- 1Doctoral Program in Water Resources, Universidad Nacional Agraria La Molina, Lima, Peru
- 2Servicio Meteorológico e Hidrológico del Perú SENAMHI, Lima, Peru
- 3Géosciences Environnement Toulouse, Université de Toulouse, Toulouse, France
Climate change will cause alterations in the hydrological cycle, a topic of great relevance to the scientific community due to its impacts on water resources. Investigating changes in hydrological characteristics at the watershed level in the context of climate change is fundamental for developing mitigation and adaptation strategies against extreme hydrological events. This study aimed to analyze the impacts of climate change on flow and sediment production in the Puyango-Tumbes watershed. Projected climate data from CMIP6 were used, corrected through a bias adjustment process to minimize discrepancies between model data and historical observations, ensuring a more accurate representation of climate behavior. The analysis combined two representative climate change scenarios (SSP2-4.5 and SSP5-8.5) with two land use and land cover (LULC) scenarios: (a) an optimistic scenario with reduced anthropogenic effects (LULC_1985) and (b) a pessimistic scenario reflecting future impacts (LULC_2015). The SWAT model estimated future flow and sediment production for two periods (2035-2065 and 2070-2100), following model calibration and validation against the reference period 1981-2015 at three hydrometric stations: Pindo, Puyango, and El Tigre, located in Ecuador and Peru. The simulations revealed a significant increase in sediment generation under the pessimistic scenario SSP5-8.5, followed by SSP2-4.5, while lower sediment yields were observed in the optimistic scenarios. Even in the best-case scenario (optimistic SSP2-4.5), sediment yields remained substantially higher than the reference conditions. Additionally, higher flows were anticipated in some scenarios, with the El Tigre station in the lower watershed being the most affected area. These findings underscore the high probability of more frequent flooding events due to increased sediment yields and flow variability. The results highlight the urgent need for implementing adaptation measures, such as improved land use management and hydrological infrastructure, to enhance social resilience and mitigate the impacts of climate change in the watershed.
1 Introduction
Variability in climate and long-term climate change, along with human activities, are the primary factors influencing flow and erosion in watersheds (Dai et al., 2020). Global climate change is widely recognized (Oliveira et al., 2018), and numerous studies address the increasing concentration of greenhouse gases and the associated changes in climatic factors, which have altered precipitation patterns, increased surface evapotranspiration, and modified river flows, thus accelerating the hydrological cycle (Liu et al., 2020; Marin et al., 2020).
Global reports highlight that the increase in the frequency of extreme hydrological events, such as floods and droughts, has led to unprecedented environmental issues, including water quality degradation and biodiversity loss (Zhang et al., 2020; Santos et al., 2021), with direct impacts on the global economy and the lives of large segments of the population (Iqbal et al., 2022). These effects include more frequent floods and droughts, contamination of water resources, soil erosion, sediment accumulation, and a decline in the soil’s ability to support plant growth and maintain fertility over time (Chanapathi and Thatikonda, 2020). These changes have a profound impact on land use and land cover (LULC), as natural systems sustaining our planet are being pushed to their limits, with agriculture and urbanization being the primary drivers. This has led to habitat destruction, as well as increased pollution and other environmental challenges (Chanapathi and Thatikonda, 2020).
According to the Sixth Assessment Report of the Intergovernmental Panel on Climate Change (IPCC), climate change is accelerating, and its impacts may become more extreme in the future. Ali et al. (2023) concluded that the sustainability of water resources is more vulnerable to socioeconomic changes than to climate trends. To mitigate the effects of climate change and adapt to its impacts, the Coupled Model Intercomparison Project (CMIP) offers a valuable tool for predicting future climate conditions under changing scenarios (Farjad et al., 2019). CMIP6, the latest version of CMIP, featured in the IPCC’s sixth assessment report, presents climate projections from multiple models based on alternative scenarios directly relevant to societal concerns regarding mitigation, adaptation, or impacts of climate change. These climate projections are driven by a new set of emission and land-use scenarios produced with Integrated Assessment Models (IAMs), based on new future pathways of social development, the Shared Socioeconomic Pathways (SSPs), and Representative Concentration Pathways (RCPs) (Calvin et al., 2023).
Recent studies on climate change have explored various aspects of precipitation patterns and climate extremes. Moradian et al. (2023) analyzed results from 12 CMIP6 models in Northern Europe, evaluating the performance of a multi-model ensemble approach. Their findings highlighted the superior effectiveness of the multi-model ensemble over individual models in projecting precipitation for the study region. Similarly, Rhymee et al. (2022) assessed CMIP6 datasets for daily and monthly precipitation projections using two multi-model ensemble methods. Both approaches revealed a significant decrease in future precipitation. Additionally, Zhu et al. (2021) focused on projections of climate extremes in China using CMIP6, reporting that CMIP6 models outperformed CMIP5, particularly in simulating extreme precipitation events.
Hydrological modeling is one of the most common and comprehensive methods used to determine how climate change can affect water resources (Mendoza et al., 2015). According to the literature, many researchers have adopted the SWAT (Soil Water and Assessment Tool) model to assess the impact of climate change on river discharge (Jajarmizadeh et al., 2014). For instance, Narsimlu et al. (2023) used the SWAT model and regional climate meteorological data for impact studies to investigate the influence of future climate changes in the Upper Sind River basin, India. They estimated that discharge would increase by 16% and 93% in the medium and long term, respectively. Mohseni et al. (2023) based their study on assessing the impact of climate change and land use on discharge in the present and future according to CMIP6 climate scenarios using SWAT. Additionally, the research by Paiva et al. (2023), which focused on evaluating the hydrological response to land cover change in a Peruvian Amazon basin affected by deforestation using the SWAT model, determined that replacing 12% of evergreen broadleaf forest area with bare land resulted in a significant increase in surface runoff by 38% monthly, a 1% annual reduction in evapotranspiration, and a 12% average monthly increase in streamflow.
The Puyango-Tumbes basin, a transboundary watershed shared between Ecuador and Peru, faces significant challenges, particularly water scarcity. This issue arises from the intensive extraction of water resources for agricultural purposes, a critical economic sector at the binational level. The situation is exacerbated by the prevalent use of inefficient irrigation systems, notably flood irrigation practices. In both countries, the agricultural sector accounts for over 90% of total water consumption, leading to declining water volumes in specific regions. For instance, recent data indicate a 26% reduction in water volumes at the El Tigre monitoring station within the Tumbes basin. Another pressing concern is the rate of deforestation, especially in areas adjacent to river mouths, streams, and aquifer recharge zones. Stakeholders have observed a notable decrease in water volumes and flows, a trend that could worsen if the impacts of climate change continue to escalate (UNDP, 2015).
In this context, it is essential to understand the impact of climate change and land use/land cover (LULC) under two future climate scenarios (optimistic and pessimistic). The main objective of this research is to establish a solid foundation for long-term water resource projection and policy by understanding how streamflow responds to climate change and LULC in the Puyango-Tumbes basin. The specific objectives are: (a) to quantify the potential impact of climate change and LULC on streamflow and sediment generation under different scenarios for future time projections (2035-2065) and distant future (2070-2100); (b) to investigate the effect of climate change on precipitation and temperature under different scenarios (SSP2-4.5 and SSP5-8.5). The findings of this study can be applied to long-term planning and policy formulation, particularly in water resource management.
2 Materials and methods
2.1 Study area
The Puyango-Tumbes basin has an area of 4,800 km2, of which 60% belongs to the provinces of El Oro and Loja, located in the southeast of Ecuador, and 40% is in the Department of Tumbes, located in the north of Peru. The Puyango-Tumbes River originates at an altitude of 3,500 m above sea level in the Portovelo area, where it is called the Pindo River, then becomes the Puyango River, and finally the Tumbes River in Peruvian territory (Figure 1). Annually, the average annual precipitation is 1,200 mm with marked variations from 100 mm to 2,700 mm, and the temperature on the plains is 24.5°C and in the mountainous area is 22°C (UNDP, 2015).
Approximately 70% of the area has soil suitability for protection and/or restoration; almost two-thirds of the basin consists of fragile lands, whose natural conditions are not suitable for agricultural production, and their utilization implies severe risks of soil erosion and degradation (MAP and GIS, 2019).
The basin is of very high socioeconomic interest for both countries since it contains a large part of the population and their productive activities. However, the economy of these areas largely depends on water availability and, therefore, is vulnerable to poor management, overexploitation, and contamination of the resource, as well as the effects of variability and climate change (Jones et al., 2017).
2.2 Data description
This study used a daily time scale and sub-basin scheme derived from the digital elevation model (DEM). The sub-basin configuration through the TauDEM tool preserved natural channels and flow paths. Stream reaches were defined by choosing the minimum surface threshold automatically set by SWAT as a criterion to create them, thus obtaining a rigorous representation of the channels (Abbaspour et al., 2015a) (Figure 2A). Hydrological Response Units (HRUs) were obtained considering soil type, land use, and landscape slope (Zhang, 2014). Modeling with SWAT also considered daily climatic information, specifically precipitation, maximum and minimum temperatures for determining the main processes related to the hydrological cycle (Abbaspour et al., 2015a), and thus, with the calibrated parameters, served for application in future projections (Figure 4).
Climatic information, specifically precipitation, was obtained from the gridded product RAIN4PE (Rain for Peru and Ecuador) (https://dataservices.gfz-potsdam.de), and maximum and minimum temperatures were obtained from the gridded product PISCO v2.0 for the period 1981–2015 (http://iridl.ldeo.columbia.edu).
Historical hydrometric information was obtained from the National Institute of Meteorology and Hydrology (INAMHI) of Ecuador and the National Service of Meteorology of Hydrology of Peru (SENAMHI) from three hydrometric stations “Pindo,” “Puyango,” and “El Tigre” for the period 1992–2015 (Figure 2C).
The SWAT model divided the slope into three categories, based on information obtained from the DEM (Figure 2A). The global soil map was obtained from the Harmonized World Soil Database v1.2 (HWSD) (Figure 2B).
2.3 Land use/land cover (LULC) scenarios
In analyzing the LULC of the Puyango-Tumbes watershed over a 35-year period (1981–2015), two LULC scenarios from the MODIS product (https://ladsweb.modaps.eosdis.nasa.gov) were selected to assess the influence of future climate on streamflow and sediment yield: (a) an optimistic scenario characterized by reduced anthropogenic impacts (LULC_1985), and (b) a pessimistic scenario, representing the most realistic impacts (LULC_2015), considering the intensification of monoculture with pasture.
The configuration of the LULC scenarios in this research was based on an analysis of historical land-use trends, acknowledging that land-cover changes in the region are driven by local factors, including agricultural practices, land-use policies, and demographic pressures. This approach is justified by the fact that the SWAT model requires inputs that accurately reflect local conditions in order to perform precise simulations of hydrological behavior.
2.4 Accuracy of simulation and SWAT performance evaluation
The model was calibrated and validated on a daily scale using daily flows along the Puyango-Tumbes River at three hydrometric stations: “Pindo,” “Puyango,” and “El Tigre” (Figure 2A). 28 years (1988–2015) were utilized for calibration and validation, including 4 years for model warm-up.
Parameter calibration was performed using the SWAT_CUP uncertainty procedures software (Abbaspour, 2015b). Based on previous studies (Cibin et al., 2010; Funk et al., 2015), 16 sensitive hydrological parameters were selected for analysis (Table 1).
A global sensitivity analysis was conducted to obtain both relative and absolute sensitivity. The Sequential Uncertainty Fitting algorithm, version No. 2 (Sufi-2) (Abbaspour et al., 2015a), was implemented to identify the most sensitive parameters according to the model’s response. In Sufi-2, parameter uncertainty, expressed as ranges (uniform distributions), accounts for all sources of uncertainty, such as uncertainty in input variables (e.g., rainfall), the conceptual model, parameters, and measurement data. The propagation of uncertainties in the parameters leads to uncertainties in the model’s output variable, expressed as 95% probability distributions, referred to as the 95% Prediction Uncertainty or 95 PPU.
These 95 PPU represent the outcomes of the models in a stochastic calibration approach. It is important to realize that we do not have a single signal representing the model results, but rather a set of good solutions expressed by the 95 PPU, generated by certain parameter ranges (Abbaspour et al., 2015a).
The calibrated parameters at the three hydrometric stations “Pindo,” “Puyango,” and “El Tigre” were used in the future flow projections. For the assessment of sediment load, the bottom solids discharge curve in liquid discharge fusion (Equation 1), developed by (Goyburo, 2017) for the El Tigre station, was employed. This involved characterizing the sediment evolution based on liquid flows during extreme events using the database of the Peruvian Geophysical Institute (IGP) and the total sediment transport during the sampling campaign (Jan-May/2016). It was determined that the methodology proposed by Rennie, Millar, and Church (2002) best approximates the observed data of bottom solid discharge, obtaining a correlation R = 0.43 between bottom solid discharge and observed liquid discharge.
where
2.5 Future climate projections and bias correction
To generate long-term simulations of future climate, climate change scenarios were defined based on CMIP6 data, as they provide climate projections from multiple models based on alternative scenarios that are directly relevant to societal concerns regarding climate change mitigation, adaptation, or impacts (Riahi et al., 2016). The “Shared Socioeconomic Pathways” scenarios were used in this study:
SSP2-4.5 (this scenario represents the middle part of the range of future forcing pathways and updates the RCP4.5 pathway. SSP2 was chosen because its land use and aerosol pathways are not extreme compared to other SSPs) and SSP5-8.5 (this scenario represents the upper extreme of the range of future pathways, updating the RCP8.5 pathway). SSP5 was chosen for this forcing pathway because it is the only SSP scenario with emissions high enough to produce a radiative forcing of 8.5 W/m2 in 2,100). The methodology applied in this study is based on the integration of 24 CMIP6 models, which allowed for the generation of more robust climate projections and minimized long-term uncertainties, especially in simulating critical variables such as precipitation and temperature (maximum and minimum) were downloaded for the near future (2035–2065) and far future (2070–2100) for the two scenarios. This was crucial for the hydrological analysis in the SWAT model, providing a solid foundation to assess the impacts of climate change on the Puyango–Tumbes River watershed under different radiative forcing scenarios (SSP2-4.5 and SSP5-8.5). By combining the mean of multiple models with delta change bias correction techniques applied to the 23 sub-watersheds, the reliability of the obtained results is ensured.
To correct CMIP6 data, bias correction was performed using the delta change method, which is an adjustment factor derived from simulated data and applied to the observed data series from 1981 to 2015. This procedure was applied to all records of the 23 obtained sub-catchments of the setup hydrological model, this method involves calculating an adjustment factor by dividing the averages of all months of the observed data by the averages of all months of the modeled data (Guo et al., 2022; Golian, El-Idrysy, and Stambuk, 2023). It is important to note that this method was also applied in the same manner for the 5th percentile (value below which 5% of the data lie) and 95th percentile (value below which 95% of the data lie) of the CMIP6 data.
Subsequently, a new series is obtained by multiplying the previously calculated adjustment factor with the modeled data series (Equation 2).
Where
The modeling of the Puyango-Tumbes basin was performed with the combination of land use and land cover scenarios (LULC_1981 and LULC_2015) and future projections (SSP2-4.5 and SSP5-8.5) (Figure 3).
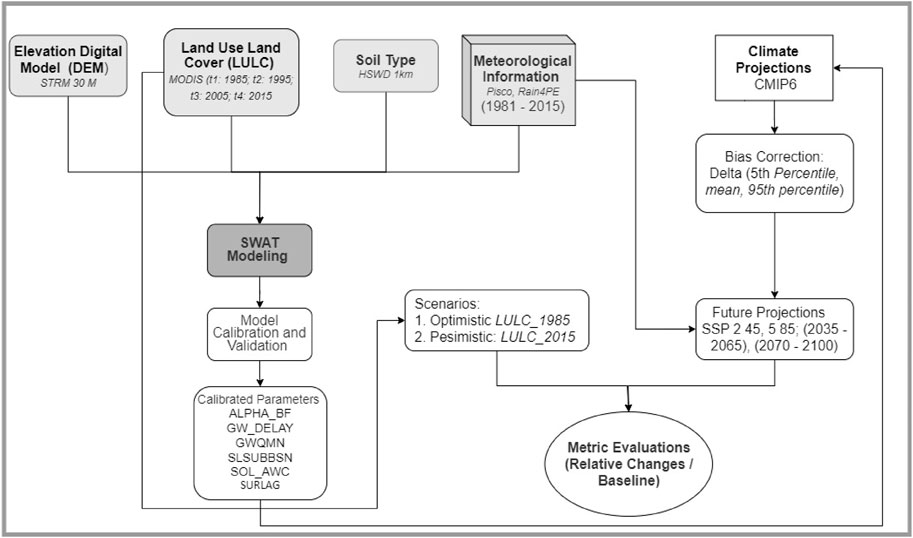
Figure 3. General conceptual methodology used for projecting future simulation in the Puyango-Tumbes Basin.
3 Results
3.1 SWAT model sensitivy analysis
According to the sensitivity analysis, six parameters significantly affect the modeled surface runoff in the Puyango-Tumbes basin. These parameters include the soil available water capacity (SOL_AWC), the average slope length (SLSUBBSN), and other parameters related to surface runoff (SURLAG) and groundwater (ALPHA_BF, GW_DELAYMM, GWQMN). These parameters were calibrated to fit the actual water balance based on literature information (Le et al., 2012). Calibration was executed using the Sequential Uncertainty Fitting version 2 (SUFI-2) algorithm (Arnold et al., 2012), a widely recognized method for automatic calibration and sensitivity analysis in SWAT models. Sensitive parameters affecting surface runoff and groundwater flow, such as soil available water capacity (SOL_AWC), average slope length (SLSUBBSN), and groundwater parameters (ALPHA_BF, GWQMN, GW_DELAYMM), were identified through global sensitivity analysis with SWAT-CUP. Defined parameter ranges enabled simulations that varied one parameter at a time, quantifying their sensitivity on model outputs. Subsequently, the SUFI-2 algorithm was applied to systematically adjust these parameters, aligning modeled outputs with observed water balance data, which ensured an accurate representation of hydrological processes in the Puyango-Tumbes basin and enhanced the reliability of water management decisions.
If compared to the default values, the ALPHA_BF parameter was increased in the model to facilitate the flow of water from the aquifer to the river, thus increasing the flow rate.
When compared to the default values, the ALPHA_BF parameter was increased in the model to facilitate the flow of water from the aquifer to the river, thereby increasing the base flow. Additionally, the GWQMN and GW_DELAY parameters were increased, leading to higher surface flows and consequently decreasing groundwater flow. The remaining parameters related to hydrological processes were calibrated to adjust both base and peak flows (Table 2).
3.2 Evaluation of downscaling for future climate scenarios
This section presents a comparison of the SSP2-4.5 and SSP5-8.5 scenarios with observed precipitation data, regarding the spatial distribution of precipitation throughout the basin (Figure 4). Table 4 shows the comparison between observed and downscaled monthly average precipitation, with coefficient of determination (R2) values of 0.95, indicating a good correspondence between observed and downscaled data after bias correction by delta change. The analysis revealed that by the end of the 2065 decade, the annual mean precipitation is projected to increase by up to 6% for SSP2-4.5, with a similar trend for SSP5-8.5 showing an increase of 14% by 2065, indicating a rising trend. The projected temperature increases ranges from 2.8°C to 4.3°C and 3.7°C–7.4°C in the SSP2-4.5 and SSP5-8.5 scenarios, respectively.
3.3 Streamflow and sediment yield
The streamflow records in response to LULC change allowed the evaluation of sediment yield for the Puyango Tumbes river basin. The results indicate that sediment yield in the basin is directly related to precipitation, suggesting that this region has a rapid flow response. The highest sediment yield values occurred in 1985, as there was less anthropogenic influence. The lowest estimated sediment yield occurred in 2015, indicating a lower streamflow generation. Furthermore, the results show a variation in the standard deviation for streamflow and sediment yield data between LULC_1985 and LULC_2015 for Pindo equal to 4 m3 s−1− and 13%; Puyango equal to 33 m3 s−1− and 18%; and El Tigre equal to 21 m3 s−1− and 9%, respectively (Table 3).
3.4 Analysis of land use and land cover change
As illustrated in Figures 5A, B, a comprehensive analysis of LULC transitions was conducted, revealing significant changes among various land cover classes during the study period. It is noteworthy that the forest, grassland, and savanna classes exhibited a high probability of persistence, exceeding 80%, indicating their stability within the basin. However, the grassland class emerged as the most dynamic, demonstrating a significant increase of 18.3%. This pronounced change suggests that grasslands are undergoing accelerated conversion, likely due to anthropogenic pressures and environmental changes.
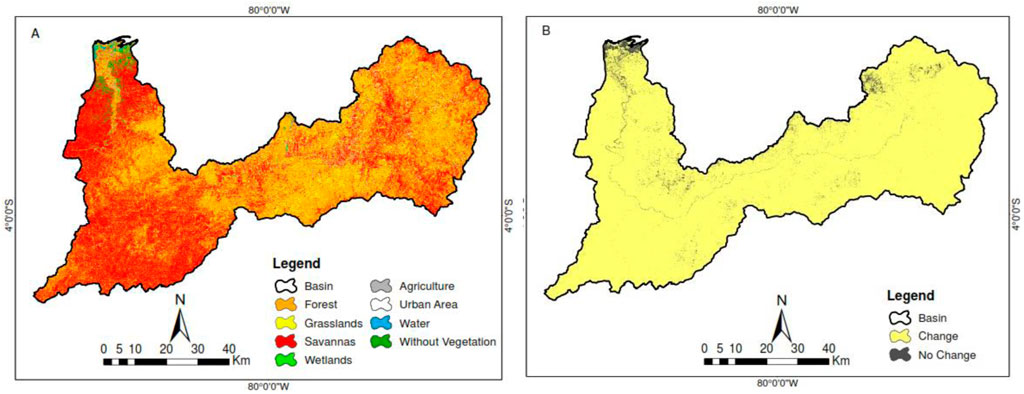
Figure 5. LULC map of the Puyango Tumbes River Basin in 1985 (A), and spatial validation of simulated LULC in 2015 (B).
These dynamics are crucial as they not only influence the current land cover but also have profound implications for future land management and ecological health in the region. The observed changes in grassland cover may contribute to alterations in hydrological cycles, habitat availability, and biodiversity, which in turn affect ecosystem services. Furthermore, the characteristics of these changes in land use and land cover are critical for future projections, as presented in Figures 7–9. Understanding these trends is essential for developing adaptive management strategies aimed at mitigating adverse impacts on the basin’s resources. This expanded analysis underscores the need for continuous monitoring and evaluation of land use dynamics to inform policies and enhance the sustainable management of resources in the Puyango-Tumbes River Basin.
3.5 The combined impacts of climate change and LULC on streamflow and sediment yield
Figure 6B shows the spatial distribution of sediment yield in the sub-basins. The results indicate that sediment production was higher in the sub-basins located in the upper part due to the predominance of areas with pasture cultivation and the influence of higher precipitation, resulting in increased streamflow (Figure 6A), contributing to accelerated erosion in these sub-basins. It was affirmed that the increase in river flow in the future depends mainly on increases in precipitation.
Table 4 shows the results of estimated streamflow and sediment yield for the Puyango-Tumbes River basin using future climate data, for the 5th percentile, mean, and 95th percentile. In this context, at the Pindo station, streamflow based on SSP2-4.5 and optimistic LULC increased relative to the baseline period (1981-2015) by 20% and 43%, respectively, in the periods 2035-2065 and 2070-2,100. According to SSP5-8.5, the expected streamflow exceeded the observed in the baseline period by 66% in the period 2035-2065, and by 86% in 2070–2,100 (Figure 7). The figure shows how the streamflow projections for future periods vary compared to the historical period, highlighting potential changes in flow patterns over time under different climate change scenarios.
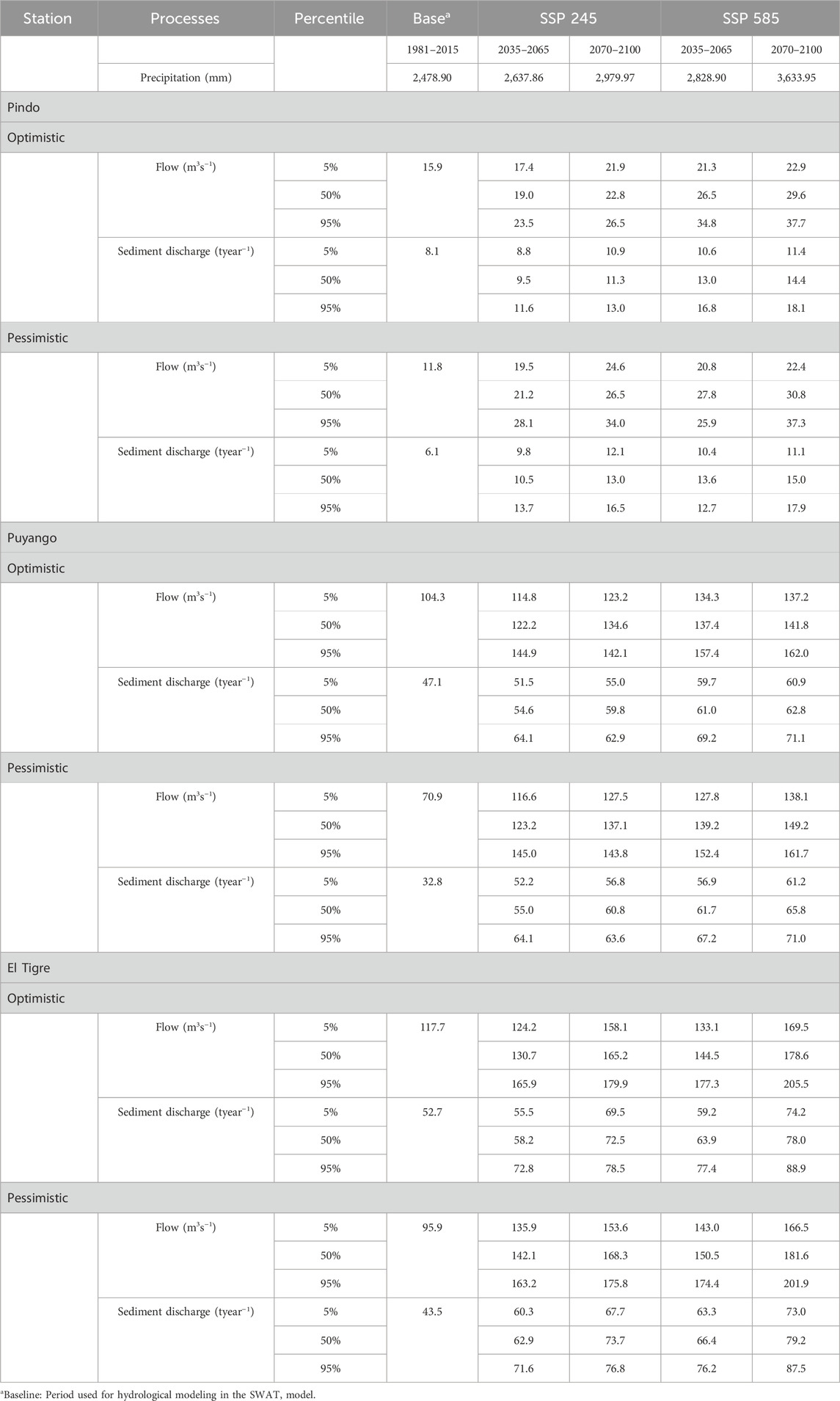
Table 4. Annual averages of precipitation. Streamflow. And sediment under different climate change and LULC scenarios.
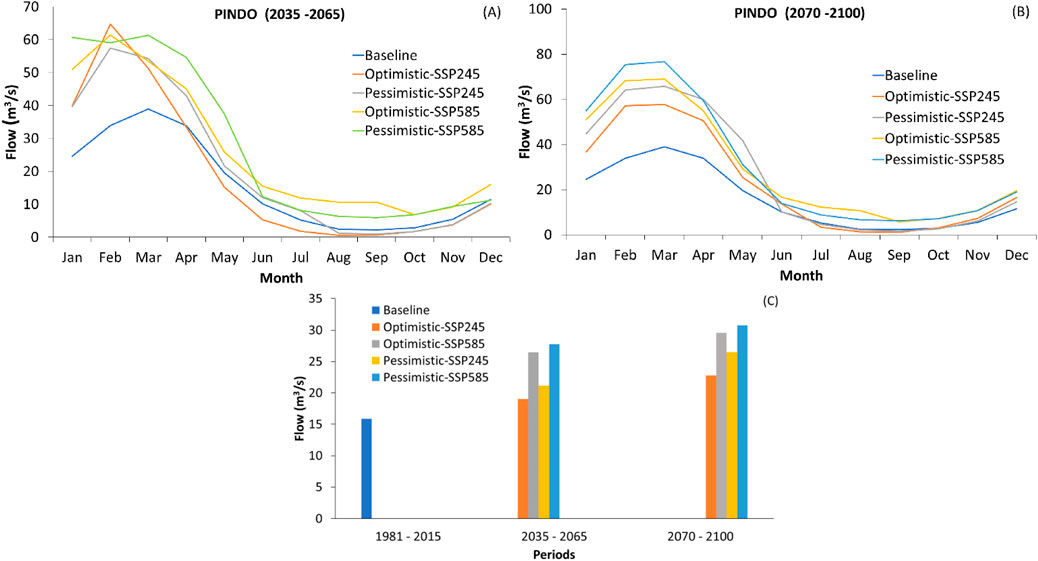
Figure 7. Streamflow behavior at the Pindo station for the near and distant future based on SSP 2035–2065 period (A); 2070–2010 period (B) and historical period, near future and distant period (C).
At the Puyango station, a similar behavior to the Pindo station was observed. Streamflow based on SSP2-4.5 and optimistic LULC increased relative to the baseline period (1981-2015) by 17% and 29%, respectively, in the periods 2035-2065 and 2070-2,100. According to SSP5-8.5, the expected streamflow exceeded the observed in the baseline period by 32% in the period 2035-2065, and by 36% in 2070–2,100 (Table 4; Figure 8).
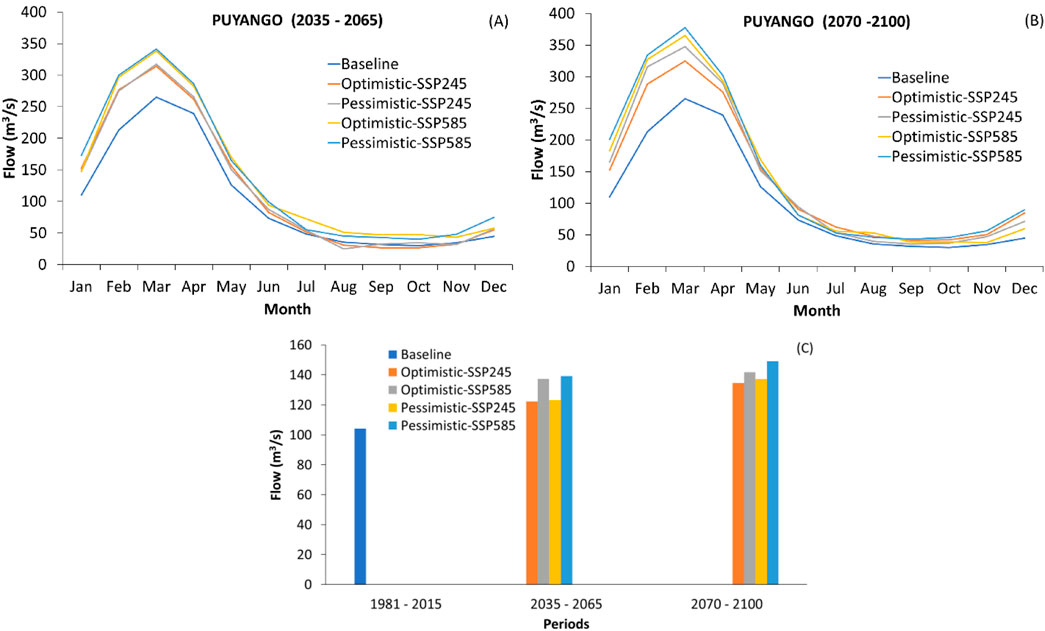
Figure 8. Streamflow behavior at the Puyango station for the near and distant future based on SSP 2035–2065 Period (A); 2070–2010 period (B); historical period, near future and distant period (C).
At the El Tigre station, streamflow based on SSP2-4.5 and optimistic LULC increased relative to the baseline period (1981-2015) by 11% and 40%, respectively, in the periods 2035-2065 and 2070-2100. According to SSP5-8.5, the expected streamflow exceeded the observed in the baseline period by 23% in the period 2035-2065, and by 52% in 2070–2100. In all three stations, this increase is due to the increase in estimated precipitation relative to SSP2-4.5 (Table 3; Figure 9). This figure provides a comparison of projected streamflow for the future and its difference from historical values, showing potential trends in flow as time progresses under different climatic scenarios.
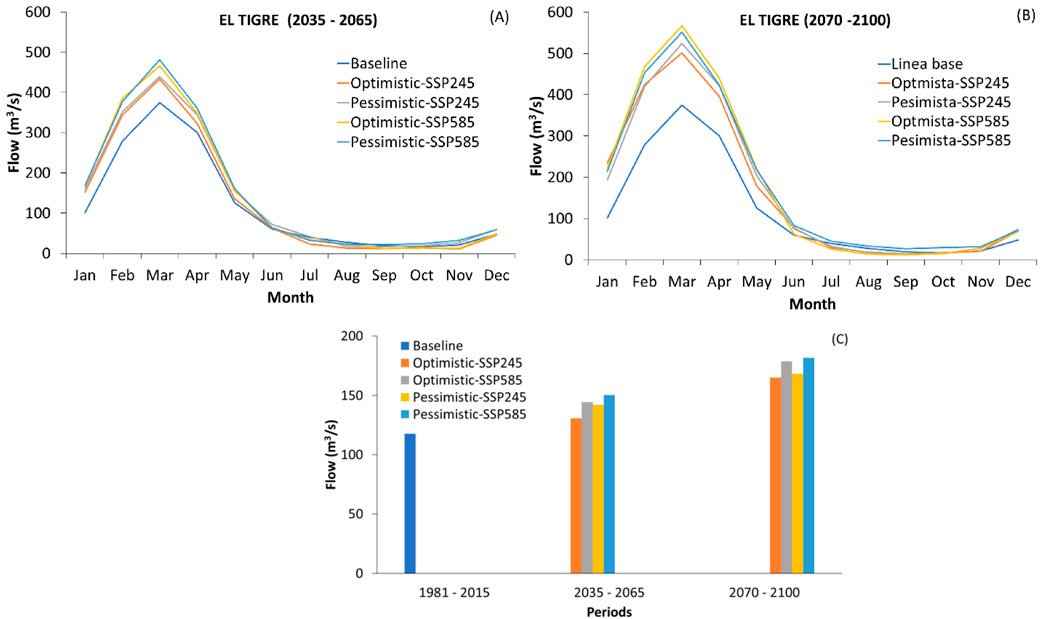
Figure 9. Streamflow behavior at the El Tigre station for the near and distant future based on SSP 2035–2065 period (A); 2070–2010 period (B); historical period, near future and distant period (C).
In this study, the SWAT hydrological model, driven by climate simulations, estimated an overall increase in the flow of the Puyango-Tumbes River for both optimistic and pessimistic scenarios.
The results obtained showed that the simulated mean annual streamflow will mainly increase between 2035 and 2065 in SSP5-8.5, while in SSP2-4.5, it presented the lowest values. Between 2035-2065 and 2070-2100, there is less variation between the SSPs and scenarios, but also with an increase in values compared to the baseline. The results of the simulated annual streamflow showed an increase in the optimistic scenario in both climate scenarios (SSP2-4.5 and SSP5-8.5). This result is sensitive to the outcome of future climate projections (Santos et al., 2021; Silva et al., 2020) affirmed that the increase in river flow in the future depends mainly on increases in precipitation.
As evidenced in Table 4 and Figure 10, sediment production in the watershed showed the same trend as streamflow in both climate change scenarios. Projected sediment yield values for SSP2-4.5 exceeded those of the baseline by 18%–40% for the Pindo station, 16%–27% for the Puyango station, and 10%–37% for the El Tigre station. Regarding SSP5-8.5, the values were even higher, ranging from 61% to 79% in Pindo, 30%–33% in Puyango, and 21%–48% in El Tigre, for the different analysis periods. The pessimistic LULC scenario showed increases in sediment yield for all years from 72% to 113% for Pindo, 68%–86% for Puyango, and 45%–69% for El Tigre compared to SSP2-4.5. As for SSP5-8.5, the increase was greater, ranging from 122% to 145% for Pindo, 88%–101% for Puyango, and 53%–82% for El Tigre compared to the optimistic scenario, demonstrating that soil erosion in the region is more sensitive to climatic variability than streamflow, and that LULC changes can have serious impacts on the watershed. These results serve as an urgent call for the adoption of mitigating measures to prevent associated environmental problems and improve land and water resource management.
In the pessimistic scenario, at the Pindo station for SSP2-4.5, the flow rate exceeds the optimistic scenario by 11% in the period 2035-2065 and by 16% in the period 2070-2100. The flow rate results for the pessimistic scenario SSP5-8.5 show a slight increase of four%–5% compared to the optimistic scenario. At the Puyango station for SSP2-4.5, there is a slight elevation of the pessimistic scenario compared to the optimistic one by 1% in the period 2035-2065 and by 2% in the period 2,070–2,100. The flow rate in the pessimistic scenario SSP5-8.5 shows an increase of 1–5% compared to the optimistic one. Regarding the El Tigre station in SSP3-4.5, it surpasses the pessimistic scenario by 9% in the period 2,035–2,065 and by 2% in the period 2,070-2,100. The flow rate results for the pessimistic scenario SSP5-8.5 show an increase of 4% in the period 2,035–2,065 and 2% in the period 2,070–2,100.
The flow rate in the pessimistic scenario SSP5-8.5 shows an increase of between 1 and 5% compared to the optimistic one. Regarding the El Tigre station in SSP3-4.5, it exceeds the pessimistic scenario by 9% in the period 2,035-2,065 and by 2% in the period 2,070-2,100. The flow rate results for the pessimistic scenario SSP5-8.5 show an increase of 4% in the period 2,035-2,065 and 2% in the period 2,070-2,100.
The highest values of flow rate and sediment yield in the SSP2-4.5 and SSP5-8.5 scenarios occurred in 2,070–2,100, which is related to the precipitation pattern, as this period is the rainiest in these scenarios. The results suggest that in the near future (2,035-2,065), there may be negative impacts on the dynamics of the Puyango-Tumbes River basin, including floods, river sedimentation, affecting conservation and preservation efforts in the area.
Based on the SWAT model projections, the results show an increase in sediment yield for the analyzed LULC scenarios and climate change. These values suggest that responsible decision-makers should implement measures for flood management and avoid land use/land cover change transitions.
4 Discussion
This study has analyzed the impact of climate change and LULC on the discharge and sediment yield in the Puyango-Tumbes River basin, using a combination of two models (climate and hydrological), which allowed for a more integrated assessment of sediment dynamics. The model’s behavior for future projections was based on the basin’s discharge behavior under different LULC scenarios, and the selection of the most sensitive parameters characterizing the basin. Similarly, the study conducted by Goyburo (2017) focuses on the evolution of suspended and bed sediment discharge in the Tumbes River in relation to liquid discharges during major floods in the El Niño phenomenon occurring from January to May 2016.
The research drew guidelines from studies conducted in different latitudes. Additionally, it utilized tools and validation of LULC characterization at a global level. For instance (Santos et al., 2021), employed the SWAT hydrological model driven by climate simulations, estimated flow, and annual sediment yield in a strategic watershed in northeastern Brazil using GCM data for two different greenhouse gas emission scenarios. On the other hand (Kiprotich et al., 2021), utilized SWAT + to assess the feasibility of using high-resolution gridded data as an alternative to station observations to investigate the response of surface runoff to continuous land use change and future climate change. Silva et al. (2012) analyzed the impact of potential climate and land use changes in the semi-arid region of Brazil, while (Krause et al., 2019) evaluated combinations of Shared Socioeconomic Pathways (SSPs) and Representative Concentration Pathways (RCPs) as input for three global dynamic vegetation models to assess impacts and associated uncertainty on various ecosystem functions: terrestrial carbon storage and fluxes, evapotranspiration, surface albedo, and runoff. These studies provided useful contextual understanding and methodological advances, allowing for the establishment of an integrated assessment and validation methodology of the effects of LULC change on flow and sediment yield within the context of alternative climate change scenarios. As it constitutes a binational basin, the strategy of cooperation guides research efforts toward generating a water management strategy.
The hydrological model provided insights into the relative importance of land use/land cover (LULC) compared to the effect of climate change on streamflow. The combined impact of climate variability and optimistic and pessimistic LULC scenarios was evaluated using multiple projections with the SSP2-4.5 and 5–8.5 scenarios.
In the future, the results during the studied period using projected datasets indicate that this watershed clearly exhibits high variability during the study period, and also that precipitation does not show a uniform trend in past observations or future projections. Additionally, both pessimistic and optimistic climate scenarios yielded streamflows higher than those of the baseline.
Furthermore, as observed in the projected data, bias correction concerning the 5th percentile, mean, and 95th percentile tends to characterize precipitation behavior relative to the baseline, which likely accounts for the increase in modeled flow and sediment for these periods compared to the baseline. Similar behavior occurred in South America in the Tapacuará River basin, where streamflow and sediment production results are influenced by the scenarios used and the selected bias correction in the model (Santos et al., 2021).
Concerning historical conditions, higher streamflows and sediment yields can be anticipated in this watershed; results that serve to alert decision-makers about the value of using hydrological models like SWAT to highlight potential changes in flow behavior and sediment yield, thereby providing technical information and potentially improving binational management (MAP and GIS, 2019).
Furthermore, limitations arise from the lack of studies on modeling the impacts of future LULC and climate change on runoff and sediment yield for the Puyango-Tumbes River basin, which could be used for decision-making by stakeholders and establish the relationship between water availability and consumption for the economic activities of the basin population. Future studies are needed to use other SSPs to improve precipitation simulation for Puyango Tumbes. Despite these uncertainties, the results of the SWAT model have contributed to developing a better understanding of the future behavior of runoff and sediment in the Puyango-Tumbes River basin.
5 Conclusion
In conclusion, this study comprehensively examined the impacts of climate change on streamflow dynamics and sediment production within the Puyango-Tumbes River Basin. The calibration and validation of the SWAT model were performed utilizing the Sequential Uncertainty Fitting version 2 (SUFI-2) algorithm, which incorporated downscaled and bias-corrected climate projections under various projected land use and land cover (LULC) scenarios. The hydrological model demonstrated robust performance in simulating erosion processes, achieving satisfactory to excellent thresholds in model evaluation metrics. Notably, streamflow and sediment production were markedly intensified in sub-basins characterized by predominant pasture grass coverage, particularly in the upper and middle reaches of the basin. Additionally, significant contributions to sediment load were attributed to illegal mining activities, underscoring the complex interplay between land use changes and hydrological responses. These findings provide critical insights for developing targeted management strategies aimed at mitigating the adverse effects of climate change on water resources and sediment dynamics in the region.
It is anticipated that the mean streamflow and, in particular, sediment yield within the basin will experience a significant increase under the SSP5-8.5 scenario, largely attributable to expected rises in mean precipitation levels. Simulation results indicate that erosion rates are projected to be highest under the pessimistic SSP5-8.5 scenario, followed closely by the pessimistic SSP2-4.5 scenario. Conversely, lower sediment yields are anticipated for both the optimistic SSP5-8.5 and SSP2-4.5 scenarios, though the latter remains substantially above baseline levels. These findings suggest that, despite inherent uncertainties in climate change impact simulations, the basin is likely to face severe environmental challenges and water availability issues arising from extreme climatic conditions.
The peak values of streamflow (16%) and sediment yield (101%) under the SSP2-4.5 and SSP5-8.5 scenarios are projected to occur between 2070 and 2,100, correlating with anticipated precipitation patterns during the rainiest period, estimated at 3,634 mm. Furthermore, in the near term (2035-2065), the basin dynamics may experience detrimental impacts, including increased flooding and enhanced river sedimentation, which could compromise conservation and preservation efforts in the area.
According to our study, the Puyango-Tumbes River basin will experience increased streamflow for both LULC and climate change scenarios. While this may lead to increased water availability at the three hydrometric stations, it also correlates with the associated increase in sediment yield, generating susceptibility to floods during extreme events, especially at the El Tigre station located in the lower part of the basin.
In recent decades, significant alterations in land use and land cover (LULC), primarily due to the proliferation of pasture monocultures, have already modified streamflow dynamics and sediment production in the Puyango-Tumbes River basin across both seasonal and long-term time scales. As the demand for LULC modifications within the basin continues to escalate, it is imperative to systematically incorporate assessments of their impacts on the projected runoff and erosion regimes into the water resource decision-making processes.
Data availability statement
The raw data supporting the conclusions of this article will be made available by the authors, without undue reservation.
Author contributions
RP-M: Conceptualization, Data curation, Formal Analysis, Funding acquisition, Investigation, Methodology, Project administration, Resources, Software, Supervision, Validation, Visualization, Writing–original draft, Writing–review and editing. WL-C: Formal Analysis, Funding acquisition, Methodology, Project administration, Resources, Validation, Visualization, Writing–original draft, Writing–review and editing. LB: Conceptualization, Data curation, Formal Analysis, Funding acquisition, Investigation, Methodology, Project administration, Resources, Software, Supervision, Validation, Visualization, Writing–original draft, Writing–review and editing.
Funding
The author(s) declare that no financial support was received for the research, authorship, and/or publication of this article.
Acknowledgments
This study is supported by the Doctorate in Water Resources at the Universidad Nacional Agraria La Molina in Lima, Peru.
Conflict of interest
The authors declare that the research was conducted in the absence of any commercial or financial relationships that could be construed as a potential conflict of interest.
Publisher’s note
All claims expressed in this article are solely those of the authors and do not necessarily represent those of their affiliated organizations, or those of the publisher, the editors and the reviewers. Any product that may be evaluated in this article, or claim that may be made by its manufacturer, is not guaranteed or endorsed by the publisher.
References
Abbaspour, K. (2015b). SWAT-CUP calibration and uncertainty programs. Available at: https://swat.tamu.edu/media/114860/usermanual_swatcup.pdf.%0A.
Abbaspour, K., Rouholahnejad, E., Vaghefi, S., Srinivasan, R., Yang, H., and Kløve, B. (2015a). A continental-scale Hydrology and water quality model for Europe: calibration and uncertainty of a high-resolution large-scale SWAT model. Hydrology 524, 733–752. doi:10.1016/j.jhydrol.2015.03.027
Ali, Z., Mudassar Iqbal, I., Muhammad, U., Muhammad, A., Umer, M., Lodhi, M. U. K., et al. (2023). Hydrological response under CMIP6 climate projection in astore River Basin, Pakistan. J. Mt. Sci. 20 (8), 2263–2281. doi:10.1007/s11629-022-7872-x
Calvin, K., Dipak, D., Gerhard, K., Aditi, M., Thorne, P., Trisos, C., et al. (2023). IPCC, 2023: climate change 2023: synthesis report. Contribution of working groups I, II and III to the sixth assessment report of the intergovernmental Panel on climate change. Geneva, Switzerland: IPCC. doi:10.59327/IPCC/AR6-9789291691647
Chanapathi, T., and Thatikonda, S. (2020). Investigating the impact of climate and land-use land cover changes on hydrological predictions over the krishna River Basin under present and future scenarios. Sci. Total Eviromental 271. doi:10.1016/j.scitotenv.2020.137736
Cibin, R., Sudheer, P., and Chaubey, I. (2010). Sensitivity and identifiability of stream FLow generation parameters of the SWAT model. Hydrology 24, 1133–1148. doi:10.1002/hyp.7568
Dai, C., Qin, X., Lu, W., and Huang, Y. (2020). Assessing adaptation measures on agricultural water productivity under climate change: a case study of huai River Basin, China. Total Environ. 721, 137777. doi:10.1016/j.scitotenv.2020.137777
Farjad, B., Gupta, A., Sartipizadeh, H., and Cannon, A. (2019). A novel approach for selecting extreme climate change scenarios for climate change impact studies. Sci. Total Evriment 678, 476–485. doi:10.1016/j.scitotenv.2019.04.218
Funk, C., Peterson, P., Landsfeld, M., Pedreros, D., Verdin, J., Shukla, S., et al. (2015). The climate hazards infrared precipitation with stations—a new environmental record for monitoring extremes. Science 2, 150066. doi:10.1038/sdata.2015.66
Golian, S., Houcyne, I., and Stambuk, D. (2023). Using CMIP6 models to assess future climate change effects on mine sites in Kazakhstan. Hydrology 10 (7), 150. doi:10.3390/hydrology10070150
Goyburo, A. (2017). Monitoreo y Caracterización Del Transporte de Sedimentos Durante Crecidas o Eventos Extremos de El Niño En El Río Tumbes. Lima: Universidad Nacional Agraria La Molina.
Guo, Y., Yu, X., Ping, Y., Guoqing, W., Xie, J., and Gu, H. (2022). A comparative assessment of CMIP5 and CMIP6 in hydrological responses of the yellow River Basin, China. Hydrology Res. 53 (6), 867–891. doi:10.2166/nh.2022.001
Iqbal, M., Wen, J., Masood, M., Umer, M., and Adnan, M. (2022). Impacts of climate and land-use changes on hydrological processes of the source region of yellow river, China. Sustainability 14 (22), 14908. doi:10.3390/su142214908
Jajarmizadeh, M., Lafdani, E., Harun, S., and Ahmadi, A. (2014). Application of SVM and SWAT models for monthly streamflow prediction, a case study in South of Iran. KSCE J. Civ. Eng. 19, 345–357. Civil Engenier. doi:10.1007/s12205-014-0060-y
Jones, J., Almeida, A., Cisneros, F., Iroumé, A., Jobbágy, E., Lara, A., et al. (2017). Forests and water in South America. Hydrology 31, 972–980. doi:10.1002/hyp.11035
Kiprotich, P., Wei, X., Zhang, Z., Ngigi, T., Qiu, F., and Wang, L. (2021). Assessing the impact of land use and climate change on surface runoff response using gridded observations and Swat+. Hydrology 8 (1), 48. doi:10.3390/hydrology8010048
Krause, A., Haverd, V., Poulter, B., Anthoni, P., Quesada, B., Rammig, A., et al. (2019). Multimodel analysis of future land use and climate change impacts on ecosystem functioning. Earth’s Future 7 (7), 833–851. doi:10.1029/2018EF001123
Le, T., Seidler, C., Kändler, M., and Tran, T. (2012). Proposed methods for potential evapotranspiration calculation of the red River Basin (north vietnam). Hydrology 26, 2782–2790. doi:10.1002/hyp.8315
Liu, W., Bailey, R., Andersen, H., Jeppesen, E., Nielsen, A., Peng, K., et al. (2020). Quantifying the effects of climate change on hydrological regime and stream biota in a groundwater-dominated catchment: a modelling approach combining SWAT-MODFLOW with flow-biota empirical models. Sci. Total Environ. 745, 140933. doi:10.1016/j.scitotenv.2020.140933
MAP and GIS (2019). Plan de Acción Estratégica -PAE-de La Cuenca Transfronteriza Puyango Tumbes. Quito.
Marin, M., Clinciu, I., Tudose, C., Ungurean, C., Adorjani, A., Mihalache, A., et al. (2020). “Assessing the vulnerability of water resources in the context of climate changes in a small forested watershed using SWAT,” in A review (Eviron), 184.
Mendoza, P., Clark, M., Newman, A., Barlage, M., Gutmann, E., and Rasmussen, R. (2015). Effects of hydrologic model choice and calibration on the portrayal of climate change impacts. Hydrometeorology 16, 762–780. doi:10.1175/JHM-D-14-0104.1
Mohseni, U., Prasit, G., Agnihotri, C., Pande, B., and Durin, B. (2023). Understanding the climate change and land use impact on streamflow in the present and future under CMIP6 climate scenarios for the parvara mula basin, India. WaterSwitzerl. 15 (9), 1753. doi:10.3390/w15091753
Moradian, S., Haghighi, A., Asadi, M., and Mirbagheri, S. (2023). Future changes in precipitation over northern urope based on a multi-model ensemble from CMIP6: focus on tana River Basin. Water 37, 2447–2463. doi:10.1007/s11269-022-03272-4
Narsimlu, B., Gosain, A., and Chahar, B. (2023). Assessment of future climate change impacts on water resources of upper Sind River Basin, India using SWAT model. Water Resourses Manag. 27, 3647–3662. doi:10.1007/s11269-013-0371-7
Oliveira, L., Miranda, J., and Cooke, R. (2018). Water management for sugarcane and corn under future climate scenarios in Brazil. Agric. Water Manag. 201, 199–206. doi:10.1016/j.agwat.2018.01.019
Paiva, K., Rau, P., Montesinos, C., Lavado, W., Bourrel, L., and Frappart, F. (2023). Hydrological response assessment of land cover change in a Peruvian amazonian basin impacted by deforestation using the SWAT model. Remote Sens. 15 (24), 5774. doi:10.3390/rs15245774
Rennie, C., Millar, R., and Church, M. (2002). Measurement of bed load velocity using an acoustic Doppler current profiler. J. Hydraulic Eng. 128 (5), 473–483. doi:10.1061/(ASCE)0733-9429(2002)128:5(473)
Rhymee, H., Shams, S., Ratnayake, U., and Rahman, E. (2022). Comparing statistical downscaling and arithmetic mean in simulating CMIP6 multi-model ensemble over Brunei. Hydrology 9, 161. doi:10.3390/hydrology9090161
Riahi, K., Van Vuuren, D., Kriegler, E., Edmonds, J., O’Neill, B., Fujimori, S., et al. (2016). The shared socioeconomic pathways and their energy, land use, and greenhouse gas emissions implications: an overview. Glob. Environ. Change 42 (January), 153–168. doi:10.1016/j.gloenvcha.2016.05.009
Santos, C., Neto, T., Nascimento, R., Silva, R., Mishra, M., and Frade, T. (2021). Geospatial drought severity analysis based on PERSIANN-CDR-estimated rainfall data for odisha state in India (1983–2018). Sci. Total Environ. 750, 141258. doi:10.1016/j.scitotenv.2020.141258
Santos, S., Lima, M., Da Silva, R., Santos, C., Quinn, N., Dantas, A., et al. (2021). Modeling the impacts of future LULC and climate change on runoff and sediment yield in a strategic Basin in the caatinga/atlantic forest ecotone of Brazil. Catena 203 (August), 105308. doi:10.1016/j.catena.2021.105308
Silva, L., Da Silva, R., and Santos, C. (2020). Modeling land cover change based on an artificial neural network for a semiarid River Basin in northeastern Brazil. Glob. Ecol. Conserv. 21, e00811. doi:10.1016/j.gecco.2019.e00811
Silva, R., Marques, D., Gico, S., Montenegro, L., and Santos, C. (2012). Integration of GIS and remote sensing for estimation of soil loss and prioritization of critical sub-catchments: a case study of tapacurá catchment. Nat. Hazards 62 (3), 953–970. doi:10.1007/s11069-012-0128-2
UNDP (United Nations Development Programme) (2015). “Integrated water resources management in puyango-tumbes, catamayo-chira and zarumilla transboundary aquifers and river basins.”
Zhang, H., Wang, B., Liu, D., Zhang, M., Lance, M., and Yu, Q. (2020). Using an improved SWAT model to simulate hydrological responses to land use change: a case study of a catchment in tropical Australia. J. Hydrology 585 (March), 124822. doi:10.1016/j.jhydrol.2020.124822
Keywords: climate change, CMIP6, hydrological modeling, future scenario, flow, sediments, Puyango-Tumbes basin, Ecuador-Peru
Citation: Peña-Murillo R, Lavado-Casimiro W and Bourrel L (2024) Impacts of LULC and climate change on runoff and sediment production for the Puyango-Tumbes basin (Ecuador-Peru). Front. Remote Sens. 5:1471144. doi: 10.3389/frsen.2024.1471144
Received: 26 July 2024; Accepted: 19 November 2024;
Published: 04 December 2024.
Edited by:
Xiaojun Li, INRAE Nouvelle-Aquitaine Bordeaux, FranceReviewed by:
Mengjia Wang, Zhengzhou University, ChinaHuan Wang, Peking University, China
Xiaojing Bai, Nanjing University of Information Science and Technology, China
Copyright © 2024 Peña-Murillo, Lavado-Casimiro and Bourrel. This is an open-access article distributed under the terms of the Creative Commons Attribution License (CC BY). The use, distribution or reproduction in other forums is permitted, provided the original author(s) and the copyright owner(s) are credited and that the original publication in this journal is cited, in accordance with accepted academic practice. No use, distribution or reproduction is permitted which does not comply with these terms.
*Correspondence: Robinson Peña-Murillo, MjAyMDA4NjdAbGFtb2xpbmEuZWR1LnBl