- 1National Research Council of Italy (CNR), Institute of Marine Science (ISMAR), Rome, Italy
- 2University of Central Florida (UCF), Department of Civil, Environmental and Construction Engineering, Orlando, FL, United States
- 3National Research Council of Italy (CNR), Institute of Marine Science (ISMAR), Naples, Italy
Coastal areas represent delicate and complex environments due to the interconnection between land and sea, where marine, fluvial and anthropogenic stressors combine threatening and undermining coastal health. Sea level rise and increasing storminess, for instance, lead to more frequent coastal flooding and habitat loss due to erosion; sediment supply by rivers, on the other hand, helps coastal areas to balance and restore habitat loss. However, excessive riverine nutrient inputs may lead to coastal eutrophication phenomena, putting coastal ecosystem as well as coastal communities at serious risk. Here, we compute high resolution (300 m) Chlorophyll-a (Chl) and Total Suspended Matter (TSM, a proxy for sediment concentration) trends over the Adriatic Sea by using the single sensors MERIS (from 2003 to 2012) and OLCI (from 2017–2024) data, to study the response of the marine ecosystem to human and/or environmental pressures, and thus for detecting coastal areas likely subject to eutrophication and/or sediment starvation. Such an analysis is complemented by Po River discharge data to investigate the role of river outputs in shaping the observed trends within the Adriatic basin. Our results reveal Chl and TSM trends in the northern part of the Adriatic basin being positively correlated with the Po River discharge during the investigated period, for both MERIS and OLCI data. Increases/decreases in the Po River outflow resulted in positive/negative Chl and TSM trends. Although a negative trend of Chl was documented within the Adriatic Sea in the last 25 years, Po River load fluctuations regulate long- and short-term, local trends of both Chl and TSM in the North Adriatic basin. This result suggests a direct relationship existing between river discharge and statistical trends of TSM and Chl in delta areas.
1 Introduction
Coastal waters represent a vital heritage due to their importance from an ecological, social and economic point of view (Creel, 2003; Kay and Alder, 2005). Around 90% of global fish yield and about 25% of global marine primary production are provided within coastal areas (Hénocque and Coccossis, 2001; Markovic et al., 2001). Furthermore, human population is concentrated in the coastal zone, with 10% of the world’s population living at 10 m or less above mean sea level (McGranahan et al., 2007; Aucelli et al., 2018), and around 150 million of Europeans living along the Mediterranean shore (Markovic et al., 2001). However, excessive anthropogenic stressors threaten coastal ecosystems. Pollution, eutrophication, urbanization, sediment starvation, overfishing and tourism are among the main activities that negatively impact marine environments (Hénocque and Coccossis, 2001; Markovic et al., 2001).
Coastal health depends on the delicate balance between climate influence and anthropogenic constraints (Falcini et al., 2012; Almar et al., 2023). For instance, sea level rise and increasing storminess lead to more frequent coastal flooding and habitat loss due to erosion (Reimann et al., 2018; Vona and Nardin, 2023; Vona et al., 2024), while sediment supply by rivers would help coastal areas to balance and restore habitat loss (Geist and Hawkins, 2016; Besset et al., 2019). On the other hand, excessive riverine nutrient inputs may lead to coastal eutrophication phenomena, threatening both coastal ecosystems and coastal communities (Howarth and Marino, 2006; Colella et al., 2016; Malone and Newton, 2020). It is therefore important to study and understand the connectivity between coastal and fluvial waters for effective environmental management, conservation efforts, and sustainable development of coastal regions.
To observe eutrophication and sediment dynamics from space, specific biogeochemical and physical parameters need to be identified. Chlorophyll-a (Chl) concentration represents the most direct indicator to monitor phytoplankton biomass and the eventual intensification of it (Colella et al., 2016; UNEP, 2021; Brando et al., 2022); Total Suspended Matter (TSM), i.e., the concentration of organic and inorganic material suspended in the water, depicts a suitable indicator to monitor sediment plume evolution along the coast, as TSM also encompasses terrigenous loads such as suspended sediment (Nechad et al., 2010; Pitarch et al., 2019).
Satellite Ocean Colour (OC) represents a unique tool for environmental observation thanks to the opportunity to retrieve biogeochemical properties at different temporal and spatial resolution. Since the Coastal Zone Colour Scanner (CZCS, i.e., a radiometer aboard Nimbus 7, launched in 1978), OC data can synoptically observe spatial features of eutrophication phenomena and plume dynamics, which cannot be detected from in situ measurements (Coppini et al., 2013; Gohin et al., 2019). Through robust time-series of OC, the estimation of statistical trends represents a valid methodology for evaluating the synergy between eutrophication phenomena and sediment loads, as well as for evaluating sediment re-distribution along the coast to assess erosive or enhancement processes.
Legislative and regulatory policies have been developed by many countries worldwide to restore, protect and preserve marine ecosystems (Sprovieri et al., 2021; Politi et al., 2024; Moretti et al., 2024). Within the Western Adriatic Sea coast (Figure 1), where multiple eutrophication phenomena occurred (Solidoro et al., 2009; Malone and Newton, 2020; Ricci et al., 2022; Aragão et al., 2024), the study of the delicate balance between riverine sediment plumes and nutrient loads is crucial for the implementation of national directives, and to ensure that human activities are sustainably pursued. In this basin (see Study Site), riverine waters, rich in nutrients and suspended sediments, modulate the water bio-production (Marini et al., 2008; Solidoro et al., 2009; Tesi et al., 2011). Over the years, excessive nutrient loads have caused numerous algae bloom phenomena in the region that were documented by several studies in the literature (Ricci et al., 2022; Aragão et al., 2024). This raised concern from governmental agencies and moved the research towards strategies (such as the European Marine Strategy Framework Directive or the European Water Framework Directive, to cite a few) aimed at restoring and maintaining a good environmental state (Karydis and Kitsiou, 2012; Colella et al., 2016). It is therefore important to monitor and assess environmental phenomena to evaluate the impact of these adopted strategies on the marine environment. To do so, we use a satellite-based analysis by using MERIS data of Chl and TSM from 2003 to 2012 (see Data and Methods). To further extend the study to the present days, we also analyzed OLCI data of Chl and TSM from 2017–2024 within the North Adriatic Sea (NAS).
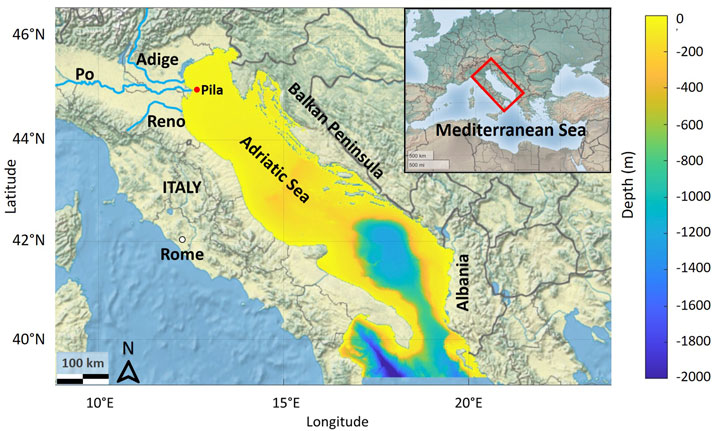
Figure 1. The Adriatic Sea with its three major Rivers of the northern sector (in light blue), the Po, Adige, and Reno Rivers. Colors denote the bathymetry data. The red dot off the Po river mouth indicates the Pila station, used later on in the paper to extract Chl and TSM time-series to be compared with the Po River discharge.
Despite several authors having investigated the Adriatic Sea biogeochemical patterns, the estimation of high-resolution statistical trends for Chl and TSM has received less consideration. Coastal plume evolution in- and off-shore the NAS has been described by several studies as strongly influenced by river discharge and meteo-marine conditions (Bignami et al., 2007; Brando et al., 2015; Braga et al., 2017; Bellafiore et al., 2019). Similarly, phytoplankton blooms in the NAS, strongly affected by the Po River discharge, wind-induced marine circulation, and seasonal period, were reported by many researchers through the years (Bignami et al., 2007; Solidoro et al., 2009; Malone and Newton, 2020; Ricci et al., 2022; Aragão et al., 2024). On the other hand, the estimation of statistical trends usually focused more at regional (Mediterranean Sea) rather than sub-basin (Adriatic Sea) scale. However, within the Mediterranean Sea, trend estimation through satellite data mostly focused on biogeochemical parameters such as Chl, rather than TSM. Barale et al. (2008) and Coppini et al. (2013) used SeaWiFS data to compute Chl concentration trends, reaching however different outcomes along coastal Mediterranean regions. Colella et al. (2016) applied the Mann-Kendall test and Sen’s slope to a de-seasonalized monthly time series of Chl SeaWiFS data at 1 km spatial resolution, from 1998 to 2009. Their results showed patterns of both negative and positive trends in the Mediterranean Sea, in agreement with other studies in the literature (Henson et al., 2010; Vantrepotte and Mélin, 2011); the Adriatic Sea was marked by a negative Chl trend, as found by Mélin et al. (2011) through a 12-year multi-sensor time series. Recent studies also confirmed a negative trend of Chl in the last 25 years in the Adriatic Sea, especially within the northern sector (Malone and Newton, 2020; Brando et al., 2022; CMEMS products, https://doi.org/10.48670/moi-00260).
Studies in the literature that do not exploit satellite data also report negative trends of Chl off the Po river mouth (Giani et al., 2012), in the western coastal zone of the NAS (Alvisi and Cozzi, 2016) and the Gulf of Trieste (Kralj et al., 2019). More recent long-term analyses on physical and biogeochemical parameters indicate that nutrient concentrations in the NAS, in the past 30 years, have been largely modulated by the evolution of the Po river runoff (Grilli et al., 2020; Cavallini et al., 2024).
Nonetheless, the studies cited above have the disadvantage of having limited spatial resolution (about 2 km for EO-based studies, which cannot highlight local phenomena that occur at smaller scales along the coast), or referring to in situ stations which do not provide an overall view of the entire basin. Hence, we believe that a high-resolution trend analysis of both Chl and TSM would be beneficial to emphasize smaller coastal environmental features occurring within the whole Adriatic Sea.
Inspired by these previous efforts and aiming to recognize high-resolution (300 m) biogeochemical coastal patterns, here we analyze trends of Chl and TSM in the Adriatic Sea by using OC products derived from the MERIS and OLCI sensors. Our goal is to study the mutual effect of coastal eutrophication and sediment starvation, also evaluating how sediment dispersion and redistribution along the shore affected coastal resilience in the considered years, from 2003–2012 (MERIS) and from 2017–2024 (OLCI). Moreover, the role of the Po River discharge in shaping the observed trends within the northern basin of the Adriatic Sea, is also examined.
2 Study site–the Adriatic Sea
The Adriatic Sea (Figure 1) is the semi-enclosed basin of the northeastern Mediterranean Sea, separating the Italian and the Balkan peninsula. It is characterized by a shallow Northern sector, i.e., the North Adriatic Sea (NAS), with 35 m average depth, a deeper central portion (average depth 140 m) and an even deeper southern sector.
Riverine freshwater input is an important forcing for the Adriatic circulation: the coast is rich in river estuaries, particularly in its north-western sector, where the Po River alone contributes to more than 70% of the total runoff (Mikhailova, 2002; Brando et al., 2015). Riverine input along the NAS is generally associated with a sharp coastal front that contributes in driving a southeastward current along the Italian coast, i.e., the West Adriatic Coastal Current (WACC), which is also fed by the buoyancy input from the Po River and other smaller rivers of the NAS (Bergamasco et al., 1999; Benincasa et al., 2019); the WACC represents the main process that carries suspended substances out along the Italian coast of the Adriatic Sea. On the other hand, sub-mesoscale patterns of plume spreading within the basin are strongly controlled by the modulation of freshwater discharge and wind regime, i.e., Bora and Scirocco (Bignami et al., 2007; Tesi et al., 2011; Falcieri et al., 2014). Stratification also affects circulation patterns: well mixed waters in the NAS likely confine coastal waters onshore, while summer stratified conditions allow offshore propagation (Sturm et al., 1992). However, intense Bora (northeasterly) events are responsible for winter offshore plume expansion (Bignami et al., 2007).
3 Data and methods
3.1 MERIS data
MERIS is an OC sensor designed to monitor changes in water quality of optically complex waters, from coastal areas to the open ocean. MERIS mission, on board of the European satellite Envisat, lasted for 10 years (from March 2002 to April 2012), providing global coverage of the Earth within 3 days (Doerffer et al., 2007; Rast et al., 1999; Levrini and Delvart, 2011). MERIS measured radiances over 15 bands in the spectral range of 412.5–900 nm, providing full resolution products at 300 m spatial resolution (Doerffer et al., 2007; Rast et al., 1999).
In our analysis, we used the fourth reprocessing of MERIS L2 data of Chl and TSM from 2003 to 2012 available in the ESA catalog (ESA, 2020; https://doi.org/10.5270/EN1-vqoj1gs). Chl and TSM are retrieved by the Case-2 Regional Coast Colour (C2RCC) algorithm (Doerffer et al., 2007; Brockmann et al., 2016). The C2RCC algorithm uses several sets of neural networks to accomplish the inversion of each spectrum for the atmospheric correction and the retrieval of the inherent optical properties then used to generate water parameters. Flags included in MERIS products were implemented to mask invalid pixels. The L2 product already contains the atmospheric correction and provides information about water properties such as Chl and TSM concentration, as well as other variables. It also contains an ortho-corrected geo-coding and different flags characterizing pixels. The L2 data, however, needs to be re-mapped on a regular grid as it still follows the earth curvature. The L3 product is the result of remapping the L2 data. Nevertheless, the L3 data still contains gaps associated with cloud covering or invalid pixels. In order to obtain L4 products (full covered images with no gaps), the following procedure has been applied. First, monthly means have been evaluated to partially fill the empty pixels; the remaining gaps have been then filled with monthly climatological values. The impact of this methodology on final results has been shown to be statistically small (Colella et al., 2016). The obtained L4 monthly averaged maps have been given as input for the trend procedure.
3.2 OLCI Full-Resolution data
The Sentinel-3 (A and B) OLCI Level-3 Full-Resolution (300 m) Chl product was downloaded from the Copernicus Marine Service (Products ID OC_MED_009_141). Chl retrieval is based on two regional algorithms blended according to the water types (Colella et al., 2024; Brando et al., 2024). For clear waters, updated version of the regionally parameterized maximum band ratio MedOC4 (Volpe et al., 2019) is applied, while the ADOC4 algorithm (Berthon and Zibordi, 2004) suitable for optically complex waters is used. Then, the determination of the water type also accounts for high Chl concentration due to phytoplankton blooms (e.g., Gulf of Lions) or water column mixing (e.g., Alborán Sea) that could be identified as optically complex waters (Colella et al., 2024; Brando et al., 2024). This OLCI-based Chl product is well calibrated and validated in the Mediterranean Sea, including the Adriatic sub-basin (Colella et al., 2024). The OLCI L3 Full-Resolution (300 m) TSM product derives from the neural network algorithm operationally implemented and distributed by EUMETSAT (as well as for Chl product). Assessment and reliability of this product were provided by Giannini et al. (2021). Both products were downloaded from 2017 to 2024 within the NAS. L4 OLCI imageries were obtained by applying the same procedure described in section 3.1 for the MERIS dataset.
3.3 Additional data
The water discharge dataset was analyzed to complement and better support our results. The discharge dataset for the Po River at Pontelagoscuro gauging station, obtained from the Environmental Protection Agency of the Region of Emilia Romagna (ARPA-ER), was integrated to explore potential correlations between river discharge and eutrophication dynamics.
Copernicus Marine Environment Monitoring Service (CMEMS) L3 monthly Chl data (https://doi.org/10.48670/moi-00260) derived from multi-sensor analysis (SeaStar-SeaWiFS, AQUA-MODIS, NOAA20-VIIRS, NPP-VIIRS, Envisat-MERIS and Sentinel-3-OLCI) at 1 km of spatial resolution were used to compare trend map results from MERIS fourth reprocessing data. L4 CMEMS imageries were obtained by applying the same procedure described in Section 3.1 for the MERIS dataset. The consistency of the two Chl time series with spatial resolutions at 300 m (MERIS) and 1 km (CMEMS) was assessed by comparing both L4 monthly datasets used for the trend estimation, through the extraction of a set of co-located data points (Supplementary Figure S2). Chl values were extracted from the multi-sensor dataset every 10 km (every 10 pixels) for each monthly image, to be then compared to the corresponding mean from a 3 × 3 pixel window of the MERIS dataset (González Vilas et al., 2024).
3.4 Detecting trends in the Adriatic Sea
The trend methodology applied in this study follows Colella et al. (2016). First, the X-11 procedure was applied to both TSM and Chl L4 products to decompose the original time series (of both TSM and Chl) into three different components: the seasonal signal, the trend component, and an irregular component (Shiskin, 1978; Dagum, 1980). Considering that the seasonal component can mask small variations in the trend (Colella et al., 2016), the series were then de-seasonalized. Finally, we applied the Mann-Kendal (MK) test and the Sen’s slope (Mann, 1945; Kendall, 1948; Sen, 1968) to the de-seasonalized time series of TSM and Chl in order to estimate the statistical trends. The non-parametrical MK test was chosen as it can detect either upward or downward trends (Colella et al., 2016), and it is particularly suitable for non-normally distributed data and for datasets containing gaps (Vantrepotte and Mélin, 2011). To better highlight the obtained trends over the entire period of observation, we provide results for each pixel in terms of relative changes (i.e., % yr-1) by dividing the magnitude of TSM and Chl trends by the corresponding climatological value, for both MERIS and OLCI datasets. A full comprehensive explanation of the applied methodology to detect the trends can be found in Colella et al. (2016).
4 Results and discussion
An example of climatological mean is given for MERIS data from 2003–2012, which showed high TSM and Chl concentration along the Italian coast and lower concentration within the rest of the basin (Figure 2). This is a well-known pattern that results from the interaction between the sedimentary and biogeochemical inputs debouching from the main rivers of the north-west Adriatic coast and the WACC, which works as a conveyor belt transporting the riverine loads southward for hundreds of kilometers. The high observed Chl concentration close to shore (as well as off the Albanian coast in the south-eastern region), however, may also be a function of an overestimation of the Chl algorithm in optically complex waters (Bignami et al., 2007; Melin et al., 2011; Odermatt et al., 2012).
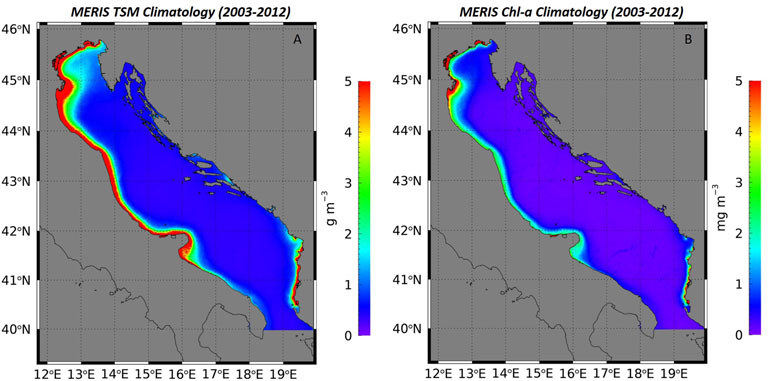
Figure 2. Climatology for the MERIS monthly-averaged L4 data of (A) TSM and (B) Chl concentration over the entire observation period (2003–2012).
4.1 The influence of the Po River discharge on trends of Chl and TSM
Regarding statistical trends (trend significance is shown in Supplementary Figure S1), MERIS data shows a marked positive trend of both TSM and Chl in the NAS, especially off the Po River delta (Figure 3); this trend is strongly significant, with Mann-Kendall test values that are higher than 80% (Supplementary Figure S1). A mixture of positive and negative trends (weakly significant) is found along the Italian coastline, for both TSM and Chl (Figure 3), while the open waters are marked by negative weakly significant TSM and positive significant Chl trends (Figures 3A–D; Supplementary Figure S1).
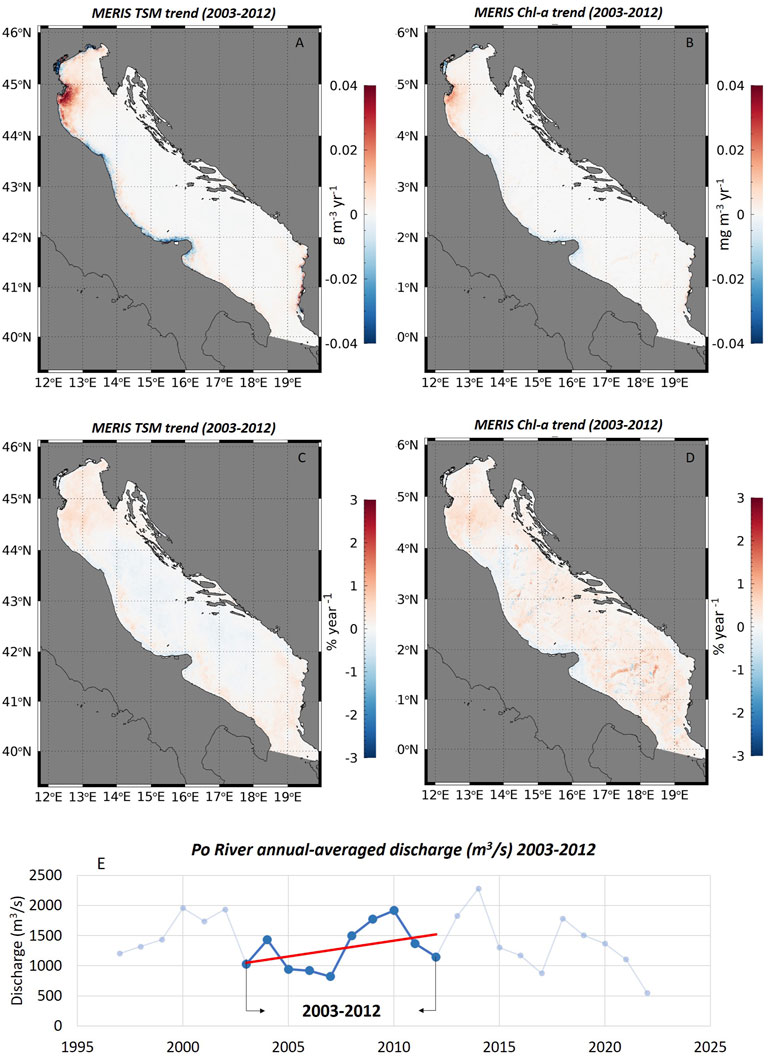
Figure 3. Trend map for the monthly-averaged, de-seasonalized MERIS data from 2003–2012. (A) TSM trend expressed in change per year (g m-3 year-1) and (B) Chl trend expressed in change per year (mg m-3 year-1). (C) TSM trend expressed in % of change (% year-1) with respect to the climatology and (D) Chl trend expressed in % of change (% year-1) with respect to the climatology. (E) Annual average Po River discharge at Pontelagoscuro station; data from ARPA-ER.
Patterns of significant positive Chl trend resulting from the 2003–2012 MERIS data in the NAS apparently disagree with the literature, which reports negative trends for Chl in the Adriatic Sea in the last 25 years, especially within its northern sector (Melin et al., 2011; Colella et al., 2016; Malone and Newton, 2020; Brando et al., 2022; CMEMS products, https://doi.org/10.48670/moi-00260). On the other hand, the TSM trend is harder to compare with other studies due to the lack of literature regarding statistical analyses of this parameter. However, several authors and monitoring programs report a general decrease of the Po River discharge between 2003–2007 (Djakovac et al., 2012; Giani et al., 2012; Colella et al., 2016; Cavallini et al., 2024; Mentaschi et al., 2024). This should imply a decrease of TSM, resulting in a negative trend that would not agree with the positive 2003–2012 MERIS TSM trend we found in the NAS. This conundrum can be explained by strictly focusing on the hydrologic analysis on the time-window 2003 to 2012, i.e., the one that matches with the MERIS time series. It results in a positive trend for the Po River discharge from 2003 to 2012 (Figure 3E) (Grilli et al., 2020; Cavallini et al., 2024), which better supports the MERIS TSM trend (Figures 3A, C).
A further analysis through CMEMS multi-sensor Chl data (1 km spatial resolution) confirmed the positive trends in the NAS we found through MERIS data (Figures 4C, D). CMEMS Chl regional data (https://doi.org/10.48670/moi-00260) shows a general negative trend in the Adriatic Sea in the last 25 years, from 1997–2022, in agreement with Colella et al. (2016) who analyzed the period 1998–2009. However, once considering the MERIS time-window from 2003–2012 only, the CMEMS data also shows a positive Chl trend, which supports the MERIS results. The comparison of L4 Chl retrievals over the Adriatic Sea used for the trend estimation between MERIS data at 300 m of spatial resolution and the multi-sensor data at 1 km resolution (Supplementary Figure S2) further reinforces trend outcomes.
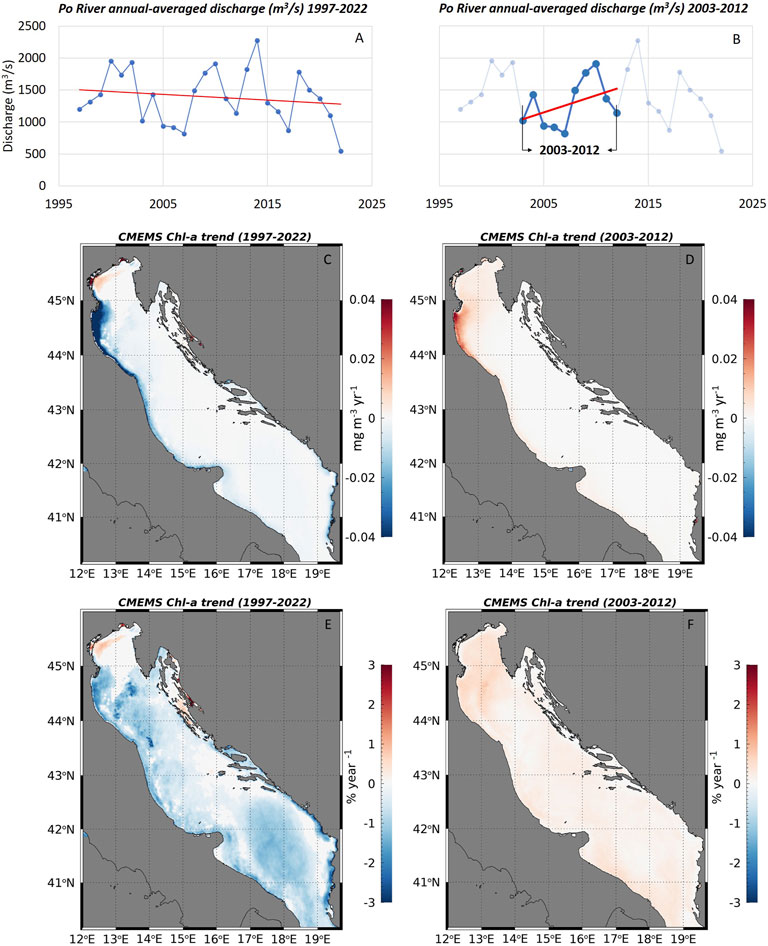
Figure 4. (A) Annual-averaged Po River discharge from 1997 to 2022; the red line indicates the negative linear trend. (B) Annual-averaged Po River discharge from 2003 to 2012; the red line indicates the positive linear trend. Data from Environmental Protection Agency of the Region of Emilia Romagna (ARPA-ER). (C) Trend map of Chl through CMEMS multi-sensor data from 1997–2022 expressed in change per year (mg m-3 year-1). (D) Trend map of Chl through CMEMS data from 2003–2012 in change per year (mg m-3 year-1). (E) Trend map of Chl through CMEMS data from 1997–2022 in % of change (% year-1) with respect to the climatology. (F) Trend map of Chl through CMEMS data from 2003–2012 in % of change (% year-1) with respect to the climatology.
The comparison between long- (1997–2022) and short-term (2003–2012) Chl and TSM trends highlights the positive relationship between water discharge and Chl and TSM trends in the NAS. Negative Chl (and TSM) long-term trends correspond to the long-term decrease of river discharge from 1997 to 2022 (Figures 4A, C), while a short-term increase in river discharge results in the positive Chl (and TSM) trends from 2003 to 2012, for both MERIS (see earlier Figure 3) and CMEMS (Figures 4B, D) data. Flow and biogeochemical/sedimentary load fluctuations of the Po River rapidly influences and determines long and short-term local trends of Chl and TSM within the NAS (results also supported by Grilli et al., 2020; Cavallini et al., 2024). Such relationship is further confirmed by OLCI data within the NAS from 2017–2022, which shows how negative trends of Chl and TSM agree with the decrease in the Po River discharge (Figure 5). Furthermore, the analysis of the Po annual discharge shows an increase in flow rate from 2023–2024. This change in the flow rate is also reflected in Chl and TSM trends (Figure 6). Trends of both Chl and TSM are negative when considering the 2017–2022 time-window (when the discharge decreases); from 2017–2023 a shift in trends towards positive values can be observed. This change in the trends becomes even more evident when considering the 2017–2024 time-series (the effect of the rising discharge is stronger), where the resulting TSM trend is positive in the NAS except for the Venice lagoon, denoting the fast response of TSM to changes in the Po River discharge; the Chl trends, on the other hand, is still slightly negative coastwise, denoting the slower response of Chl to changes in the Po discharge (Figure 6). OLCI data suggests the trends within the NAS (for Chl and TSM), not only at the Po River mouth, are affected and regulated by oscillations in the Po River discharge.
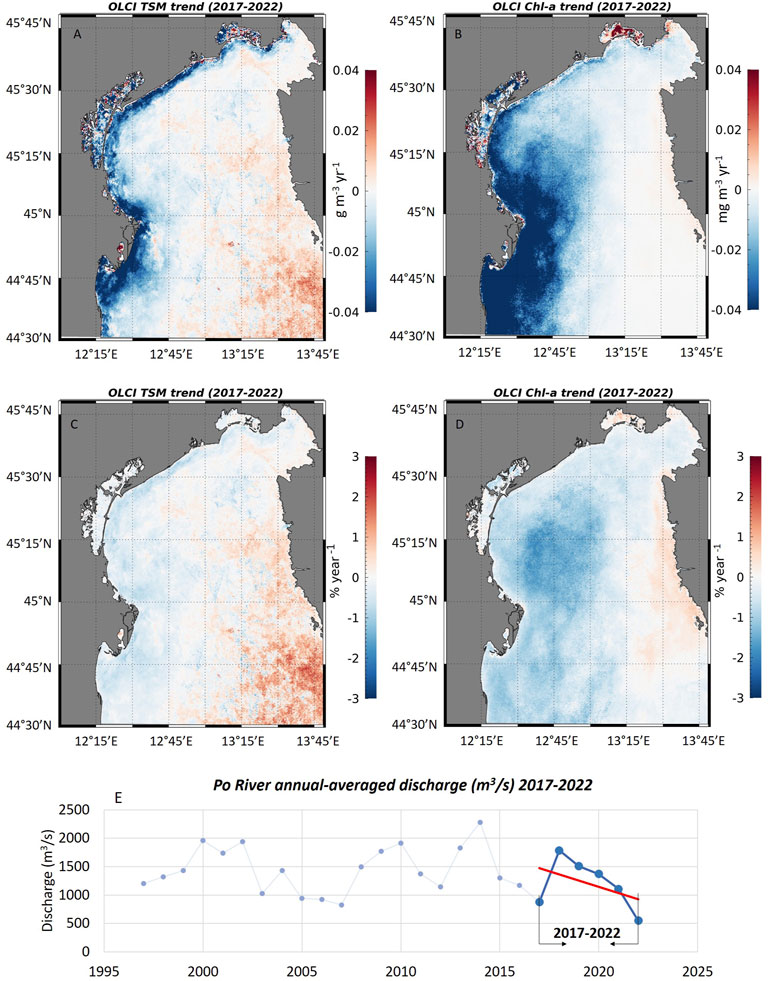
Figure 5. Trend map for the monthly-averaged, de-seasonalized OLCI data from 2017–2022. (A) TSM trend expressed in change per year (g m-3 year-1) and (B) Chl trend expressed in change per year (mg m-3 year-1). (C) TSM trend expressed in % of change (% year-1) with respect to the climatology and (D) Chl trend expressed in % of change (% year-1) with respect to the climatology. (E) Annual average Po River discharge at Pontelagoscuro station; data from ARPA-ER.
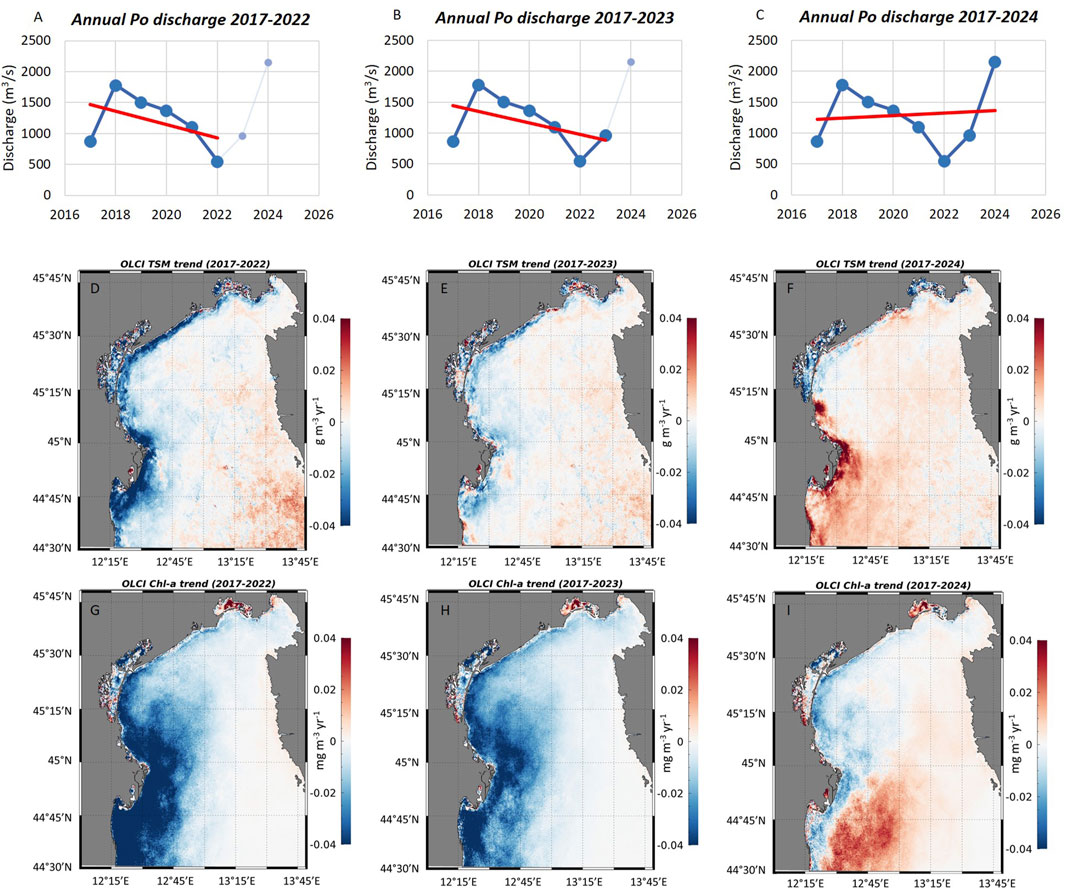
Figure 6. Annual average Po River discharge at Pontelagoscuro station from (A) 2017–2022, (B) 2017–2023 and (C) from 2017–2024. Data from ARPA-ER. OLCI TSM trend from (D) 2017–2022, (E) 2017–2023 and (F) 2017–2024. OLCI Chl trend from (G) 2017–2022, (H) 2017–2023 and (I) 2017–2024.
The influence of the Po River on TSM and Chl trends is further reinforced by comparing time series of TSM and Chl L4 products (extracted at the PILA station, see earlier Figure 1) with discharge data. As the trends are expressed as change per year (mg m-3 yr-1 or % yr-1) and our intent is to support the correlation between discharge and trend, we compared the annual-averaged MERIS (2003–2012) and OLCI (2017–2024) L4 data of TSM and Chl (extracted at PILA station) with the annual-averaged Po discharge (Figures 7A–D). Significant correlations emerged, reinforcing the assumption that the annual cycle of Po discharge has an influence on annual trends of Chl and TSM in the NAS. Similar outcomes verify when comparing annual-averaged L4 CMEMS Chl data with the Po River flow from 1997–2022 (Figures 7E, F). The decrease of river flow correlates with the decrease of Chl at the PILA station, supporting the hypothesis that the annual Po discharge cycle affects annual trends.
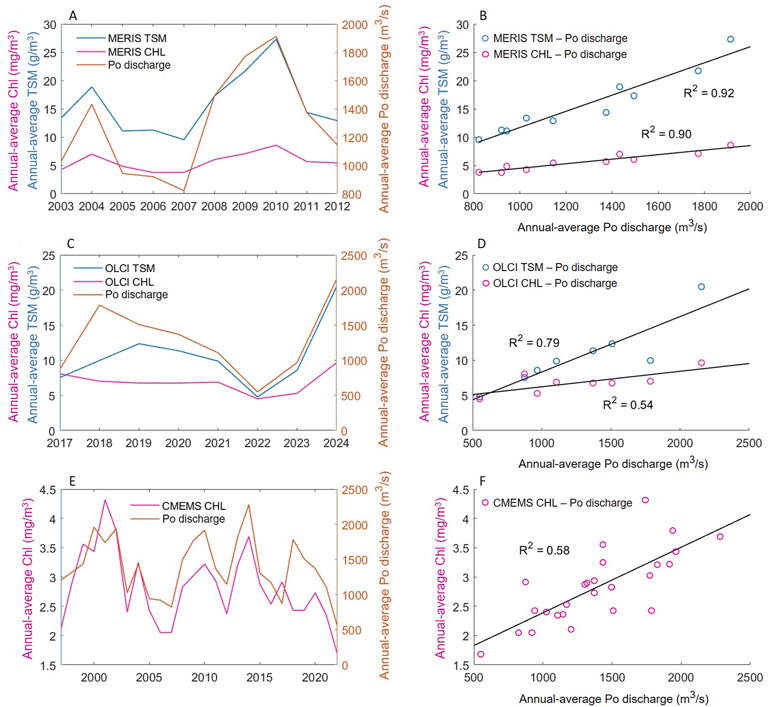
Figure 7. (A) Time-series of annual-averaged MERIS L4 TSM and Chl data (extracted at PILA station) with annual averaged discharge data at Pontelagoscuro from 2003–2012. (B) Correlation between annual-averaged MERIS L4 TSM vs annual-averaged discharge and annual-averaged MERIS L4 Chl vs annual-averaged discharge. (C) Time-series of annual-averaged OLCI L4 TSM and Chl data (extracted at PILA station) with annual averaged discharge data at Pontelagoscuro from 2017–2024. (D) Correlation between annual-averaged OLCI L4 TSM vs annual-averaged discharge and annual-averaged OLCI L4 Chl vs annual-averaged discharge. (E) Time-series of annual-averaged CMEMS L4 Chl data (extracted at PILA station) with annual averaged discharge data at Pontelagoscuro from 1997–2022. (F) Correlation between annual-averaged CMEMS L4 Chl with annual-averaged discharge data from 1997–2022.
4.2 The importance of appropriate spatial resolution for monitoring coastal phenomena
As noted by comparing trend results, both CMEMS multi-sensor and MERIS fourth reprocessing data from 2003 to 2012 give positive and statistically significant Chl trends in the NAS. Along the western coast, however, multi-sensor and MERIS data differs from each other: while the multi-sensor Chl trend is positive and strongly significant along the whole western coast (Supplementary Figure S3), MERIS data shows some negative patterns coastwise. Despite the lower statistical significance likely due to the interpolation of a greater number of invalid pixels with climatological values, the higher spatial resolution of MERIS data (300 m) emphasizes negative Chl trends along the coast (from 2003–2012) that the lower resolution of CMEMS data (1 km) cannot capture. The difference between CMEMS and MERIS coastal trends denotes the importance of the adopted satellite sensor’s spatial resolution to properly describe the highly variable coastal processes that occur on a really small spatial and temporal scale, heavily influenced by climatic and anthropogenic stressors. An appropriate monitoring is the key to adequately describe phenomena that affect coastal environment and ecosystem health. The ESA Sentinel-2 mission, which provides satellite data up to 10 m spatial resolution, will allow scientists to accurately monitor and study coastal processes in the future.
On the other hand, the negative and positive MERIS TSM trend patterns we observe coastwise might be attributed to either reduced alongshore transport of suspended sediment (due to a decrease in riverine inputs) and/or local sediment resuspension. However, the weak TSM trend significance, alongside the lack of supporting data, cannot further confirm such hypothesis. Therefore, coastal TSM trends will need further analysis.
4.3 The importance of considering a trade-off between TSM and Chl
The balance between suspended sediment debouching from river mouths and chlorophyll concentrations is a key aspect for marine and coastal health (Twilley and Rivera-Monroy, 2009). Our results highlighted a correlated trend between Chl and TSM in the NAS, denoting that Chl is strongly influenced by the TSM carried out by riverine loads. Furthermore, TSM does not only account for sediments but also for any organic and inorganic material suspended in the water (Nechad et al., 2010) and it is therefore reasonable to expect Chl and TSM values that positively correlate with each other. The high correlation between TSM and Chl trends found with both MERIS and OLCI data within the NAS is highlighted by comparing the monthly L4 data of TSM and Chl within the NAS, for both MERIS and OLCI sensors (Figure 8). TSM and Chl data are strongly correlated with each other in the NAS, denoting how TSM influences Chl. To avoid considering an excessive number of values, monthly TSM and Chl were extracted within the NAS every 4 monthly images, every 4 pixels.
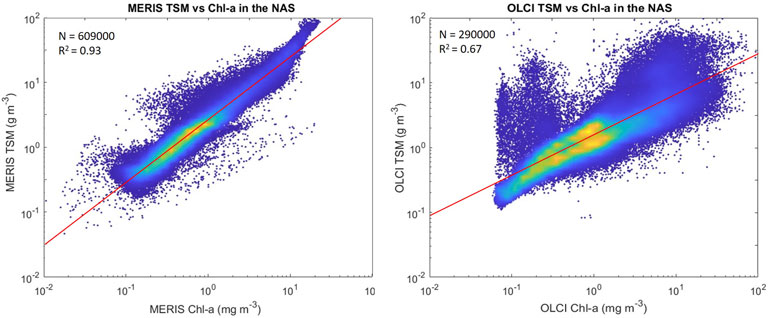
Figure 8. Density-Scatter plot of MERIS and OLCI L4 monthly data to highlight the correlation between TSM and Chl in the NAS. The colors in the plots indicate density values; dark blue indicates scattered and not very concentrated points, while yellow indicates high density and concentration. Most of the points, for both MERIS and OLCI data, are concentrated along the 1:1 line.
Given the high correlation between TSM and Chl in the NAS, our results highlight the importance of finding a trade-off between TSM and Chl to maintain a good environmental status of coastal environments, as large inputs of suspended substances might lead to undesirable levels of chlorophyll concentration. However, TSM did not particularly alter Chl concentration between 2002–2012 and 2017–2024, as no relevant eutrophication phenomena have been reported in the literature. Rather, many authors found negative trends of chlorophyll in the last 2 decades that resulted in no remarkable algae bloom event, as well as a projected oligotrophication of the whole Adriatic marine ecosystem (Giani et al., 2024; Mentaschi et al., 2024; Ricci et al., 2024; Soana et al., 2024). On the other hand, although excessive riverine loads might enhance coastal eutrophication, sediment delivery is an important aspect for coastal restoration purposes (Twilley and Rivera-Monroy, 2009), as it will help to balance habitat loss due to erosion phenomena. Inadequate sediment supply may not be sufficient to maintain the sedimentary equilibrium and result in a retreating shoreline. Signs of erosion along the Adriatic coast have been reported by several authors in the literature (Bonaldo et al., 2019; Gallina et al., 2019; Di Paola et al., 2022), enhancing the importance of considering the trade-off between TSM and Chl towards a more resilient and healthier coastal environment.
5 Conclusion
The estimation of Chl trends, as a proxy for eutrophication phenomena, has been identified as a proper descriptor by the European Marine Strategy (Descriptor 5) and UNEP for Sustainable Development Goals (SDG 14.1.1a) (UNEP, 2021) to ensure compatibility between human activities and marine health. As Chl is strongly influenced by riverine inputs, our research strengthens the assessment of vulnerable coastal areas through coupling Chl with TSM trends. The methodology adopted in this paper (the Mann- Kendall and Sen’s tests applied to the X-11 de-seasonalized signal of MERIS data) applied to satellite data, represents a proper technique to investigate and monitor the environmental status of coastal regions, as it allows to spatially assess the evolution of important environmental parameters such as Chl and TSM. Moreover, such technique has already been successfully applied by other researchers in the literature (Colella et al., 2016; CMEMS products, https://doi.org/10.48670/moi-00260).
Study results highlighted the northern sector as the most vulnerable area of the Adriatic Sea, heavily affected by fluvial contributions, such as the Po River. TSM and Chl were heavily correlated within the NAS (from both MERIS and OLCI data), suggesting that TSM (sediment, as well as any organic and inorganic material suspended in the water) strongly influences Chl concentration over time. Despite the high correlation found between TSM and Chl, no remarkable eutrophication event was reported in the NAS, indicating TSM did not particularly alter Chl concentration between 2002–2012 and 2017–2024. Italian and European environmental regulations allowed an overall improvement of the marine environment, supported by several authors in the literature (Solidoro et al., 2009; Djakovac et al., 2012; Giani et al., 2012; Colella et al., 2016; Giani et al., 2024; Mentaschi et al., 2024; Ricci et al., 2024; Soana et al., 2024).
Despite the well documented negative Chl trend within the Adriatic Sea in the last 25 years, our research demonstrated how flow fluctuations of the Po River regulate long and short-term trends of Chl and TSM in the northern part of the Adriatic Sea, suggesting that a direct relationship exists between river discharge and statistical trends. Hydrological data highlighted the Po River discharge time-series reflects changes over time in TSM and Chl, supporting and strengthening our results. Observed coastal negative and positive TSM trend patterns through MERIS data, on the other hand, likely attributed to either reduced solid transport along the shore and/or coastal erosion phenomena, will need further investigations due to the weak statistical significance and the lack of supporting data. In summary, the main outcomes of the paper are reported below:
• Negative trend of chlorophyll in the Adriatic Sea is reported in the literature (for the past 25 years), as well as a projected oligotrophication of the whole Adriatic marine ecosystem in the near future;
• However, our study demonstrates trends of chlorophyll and total suspended matter are regulated by oscillations in the Po river discharge, in both long and short terms. A positive correlation is found between the trend of the Po river outflow and Chl/TSM trends;
• High correlation between TSM and Chl is found within the whole North Adriatic Sea, highlighting the importance of finding a trade-off between TSM and Chl to maintain a good environmental status;
• Nevertheless, no remarkable eutrophication event has been reported in the NAS during the examined time period (meaning TSM did not particularly alter Chl concentration), mostly thanks to Italian and European regulation aimed at preserving and protecting the marine environment.
Marine health is a complex phenomenon that depends on the delicate balance between human pressure and oceanographic processes. Global warming, sea level rise and increasing storminess will put ecosystems and coastal communities even at greater risk. Satellite measurements for the estimation of Chl and TSM trends can help to monitor and assess applications of environmental regulations on marine and aquatic health, in particular, for coastal environments. Our approach, based on the combination of X-11 procedure, Mann-Kendall test and Sen’s slope on MERIS, CMEMS and OLCI satellite imageries (supported by discharge data of the Po River), allowed us to identify those delicate and impacted areas along the Italian coast of the Adriatic Sea vulnerable to Chl and TSM fluctuations, suggesting inter-connections between river discharge and statistical trends. In an ever-evolving world subjected to rapid and threatening climate change, scientific advances in remote sensing such as the ESA Sentinel-2 mission which provides satellite data up to 10 m spatial resolution (designed for monitoring vegetation, soil and water cover, inland waterways and coastal areas, providing Earth covering every 5 days at the equator since 2015 (Drusch et al., 2012)), as well as other research disciplines (artificial intelligence, modeling technique, etc.), together with the applications of environmental regulations, will provide greater support for monitoring, protecting and restoring marine habitat.
Data availability statement
The original contributions presented in the study are included in the article/Supplementary Material, further inquiries can be directed to the corresponding author.
Author contributions
IV: Formal Analysis, Investigation, Methodology, Validation, Visualization, Writing – original draft. SC: Methodology, Supervision, Validation, Writing – review and editing. MS: Methodology, Writing – review and editing. VB: Supervision, Validation, Writing – review and editing. FF: Conceptualization, Investigation, Methodology, Supervision, Writing – original draft.
Funding
The author(s) declare that financial support was received for the research and/or publication of this article. This work was primarily supported by MITHO (Multi-stressors impact on Ocean Health) project, funded by the European Space Agency via the contract Grant No. 4000142100/23/I-DT, and by the Horizon-Europe project FOCCUS (Forecasting and observing the open-to-coastal ocean for Copernicus users, Grant Agreement 10113391). This work has also been performed in the context of the Copernicus Marine Service (2015-2021: 77-CMEMS-TAC-OC, 2021-2024: 21001L2-COP-TAC OC-2200).
Acknowledgments
We thank Gianluca Volpe, Angela Landolfi, and Jaime Pitarch for the insightful discussion at the initial stage of the investigation.
Conflict of interest
The authors declare that the research was conducted in the absence of any commercial or financial relationships that could be construed as a potential conflict of interest.
Generative AI statement
The author(s) declare that no Generative AI was used in the creation of this manuscript.
Publisher’s note
All claims expressed in this article are solely those of the authors and do not necessarily represent those of their affiliated organizations, or those of the publisher, the editors and the reviewers. Any product that may be evaluated in this article, or claim that may be made by its manufacturer, is not guaranteed or endorsed by the publisher.
Supplementary material
The Supplementary Material for this article can be found online at: https://www.frontiersin.org/articles/10.3389/frsen.2025.1574347/full#supplementary-material
References
Almar, R., Boucharel, J., Graffin, M., Abessolo, G. O., Thoumyre, G., Papa, F., et al. (2023). Influence of El Niño on the variability of global shoreline position. Nat. Commun. 14 (1), 3133. doi:10.1038/s41467-023-38742-9
Alvisi, F., and Cozzi, S. (2016). Seasonal dynamics and long-term trend of hypoxia in the coastal zone of Emilia Romagna (NW Adriatic Sea, Italy). Sci. Total Environ. 541, 1448–1462. doi:10.1016/j.scitotenv.2015.10.011
Aragão, L., Mentaschi, L., Pinardi, N., Verri, G., Senatore, A., and Di Sabatino, S. (2024). The freshwater discharge into the Adriatic Sea revisited. Front. Clim. 6, 1368456. doi:10.3389/fclim.2024.1368456
Aucelli, P. P., Di Paola, G., Rizzo, A., and Rosskopf, C. M. (2018). Present day and future scenarios of coastal erosion and flooding processes along the Italian Adriatic coast: the case of Molise region. Environ. Earth Sci. 77, 371–419. doi:10.1007/s12665-018-7535-y
Barale, V., Jaquet, J.-M., and Ndiaye, M. (2008). Algal blooming patterns and anomalies in the Mediterranean Sea as derived from the SeaWiFS data set (1998–2003). Remote Sens. Environ. 112, 3300–3313. doi:10.1016/j.rse.2007.10.014
Bellafiore, D., Ferrarin, C., Braga, F., Zaggia, L., Maicu, F., Lorenzetti, G., et al. (2019). Coastal mixing in multiple-mouth deltas: a case study in the Po delta, Italy. Estuar. Coast. Shelf Sci. 226, 106254. doi:10.1016/j.ecss.2019.106254
Benincasa, M., Falcini, F., Adduce, C., Sannino, G., and Santoleri, R. (2019). Synergy of satellite remote sensing and numerical ocean modelling for coastal geomorphology diagnosis. Remote Sens. 11 (22), 2636. doi:10.3390/rs11222636
Bergamasco, A., Oguz, T., and Malanotte-Rizzoli, P. (1999). Modeling dense water mass formation and winter circulation in the northern and central Adriatic Sea. J. Mar. Syst. 20 (1-4), 279–300. doi:10.1016/s0924-7963(98)00087-6
Berthon, J. F., and Zibordi, G. (2004). Bio-optical relationships for the northern Adriatic Sea. Int. J. Remote Sens. 25 (7-8), 1527–1532. doi:10.1080/01431160310001592544
Besset, M., Anthony, E. J., and Bouchette, F. (2019). Multi-decadal variations in delta shorelines and their relationship to river sediment supply: an assessment and review. Earth-science Rev. 193, 199–219. doi:10.1016/j.earscirev.2019.04.018
Bignami, F., Sciarra, R., Carniel, S., and Santoleri, R. (2007). Variability of Adriatic Sea coastal turbid waters from SeaWiFS imagery. J. Geophys. Res. Oceans 112 (C3). doi:10.1029/2006jc003518
Bonaldo, D., Antonioli, F., Archetti, R., Bezzi, A., Correggiari, A., Davolio, S., et al. (2019). Integrating multidisciplinary instruments for assessing coastal vulnerability to erosion and sea level rise: lessons and challenges from the Adriatic Sea, Italy. J. Coast. Conservation 23, 19–37. doi:10.1007/s11852-018-0633-x
Braga, F., Zaggia, L., Bellafiore, D., Bresciani, M., Giardino, C., Lorenzetti, G., et al. (2017). Mapping turbidity patterns in the Po river prodelta using multi-temporal Landsat 8 imagery. Estuar. Coast. Shelf Sci. 198, 555–567. doi:10.1016/j.ecss.2016.11.003
Brando, V. E., Braga, F., Zaggia, L., Giardino, C., Bresciani, M., Matta, E., et al. (2015). High-resolution satellite turbidity and sea surface temperature observations of river plume interactions during a significant flood event. Ocean Sci. 11 (6), 909–920. doi:10.5194/os-11-909-2015
Brando, V. E., Pardo, S., Sathyendranath, S., Howey, B., Land, P., Jackson, T., et al. (2022). Potential eutrophication of European waters using satellite derived chlorophyll following the UN Sustainable Development Goal 14 framework. J. Operational Oceanogr. 15, s83–s91. sup1. doi:10.1080/1755876X.2022.2095169
Brando, V. E., Santoleri, R., Colella, S., Volpe, G., Di Cicco, A., Sammartino, M., et al. (2024). Overview of operational global and regional Ocean Colour essential ocean variables within the Copernicus marine Service. Remote Sens. 16 (23), 4588. doi:10.3390/rs16234588
Brockmann, C., Doerffer, R., Peters, M., Kerstin, S., Embacher, S., and Ruescas, A. (2016). Evolution of the C2RCC neural network for Sentinel 2 and 3 for the retrieval of ocean colour products in normal and extreme optically complex waters. Living Planet Symp. 740, 54.
Cavallini, E., Viaroli, P., Naldi, M., Saccò, M., Scibona, A., Barbieri, E., et al. (2024). Seasonal variability and hydrological patterns influence the long-term trends of nutrient loads in the river Po. Water (20734441) 16 (18), 2628. doi:10.3390/w16182628
Colella, S., Brando, V. E., Di Cicco, A., D’Alimonte, D., Forneris, V., and Bracaglia, M. (2024). Quality information document for Ocean Colour mediterranean and black sea observation product. Available online at: https://documentation.marine.copernicus.eu/QUID/CMEMS-OC-QUID-009-141to144-151to154.pdf.
Colella, S., Falcini, F., Rinaldi, E., Sammartino, M., and Santoleri, R. (2016). Mediterranean ocean colour chlorophyll trends. PloS one 11 (6), e0155756. doi:10.1371/journal.pone.0155756
Coppini, G., Lyubarstev, V., Pinardi, N., Colella, S., Santoleri, R., and Christiansen, T. (2013). The use of ocean-colour data to estimate chl-a trends in European seas. Int. J. Geosci. 04, 927–949. doi:10.4236/ijg.2013.46087
Creel, L. (2003). Ripple effects: population and coastal regions. Washington, DC: Population Reference Bureau, 1–7.
Dagum, E. B. (1980). The X-11-ARIMA seasonal adjustment method. Ottawa: Statistics Canada. Number 12–564E.
Di Paola, G., Minervino Amodio, A., Dilauro, G., Rodriguez, G., and Rosskopf, C. M. (2022). Shoreline evolution and erosion vulnerability assessment along the Central Adriatic coast with the contribution of UAV beach monitoring. Geosciences 12 (10), 353. doi:10.3390/geosciences12100353
Djakovac, T., Degobbis, D., Supić, N., and Precali, R. (2012). Marked reduction of eutrophication pressure in the northeastern Adriatic in the period 2000–2009. Estuar. Coast Shelf Sci. 115, 25–32. doi:10.1016/j.ecss.2012.03.029
Doerffer, R., and Schiller, H. (2007). The MERIS Case 2 water algorithm. Int. J. Remote Sens. 28 (3-4), 517–535. doi:10.1080/01431160600821127
Drusch, M., Del Bello, U., Carlier, S., Colin, O., Fernandez, V., Gascon, F., et al. (2012). Sentinel-2: ESA's optical high-resolution mission for GMES operational services. Remote Sens. Environ. 120, 25–36. doi:10.1016/j.rse.2011.11.026
Falcieri, F. M., Benetazzo, A., Sclavo, M., Russo, A., and Carniel, S. (2014). Po River plumepattern variability investigated from model data. Cont. Shelf Res. 87, 84–95. doi:10.1016/j.csr.2013.11.001
Falcini, F., Khan, N. S., Macelloni, L., Horton, B. P., Lutken, C. B., McKee, K. L., et al. (2012). Linking the historic 2011 Mississippi River flood to coastal wetland sedimentation. Nat. Geosci. 5 (11), 803–807. doi:10.1038/ngeo1615
Gallina, V., Torresan, S., Zabeo, A., Rizzi, J., Carniel, S., Sclavo, M., et al. (2019). Assessment of climate change impacts in the North Adriatic coastal area. Part II: consequences for coastal erosion impacts at the regional scale. Water 11 (6), 1300. doi:10.3390/w11061300
Geist, J., and Hawkins, S. J. (2016). Habitat recovery and restoration in aquatic ecosystems: current progress and future challenges. Aquatic Conservation Mar. Freshw. Ecosyst. 26 (5), 942–962. doi:10.1002/aqc.2702
Giani, M., Djakovac, T., Degobbis, D., Cozzi, S., Solidoro, C., and Umani, S. F. (2012). Recent changes in the marine ecosystems of the northern Adriatic Sea. Estuar. Coast Shelf Sci. 115, 1–13. doi:10.1016/j.ecss.2012.08.023
Giani, M., Pavlidou, A., Kralj, M., Varkitzi, I., Borja, A., Menchaca, I., et al. (2024). Assessment of the eutrophication status at mediterranean sub-basin scale, within the European marine Strategy framework directive. Sci. total Environ. 945, 173876. doi:10.1016/j.scitotenv.2024.173876
Giannini, F., Hunt, B. P., Jacoby, D., and Costa, M. (2021). Performance of OLCI sentinel-3A satellite in the northeast pacific coastal waters. Remote Sens. Environ. 256, 112317. doi:10.1016/j.rse.2021.112317
Gohin, F., Van der Zande, D., Tilstone, G., Eleveld, M. A., Lefebvre, A., Andrieux-Loyer, F., et al. (2019). Twenty years of satellite and in situ observations of surface chlorophyll-a from the northern Bay of Biscay to the eastern English Channel. Is the water quality improving? Remote Sens. Environ. 233, 111343. doi:10.1016/j.rse.2019.111343
González Vilas, L., Brando, V. E., Di Cicco, A., Colella, S., D’Alimonte, D., Kajiyama, T., et al. (2024). Assessment of ocean color atmospheric correction methods and development of a regional ocean color operational dataset for the Baltic Sea based on Sentinel-3 OLCI. Front. Mar. Sci. 10, 1256990. doi:10.3389/fmars.2023.1256990
Grilli, F., Accoroni, S., Acri, F., Bernardi Aubry, F., Bergami, C., Cabrini, M., et al. (2020). Seasonal and interannual trends of oceanographic parameters over 40 years in the northern Adriatic Sea in relation to nutrient loadings using the EMODnet chemistry data portal. Water 12 (8), 2280. doi:10.3390/w12082280
Hénocque, Y., and Coccossis, H. (2001). White paper coastal zone management in the Mediterranean. UNEP/MAP/PAP.
Henson, S. A., Sarmiento, J. L., Dunne, J. P., Bopp, L., Lima, I. D., Doney, S. C., et al. (2010). Detection of anthropogenic climate change in satellite records of ocean chlorophyll and productivity. Biogeosciences 7, 621–640. doi:10.5194/bg-7-621-2010
Howarth, R. W., and Marino, R. (2006). Nitrogen as the limiting nutrient for eutrophication in coastal marine ecosystems: evolving views over three decades. Limnol. Oceanogr. 51 (1part2), 364–376. doi:10.4319/lo.2006.51.1_part_2.0364
Karydis, M., and Kitsiou, D. (2012). Eutrophication and environmental policy in the Mediterranean Sea: a review. Environ. Monit. Assess. 184, 4931–4984. doi:10.1007/s10661-011-2313-2
Kay, R., and Alder, J. (2005). Coastal planning and management. 2nd ed. Taylor and Francis, 270. Madison Ave.
Kralj, M., Lipizer, M., Čermelj, B., Celio, M., Fabbro, C., Brunetti, F., et al. (2019). Hypoxia and dissolved oxygen trends in the northeastern Adriatic Sea (Gulf of Trieste). Deep Sea Res. Part II Top. Stud. Oceanogr. 164, 74–88. doi:10.1016/j.dsr2.2019.06.002
Malone, T. C., and Newton, A. (2020). The globalization of cultural eutrophication in the coastal ocean: causes and consequences. Front. Mar. Sci. 7, 670. doi:10.3389/fmars.2020.00670
Mann, H. B. (1945). Nonparametric tests against trend. Econometrica 13, 245–259. doi:10.2307/1907187
Marini, M., Jones, B. H., Campanelli, A., Grilli, F., and Lee, C. M. (2008). Seasonal variability and Po River plume influence on biochemical properties along western Adriatic coast. J. Geophys. Res. Oceans 113 (C5). doi:10.1029/2007jc004370
Markovic, M., Satta, A., Skaricic, Z., and Trumbic, I. (2001). Sustainable coastal tourism/an integrated planning and management approach. U. N. Environ. Programme, Div. Technol. Industry Econ. (UNEP-DTIE) “Practical Manuals Sustain. Tourism” Publ. Ser. Available online at: http://www.unep.org/pdf/DTIE_PDFS/DTIx1091xPA-SustainableCoastalTourism-Planning.pdf.
McGranahan, G., Balk, D., and Anderson, B. (2007). The rising tide: assessing the risks of climate change and human settlements in low elevation coastal zones. Environ. urbanization 19 (1), 17–37. doi:10.1177/0956247807076960
Mélin, F., Vantrepotte, V., Clerici, M., D’Alimonte, D., Zibordi, G., Berthon, J.-F., et al. (2011). Multi-sensor satellite time series of optical properties and chlorophyll-a concentration in the Adriatic Sea. Prog. Oceanogr. 91 (3), 229–244. doi:10.1016/j.pocean.2010.12.001
Mentaschi, L., Lovato, T., Butenschön, M., Alessandri, J., Aragão, L., Verri, G., et al. (2024). Projected climate oligotrophication of the Adriatic marine ecosystems. Front. Clim. 6, 1338374. doi:10.3389/fclim.2024.1338374
Mikhailova, M. V. (2002). The hydrological regime and the peculiarities of formation of the Po river delta. Water Resour. 29 (4), 370–380. doi:10.1023/a:1019614005285
Moretti, P. F., D’Alelio, D., Drago, A., Pitarch, J., Roose, P., Schön, I., et al. (2024). A process-based approach to guide the observational strategies for the assessment of the marine environment. Sustainability 16 (19), 8335. doi:10.3390/su16198335
Nechad, B., Ruddick, K. G., and Park, Y. (2010). Calibration and validation of a generic multisensor algorithm for mapping of total suspended matter in turbid waters. Remote Sens. Environ. 114 (4), 854–866. doi:10.1016/j.rse.2009.11.022
Odermatt, D., Gitelson, A., Brando, V. E., and Schaepman, M. (2012). Review of constituent retrieval in optically deep and complex waters from satellite imagery. Remote Sens. Environ. 118, 116–126. doi:10.1016/j.rse.2011.11.013
Pitarch, J., Falcini, F., Nardin, W., Brando, V. E., Di Cicco, A., and Marullo, S. (2019). Linking flow-stream variability to grain size distribution of suspended sediment from a satellite-based analysis of the Tiber River plume (Tyrrhenian Sea). Sci. Rep. 9 (1), 19729. doi:10.1038/s41598-019-56409-8
Politi, E., Brito, A. C., Gomes, M. R., Lebreton, C., and Falcini, F. (2024). Listening to stakeholders: development of water quality indicators for transitional environments using satellite data. Ocean and Coast. Manag. 253, 107140. doi:10.1016/j.ocecoaman.2024.107140
Rast, M., Bezy, J. L., and Bruzzi, S. (1999). The ESA medium resolution imaging spectrometer MERIS a review of the instrument and its mission. Int. J. Remote Sens. 20 (9), 1681–1702. doi:10.1080/014311699212416
Reimann, L., Vafeidis, A. T., Brown, S., Hinkel, J., and Tol, R. S. (2018). Mediterranean UNESCO World Heritage at risk from coastal flooding and erosion due to sea-level rise. Nat. Commun. 9 (1), 4161. doi:10.1038/s41467-018-06645-9
Ricci, F., Capellacci, S., Campanelli, A., Grilli, F., Marini, M., and Penna, A. (2022). Long-term dynamics of annual and seasonal physical and biogeochemical properties: role of minor river discharges in the north-western Adriatic coast. Estuar. Coast. Shelf Sci. 272, 107902. doi:10.1016/j.ecss.2022.107902
Ricci, F., Capellacci, S., Casabianca, S., Grilli, F., Campanelli, A., Marini, M., et al. (2024). Variability of hydrographic and biogeochemical properties in the North-western Adriatic coastal waters in relation to river discharge and climate changes. Chemosphere 361, 142486. doi:10.1016/j.chemosphere.2024.142486
Sen, P. K. (1968). Estimates of the regression coefficient based on Kendall's tau. J. Am. Stat. Assoc. 63, 1379–1389. doi:10.2307/2285891
Shiskin, J. (1978). “Seasonal adjustment of sensitive indicators,” in Seasonal analysis of economic time series. Editor A. Zellner (U.S. Department of Commerce, Bureau of the Census), 97–103.
Soana, E., Gervasio, M. P., Granata, T., Colombo, D., and Castaldelli, G. (2024). Climate change impacts on eutrophication in the Po River (Italy): temperature-mediated reduction in nitrogen export but no effect on phosphorus. J. Environ. Sci. 143, 148–163. doi:10.1016/j.jes.2023.07.008
Solidoro, C., Bastianini, M., Bandelj, V., Codermatz, R., Cossarini, G., Melaku Canu, D., et al. (2009). Current state, scales of variability, and trends of biogeochemical properties in the northern Adriatic Sea. J. Geophys. Res. Oceans 114 (C7). doi:10.1029/2008jc004838
Sprovieri, M., Ribera d’Alcalà, M., Roose, P., Drago, A., De Cauwer, K., Falcini, F., et al. (2021). Science for good environmental status: a European joint action to support marine policy. Sustainability 13 (15), 8664. doi:10.3390/su13158664
Sturm, B., Kuzmić, M., and Orlic, M. (1992). An evaluation and iterpretation of CZCS-derived patterns on the Adriatic shelf. Oceanol. Acta 15 (1), 13–23.
Tesi, T., Miserocchi, S., Goñi, M. A., Turchetto, M., Langone, L., De Lazzari, A., et al. (2011). Influence of distributary channels on sediment and organic matter supply in event-dominated coastal margins: the Po prodelta as a study case. Biogeosciences 8 (2), 365–385. doi:10.5194/bg-8-365-2011
Twilley, R. R., and Rivera-Monroy, V. (2009). Sediment and nutrient tradeoffs in restoring Mississippi River Delta: restoration vs eutrophication. J. Contemp. Water Res. and Educ. 141 (1), 39–44. doi:10.1111/j.1936-704x.2009.00035.x
UNEP (2021). Understanding the state of the ocean: a global manual on measuring SDG 14.1. 1. SDG 14.2. 1 and SDG 14.5. 1.
Vantrepotte, V., and Mélin, F. (2011). Inter-annual variations in the SeaWiFS global chlorophyll a concentration (1997–2007). Deep Sea Res. Part I. 58 (4), 429–441. doi:10.1016/j.dsr.2011.02.003
Volpe, G., Colella, S., Brando, V. E., Di Cicco, A., D’Alimonte, D., Forneris, V., et al. (2019). Ocean colour production centre.
Vona, I., and Nardin, W. (2023). Oysters' integration on submerged breakwaters offers new adaptive shoreline protection in low-energy environments in the face of sea level rise. J. Geophys. Res. Earth Surf. 128 (11), e2023JF007249. doi:10.1029/2023jf007249
Keywords: remote sensing, coastal processes, total suspended matter, Chlorophyll, Adriatic Sea, statistical trends
Citation: Vona I, Colella S, Sammartino M, Brando VE and Falcini F (2025) Positive correlation between the Po River discharge and ocean colour trends of Chl and TSM in the Adriatic Sea. Front. Remote Sens. 6:1574347. doi: 10.3389/frsen.2025.1574347
Received: 10 February 2025; Accepted: 28 March 2025;
Published: 10 April 2025.
Edited by:
Srinivas Kolluru, Florida Atlantic University, United StatesReviewed by:
Sundarabalan Balasubramanian, Geo-Sensing and Imaging Consultancy, IndiaDaniela Gurlin, Wisconsin Department of Natural Resources, United States
Copyright © 2025 Vona, Colella, Sammartino, Brando and Falcini. This is an open-access article distributed under the terms of the Creative Commons Attribution License (CC BY). The use, distribution or reproduction in other forums is permitted, provided the original author(s) and the copyright owner(s) are credited and that the original publication in this journal is cited, in accordance with accepted academic practice. No use, distribution or reproduction is permitted which does not comply with these terms.
*Correspondence: Federico Falcini, ZmVkZXJpY28uZmFsY2luaUBjbnIuaXQ=