, a photodynamic activity was observed because of a singlet oxygen species generation. This is the first example of hybrid cubosomes for NIR-activated photodynamic therapy (Bazylińska et al., 2022).
The oral administration of drugs represents a non-invasive and easy route. Indeed, the age of the patient can hinder the therapy since parameters such as the taste and the smell of the formulation, non-relevant in other administrations, are indeed crucial.
The complex digestive mechanism of the gastrointestinal tract is designed to absorb as many nutrients as possible from our diet. The materials administered orally experience a harsh acidic environment in the stomach (pH 2–3), containing several enzymes, e.g., lipases and proteases (Krieser et al., 2020). The partially digested material in the stomach, chyme, is then passed to the first segment of the small intestine (duodenum), where the pancreatic enzymes and bile salts are released from the common bile duct. The pH rises to 5–6 in the duodenum and is close to neutrality in the mid-to-distal jejunum. Such knowledge is crucial whenever a pH-sensitive carrier is used, keeping in mind the possible phase transition of the structure (Pham et al., 2015). The digestive processes are coordinated to sequentially break down complex nutrients into their building blocks and then selectively absorb these nutritional elements in an organized fashion.
Many efforts have focused on bypassing these harsh environments by encapsulating the drug in carriers. Indeed, the design of the formulation needs to take into account the effect of the lipase. Lipid nanoparticles are suitable for this task, given the natural biodegradability of the lipids.
The group of Boyd extensively studied the application of non-lamellar lipid nanoparticles (especially cubosomes) for oral administration (Nguyen et al., 2011; Boyd et al., 2019). PHYT cubosomes showed good delivering properties for intestine targeting (Porter et al., 2004; Nielsen et al., 2017), while GMO cubosomes are more labile due to their lipase actions and are, thereby, efficient for stomach targeting (Negrini and Mezzenga, 2011). This finding is related to the activity of lipases that are not able to hydrolyze PHYT, in comparison with GMOs.
Another aspect to be considered for oral administration is the strong taste of several drugs, which hinders their therapeutic application in pediatric patients. Aiming to improve the administration properties of cefpodoxime, a common antibiotic for children, Fan and coauthors prepared a taste-masking cubosome formulation, and its taste-making ability was confirmed by using an electronic tongue, reaching better performance in comparison with other commercial products (Fan et al., 2020). These results show the efficacy of drug encapsulation within a cubosome formulation, removing a common drawback (the strong taste) but ensuring the efficacy of the therapy.
Since cubosomes enable the encapsulation of poorly water-soluble drugs and can be engineered with specific functionalities, they are proposed as suitable candidates as nanomedicines. The variety of applications of such formulations shows their potency and versatility. Moreover, given their bigger water channel diameters, hexosomes and sponge phase nanoparticles can ensure the immobilization of large macromolecules (Meli et al., 2015; Meli et al., 2017; Barriga et al., 2019; Gilbert et al., 2019; Murgia et al., 2020). Even though hexosomes and spongosomes have been studied for more than 20 years, few papers have been published in comparison with cubosomes. For instance, most of the research papers devoted to spongosomes are related to their physicochemical aspects, while their biological impact remains poorly understood, and studies on their possible use as nanomedicines are missing. Furthermore, their surface/interfacial properties lack proper investigation. While cubosomes were investigated early under this aspect (Larsson, 1983; Hyde et al., 1984; Neto et al., 1999), hexosomes and sponge nanoparticles surface are yet to be probed in a systematic manner on bare surfaces (hydrophilic and hydrophobic) and on model membranes. This appears as one of the most important missing links between the physicochemical features and the biological/medical applications of these nanoparticles. In general, as underlined by many reviews (Biffi et al., 2017; Tran et al., 2017; Zhai et al., 2020b), only a limited number of research papers have appeared presenting in vitro and in vivo investigations on non-lamellar nanoparticles, and this is, especially, true for hexosomes and spongosomes. As a consequence, important aspects, such as the nanostructure relationship with nanoparticles’ cellular uptake, remain rather obscure and yet to be fully clarified (Bor et al., 2022b), not allowing the drawing of fundamental conclusions on the fate of these nanoparticles once administered and on their effect on our bodies. In addition, non-lamellar nanoparticles are often proposed as possible alternatives to liposomes, so the question arises in which cases they may be superior. Due to the higher lipid volume convoluted in the space in non-lamellar nanoparticles, they certainly allow greater encapsulation of drugs per unit of volume, but a control sample of vesicles is usually missing as a comparison in most of the studies, making it harder to assess the best option of treatment between lamellar or non-lamellar formulations.
All the nanoparticles discussed in this review, either lamellar, cubic, sponge, or hexagonal, exhibit peculiar aspects that place them, in terms of use, in the pharmaceutical area of controlled drug release for various pathologies. Definitely, each of them has intrinsic advantages and disadvantages, ultimately related to the physics and the chemistry of the molecules used for their preparation. A clarifying example could be the better performance shown by the non-hydrolyzable PHYT compared to GMO in oral drug administration (see Section 6.3), although it is commonly found more cytotoxic than GMO. However, rather than simple limitations, such disadvantages should be considered florid fields of research where investigations are needed to clarify the biological role of the components of these nanoparticles, which is well beyond the physicochemical properties of the nanostructure they originate when dispersed in water.
MF conducted the bibliographic research and wrote the first draft of the review. SM supervised the work and edited the review.
The authors declare that the research was conducted in the absence of any commercial or financial relationships that could be construed as a potential conflict of interest.
All claims expressed in this article are solely those of the authors and do not necessarily represent those of their affiliated organizations, or those of the publisher, the editors, and the reviewers. Any product that may be evaluated in this article, or claim that may be made by its manufacturer, is not guaranteed or endorsed by the publisher.
Akhlaghi, S. P., Ribeiro, I. R., Boyd, B. J., and Loh, W. (2016). Impact of preparation method and variables on the internal structure, morphology, and presence of liposomes in phytantriol-Pluronic® F127 cubosomes. Colloids Surfaces B Biointerfaces 145, 845–853. doi:10.1016/j.colsurfb.2016.05.091
Aleandri, S., and Mezzenga, R. (2020). The physics of lipidic mesophase delivery systems. Phys. Today 73 (7), 38–44. doi:10.1063/pt.3.4522
Angelov, B., Angelova, A., Mutafchieva, R., Lesieur, S., Vainio, U., Garamus, V. M., et al. (2011). SAXS investigation of a cubic to a sponge (L3) phase transition in self-assembled lipid nanocarriers. Phys. Chem. Chem. Phys. 13 (8), 3073–3081. doi:10.1039/c0cp01029d
Azhari, H., Younus, M., Hook, S. M., Boyd, B. J., and Rizwan, S. B. (2021). Cubosomes enhance drug permeability across the blood – brain barrier in zebrafish. Int. J. Pharm. [Internet] 600, 120411. doi:10.1016/j.ijpharm.2021.120411
Azmi, I. D. M., Wibroe, P. P., Wu, L. P., Kazem, A. I., Amenitsch, H., Moghimi, S. M., et al. (2016). A structurally diverse library of safe-by-design citrem-phospholipid lamellar and non-lamellar liquid crystalline nano-assemblies. J. Control Release 239, 1–9. doi:10.1016/j.jconrel.2016.08.011
Balestri, A., Lonetti, B., Harrisson, S., Farias-Mancilla, B., Zhang, J., Amenitsch, H., et al. (2022). Thermo-responsive lipophilic NIPAM-based block copolymers as stabilizers for lipid-based cubic nanoparticles. Colloids Surfaces B Biointerfaces [Internet] 220, 112884. doi:10.1016/j.colsurfb.2022.112884
Barenholz, Y. (2012). Doxil® - the first FDA-approved nano-drug: Lessons learned. J. Control Release 160 (2), 117–134. doi:10.1016/j.jconrel.2012.03.020
Barriga, H. M. G., Holme, M. N., and Stevens, M. M. (2019). Cubosomes: The next generation of smart lipid nanoparticles? Angew. Chem. - Int. Ed. 58 (10), 2958–2978. doi:10.1002/anie.201804067
Bazylińska, U., Kulbacka, J., Schmidt, J., Talmon, Y., and Murgia, S. (2018). Polymer-free cubosomes for simultaneous bioimaging and photodynamic action of photosensitizers in melanoma skin cancer cells. J. Colloid Interface Sci. 522, 163–173. doi:10.1016/j.jcis.2018.03.063
Bazylińska, U., Wawrzyńczyk, D., Kulbacka, J., Picci, G., Manni, L. S., Handschin, S., et al. (2022). Hybrid theranostic cubosomes for efficient NIR-induced photodynamic therapy. ACS Nano 16 (4), 5427–5438. doi:10.1021/acsnano.1c09367
Beattie, J. K., and Djerdjev, A. M. (2004). The pristine oil/water interface: Surfactant-free hydroxide-charged emulsions. Angew. Chem. - Int. Ed. 43 (27), 3568–3571. doi:10.1002/anie.200453916
Bessone, C. D. V., Akhlaghi, S. P., Tártara, L. I., Quinteros, D. A., Loh, W., and Allemandi, D. A. (2021). Latanoprost-loaded phytantriol cubosomes for the treatment of glaucoma. Eur. J. Pharm. Sci. 160, 105748. doi:10.1016/j.ejps.2021.105748
Biffi, S., Andolfi, L., Caltagirone, C., Garrovo, C., Falchi, A. M., Lippolis, V., et al. (2017). Cubosomes for in vivo fluorescence lifetime imaging. Nanotechnology 28 (5), 055102. doi:10.1088/1361-6528/28/5/055102
Biffi, S., Voltan, R., Rampazzo, E., Prodi, L., Zauli, G., and Secchiero, P. (2015). Applications of nanoparticles in cancer medicine and beyond: Optical and multimodal in vivo imaging, tissue targeting and drug delivery. Expert Opin. Drug Deliv. 12 (12), 1837–1849. doi:10.1517/17425247.2015.1071791
Blanazs, A., Armes, S. P., and Ryan, A. J. (2009). Self-assembled block copolymer aggregates: From micelles to vesicles and their biological applications. Macromol. Rapid Commun. 30 (4–5), 267–277. doi:10.1002/marc.200800713
Boge, L., Browning, K. L., Nordström, R., Campana, M., Damgaard, L. S. E., Seth Caous, J., et al. (2019). Peptide-loaded cubosomes functioning as an antimicrobial unit against Escherichia coli. ACS Appl. Mater Interfaces 11 (24), 21314–21322. doi:10.1021/acsami.9b01826
Boge, L., Umerska, A., Matougui, N., Bysell, H., Ringstad, L., Davoudi, M., et al. (2017). Cubosomes post-loaded with antimicrobial peptides: Characterization, bactericidal effect and proteolytic stability. Int. J. Pharm. [Internet] 526 (1–2), 400–412. doi:10.1016/j.ijpharm.2017.04.082
Bor, G., Lin, J. H., Lin, K. Y., Chen, H. C., Prajnamitra, R. P., Salentinig, S., et al. (2022). PEGylation of phosphatidylglycerol/docosahexaenoic acid hexosomes with d -α-Tocopheryl succinate poly(ethylene glycol)2000Induces morphological transformation into vesicles with prolonged circulation times. ACS Appl. Mater Interfaces 14, 48449–48463. doi:10.1021/acsami.2c14375
Bor, G., Salentinig, S., Şahin, E., Nur Ödevci, B., Roursgaard, M., Liccardo, L., et al. (2022). Cell medium-dependent dynamic modulation of size and structural transformations of binary phospholipid/ω-3 fatty acid liquid crystalline nano-self-assemblies: Implications in interpretation of cell uptake studies. J. Colloid Interface Sci. 606, 464–479. doi:10.1016/j.jcis.2021.07.149
Borné, J., Nylander, T., and Khan, A. (2002). Effect of lipase on monoolein-based cubic phase dispersion (cubosomes) and vesicles. J. Phys. Chem. B 106 (40), 10492–10500. doi:10.1021/jp021023y
Boyd, B. J., Bergström, C. A. S., Vinarov, Z., Kuentz, M., Brouwers, J., Augustijns, P., et al. (2019). Successful oral delivery of poorly water-soluble drugs both depends on the intraluminal behavior of drugs and of appropriate advanced drug delivery systems. Eur. J. Pharm. Sci. 137, 104967. doi:10.1016/j.ejps.2019.104967
Caltagirone, C., Arca, M., Falchi, A. M., Lippolis, V., Meli, V., Monduzzi, M., et al. (2015). Solvatochromic fluorescent BODIPY derivative as imaging agent in camptothecin loaded hexosomes for possible theranostic applications. RSC Adv. 5 (30), 23443–23449. doi:10.1039/c5ra01025j
Chang, C., Meikle, T. G., Drummond, C. J., Yang, Y., and Conn, C. E. (2021). Comparison of cubosomes and liposomes for the encapsulation and delivery of curcumin. Soft Matter 17 (12), 3306–3313. doi:10.1039/d0sm01655a
Chen, H., and Li, M. (2021). Recent progress in polymer cubosomes and hexosomes. Macromol. Rapid Commun. 42, e2100194. doi:10.1002/marc.202100194
Chen, Q., Xu, S., Liu, Q., Masliyah, J., and Xu, Z. (2016). QCM-D study of nanoparticle interactions. Adv. Colloid Interface Sci. 233, 94–114. doi:10.1016/j.cis.2015.10.004
Chen, Y., Angelova, A., Angelov, B., Drechsler, M., Garamus, V. M., Willumeit-Römer, R., et al. (2015). Sterically stabilized spongosomes for multidrug delivery of anticancer nanomedicines. J. Mater Chem. B 3 (39), 7734–7744. doi:10.1039/c5tb01193k
Chong, J. Y. T., Mulet, X., Boyd, B. J., and Drummond, C. J. (2015). Steric stabilizers for cubic phase lyotropic liquid crystal nanodispersions (cubosomes) [internet], Adv. Planar Lipid Bilayers Liposomes 21. 131–187. doi:10.1016/bs.adplan.2014.11.001
Crommelin, D. J. A., van Hoogevest, P., and Storm, G. (2020). The role of liposomes in clinical nanomedicine development. What now? Now what? J. Control Release [Internet] 318, 256–263. doi:10.1016/j.jconrel.2019.12.023
Cytryniak, A., Nazaruk, E., Bilewicz, R., Emilia, G., Walczak, R., Mames, A., et al. (2020). Lipidic cubic-phase nanoparticles (cubosomes) loaded with doxorubicin and labeled with 177 Lu as a potential tool for combined chemo and internal radiotherapy for cancers. Nanomater. (Basel) 10, 2272. doi:10.3390/nano10112272
Damiani, F., Salvati, L., Biffi, S., Bortot, B., Harb, H., Lutz-bueno, V., et al. (2022). Potential of curcumin-loaded cubosomes for topical treatment of cervical cancer. J. Colloid Interface Sci. [Internet] 620, 419–430. doi:10.1016/j.jcis.2022.04.031
Demurtas, D., Guichard, P., Martiel, I., Mezzenga, R., Hébert, C., and Sagalowicz, L. (2015). Direct visualization of dispersed lipid bicontinuous cubic phases by cryo-electron tomography. Nat. Commun. 6, 8915–8918. doi:10.1038/ncomms9915
Driever, C. D., Mulet, X., Waddington, L. J., Postma, A., Thissen, H., Caruso, F., et al. (2013). Layer-by-layer polymer coating on discrete particles of cubic lyotropic liquid crystalline dispersions (cubosomes). Langmuir 29 (42), 12891–12900. doi:10.1021/la401660h
Dyett, B. P., Yu, H., Sarkar, S., Strachan, J. B., Drummond, C. J., and Conn, C. E. (2021). Uptake dynamics of cubosome nanocarriers at bacterial surfaces and the routes for cargo internalization. ACS Appl. Mater Interfaces 13 (45), 53530–53540. doi:10.1021/acsami.1c09909
Dyett, B. P., Yu, H., Strachan, J., Drummond, C. J., and Conn, C. E. (2019). Fusion dynamics of cubosome nanocarriers with model cell membranes. Nat. Commun. 10 (1), 4492. doi:10.1038/s41467-019-12508-8
El Zaafarany, G. M., Awad, G. A. S., Holayel, S. M., and Mortada, N. D. (2010). Role of edge activators and surface charge in developing ultradeformable vesicles with enhanced skin delivery. Int. J. Pharm. [Internet] 397 (1–2), 164–172. doi:10.1016/j.ijpharm.2010.06.034
Elias, P. M. (2012). Structure and function of the stratum corneum extracellular matrix. J. Invest. Dermatol 132 (9), 2131–2133. doi:10.1038/jid.2012.246
Ellipsometry, F. H. (2015). Handbook of optical metrology: Principles and applications. United Kingdom: Taylor & Francis.
Fahmy, U. A., Fahmy, O., and Alhakamy, N. A. (2021). Optimized icariin cubosomes exhibit augmented cytotoxicity against SKOV-3 ovarian cancer cells. Pharmaceutics 13, 20. doi:10.3390/pharmaceutics13010020
Fan, Y., Chen, H., Huang, Z., Zhu, J., Wan, F., Peng, T., et al. (2020). Taste-masking and colloidal-stable cubosomes loaded with Cefpodoxime proxetil for pediatric oral delivery. Int. J. Pharm. [Internet] 575, 118875. doi:10.1016/j.ijpharm.2019.118875
Flak, D. K., Adamski, V., Nowaczyk, G., Szutkowski, K., Synowitz, M., Jurga, S., et al. (2020). <p>AT101-Loaded Cubosomes as an Alternative for Improved Glioblastoma Therapy</p>. Int. J. Nanomedicine 15, 7415–7431. doi:10.2147/ijn.s265061
Fong, C., Zhai, J., John, C., and Tran, N. (2020). Micellar Fd3m cubosomes from monoolein – Long chain unsaturated fatty acid mixtures: Stability on temperature and pH response. J. Colloid Interface Sci. [Internet] 566, 98–106. doi:10.1016/j.jcis.2020.01.041
Fornasier, M., Biffi, S., Bortot, B., Macor, P., Manhart, A., Wurm, F. R., et al. (2020). Cubosomes stabilized by a polyphosphoester-analog of Pluronic F127 with reduced cytotoxicity. J. Colloid Interface Sci. [Internet] 580, 286–297. doi:10.1016/j.jcis.2020.07.038
Fornasier, M., Dessì, F., Pireddu, R., Sinico, C., Carretti, E., and Murgia, S. (2022). Lipid vesicular gels for topical administration of antioxidants. Colloids Surfaces B Biointerfaces [Internet] 213, 112388. doi:10.1016/j.colsurfb.2022.112388
Fornasier, M., Pireddu, R., Del Giudice, A., Sinico, C., Nylander, T., Schillén, K., et al. (2021). Tuning lipid structure by bile salts: Hexosomes for topical administration of catechin. Colloids Surfaces B Biointerfaces 199, 111564. doi:10.1016/j.colsurfb.2021.111564
Francia, V., Yang, K., Deville, S., Reker-Smit, C., Nelissen, I., and Salvati, A. (2019). Corona composition can affect the mechanisms cells use to internalize nanoparticles. ACS Nano 13, 11107–11121. doi:10.1021/acsnano.9b03824
Fraser, S. J., Mulet, X., Martin, L., Praporski, S., Mechler, A., Hartley, P. G., et al. (2012). Surface immobilization of bio-functionalized cubosomes: Sensing of proteins by quartz crystal microbalance. Langmuir 28 (1), 620–627. doi:10.1021/la2032994
Gaballa, S. A., Garhy, O. H., Moharram, H., and Abdelkader, H. (2020). Preparation and evaluation of cubosomes/cubosomal gels for ocular delivery of beclomethasone dipropionate for management of uveitis. Pharm. Res. 37, 198. doi:10.1007/s11095-020-02857-1
Gajda, E., Godlewska, M., Mariak, Z., and Nazaruk, E. (2020). Combinatory treatment with miR-7-5p and drug-loaded cubosomes E ff ectively impairs cancer cells. Int. J. Mol. Sci. 21, 5039. doi:10.3390/ijms21145039
Gilbert, J., Valldeperas, M., Dhayal, S. K., Barauskas, J., Dicko, C., and Nylander, T. (2019). Immobilisation of β-galactosidase within a lipid sponge phase: Structure, stability and kinetics characterisation. Nanoscale 11, 21291–21301. doi:10.1039/c9nr06675f
Glatter, O. (2018). Scattering methods and their application in colloid and interface science. Scattering Methods and their Application in Colloid and Interface Science. Shadow Health: Elsevier.
Gradella, D., Giometti, C., and Loh, W. (2022). Colloids and Surfaces B: Biointerfaces Characterization of cubosomes immobilized in hydrogels of hyaluronic acid and their use for diclofenac controlled delivery. Colloids Surfaces B Biointerfaces 212, 112352. doi:10.1016/j.colsurfb.2022.112352
Gustafsson, J., Ljusberg-Wahren, H., Almgren, M., and Larsson, K. (1997). Submicron particles of reversed lipid phases in water stabilized by a nonionic amphiphilic polymer. Langmuir 13 (26), 6964–6971. doi:10.1021/la970566+
Ha, S., La, Y., and Kim, K. T. (2020). Polymer cubosomes: In fi nite cubic mazes and possibilities. Acc. Chem. Res. 53, 620–631. doi:10.1021/acs.accounts.9b00563
Hyde, S. T., Andersson, S., Ericsson, B., and Larsson, K. (1984). A cubic structure consisting of a lipid bilayer forming an infinite periodic minimum surface of the gyroid type in the glycerolmonooleat-water system. Z. fur Krist. - New Cryst. Struct. 168, 213–219. doi:10.1524/zkri.1984.168.14.213
Jabłonowska, E., Matyszewska, D., Nazaruk, E., Godlewska, M., Gaweł, D., and Bilewicz, R. (2021). Lipid membranes exposed to dispersions of phytantriol and monoolein cubosomes: Langmuir monolayer and HeLa cell membrane studies. BBA - Gen. Subj. [Internet] 1865 (1), 129738. doi:10.1016/j.bbagen.2020.129738
Jackman, J. A., and Cho, N. J. (2020). Supported lipid bilayer formation: Beyond vesicle fusion. Langmuir 36 (6), 1387–1400. doi:10.1021/acs.langmuir.9b03706
Jenni, S., Picci, G., Fornasier, M., Mamusa, M., Schmidt, J., Talmon, Y., et al. (2020). Multifunctional cubic liquid crystalline nanoparticles for chemo- A nd photodynamic synergistic cancer therapy. Photochem Photobiol. Sci. 19, 674–680. doi:10.1039/c9pp00449a
Knapek, E., and Dubochet, J. (1980). Beam damage to organic material is considerably reduced in cryo-electron microscopy. J. Mol. Biol. 141, 147–161. doi:10.1016/0022-2836(80)90382-4
Krieser, K., Emanuelli, J., Daudt, R. M., Bilatto, S., Willig, J. B., Guterres, S. S., et al. (2020). Taste-masked nanoparticles containing Saquinavir for pediatric oral administration. Mater Sci. Eng. C 117, 111315. doi:10.1016/j.msec.2020.111315
Kulkarni, C. V., Wachter, W., Iglesias-Salto, G., Engelskirchen, S., and Ahualli, S. (2011). Monoolein: A magic lipid? Phys. Chem. Chem. Phys. 13 (8), 3004–3021. doi:10.1039/c0cp01539c
Lai, F., Caddeo, C., Manca, M. L., Manconi, M., Sinico, C., and Fadda, A. M. (2020). What’s new in the field of phospholipid vesicular nanocarriers for skin drug delivery. Int. J. Pharm. 583, 119398. doi:10.1016/j.ijpharm.2020.119398
Lai, X., Han, M., Ding, Y., Chow, S. H., Brun, A. P., Wu, C., et al. (2022). A polytherapy based approach to combat antimicrobial resistance using cubosomes. Nat. Commun. 13, 343. doi:10.1038/s41467-022-28012-5
Lammers, T., Aime, S., Hennink, W. E., Storm, G., and Kiessling, F. (2011). Theranostic nanomedicine. Theranostic Nanomedicine. Acc. Chem. Res. 44, 1029–1038. doi:10.1021/ar200019c
Larsson, K. (1983). Two cubic phases in monoolein-water system. Nature 304, 664. doi:10.1038/304664c0
Lesniak, A., Salvati, A., Santos-Martinez, M. J., Radomski, M. W., Dawson, K. A., and Åberg, C. (2013). Nanoparticle adhesion to the cell membrane and its effect on nanoparticle uptake efficiency. J. Am. Chem. Soc. 135, 1438–1444. doi:10.1021/ja309812z
Liu, Z., Ni, H., Yu, L., Xu, S., Bo, R., Qiu, T., et al. (2020). Adjuvant activities of CTAB-modified Polygonatum sibiricum polysaccharide cubosomes on immune responses to ovalbumin in mice. Int. J. Biol. Macromol. [Internet] 148, 793–801. doi:10.1016/j.ijbiomac.2020.01.174
Liu, Z., Yu, L., Gu, P., Bo, R., Xu, S., Wusiman, A., et al. (2020). <p>Surface-Engineered cubosomes serve as a novel vaccine adjuvant to modulate innate immunity and improve adaptive immunity in vivo</p>. Int. J. Nanomedicine 15, 8595–8608. doi:10.2147/ijn.s266165
Longley, W., and McIntosh, T. J. (1983). A bicontinuous tetrahedral structure in a liquid-crystalline lipid. Nature 303 (5918), 612–614. doi:10.1038/303612a0
Malheiros, B., Dias, R., Castro, D., Lotierzo, M. C., Casadei, B. R., Mariani, P., et al. (2022). Aspects influence of hexadecylphosphocholine (miltefosine) in phytantriol-based cubosomes: A structural investigation. Colloids Surfaces A Physicochem. Eng. 632, 127720. doi:10.1016/j.colsurfa.2021.127720
Meikle, T. G., Dharmadana, D., Hoffmann, S. V., Jones, N. C., Drummond, C. J., and Conn, C. E. (2021). Analysis of the structure, loading and activity of six antimicrobial peptides encapsulated in cubic phase lipid nanoparticles. J. Colloid Interface Sci. 587, 90–100. doi:10.1016/j.jcis.2020.11.124
Meli, V., Caltagirone, C., Falchi, A. M., Hyde, S. T., Lippolis, V., Monduzzi, M., et al. (2015). Docetaxel-loaded fluorescent liquid-crystalline nanoparticles for cancer theranostics. Langmuir 31 (35), 9566–9575. doi:10.1021/acs.langmuir.5b02101
Meli, V., Caltagirone, C., Sinico, C., Lai, F., Falchi, A. M., Monduzzi, M., et al. (2017). Theranostic hexosomes for cancer treatments: An in vitro study. New J. Chem. 41 (4), 1558–1565. doi:10.1039/c6nj03232j
Mezzenga, R., Seddon, J. M., Drummond, C. J., Boyd, B. J., Schröder-Turk, G. E., and Sagalowicz, L. (2019). Nature-inspired design and application of lipidic lyotropic liquid crystals. Adv. Mater 31 (35), 1900818–1900819. doi:10.1002/adma.201900818
Mohammad, Y., Fallah, A. B., Reynolds, J. N. J., Boyd, B. J., and Rizwan, S. B. (2020). Colloids and Surfaces B: Biointerfaces Steric stabilisers govern the colloidal and chemical stability but not in vitro cellular toxicity of linoleoylethanolamide cubosomes. Colloids Surfaces B Biointerfaces [Internet 192, 111063. doi:10.1016/j.colsurfb.2020.111063
Mokhtar, S., Khattab, S. N., Elkhodairy, K. A., and Teleb, M. (2022). Methotrexate-lactoferrin targeted exemestane cubosomes for synergistic breast cancer therapy. Front. Chem. 10, 847573. doi:10.3389/fchem.2022.847573
Mulet, X., Boyd, B. J., and Drummond, C. J. (2013). Advances in drug delivery and medical imaging using colloidal lyotropic liquid crystalline dispersions. J. Colloid Interface Sci. [Internet] 393 (1), 1–20. doi:10.1016/j.jcis.2012.10.014
Muller, F., Salonen, A., and Glatter, O. (2010). Phase behavior of Phytantriol/water bicontinuous cubic Pn3m cubosomes stabilized by Laponite disc-like particles. J. Colloid Interface Sci. [Internet] 342 (2), 392–398. doi:10.1016/j.jcis.2009.10.054
Murgia, S., Biffi, S., and Mezzenga, R. (2020). Recent advances of non-lamellar lyotropic liquid crystalline nanoparticles in nanomedicine. Curr. Opin. Colloid Interface Sci. 48, 28–39. doi:10.1016/j.cocis.2020.03.006
Murgia, S., Bonacchi, S., Falchi, A. M., Lampis, S., Lippolis, V., Meli, V., et al. (2013). Drug-loaded fluorescent cubosomes: Versatile nanoparticles for potential theranostic applications. Langmuir 29, 6673–6679. doi:10.1021/la401047a
Naidjonoka, P., Fornasier, M., Pålsson, D., Rudolph, G., Al-Rudainy, B., Murgia, S., et al. (2021). Bicontinuous cubic liquid crystalline phase nanoparticles stabilized by softwood hemicellulose. Colloids Surfaces B Biointerfaces [Internet] 203, 111753. doi:10.1016/j.colsurfb.2021.111753
Nakano, M., Sugita, A., Matsuoka, H., and Handa, T. (2001). Small-angle X-ray scattering and 13C NMR investigation on the internal structure of “cubosomes. Langmuir 17 (13), 3917–3922. doi:10.1021/la010224a
Nasr, M. (2020). Formulation and evaluation of cubosomes containing colchicine for transdermal delivery. Drug Deliv. Transl. Res. 10, 13021302–13131313. doi:10.1007/s13346-020-00785-6
Negrini, R., and Mezzenga, R. (2011). PH-responsive lyotropic liquid crystals for controlled drug delivery. Langmuir 27 (9), 5296–5303. doi:10.1021/la200591u
Neto, C., Aloisi, G., Baglioni, P., and Larsson, K. (1999). Imaging soft matter with the atomic force microscope: Cubosomes and hexosomes. J. Phys. Chem. B 103, 3896–3899. doi:10.1021/jp984551b
Nguyen, T. H., Hanley, T., Porter, C. J. H., and Boyd, B. J. (2011). Nanostructured liquid crystalline particles provide long duration sustained-release effect for a poorly water soluble drug after oral administration. J. Control Release 153 (2), 180–186. doi:10.1016/j.jconrel.2011.03.033
Nielsen, L. H., Rades, T., Boyd, B., and Boisen, A. (2017). Microcontainers as an oral delivery system for spray dried cubosomes containing ovalbumin. Eur. J. Pharm. Biopharm. [Internet] 118, 13–20. doi:10.1016/j.ejpb.2016.12.008
Patil, S. M., Sawant, S. S., and Kunda, N. K. (2021). Inhalable bedaquiline-loaded cubosomes for the treatment of non-small cell lung cancer (NSCLC). Int. J. Pharm. [Internet] 607, 121046. doi:10.1016/j.ijpharm.2021.121046
Pham, A. C., Nguyen, T. H., Nowell, C. J., Graham, B., and Boyd, B. J. (2015). Examining the gastrointestinal transit of lipid-based liquid crystalline systems using whole-animal imaging. Drug Deliv. Transl. Res. 5, 566–574. doi:10.1007/s13346-015-0253-z
Pham, Q. D., Mojumdar, E. H., Gooris, G. S., Bouwstra, J. A., Sparr, E., and Topgaard, D. (2018). Solid and fluid segments within the same molecule of stratum corneum ceramide lipid. Q. Rev. Biophys. 51, e7. doi:10.1017/s0033583518000069
Porter, C. J. H., Kaukonen, A. M., Taillardat-Bertschinger, A., Boyd, B. J., O’Connor, J. M., Edwards, G. A., et al. (2004). Use of in vitro lipid digestion data to explain the in vivo performance of triglyceride-based oral lipid formulations of poorly water-soluble drugs: Studies with halofantrine. J. Pharm. Sci. 93 (5), 1110–1121. doi:10.1002/jps.20039
Pramanik, A., Xu, Z., Shamsuddin, S. H., Khaled, Y. S., Ingram, N., Maisey, T., et al. (2022). Affimer tagged cubosomes: Targeting of carcinoembryonic antigen expressing colorectal cancer cells using in vitro and in vivo models. ACS Appl. Mater Interfaces 14, 11078–11091. doi:10.1021/acsami.1c21655
Ptissam, B. (2021). Fluorescent polymer cubosomes and hexosomes with aggregation-induced emission. Chem. Sci. 22 (1), 5495–5504. doi:10.1039/d1sc00270h
Pyo, S. M., and Maibach, H. I. (2019). Skin metabolism: Relevance of skin enzymes for rational drug design. Skin. Pharmacol. Physiol. 32 (5), 283–294. doi:10.1159/000501732
Rajesh, S., Zhai, J., Drummond, C. J., and Tran, N. (2021). Synthetic ionizable aminolipids induce a pH dependent inverse hexagonal to bicontinuous cubic lyotropic liquid crystalline phase transition in monoolein nanoparticles. J. Colloid Interface Sci. [Internet] 589, 85–95. doi:10.1016/j.jcis.2020.12.060
Rapalli, V. K., Banerjee, S., Khan, S., Jha, P. N., Gupta, G., Dua, K., et al. (2021). QbD-driven formulation development and evaluation of topical hydrogel containing ketoconazole loaded cubosomes. Mater Sci. Eng. C [Internet] 119, 111548. doi:10.1016/j.msec.2020.111548
Rapalli, V. K., Waghule, T., Hans, N., Mahmood, A., Gorantla, S., Dubey, S. K., et al. (2020). Insights of lyotropic liquid crystals in topical drug delivery for targeting various skin disorders. J. Mol. Liq. 315, 113771. doi:10.1016/j.molliq.2020.113771
Rodrigues, L., Raftopoulos, K. N., Tandrup Schmidt, S., Schneider, F., Dietz, H., Rades, T., et al. (2018). Immune responses induced by nano-self-assembled lipid adjuvants based on a monomycoloyl glycerol analogue after vaccination with the Chlamydia trachomatis major outer membrane protein. J. Control Release [Internet] 285, 12–22. doi:10.1016/j.jconrel.2018.06.028
Sahdev, P., Ochyl, L. J., and Moon, J. J. (2014). Biomaterials for nanoparticle vaccine delivery systems. Pharm. Res. 31 (10), 2563–2582. doi:10.1007/s11095-014-1419-y
Salentinig, S., Sagalowicz, L., and Glatter, O. (2010). Self-assembled structures and pKa value of oleic acid in systems of biological relevance. Langmuir 26, 11670–11679. doi:10.1021/la101012a
Siegel, D. P., Burns, J. L., Chestnut, M. H., and Talmon, Y. (1989). Intermediates in membrane fusion and bilayer/nonbilayer phase transitions imaged by time-resolved cryo-transmission electron microscopy. Biophys. J. 56 (1), 161–169. doi:10.1016/s0006-3495(89)82661-x
Suk, J. S., Xu, Q., Kim, N., Hanes, J., and Ensign, L. M. (2016). PEGylation as a strategy for improving nanoparticle-based drug and gene delivery. Adv. Drug Deliv. Rev. 99, 28–51. doi:10.1016/j.addr.2015.09.012
Tajik-Ahmadabad, B., Mechler, A., Muir, B. W., McLean, K., Hinton, T. M., Separovic, F., et al. (2017). A QCM-D and SAXS study of the interaction of functionalised lyotropic liquid crystalline lipid nanoparticles with siRNA. ChemBioChem 18 (10), 921–930. doi:10.1002/cbic.201600613
Tan, C., Hosseini, S. F., and Jafari, S. M. (2022). Cubosomes and hexosomes as novel nanocarriers for bioactive compounds. J. Agric. Food Chem. 70, 1423–1437. doi:10.1021/acs.jafc.1c06747
Tran, N., Bye, N., Moffat, B. A., Wright, D. K., Cuddihy, A., Hinton, T. M., et al. (2017). Dual-modality NIRF-MRI cubosomes and hexosomes: High throughput formulation and in vivo biodistribution. Mater Sci. Eng. C [Internet] 71, 584–593. doi:10.1016/j.msec.2016.10.028
Valldeperas, M., Dabkowska, A. P., Pálsson, G. K., Rogers, S., Mahmoudi, N., Carnerup, A., et al. (2019). Interfacial properties of lipid sponge-like nanoparticles and the role of stabilizer on particle structure and surface interactions. Soft Matter [Internet] 15 (10), 2178–2189. doi:10.1039/C8SM02634C
Valldeperas, M., Wiśniewska, M., Ram-On, M., Kesselman, E., Danino, D., Nylander, T., et al. (2016). Sponge phases and nanoparticle dispersions in aqueous mixtures of mono- and diglycerides. Langmuir 32 (34), 8650–8659. doi:10.1021/acs.langmuir.6b01356
Varghese, R., Salvi, S., Sood, P., Kulkarni, B., and Kumar, D. (2022). Cubosomes in cancer drug delivery: A review. Colloid Interface Sci. Commun. [Internet] 46, 100561. doi:10.1016/j.colcom.2021.100561
Wu, Y., Cui, J., Ling, Y., Wang, X., Fu, J., Jing, C., et al. (2022). Polypyrrole cubosomes with ordered ultralarge mesopore for controllable encapsulation and release of albumin. Nano Lett. 22, 3685–3690. doi:10.1021/acs.nanolett.2c00330
Yaghmur, A., and Glatter, O. (2009). Characterization and potential applications of nanostructured aqueous dispersions. Adv. Colloid Interface Sci. 147-148, 333–342. doi:10.1016/j.cis.2008.07.007
Yaghmur, A., and Mu, H. (2021). Recent advances in drug delivery applications of cubosomes, hexosomes, and solid lipid nanoparticles. Acta Pharm. Sin. B [Internet] 11 (4), 871–885. doi:10.1016/j.apsb.2021.02.013
Zabara, A., and Mezzenga, R. (2014). Controlling molecular transport and sustained drug release in lipid-based liquid crystalline mesophases. J. Control. Release 188, 31–43. doi:10.1016/j.jconrel.2014.05.052
Zhai, J., Fong, C., Tran, N., and Drummond, C. J. (2019). Non-lamellar lyotropic liquid crystalline lipid nanoparticles for the next generation of nanomedicine. ACS Nano 13 (6), 6178–6206. doi:10.1021/acsnano.8b07961
Zhai, J., Sarkar, S., Conn, C. E., and Drummond, C. J. (2020). Molecular engineering of super-swollen inverse bicontinuous cubic and sponge lipid phases for biomedical applications. Mol. Syst. Des. Eng. 5 (8), 1354–1375. doi:10.1039/d0me00076k
Zhai, J., Tan, F. H., Luwor, R. B., Reddy, T. S., Ahmed, N., Drummond, C. J., et al. (2020). Vitro and in vivo toxicity and biodistribution of paclitaxel-loaded cubosomes as a drug delivery nanocarrier: A case study using an A431 skin cancer xenograft model. ACS Appl. Bio Mater 3, 4198–4207. doi:10.1021/acsabm.0c00269
Zhang, B., and Wang, Q. (2012). “Quartz crystal microbalance with dissipation,” in Nanotechnology research methods for foods and bioproducts (New Delhi: Acs Publisher).
Keywords: cubosomes, hexosomes, sponge nanoparticles, non-lamellar, drug delivery, drug administration
Citation: Fornasier M and Murgia S (2023) Non-lamellar lipid liquid crystalline nanoparticles: A smart platform for nanomedicine applications. Front. Soft. Matter 3:1109508. doi: 10.3389/frsfm.2023.1109508
Received: 27 November 2022; Accepted: 15 February 2023;
Published: 15 March 2023.
Edited by:
Charlotte Conn, RMIT University, AustraliaReviewed by:
Yuri Gerelli, National Research Council (CNR), ItalyCopyright © 2023 Fornasier and Murgia. This is an open-access article distributed under the terms of the Creative Commons Attribution License (CC BY). The use, distribution or reproduction in other forums is permitted, provided the original author(s) and the copyright owner(s) are credited and that the original publication in this journal is cited, in accordance with accepted academic practice. No use, distribution or reproduction is permitted which does not comply with these terms.
*Correspondence: Marco Fornasier, bWFyY28uZm9ybmFzaWVyQGZrZW0xLmx1LnNl Sergio Murgia, bXVyZ2lhc0B1bmljYS5pdA==
Disclaimer: All claims expressed in this article are solely those of the authors and do not necessarily represent those of their affiliated organizations, or those of the publisher, the editors and the reviewers. Any product that may be evaluated in this article or claim that may be made by its manufacturer is not guaranteed or endorsed by the publisher.
Research integrity at Frontiers
Learn more about the work of our research integrity team to safeguard the quality of each article we publish.