- 1Seascape Ecology & Mapping Lab, Department of Oceanography, Dalhousie University, Halifax, NS, Canada
- 2Marine Geosciences Lab, Departamento de Oceanografia e Ecologia, Universidade Federal do Espírito Santo, Vitória, Espírito Santo, Brazil
In recent decades, the value and utility of multibeam bathymetry and backscatter data has been increasingly recognized within the field of seascape ecology (the ocean-centric equivalent of landscape ecology) to map the bio- and geodiversity of the ocean floor. More recently, multispectral multibeam backscatter has emerged as a promising endeavor in seabed classification, and its acoustic response across multiple frequencies has been studied in a range of substrates. Coral reef systems are an under-represented seabed type within this research, as these shallow water ecosystems are more commonly mapped with optical remote sensing techniques. Further investigation is necessary to determine the extent to which backscatter data can contribute to the characterization of these habitats–especially for reef systems that are beyond the reach of optical remote sensing approaches. This study investigates the multifrequency acoustic response of coral reefs in two study areas on the Abrolhos Shelf, Brazil. Backscatter mosaics and angular response curves (ARC) are investigated to explore the potential applications of these data for enhanced reef seascape characterization. In both survey areas, the acoustic mapping revealed extensive reef features, and the assessment of acoustic response was influenced by reef surface roughness and biological attributes (algal cover). Results contribute significantly to the understanding of multi-frequency signatures in reef seascapes and highlight the potential of backscatter for mapping and monitoring the health of these ecosystems. Herein, the higher frequencies were found to be fundamental for detection of subtle variations in texture in the inter-reef region of both study areas; this underscores the value of employing these frequencies in a multispectral framework for discerning heterogeneities in coarse substrate types. Furthermore, the lower frequencies were indispensable for more precise delineation of reef characteristics in the Abrolhos Channel area, whereas the higher frequencies were the most effective in identifying reefs with epibiotic cover (those characterized by flatter ARC associated with lower backscatter values) in the Forgotten Reefs region.
1 Introduction
The scientific field of seabed mapping has expanded dramatically over the past 3 decades (Misiuk and Brown, 2024), driven by the increasing demand for geospatial seafloor maps to support a variety of marine resource management needs (Baker and Harris, 2020), and innovations and evolution of the seafloor acoustic remote sensing mapping technologies (Mayer et al., 2018). Increasingly robust and complex analysis techniques have subsequently developed and are now in widescale use in the interdisciplinary field of seabed classification, which have contributed to improving our knowledge about the geodiversity of the seafloor (Strong et al., 2019; Dolan et al., 2022; Misiuk and Brown, 2024). Among the types of acoustic seafloor mapping data sets in use, multibeam backscatter has emerged as a particularly noteworthy proxy for understanding seafloor geodiversity (Che Hasan et al., 2014; Ierodiaconou et al., 2018; Harris and Baker, 2020; Menandro et al., 2023; Bai et al., 2023); more recently, a number of backscatter studies have been conducted in a range of seabed types to examine the potential advantages of multibeam systems that can operate at multiple frequencies (Feldens et al., 2018; Brown et al., 2019).
There are a number of established approaches to mapping coral reef ecosystems (see a review of historical methods for coral reef mapping in Hamylton (2017), including satellite remote sensing methods (Li et al., 2019; Zoffoli et al., 2022; Lyons et al., 2024) and multibeam bathymetry attributes to characterise and classify reef morphology (Costa and Battista, 2013; Diesing and Thorsnes, 2018; Menandro et al., 2020; Vieira et al., 2023). However, there has been limited investigation of how multibeam backscatter can also add value to characterizing coral reef habitat, and whether biological attributes of reef systems (e.g., density and cover of epibiota) can be measured based on their backscattering records as a function of survey frequency.
In a non-exhaustive review of recent studies on multibeam backscatter, reefs do not feature among the seafloor types studied with backscatter (Brown et al., 2019; Fezzani et al., 2021; Menandro et al., 2022a; Vieira et al., 2023), although other forms of reef habitat have been investigated and characterized using acoustic backscatter measurements [e.g., boulder substratum in Trzcinska et al. (2021), rock reefs in Fakiris et al. (2019), bedrock outcrops in Recouvreur et al. (2024)]. With multifrequency backscatter, this panorama becomes more evident, with a few published papers on mussels reefs (Schulze et al., 2022) and rhodolith beds (Menandro et al., 2023), but none on coral reefs to date.
The purpose of this work is to analyze the multispectral acoustic response of coral reefs through the investigation of backscatter mosaics and angular response curves and explore potential applications of these data streams for improved reef seascape characterization, which may encompass the heterogeneity of the reef morphology and the interconnectivity with inter-reef areas. Specifically, we investigate the capability of multispectral backscatter to discriminate additional biological and/or morphological characteristics of the reef ecosystems (e.g., macroalgae coverage; reef surface complexity) which other methods may fail to capture. Moreover, important and novel results have been added to the catalog of seabed types studied with backscatter within the two regions of the Abrolhos Shelf–an area that is particularly important for conservation given the extensive presence of reefs (Leão, 2002; Moura et al., 2013; Ferreira et al., 2020; Zoffoli et al., 2022). Specific goals from the study were: i) investigate whether reefs have a distinctive backscatter response compared to non-reef areas; ii) analyse whether the acoustic response on reefs is frequency dependent based on mosaics and angular response curves; iii) apply an image segmentation based on multi-frequency backscatter mosaics to analyze the output of each frequency to discriminate reef and inter-reef areas; iv) explore how roughness and macroalgae cover affect multispectral backscattering.
2 Methodology
Multispectral multibeam surveys were carried out in two study areas (1 -Abrolhos Channel: 2 - Forgotten Reefs), both located on the Abrolhos Shelf, between the states of Espirito Santo and Bahia (Brazil) (Figure 1A). The largest and richest reef environment in the South Atlantic is found in this section of the Brazilian continental shelf (Leão, 2002; Moura et al., 2013).
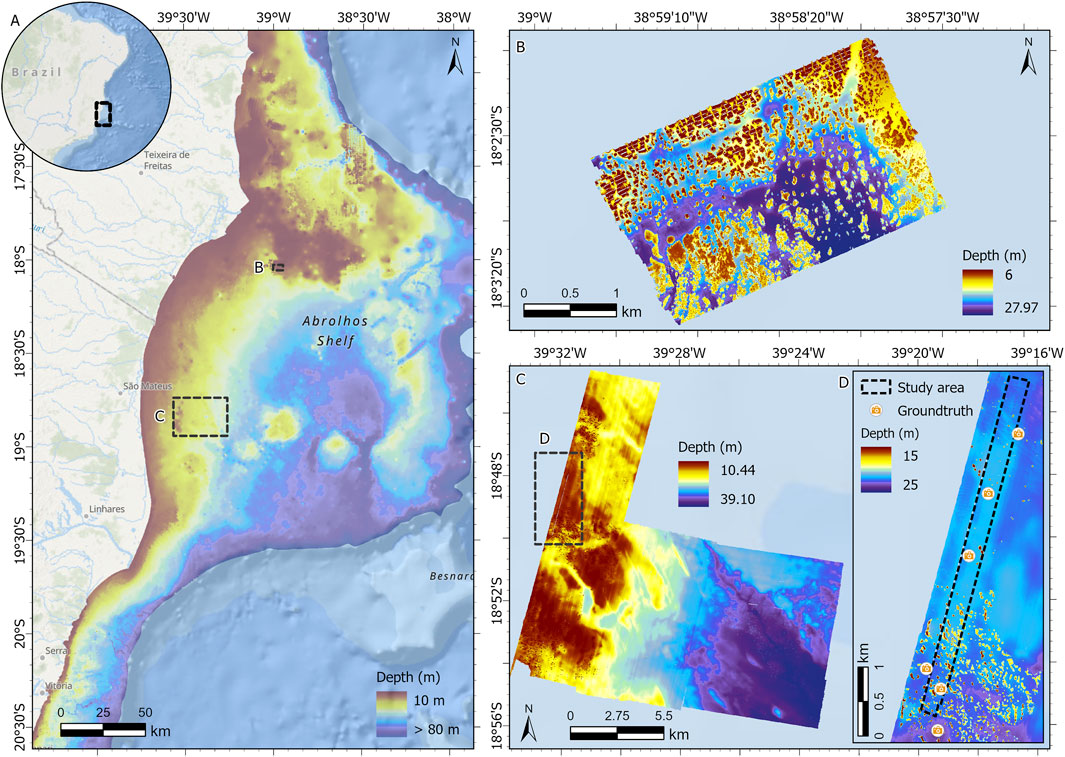
Figure 1. Study area. (A) Overview of the continental shelf showing the location of the survey sites; (B) Digital bathymetric model of the Abrolhos Channel; (C) Digital bathymetric model of Forgotten Reefs; (D) Bathymetry detail showing part of the Forgotten Reefs and the outline of the study area.
The Abrolhos Channel survey site (Figure 1B) covers an area of approximately 6 km2. The area comprised a complex morphology, with depths ranging from 6 to 28 m. The Forgotten Reefs survey area is located approximately 100 km further to the south (Figures 1C,D). This region has already been the subject of studies either involving the automated classification of reefs using the RSOBIA tool [in which the output was based on slope, roughness, and backscatter–see Menandro et al. (2022b)] or exploring the geodiversity of these features within the context of relative sea changes (Vieira et al., 2023). Although more than 200 km2 of the Forgotten Reefs have already been surveyed using MBES, the focus area for this study (Figure 1D) covers approximately 1.5 km2 of seafloor with depths ranging from 13 to 25 m. The study area was selected based on the availability of ground truth samples, and the extent of the dataset coverage encompassing a single day of acoustic survey covering both reef and non-reef regions.
2.1 Acoustic data acquisition and processing
The surveys were carried out in July of 2019 (Forgotten Reefs) and in April 2023 (Abrolhos Channel). Acoustic data acquisition at multiple frequencies was performed using an R2Sonic 2024 multibeam echosounder system (MBES). The multispectral mode was configured to collect data at 170, 280, 400, and 700 kHz operating in equiangular mode. The 700 kHz did not operate in Abrolhos Channel study area due to the effects of depth on data quality. Parameters such as swath angle (90° for Forgotten Reefs and 120° for Abrolhos Channel), power (212 dB for Forgotten Reefs and Abrolhos Channel), pulse length (80 µsec for Forgotten Reefs and Abrolhos Channel), gain (adjusted for each frequency in each study area after preliminary survey test), and spreading (5 dB for Forgotten Reefs and for Abrolhos Channel) were held constant. The MBES was paired with a POS MV Wave Master Inertial Navigation System (INS). In the course of the survey, sound velocity profiler (SVP) casts were deployed every 3 h using a Valeport Mini, providing sound velocity, salinity, temperature and pressure. This information is essential to ensure the correction of sound velocity effects and for the calculation and application of the absorption coefficient for backscatter processing.
In accordance with the International Hydrographic Organization, the bathymetric processing consisted of a manual cleaning of the erroneous soundings and a tidal correction using QPS Qimera 2.5.4. A digital bathymetric model was output at 2 m resolution (combining all frequencies), which allowed the interpretation of the morphology of the area and delineation of the reefs in detail. The bathymetric data were also used as input to calculate the roughness of the seafloor/reefs using the MultiscaleDTM package (Ilich et al., 2023) in R - the roughness index-elevation is calculated based on the standard deviation of residual topography in a focal window. As reefs are readily conspicuous features, the roughness surface was used as a reference dataset for the purpose of a visual comparison with the backscatter segmentation analysis.
The multispectral backscatter was processed following the guidelines and recommendations found in Lurton et al. (2015), Lamarche and Lurton (2018) and Schimel et al. (2018). The main steps involved the frequency filtering, correction of acquisition parameters for each frequency, and AVG correction for the mosaics–which were output at 2 m resolution. In addition, corrected backscatter values were extracted to plot angular response curves (ARC) – these data are extracted prior to the mosaic corrections such as AVG. All the backscatter processing was completed using QPS FMGT 7.9.6.
Both mosaics and angular response curves were analyzed to investigate the multifrequency backscatter and explore the across-frequency response of reef and inter-reef regions (herein, all the unconsolidated sediment seabed which was not reef is considered as inter-reef seafloor). The ARC represents a patch section of 30 pings sampled from nadir to the outer beam on both the port and starboard sides of the swath, and these were manually selected at different locations as representative examples from the different seafloor types.
2.2 Ground truthing data
Underwater images collected using a drop camera system were used to ground truth reef characteristics at the Forgotten Reefs study site; no drop camera images were collected in the Abrolhos Channel due to logistical and financial limitations of the project. The drop camera system consisted of a pyramidal metal frame (60 cm × 60 cm) coupled with two cameras (GoPro Hero 7) and lights. The system was set up with one camera looking down towards the seafloor (at the base of the structure) and a second camera looking sideways across the seafloor. In the absence of an underwater positioning system, the position of the image was estimated in relation to the boat’s DGPS at the moment the structure reached the bottom (positional accuracy estimated at 20 m). No automatic or quantitative analysis of the collected footage was conducted, but a qualitative description was made based on a classification scheme similar to the CATAMI model (Althaus et al., 2015).
2.3 Image-based analysis
Following preliminary visual inspection of the mosaics and assessment of the uncalibrated backscatter values for each frequency, an image-based segmentation approach was applied to the mosaics. The backscatter mosaic of each frequency was segmented using the “mean shift” algorithm (Yizong, 1995; Comaniciu and Meer, 2002) in ArcGIS Pro using the maximum spectral detail (20) and spatial detail as default (15), but a minimum segment size of 50 pixels due to the high heterogeneity of the study sites. The mean shift is a mode-seeking algorithm that assigns data points to clusters based on their position relative to the local maxima of a multivariate kernel density estimate. As there is a paucity of ground truth data to estimate accuracy metrics, the backscatter segmentation result was visually compared to the roughness surface to visually analyze the differing capabilities across frequencies in discriminating the reef features and inter-reef bottom.
3 Results
The digital bathymetric model revealed the presence of extensive reef features in both survey areas (see 3D frames on Figure 2). At the Forgotten Reef study area, the tops of the reefs typically reached depths between 14 and 16 m. At the Abrolhos Channel study area, the reefs were more typically reef banks, with shallower reefs in the northwestern region of the survey area (reef tops mostly between 8 and 12 m) and deeper reef tops in other areas (mostly between 15 and 22 m depth). There is considerable variation in the dimensions of the reef structures observed in both study areas–average of 370 m2 for Forgotten Reefs and 580 m2 for Abrolhos Channel, with the smallest measured diameters in both sites reaching approximately 10 m and the longest banks in Abrolhos Channel extending to over 200 m. Surface roughness calculated from the bathymetry for each study area is presented in Figure 2. The greatest roughness was observed over the reef features in both areas, in contrast to low surface roughness between reefs.
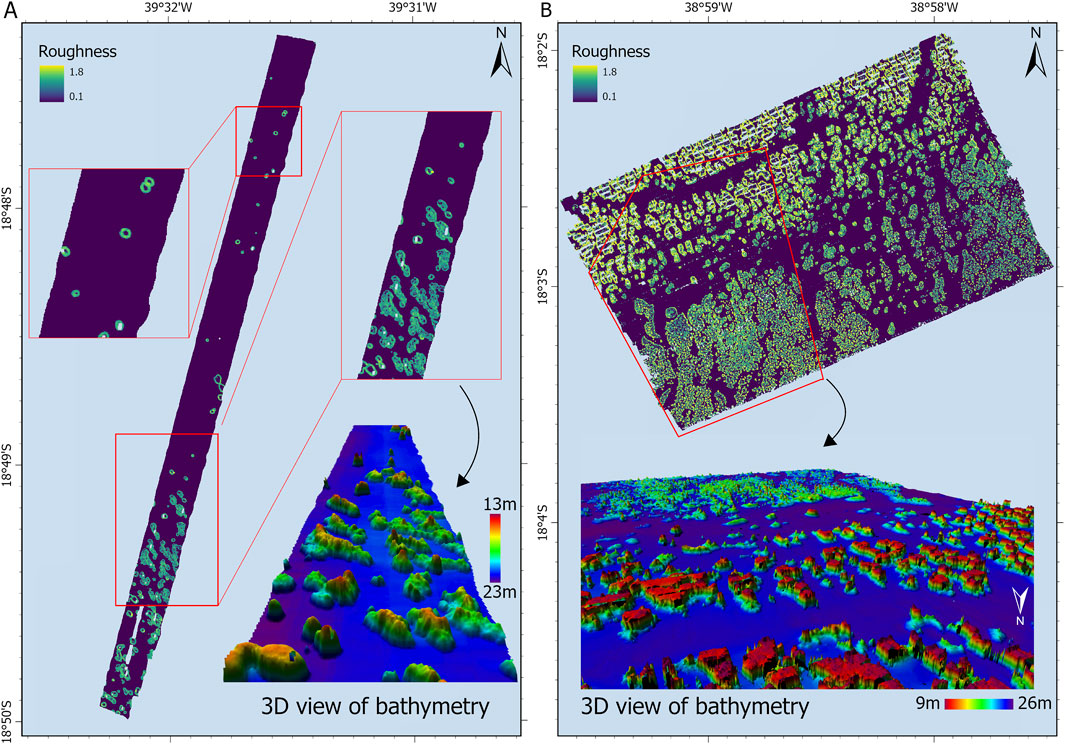
Figure 2. Frames showing the roughness for each study areas (A)–Forgotten Reefs; (B) Abrolhos Channel, and detailed 3D viewing of reefs.
3.1 Multispectral backscatter
3.1.1 Forgotten Reefs
Backscatter mosaics from the Forgotten Reefs showed higher backscatter values for the inter-reef area and lower backscatter intensities over the reef features at all frequencies (Figure 3); these observed discrepancies are particularly pronounced at 400 kHz (boxplot on Figure 3). Despite limitations of using uncalibrated backscatter data, it is difficult to visually distinguish the differences in backscatter across frequencies between the reef and inter-reef areas from the mosaics; they appear slightly greater between 280 and 400 kHz (mean difference = 2.52 dB) than between 280 and 170 kHz (mean difference = 1.55 dB). The 700 kHz frequency resulted in values that were significantly higher than those of the other frequencies, while it also showed higher values for the inter-reef region. In general, the 280 kHz mosaic showed higher values in the inter-reef region when compared to the other frequencies (170 and 400 kHz), while this panorama is not so well established for the reef features.
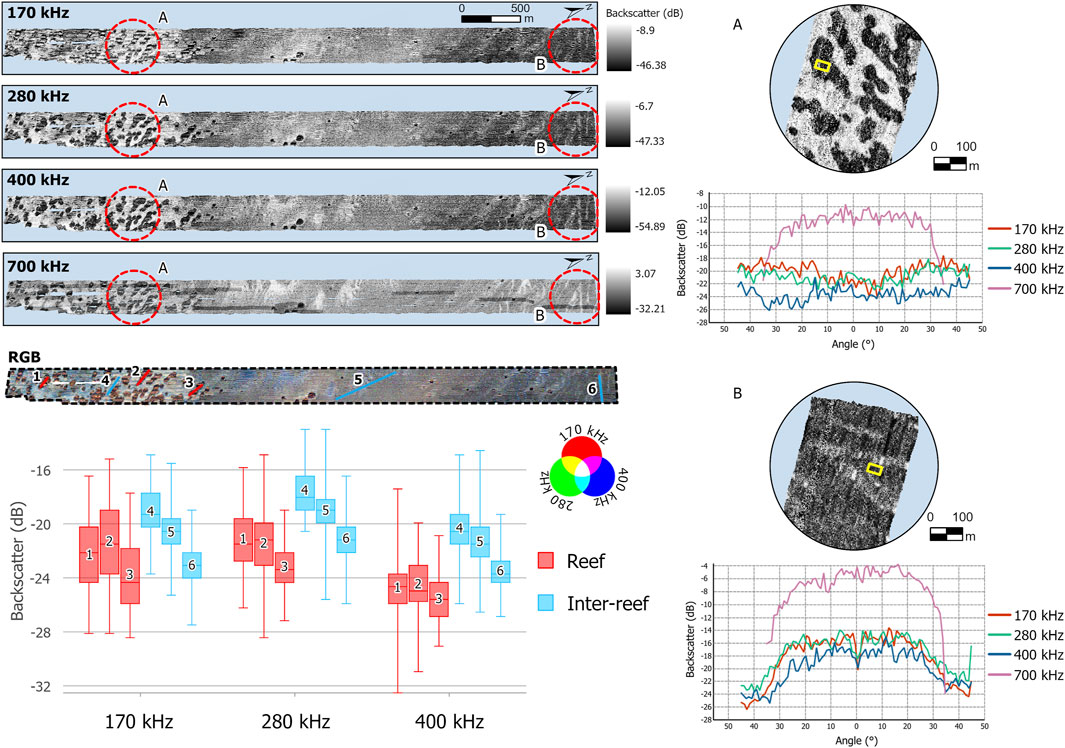
Figure 3. Forgotten reefs results - mosaics for each frequency and RGB composite mosaic with some enumerated profiles over reef and inter-reef regions graphically represented in the boxplot. Also, (A) an example of angular response curve extracted over the reef feature; (B) an example of angular response curve extracted over the inter-reef region.
By observing the ARC (Figure 4), we can draw similar conclusions–with lower backscatter values recorded over the reef features than for inter-reef regions, and with a well defined and frequency-independent backscatter difference throughout the swath. Additionally, other details came to light looking at the corrected backscatter values, such as the ARC shape for inter-reef regions indicating higher backscatter level loss with increasing incidence angles compared to those ARC over reef features, and the larger differences between the far-nadir region and the nadir region for the lower frequencies (170 and 280 kHz) over the reefs - i.e., increasing backscatter values in the outermost beams, and a flatter shape for the 400 kHz.
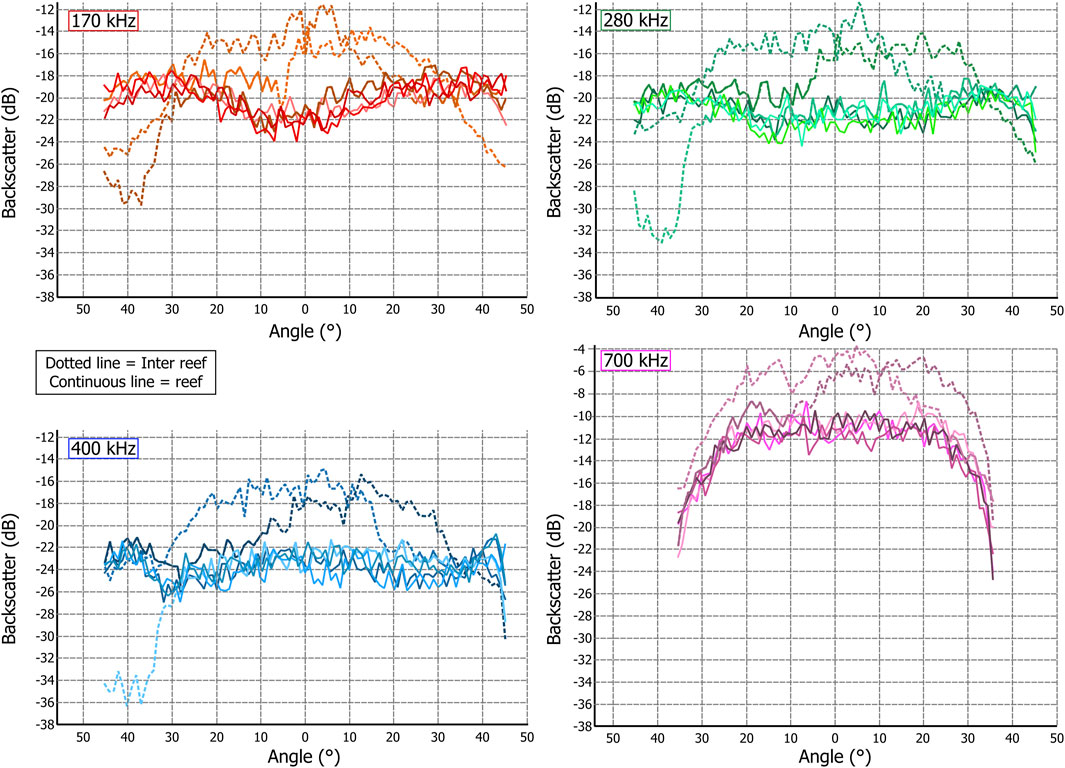
Figure 4. Comparison of angular response curves for each frequency extracted from reef and inter-reef locations in Forgotten Reefs region.
3.1.2 Abrolhos Channel
In general, the interpretation of the mosaics (Figure 5) allows us to infer the occurrence of two types of inter-reef regions - one with the highest mosaic backscatter values and another with the lowest. In addition, the different reefs observed by bathymetry and roughness (Figures 1, 2) do not show much backscatter difference, except for the shadow zones marking some of the shallower reef walls, which end up generating patches of low backscatter.
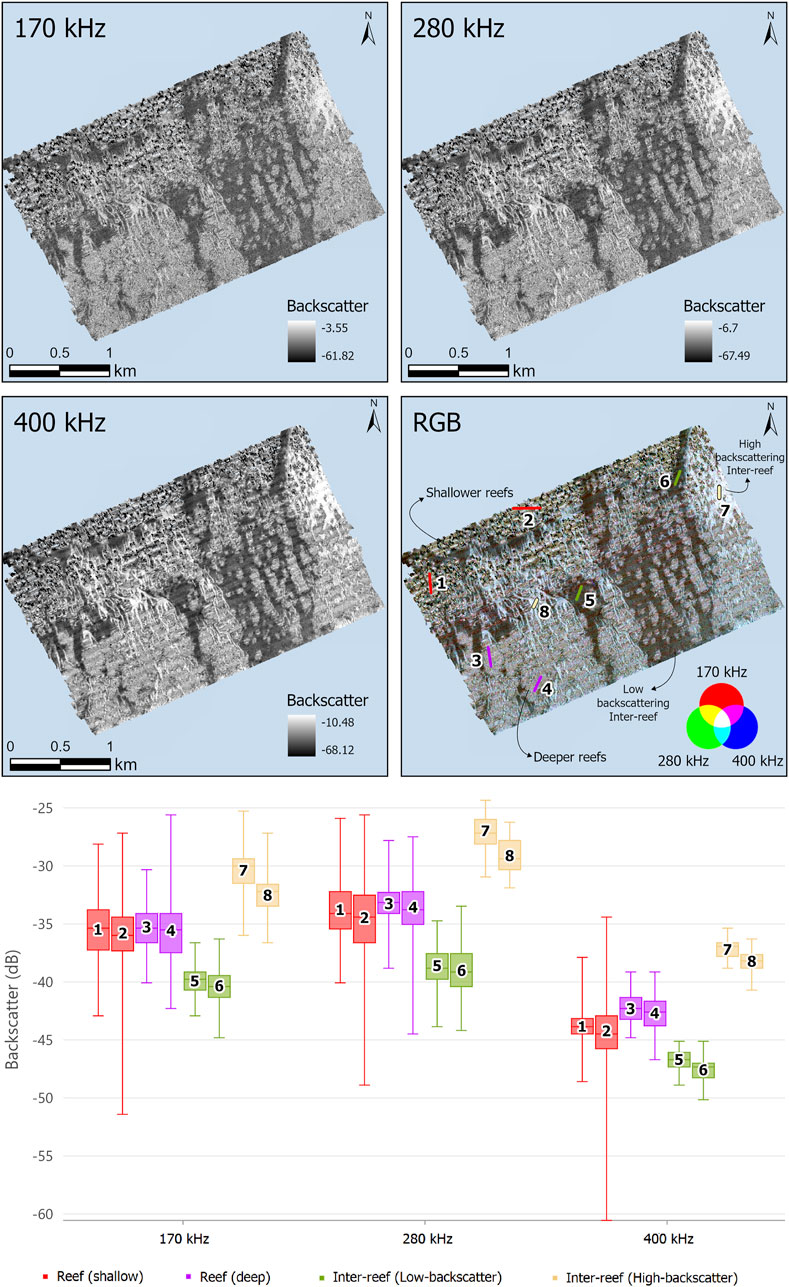
Figure 5. Abrolhos Channel results - backscatter mosaic for each frequency, RGB mosaic composite band, and boxplot with uncalibrated backscatter values extracted from specific profiles displayed on RGB mosaic.
Distinguishing the reefs solely based on the single-frequency mosaics is difficult in some sections (e.g., shallow reef against low backscatter inter-reef region in the 400 kHz mosaic), but this is greatly facilitated by observing the RGB composite band mosaic, in which the reefs become easily recognizable from their surrounding inter-reef region. From the RGB, in the high backscatter inter-reef seafloor, all the frequencies recorded the highest values, with those of 280 kHz being predominantly higher. In contrast, all the frequencies returned the lowest values for the low backscatter inter-reef bottom type, with smaller differences between 170 and 280 kHz. Over the reef features, the frequencies of 170–280 kHz recorded the higher backscatter strengths compared to those from 400 kHz. Additionally, in general, the difference between reef and low backscatter inter-reef seafloor was more pronounced for lower frequencies even considering the caveat of using absolute values for uncalibrated backscatter.
Investigation of the corrected backscatter values through the angular response curves (Figure 6), revealed that the two types of reef morphology (shallower and deeper) exhibit no apparent ARC differences, while the two types of inter-reef substrate are easily distinguishable from the ARC. Even though these are not calibrated backscatter values, it is worth noting that the differences in backscatter intensities across ensonification angles between 170 and 280 kHz appear smaller than the differences between 280 and 400 kHz. Overall, in the near nadir region of the ARCs, the backscatter values over reef features are more similar to those of the low backscatter inter-reef bottom; as the angle of incidence increases (approximately from 25°), the backscatter values increase and get closer to the curve of the high backscatter inter-reef bottom. In other words, this indicates the greater increase in backscatter values in the outermost regions of the swath over the reef beds compared to the inter-reef regions.
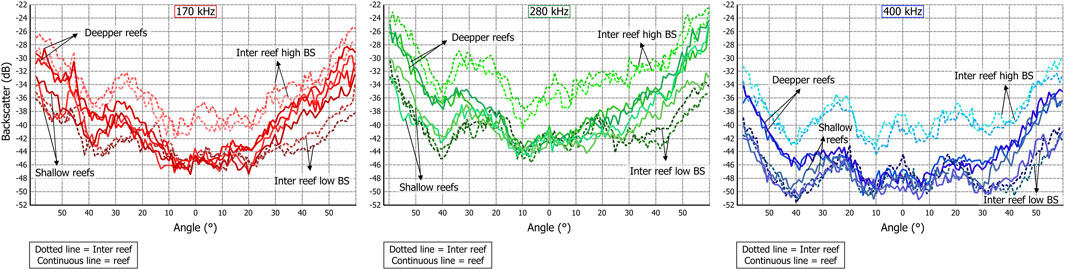
Figure 6. Comparison of angular response curves for each frequency extracted from reef and inter-reef locations in Abrolhos Channel region.
3.2 Ground truth
The underwater images (Figure 7) were described visually based on the hierarchy proposed by the CATAMI model classification (Althaus et al., 2015), indicating the physical substrate (consolidated and unconsolidated), and identifying the presence of coral reefs and macroalgae. The reef was only validated at one station due to the risks of losing the camera equipment and/or damaging the reefs, but the data did reveal the presence of dense coral and macroalgae. The inter-reef seabed comprised of unconsolidated sediment, mostly sand with carbonate fragments and occasional sparse presence of algae.
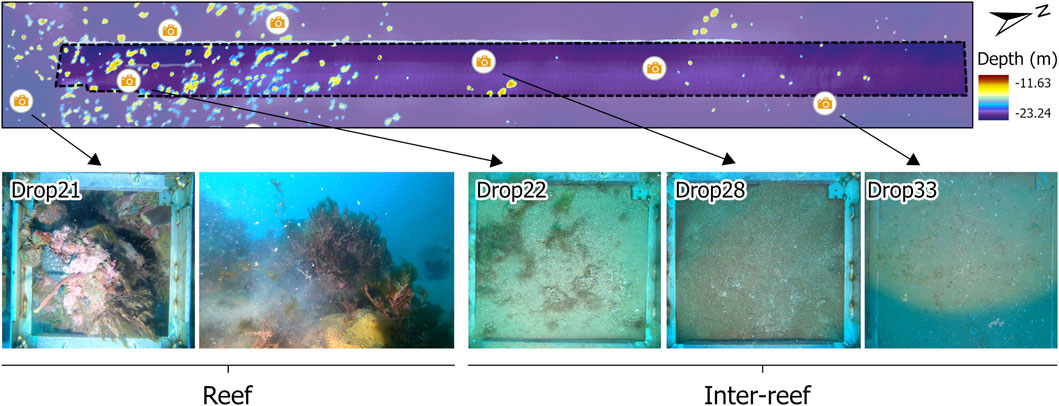
Figure 7. Examples of underwater images collected in reef and inter-reef seafloor–Forgotten Reefs site. The black dotted line delineates the study area in the upper bathymetric inset.
3.3 Image-based analysis
The results from the image segmentation are summarized in Figure 8.
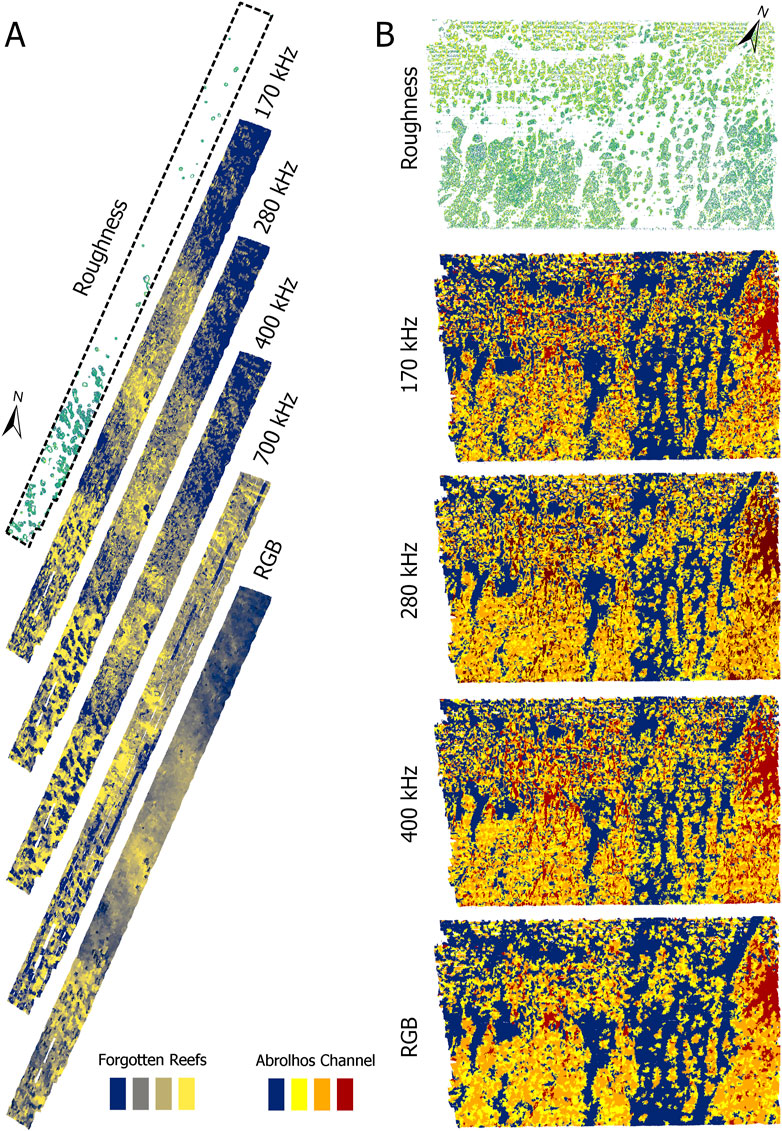
Figure 8. Roughness surface emphasizing the reef features and image-based segmentation based on backscatter mosaics for (A) Forgotten Reefs; (B) Abrolhos Channel.
In the Forgotten Reef area (Figure 8A), reef recognition was more detailed with the 400 kHz frequency, with the features outlined more clearly. This suggests that the reef cover or texture responds in different ways according to frequency. In the inter-reef regions, where the sediment is composed of sand and carbonate fragments, the higher frequencies were also more effective at differentiating the disparities present. In fact, the 700 kHz frequency was able to identify some bedforms in the northernmost study area. Segmentation based on RGB (170, 280 and 400) was found to be more advantageous for differentiating inter-reef bottoms but did not improve the delineation of reef features.
In the Abrolhos Channel region (Figure 8B), the seabed features in the inter-reef areas were resolved in more detail in the 400 kHz mosaics compared to the lower frequency mosaics. Additionally, the differentiation between reef bottom (according to the roughness reference) and low backscatter inter-reef bottom was more pronounced with the lower frequency input, resulting in a clearer delineation of the edges of the reef features. The RGB output was found to be a more effective method in differentiating between reefs and low backscatter inter-reef bottom, while the single-frequency 400 kHz result proved to be more suitable for the delineation of high backscatter inter-reef seafloor. Consequently, if the objective was to identify reef features in this specific case, it would be preferable to utilize only the lowest frequency considering this range (170–400 kHz), maximizing the distinction between reef and inter-reef regions and enhancing the along-track resolution of the data (given that there would be no alternating pings for each frequency).
4 Discussion
The exploration of the multibeam backscatter response on reefs through this study has demonstrated its great potential as a tool for enhancing our understanding of reef and inter-reef habitats, providing relevant information on the substratum types studied, especially where reefs occur beyond the reach of optical remote sensing approaches. It should be noted that both study areas investigated here are not represented in the global distribution of coral reef based on satellite data presented in Lyons et al. (2024), and these areas are beyond the water depths which can be surveyed using optical remote sensing approaches. The application of acoustic remote sensing for mapping these systems, combining analysis using elements of both the corrected backscatter mosaics and the angular response curves [as recommended in other studies - see Che Hasan et al. (2014), Menandro et al. (2022a)] has proven an effective approach for reef and inter-reef characterization.
Overall, the backscatter observed from the single-frequency mosaics provided an important baseline for understanding the distribution of seabed types, particularly if the aim had been to classify the presence or absence of reefs–which was demonstrated to be feasible based on the image segmentation results (Figure 8). Based on the backscatter mosaics of the Forgotten Reef region, although the distinction between reef and inter-reef seafloor was more straightforward and frequency independent - with lower median uncalibrated backscatter values for the reef features compared to the surrounding area, the 400 kHz was found to be more effective in delineating the reef features in the segmentation output. In contrast, in the Abrolhos Channel study area, the distinction between reef and inter-reef was not so clear in some regions for the higher frequency, and the RGB mosaic proved to be an effective solution to easily differentiate the reefs from the inter-reef bottom from the combined backscatter composite, thus confirming the importance of concatenating multispectral information to achieve a better product. Although different frequencies performed differently across the seafloor types, the use of multifrequency approach suggests benefits for distinguishing seabed composition differences and aiding in segmentation result.
Reefs comprise a complex habitat with heterogeneous morphologies of different sizes, roughness, composition, and biological attributes (such as epibiotic cover). In contrast to most substrate types (e.g., bedrock, and unconsolidated sediments of various grain sizes), they therefore produce a more complex backscatter response. Previous studies exploring the use of backscatter to characterize reef habitat have reported a wide range of backscatter responses depending on the different types and features of reef. For example, Roberts et al. (2005), used MBES to map cold-water coral reefs, which were recorded with low-intensity backscatter. In contrast, Schulze et al. (2022) reported that mussel-covered reefs were characterized by generally high-backscatter intensities (using 200, 400, 550 and 700 kHz). Pillay et al. (2021) mapped coral reef or scattered reef outcrop based on high reflectivity of the backscatter at 400 kHz in areas with high species diversity. Recouvreur et al. (2024) argued the high reflectivity shown by the backscatter values also illustrates induration characteristics of potential bedrock occurrence. Finally, Zoffoli et al. (2022) showed relevant findings very similar with the results from Forgotten Reefs site, with low reflectance values for reefs and algae based on satellite remote sensing techniques. In the Forgotten Reefs region, the ARCs allowed the angular response to be detailed along with the mosaic results, providing a more refined understanding of the types of substrates being studied. The inter-reef bottom, which showed the highest mean backscatter values in the mosaics, generally showed an ARC shape with a decrease in backscatter intensity with increasing incidence angle from 20° and relatively high backscatter values, typical of sandy sediment bottoms [see APL model–Applied Physics Laboratory (1994) curve comparable to fine sand at 170, 280 and 400 kHz in Menandro et al. (2022a) and similar to the one obtained for sand at 150 kHz in Trzcinska et al. (2021)]. It is important to note that this information was validated by the underwater images collected by the drop camera. Conversely, over reef features, the ARCs exhibit a flatter profile, typical of rocky bottoms [APL model, see Jackson and Richardson (2007)] in which the backscatter level loss is small across the entire swath. Nevertheless, the flatter shape of the curves (including increased values in the outermost beams for the lower frequencies) and the lower backscatter values clearly noted by the mosaics have drawn attention to a pattern in which the high roughness of the reefs is an important factor driving the shape of the curves, while the substrate, including the macroalgae cover of the reefs, appears to be a key factor in explaining the low values observed.
The investigation of algal dominated habitat using backscatter is not unprecedented, and past studies have demonstrated that the backscatter characteristics may be strongly influenced by the specific species and growth-forms of algae. Studies have reported distinctive backscatter differences between vegetated and non-vegetated substratum (Stocks et al., 2019), the detection of species of kelp in a region with low seafloor backscatter (McGonigle et al., 2011) or detected in the water-column backscatter data (McGonigle et al., 2011; Schimel et al., 2020), and records of seagrass with high backscatter strength due to the leaf, gas bubbles and rhizomes (De Falco et al., 2010; Schulze et al., 2022). Fakiris et al. (2019) observed lower backscatter values on the mean ARC for vegetated soft bottoms than unvegetated; moreover, slight differences between boulders covered by mussels and by red algae were noted by Trzcinska et al. (2021). Similarly, Porskamp et al. (2022) suggested the presence of dense algae on consolidated substrate could be a possible cause for near nadir-region beams (0°–30°) to have low backscatter intensities, and Schulze et al. (2022) observed a strong increase of backscatter values at high angles of incidence, suggesting that the larger footprint in the outermost beams contributes to a higher number of seagrass leaves ensonified. In one of the examples of the ARC for the Forgotten Reefs shown in Figure 4, the curve was extracted partly on a reef and partly on a sandy bottom, which succinctly summarizes the results obtained for this area - low backscatter values and a flat curve over the reefs, and higher backscatter values with decreasing backscatter with increasing angle of incidence for the inter-reef region. In the Abrolhos Channel, although the curves displayed an overall increasing value in the far-nadir region, this difference was greater for the curves over the reef features. This acoustic trend suggests that, even considering that the absolute values are not calibrated, this more pronounced increase between nadir and far nadir on the reefs could be caused a greater roughness of these features compared to the inter-reef bottom. However, the backscatter values, which were intermediate between the two types of inter-reef bottoms observed, do not seem to indicate the presence of macroalgae covering the reefs as much as observed in the Forgotten Reefs. The roughness throughout the inter-reef regions and different backscatter levels indicates different sediment types.
An absolute calibrated backscatter level would not be a strict requirement for some applications related to qualitative interpretation [for example, see Lamarche et al. (2011)]. However, comparing the backscatter values of the different morphologies and between the two study areas can be a somewhat speculative task, since the values are not calibrated and there is a 3-year difference between the surveys, which ultimately introduces the risk of electronic components within the MBES system aging that can affect the backscatter measurements. Backscatter calibration, which is currently one of the main topics for advances in backscatter studies and technologies (Fezzani et al., 2021; Van Dijk et al., 2024), suggests that it is particularly challenging for reef bottoms, since you no longer have a single type of substrate (e.g., sand, mud), but rather the complex mixture of geo- and biodiversity of a habitat (with spatial and temporal variability on surface roughness, composition, and cover).
These results offer important contributions both from the point of view of multi-frequency signatures for reefs, and draw attention to potential applications of backscatter for mapping and studying the health of these ecosystems. The presence or absence of macroalgae on reefs (and whether they are transparent for certain frequencies) may offer indications of reef health [but see Smith et al. (2016)], and emphasizes the importance of backscatter as a variable for geo and biodiversity studies. Measuring the contribution of the use of the different frequencies was beyond the scope of this study, but it is worth noting that in the most heterogeneous region (Abrolhos channel), the multispectral data were particularly important in obtaining the RGB mosaic, which was a valuable input for discriminate reef features from inter-reef sediments using the image segmentation approach. Moreover, the large amount of spectral acoustic information in sensitive habitats with coral reefs and significant macroalgae coverage is of great importance to include these different habitats in the catalog of seabed types successfully studied by multispectral backscatter, and future studies could yield potential benefits by combining the multifrequency geomorphometry and the multispectral backscatter response with the roughness captured and measured by structure from motion.
5 Conclusion
The results from this investigation highlight the complexities in backscatter response across different reef habitats. While the pattern observed displayed an overall frequency independent response, the reef roughness and biological attributes were key factors to compare against the acoustic response.
The higher frequencies were essential for capturing in greater detail the small differences in texture in the inter-reef substrate of both locations. This makes the use of these frequencies in the multispectral context important for recognizing heterogeneities in coarse substrate type. In addition, while the lower frequencies were crucial for more accurate differentiation of reef features in the Abrolhos Channel region, it was the higher frequencies that most effectively distinguished reefs with epibiotic cover in the Forgotten Reefs. The combined use of ARC along with the backscatter mosaics successfully provided a more refined response from the seafloor at different frequencies. In the Forgotten Reefs site, the low mean backscatter values observed both in the mosaics and the shape of the ARCs strongly suggested the presence of macroalgae cover (surface or material with low acoustic impedance) over the rough hardground features.
Data availability statement
The raw data supporting the conclusions of this article will be made available by the authors, without undue reservation.
Author contributions
PM: Conceptualization, Formal Analysis, Investigation, Writing–original draft. FV: Formal Analysis, Methodology, Writing–original draft. AB: Conceptualization, Funding acquisition, Writing–review and editing. CB: Conceptualization, Funding acquisition, Writing–review and editing.
Funding
The author(s) declare that financial support was received for the research, authorship, and/or publication of this article. Data collection was carried out as part of Environmental Monitoring Program (PMBA–Fundacao Renova-FEST), PELD Abrolhos (CAPES-CNPq) and PRONEX-GEOMAR (Fapes). Funding was provided by the Ocean Frontier Institute, through an award from the Canada First Research Excellence Fund.
Acknowledgments
This research is part of Benthic Ecosystem Mapping and Engagement (BEcoME) Project (www.ofibecome.org).
Conflict of interest
The authors declare that the research was conducted in the absence of any commercial or financial relationships that could be construed as a potential conflict of interest.
The author(s) declared that they were an editorial board member of Frontiers, at the time of submission. This had no impact on the peer review process and the final decision.
Publisher’s note
All claims expressed in this article are solely those of the authors and do not necessarily represent those of their affiliated organizations, or those of the publisher, the editors and the reviewers. Any product that may be evaluated in this article, or claim that may be made by its manufacturer, is not guaranteed or endorsed by the publisher.
References
Althaus, F., Hill, N., Ferrari, R., Edwards, L., Przeslawski, R., Schönberg, C. H. L., et al. (2015). A standardised vocabulary for identifying benthic biota and substrata from underwater imagery: the CATAMI classification scheme. PLOS One 10 (10), e0141039. doi:10.1371/journal.pone.0141039
Applied Physics Laboratory - APL (1994). University of Washington. APL-UW High-Frequency Ocean Environmental Acoustic Models Handbook, Seattle, WA, USA: Applied Physics Laboratory, University of Washington. Technical Report APL-UW TR9407.
Bai, Q., Mestdagh, S., Snellen, M., and Simons, D. G. (2023). Indications of marine benthos occurrence from multi-spectral multi-beam backscatter data: a case study in the North Sea. Front. Earth Sci. 11, 1140649. doi:10.3389/feart.2023.1140649
Baker, E. K., and Harris, P. T. (2020). “Habitat mapping and marine management,” in Seafloor Geomorphology as Benthic Habitat (Elsevier), 17–33. doi:10.1016/B978-0-12-814960-7.00002-6
Brown, C., Beaudoin, J., Brissette, M., and Gazzola, V. (2019). Multispectral multibeam echo sounder backscatter as a tool for improved seafloor characterization. Geosciences 9 (3), 126. doi:10.3390/geosciences9030126
Che Hasan, R., Ierodiaconou, D., Laurenson, L., and Schimel, A. (2014). Integrating multibeam backscatter angular response, mosaic and bathymetry data for benthic habitat mapping. PLoS One 9 (5), e97339. doi:10.1371/journal.pone.0097339
Comaniciu, D., and Meer, P. (2002). Mean shift: a robust approach toward feature space analysis. IEEE Trans. Pattern Analysis Mach. Intell. 24 (5), 603–619. doi:10.1109/34.1000236
Costa, B. M., and Battista, T. A. (2013). The semi-automated classification of acoustic imagery for characterizing coral reef ecosystems. Int. J. Remote Sens. 34 (18), 6389–6422. doi:10.1080/01431161.2013.800661
De Falco, G., Tonielli, R., Di Martino, G., Innangi, S., Simeone, S., and Michael Parnum, I. (2010). Relationships between multibeam backscatter, sediment grain size and Posidonia oceanica seagrass distribution. Cont. Shelf Res. 30 (18), 1941–1950. doi:10.1016/j.csr.2010.09.006
Diesing, M., and Thorsnes, T. (2018). Mapping of cold-water coral carbonate mounds based on geomorphometric features: an object-based approach. Geosciences 8 (2), 34. doi:10.3390/geosciences8020034
Dolan, M. F. J., Bøe, R., and Bjarnadóttir, L. R. (2022). Delivering seabed geodiversity information through multidisciplinary mapping initiatives: experiences from Norway. GEUS Bull. 52. doi:10.34194/geusb.v52.8325
Fakiris, E., Blondel, P., Papatheodorou, G., Christodoulou, D., Dimas, X., Georgiou, N., et al. (2019). Multi-frequency, multi-sonar mapping of shallow habitats—efficacy and management implications in the national marine park of Zakynthos, Greece. Remote Sens. 11 (4), 461. doi:10.3390/rs11040461
Feldens, P., Schulze, I., Papenmeier, S., Schönke, M., and Schneider Von Deimling, J. (2018). Improved interpretation of marine sedimentary environments using multi-frequency multibeam backscatter data. Geosciences 8 (6), 214. doi:10.3390/geosciences8060214
Ferreira, L. C., Bastos, A. C., Amado Filho, G. M., Leite, M. D. A., Boni, G. C., Moraes, F., et al. (2020). “Submerged reefs in the Abrolhos Shelf: morphology and habitat distribution,” in Seafloor Geomorphology as Benthic Habitat (Elsevier), 519–532. doi:10.1016/B978-0-12-814960-7.00030-0
Fezzani, R., Berger, L., Le Bouffant, N., Fonseca, L., and Lurton, X. (2021). Multispectral and multiangle measurements of acoustic seabed backscatter acquired with a tilted calibrated echosounder. J. Acoust. Soc. Am. 149 (6), 4503–4515. doi:10.1121/10.0005428
Hamylton, S. M. (2017). Mapping coral reef environments: a review of historical methods, recent advances and future opportunities. Prog. Phys. Geogr. Earth Environ. 41 (6), 803–833. doi:10.1177/0309133317744998
Harris, P. T., and Baker, E. K. (2020). Seafloor geomorphology as benthic habitat: GeoHAB atlas of seafloor geomorphic features and benthic habitats. Amsterdam: Elsevier
Ierodiaconou, D., Schimel, A. C. G., Kennedy, D., Monk, J., Gaylard, G., Young, M., et al. (2018). Combining pixel and object based image analysis of ultra-high resolution multibeam bathymetry and backscatter for habitat mapping in shallow marine waters. Mar. Geophys. Res. 39 (1–2), 271–288. doi:10.1007/s11001-017-9338-z
Ilich, A. R., Misiuk, B., Lecours, V., and Murawski, S. A. (2023). MultiscaleDTM: an open-source R package for multiscale geomorphometric analysis. Trans. GIS 27 (4), 1164–1204. doi:10.1111/tgis.13067
Jackson, D. R., and Richardson, M. D. (2007). “High-frequency seafloor acoustics,” in Monograph Series in Seafloor Acoustics (Springer), 616.
Lamarche, G., and Lurton, X. (2018). Recommendations for improved and coherent acquisition and processing of backscatter data from seafloor-mapping sonars. Mar. Geophys. Res. 39 (1–2), 5–22. doi:10.1007/s11001-017-9315-6
Lamarche, G., Lurton, X., Verdier, A.-L., and Augustin, J.-M. (2011). Quantitative characterisation of seafloor substrate and bedforms using advanced processing of multibeam backscatter—application to Cook Strait, New Zealand. Cont. Shelf Res. 31 (2), S93–S109. doi:10.1016/j.csr.2010.06.001
Leão, Z. M. A. N. (2002) “O complexo recifal mais extenso do Atlântico Sul,” in Sítios Geológicos E Paleontológicos do Brasil. Brasília: DNPM, 345–359.
Li, J., Schill, S. R., Knapp, D. E., and Asner, G. P. (2019). Object-based mapping of coral reef habitats using planet dove satellites. Remote Sens. 11 (12), 1445. doi:10.3390/rs11121445
Lurton, X., Lamarche, G., Brown, C., Lucieer, V., Rice, G., Schimel, A., et al. (2015) “Backscatter measurements by seafloor-mapping sonars,” in Guidelines and Recommendations. GeoHab. Version 1.0. doi:10.5281/zenodo.10089261
Lyons, M. B., Murray, N. J., Kennedy, E. V., Kovacs, E. M., Castro-Sanguino, C., Phinn, S. R., et al. (2024). New global area estimates for coral reefs from high-resolution mapping. Cell Rep. Sustain. 1 (2), 100015. doi:10.1016/j.crsus.2024.100015
Mayer, L., Jakobsson, M., Allen, G., Dorschel, B., Falconer, R., Ferrini, V., et al. (2018). The nippon foundation—GEBCO seabed 2030 Project: the quest to see the world’s oceans completely mapped by 2030. Geosciences 8 (2), 63. doi:10.3390/geosciences8020063
McGonigle, C., Grabowski, J. H., Brown, C. J., Weber, T. C., and Quinn, R. (2011). Detection of deep water benthic macroalgae using image-based classification techniques on multibeam backscatter at Cashes Ledge, Gulf of Maine, USA. Estuar. Coast. Shelf Sci. 91 (1), 87–101. doi:10.1016/j.ecss.2010.10.016
Menandro, P. S., Bastos, A. C., Boni, G., Ferreira, L. C., Vieira, F. V., Lavagnino, A. C., et al. (2020). Reef mapping using different seabed automatic classification tools. Geosciences 10 (2), 72. doi:10.3390/geosciences10020072
Menandro, P. S., Bastos, A. C., Misiuk, B., and Brown, C. J. (2022a). Applying a multi-method framework to analyze the multispectral acoustic response of the seafloor. Front. Remote Sens. 3, 860282. doi:10.3389/frsen.2022.860282
Menandro, P. S., Lavagnino, A. C., Vieira, F. V., Boni, G. C., Franco, T., and Bastos, A. C. (2022b). The role of benthic habitat mapping for science and managers: a multi-design approach in the Southeast Brazilian Shelf after a major man-induced disaster. Front. Mar. Sci. 9, 1004083. doi:10.3389/fmars.2022.1004083
Menandro, P. S., Misiuk, B., Brown, C. J., and Bastos, A. C. (2023). Multispectral multibeam backscatter response of heterogeneous rhodolith beds. Sci. Rep. 13 (1), 20220. doi:10.1038/s41598-023-46240-7
Misiuk, B., and Brown, C. J. (2024). Benthic habitat mapping: a review of three decades of mapping biological patterns on the seafloor. Estuar. Coast. Shelf Sci. 296, 108599. doi:10.1016/j.ecss.2023.108599
Moura, R. L., Secchin, N. A., Amado-Filho, G. M., Francini-Filho, R. B., Freitas, M. O., Minte-Vera, C. V., et al. (2013). Spatial patterns of benthic megahabitats and conservation planning in the Abrolhos Bank. Cont. Shelf Res. 70, 109–117. doi:10.1016/j.csr.2013.04.036
Pillay, T., Cawthra, H. C., Lombard, A. T., and Sink, K. (2021). Benthic habitat mapping from a machine learning perspective on the Cape St Francis inner shelf, Eastern Cape, South Africa. Mar. Geol. 440, 106595. doi:10.1016/j.margeo.2021.106595
Porskamp, P., Young, M., Rattray, A., Brown, C. J., Hasan, R. C., and Ierodiaconou, D. (2022). Integrating angular backscatter response analysis derivatives into a hierarchical classification for habitat mapping. Front. Remote Sens. 3, 903133. doi:10.3389/frsen.2022.903133
Recouvreur, A., Wheeler, A. J., Strachan, R., Meere, P. A., Unitt, R. P., and Lim, A. (2024). Large-scale bedrock outcrop mapping on the NE Atlantic Irish continental margin. Front. Mar. Sci. 10, 1258070. doi:10.3389/fmars.2023.1258070
Roberts, J. M., Brown, C. J., Long, D., and Bates, C. R. (2005). Acoustic mapping using a multibeam echosounder reveals cold-water coral reefs and surrounding habitats. Coral Reefs 24 (4), 654–669. doi:10.1007/s00338-005-0049-6
Schimel, A. C. G., Beaudoin, J., Parnum, I. M., Le Bas, T., Schmidt, V., Keith, G., et al. (2018). Multibeam sonar backscatter data processing. Mar. Geophys. Res. 39 (1–2), 121–137. doi:10.1007/s11001-018-9341-z
Schimel, A. C. G., Brown, C. J., and Ierodiaconou, D. (2020). Automated filtering of multibeam water-column data to detect relative abundance of giant kelp (Macrocystis pyrifera). Remote Sens. 12 (9), 1371. doi:10.3390/rs12091371
Schulze, I., Gogina, M., Schönke, M., Zettler, M. L., and Feldens, P. (2022). Seasonal change of multifrequency backscatter in three Baltic Sea habitats. Front. Remote Sens. 3, 956994. doi:10.3389/frsen.2022.956994
Smith, J. E., Brainard, R., Carter, A., Grillo, S., Edwards, C., Harris, J., et al. (2016). Re-evaluating the health of coral reef communities: baselines and evidence for human impacts across the central Pacific. Proc. R. Soc. B Biol. Sci. 283 (1822), 20151985. doi:10.1098/rspb.2015.1985
Stocks, J. R., Rodgers, M. P., Pera, J. B., and Gilligan, D. M. (2019). Monitoring aquatic plants: an evaluation of hydroacoustic, on-site digitising and airborne remote sensing techniques. Knowl. and Manag. Aquatic Ecosyst. 420, 27. doi:10.1051/kmae/2019016
Strong, J. A., Clements, A., Lillis, H., Galparsoro, I., Bildstein, T., and Pesch, R. (2019). A review of the influence of marine habitat classification schemes on mapping studies: inherent assumptions, influence on end products, and suggestions for future developments. ICES J. Mar. Sci. 76 (1), 10–22. doi:10.1093/icesjms/fsy161
Trzcinska, K., Tegowski, J., Pocwiardowski, P., Janowski, L., Zdroik, J., Kruss, A., et al. (2021). Measurement of seafloor acoustic backscatter angular dependence at 150 kHz using a multibeam echosounder. Remote Sens. 13 (23), 4771. doi:10.3390/rs13234771
Van Dijk, T. A. G. P., Roche, M., Lurton, X., Fezzani, R., Simmons, S. M., Gastauer, S., et al. (2024). Bottom and suspended sediment backscatter measurements in a flume—towards quantitative bed and water column properties. J. Mar. Sci. Eng. 12 (4), 609. doi:10.3390/jmse12040609
Vieira, F. V., Bastos, A. C., and Quaresma, V. S. (2023). Submerged reef and inter-reef morphology in the Western South Atlantic, Abrolhos Shelf (Brazil). Geomorphology 442, 108917. doi:10.1016/j.geomorph.2023.108917
Yizong, C. (1995). Mean shift, mode seeking, and clustering. IEEE Trans. Pattern Analysis Mach. Intell. 17 (8), 790–799. doi:10.1109/34.400568
Keywords: multifrequency backscatter, seafloor mapping, reef, multibeam echosounder, Benthic habitat mapping
Citation: Menandro PS, Vieira FV, Bastos AC and Brown CJ (2024) Exploring the multispectral acoustic response of reef habitats. Front. Remote Sens. 5:1490741. doi: 10.3389/frsen.2024.1490741
Received: 03 September 2024; Accepted: 07 November 2024;
Published: 19 November 2024.
Edited by:
DelWayne Roger Bohnenstiehl, North Carolina State University, United StatesReviewed by:
Peter Feldens, Leibniz Institute for Baltic Sea Research (LG), GermanyTim Le Bas, University of Southampton, United Kingdom
Copyright © 2024 Menandro, Vieira, Bastos and Brown. This is an open-access article distributed under the terms of the Creative Commons Attribution License (CC BY). The use, distribution or reproduction in other forums is permitted, provided the original author(s) and the copyright owner(s) are credited and that the original publication in this journal is cited, in accordance with accepted academic practice. No use, distribution or reproduction is permitted which does not comply with these terms.
*Correspondence: Pedro S. Menandro, cGVkcm8ubWVuYW5kcm9AZGFsLmNh