- 1Bio-optical Oceanography Laboratory, Department of Marine Sciences, University of Puerto Rico, Mayagüez, PR, United States
- 2Laboratory of Experimental Ecology, Department of Marine Sciences, University of Puerto Rico, Mayagüez, PR, United States
Decreased water quality in coastal environments due to land alterations by human activities has caused stressed and degraded coral reefs worldwide. The consequences of decreased water quality are not limited to coral reefs but also affect the quality of people’s lives by increasing the incidence of diseases, so areas highly impacted have been prioritized for management. The Guánica Bay Watershed Management Plan was developed to reduce the non-point sources of pollution that arrive at the bay and to protect adjacent coral reefs, however, 15 years have passed since its creation and management actions have not been evaluated. The purpose of this study was to evaluate the effectiveness of the management actions implemented in the Guánica Bay watershed. Therefore, the objective of this study was to describe temporal trends (2002–2008 and 2016–2022) of remotely sensed diffuse attenuation coefficient at 490 nm (Kd490), a water quality indicator, in one managed (Guánica Bay) and three non-managed (Guayanilla Bay, Descalabrado River, and Guanajibo River) estuaries in Southwestern Puerto Rico. This was achieved by integrating ocean-color satellite imagery from MERIS-Envisat and OLCI-Sentinel-3 sensors that were sampled using a beyond-Before-After-Control-Impact (beyond-BACI) approach. An additional oceanic site was selected to evaluate continuity between sensors estimates. The imagery was processed using SNAP to extract Kd490 values in the estuaries. The analysis for the beyond-BACI found significant differences between periods (before and after) but the changes were unique to each location within estuary. The lowest Kd490 values and variability within estuary was observed in Guánica (range 0.05–0.1 m-1) and the highest Kd490 values were observed in Guayanilla (0.35 m-1). The southern estuaries showed similar temporal trends, all having a peak in 2018 and a trough in 2020. Kd490 did not decrease in Guánica after the implementation of management actions, which can be related to the passage of several hurricanes during the after period. Further analysis should be done as new data is available and after the implementation of the last management actions suggested in the plan.
1 Introduction
Water quality in coastal environments has decreased in many areas around the world due to anthropogenic impacts (Mallin et al., 2000; Bordalo, 2003; Ahn et al., 2005; Dwight et al., 2011; Wiegner et al., 2021). Some of these human activities include watershed alteration for agriculture, irrigation practices, domestic and recreational activities, land development, and littering (Khatri and Tyagi, 2015; Sidabutar et al., 2017; Tavakoly Sany et al., 2019). Furthermore, according to a recent investigation conducted by Lin and colleagues (2022), over 80% of sewage produced by human actions is released into rivers and oceans untreated, leading to environmental contamination and over 50 different diseases, affecting valuable coastal ecosystems and the quality of people’s lives (Halliday and Gast, 2011; Freeman et al., 2019; Nalley et al., 2021; Lin et al., 2022). As a result, efforts to reverse the effects of poor planning of land alterations have been made in some regions, one example being the creation of the Guánica Bay Watershed Management Plan (CWP, 2008).
The Guánica Bay Watershed is a 151 square miles system located in southwest Puerto Rico, encompassing Guánica’s municipality, a portion of Yauco, Sabana Grande, San Germán, Maricao, Adjuntas, and Lares municipalities, and a segment of the Lajas Valley Agricultural region, all of which drain to the bay through the Loco River (CWP, 2008; Viqueira and Rios, 2018). Watersheds in southern Puerto Rico have been highly developed to sustain domestic activities, irrigation, and hydroelectric power infrastructures (CWP, 2008; Gómez-Gómez et al., 2014). Specifically, in Guánica, the Guánica Lagoon, which covered an area of approximately 1,200 acres, was drained in the 1950s as part of the Southwest Puerto Rico Project for agricultural and hydroelectric power purposes (Viqueira Rios et al., 2012). These developments come with a cost to marine coastal ecosystems, as impacts such as marine sedimentary deposition, eutrophication, and shifts on coastal species composition have been reported in some countries (Morais, 2008; Sanger et al., 2013).
One of the most impacted ecosystems of watershed development are adjacent coral reefs that face challenges like the introduction of pollutants causing nutrient enrichment beyond tolerance of reefs, along with marine sedimentary deposition, pathogens, and toxic contaminants (Richmond et al., 2007; CWP, 2008). In Puerto Rico, stressed and degraded coral reefs have been reported along the insular shelf, particularly in the Guánica to Guayanilla region in the southwest (Warne et al., 2005). As a result, the Guánica Bay Watershed was selected as a priority area for management by the U.S. Coral Reef Task Force (Whitall et al., 2013; Takesue et al., 2021). The Guánica Bay Watershed Management Plan was developed in 2008 to reduce the non-point sources of pollution that arrived at the bay and protect adjacent coral reefs (CWP, 2008). Some of the recommendations in the plan included the restoration of the Guánica Lagoon, agricultural outreach, the creation of treatment wetlands, among others (CWP, 2008). Reports created by Viqueira Ríos, 2018, Viqueira Ríos, 2021) highlight the management actions implemented from 2012 to 2022, which include the application of hydroseeding (2013–2017), training farmers on Best Management Practices (BMP) and shade coffee farming, and the creation of infiltration systems in farms using native trees and vetiver grass (2010–2016), to name a few. However, some of these management actions, i.e., the restoration of the Guánica Lagoon and the creation of the treatment wetlands, which were the first two most important recommendations from the management plan, had not yet been implemented by the end of this work.
Assessment of watershed management requires integrating a broad range of spatial and temporal data (Wang et al., 2016). Although no reports assessing the effectiveness of the management actions in Guánica were found, refinements and suggestions to improve the management plan considering stakeholder concerns have been made (Carriger et al., 2013; Smith et al., 2017). Therefore, the goal of this work was to evaluate the effectiveness of the management actions implemented from the Guánica Bay Watershed Management Plan created to protect adjacent coral reefs using remote sensing techniques. We aimed to describe temporal trends of the diffuse attenuation coefficient at 490 nm (Kd490) in one managed (Guánica Bay) and three non-managed (reference) estuaries in Southwest Puerto Rico before and after the implementation of the Guánica Bay Watershed Management Plan. It is noteworthy to mention that during the after period of this study the following major events affected our area of study: Hurricanes Irma and María in 2017, and Fiona in 2022, with significant impacts to the area. Also, the Covid-19 pandemic outbreak and associated lockdowns, and a magnitude 6.4 earthquake near Guánica, all of which could result in decreased human impact in the watershed and coastal waters.
The diffuse attenuation coefficient (Kd) of downward irradiance is an apparent optical property of water widely used in oceanographic studies, a good indicator of turbidity, and one of the parameters for which ocean color algorithms have been developed using satellite sensors (Lee et al., 2005; Wang et al., 2009; Tomlinson et al., 2019; Y; Wang et al., 2022). For this purpose, the European Space Agency (ESA) launched in 2002 the Medium Resolution Imaging Spectrometer (MERIS) on board the Envisat satellite to provide ocean color observations. The Ocean Land Colour Instrument (OLCI) was launched in 2016 and 2018 on Sentinel-3 A and B, respectively, as successors of MERIS to ensure long term monitoring with improvements such as additional spectral bands, mitigation of sun-glint by tilting the sensors, and improved signal to noise ratio (Nieke et al., 2015). In Puerto Rico, satellite estimates have been used to study long term trends and disturbance impacts (e.g., hurricanes) in coastal water quality using chlorophyll-a, Kd at 490 nm (Kd490), and absorption of colored dissolved organic matter (Gilbes et al., 2001; Amirrezvani, 2016; García-Sais et al., 2017; Hernández et al., 2020; Ortiz-Rosa et al., 2020). Furthermore, an inverse relationship between Kd and percent coral cover was observed in Ponce, Guayanilla, La Parguera, Mayagüez Bay, and other areas, which suggests that Kd can be used for indirect assessment of the percent living coral cover in coral reefs (Cardona-Maldonado, 2008; García-Sais et al., 2017; Freitas et al., 2019). While Puerto Rico has no coastal water quality standards of light attenuation, Hernández et al. (2020) suggest using 0.1 m-1 to reference degraded water quality adopted from Hawai’i standards.
If management actions implemented in the Guánica Bay watershed have been effective, it was expected that: i) values of Kd490 decreased in Guánica Bay after the implementation of management actions, and ii) this temporal change would be observed only in Guánica Bay as compared to reference estuaries. It is important to note that the matter in question was not to compare estuaries as they are part of different watersheds and might change through time but to detect whether trends in Guánica Bay were unique when compared to reference estuaries which could be attributable to the implementation of management actions to this watershed.
2 Materials and methods
2.1 Study area
The areas of interest for this study were one managed and three not managed estuaries located in south and southwest Puerto Rico (Figure 1). Puerto Rico has two defined seasons, the dry (December-April) and wet (May-November) seasons (Hernández Ayala and Méndez Tejeda, 2023). Guanajibo River, located in southwestern Puerto Rico, has a relatively humid climate and prevailing winds are from the west, however, the southern estuaries, Guánica Bay, Guayanilla Bay and Descalabrado River, have a semi-arid climate and wind direction is from the northeast from midnight to early morning, and from the southeast during the day (Gómez-Gómez et al., 2014). Guánica and Guayanilla Bays are estuaries classified as semi-closed bays where exchange between fresh and seawater is direct. On the other hand, Guanajibo and Descalabrado estuaries are river mouths, which tend to be stratified as seawater is denser than fresh water (DNER, 2016). Seawater temperatures in Puerto Rico do not change much since solar radiation is nearly constant throughout the year, with October having a mean maximum water temperature of 28°C, and January having the mean minimum of 24°C (Gómez-Gómez et al., 2014). Three of the study sites are in regions where stressed coral reefs have been reported, these sites are Guanajibo River, Guánica, and Guayanilla Bays, in contrast, coral reefs from the south where Descalabrado River is have been classified as “healthy” (Warne et al., 2005). The water depth of the estuaries was assessed using bathymetry data for Puerto Rico derived from Sentinel-2 imagery and the apparent optical depth (AOD = 1.3/Kd), which is the depth at which light penetration is optically significant, was calculated (Figure 2; Supplementary Table S1) (Bailey and Werdel, 2006; Li et al., 2021). Lastly, the shelf sediment composition in the southern estuaries is carbonate clastic, which has the effect of increasing Kd, and in the western estuary is mixed terrigenous and carbonate clastic sediments (Warne et al., 2005; Hochberg et al., 2020).
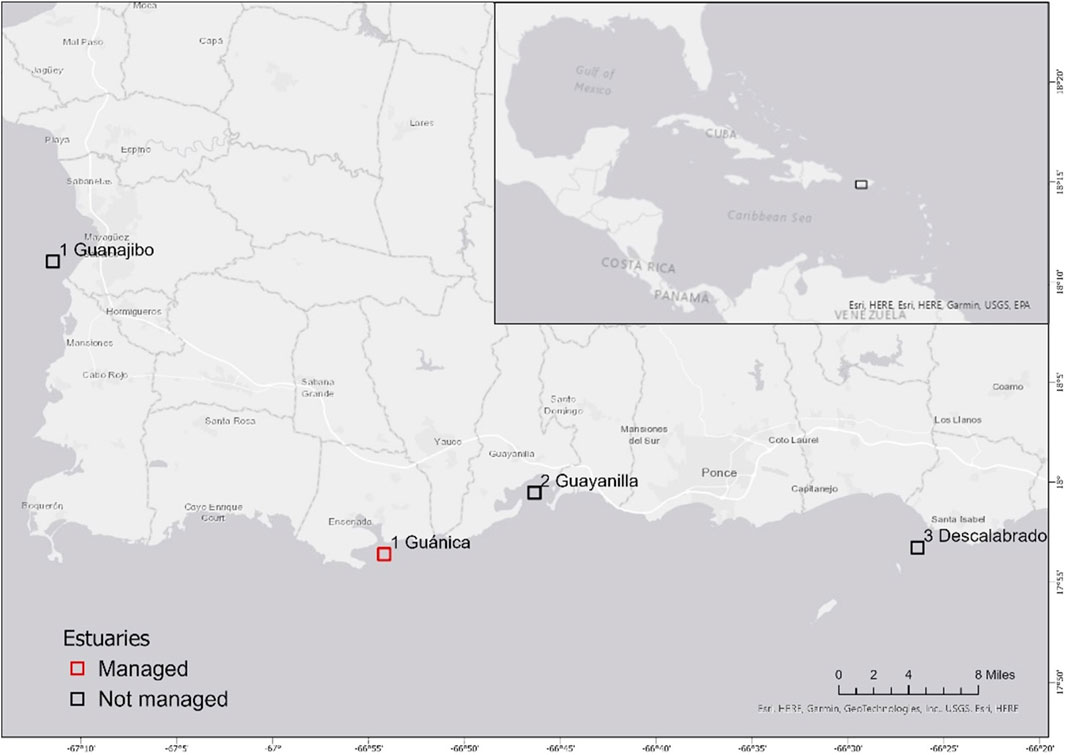
Figure 1. Map of the study area in Southwest Puerto Rico consisting of one managed estuary (Guánica Bay) and three not managed estuaries (1: Guanajibo River, 2: Guayanilla Bay, 3: Descalabrado River).
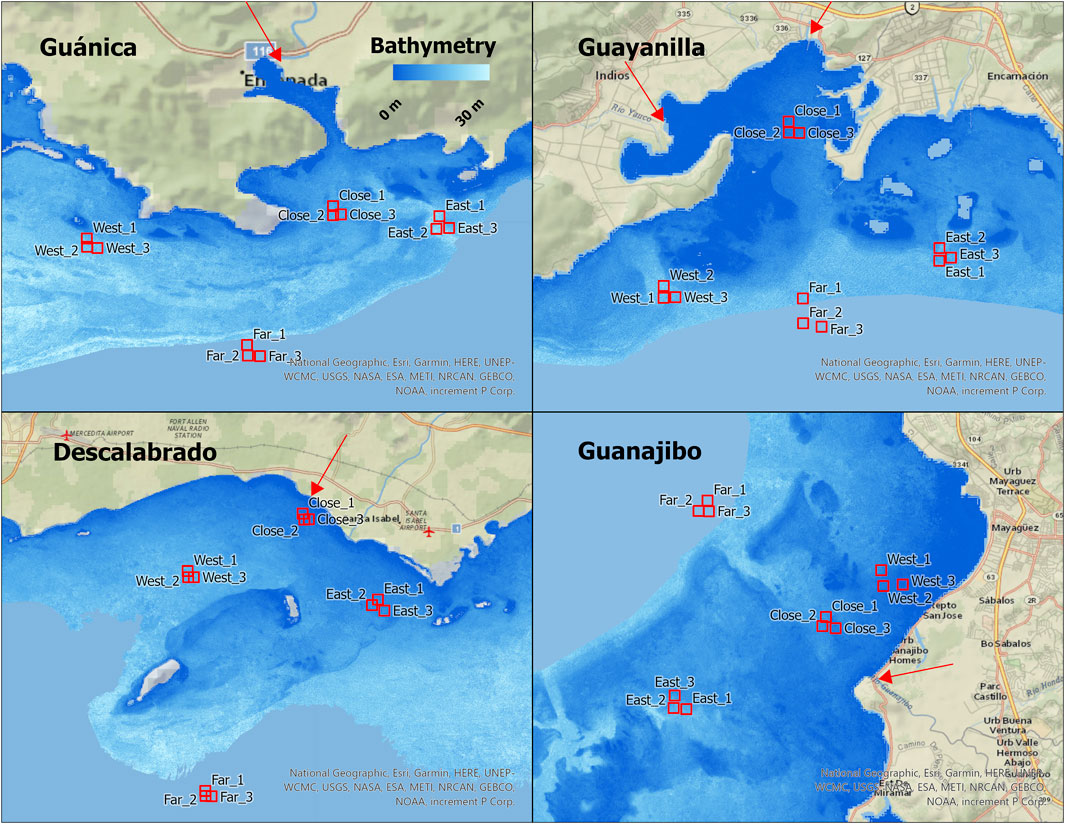
Figure 2. Map showing the four estuaries selected for the study with the locations and their bathymetries. The red arrows indicate the river mouths and where the bathymetry layer ends it is the shelf break.
2.2 Study design
To assess changes of Kd490 related to the implementation of the Guánica Bay management plan, a beyond-Before-After-Control-Impact (beyond-BACI) design was used (Figure 3). This method, proposed to evaluate environmental impacts, consists in measuring the variable of interest before and after the occurrence of an anthropogenic disturbance at the location of the disturbance and reference location/s (Green, 1979; Hurlbert, 1984; Stewart-Oaten et al., 1986; Underwood, 1994). It has been described as one of the most powerful models for assessing environmental impacts when well-designed (Smokorowski and Randall, 2017; Bertocci et al., 2021). Additionally, the BACI design has been combined with novel remote sensing techniques to develop a web-based application to monitor changes in vegetation using the MODIS sensor (Poortinga et al., 2018). BACI studies in the ocean have been in acoustical studies, but it is not a widely used method in oceanography (Seger et al., 2021).
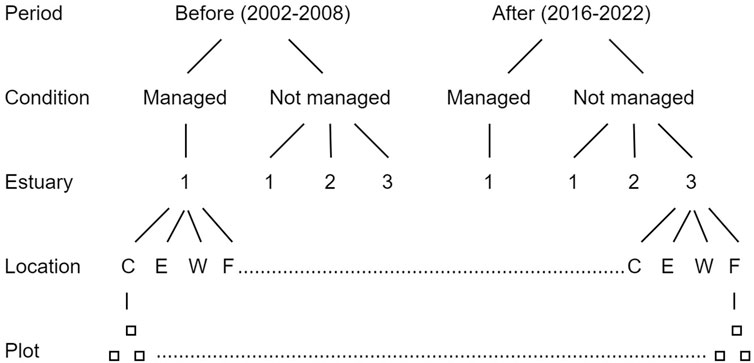
Figure 3. The beyond-BACI approach used to evaluate management efforts for the Guánica Bay Watershed. The locations are classified as C: Close, E: East, W: West, and F: Far from the river mouths. The plot boxes indicate the number of pixels selected for analysis at each location (3).
The before period for this study consists of 6 years before the development of the Guánica Bay Watershed Management Plan (2002–2008) and the after period consists of 6 years after (2016–2022) in which management actions were implemented in the Guánica Bay Watershed (Viqueira Rios, 2018). There is an 8-year gap between both periods because of a gap between sensors (2012–2016) and to ensure implementation of the management recommendations. In each estuary, the managed and three not managed, four locations were selected in terms of proximity to the river mouths. These locations were classified as Close, East, West, and Far from the river mouths (Figure 2). The Far locations at all estuaries were selected outside of the insular shelf (offshore). Bailey and Werdell (2006) recommend the use of a pixel box for more accurate satellite estimations, nonetheless, at each location the plot consisted of three pixels randomly selected instead of a pixel box, avoiding land and bottom reflectance interference, due to limitations in the spatial resolution of the satellite sensors (300 m) used for the proposed study sites.
2.3 Remotely sensed data collection and analysis
Level 2 Water Full Resolution Imagery for Puerto Rico was obtained from two sensors, MERIS on board Envisat and OLCI on board Sentinel-3 A and B. MERIS imagery was downloaded through the MERIS Online Dissemination Service and OLCI imagery was downloaded from the EUMETSAT Data Services portal (EUMETSAT, 2017; ESA, 2020; EUMETSAT, 2022). The MERIS dataset for this study covers the years 2002–2008 and the OLCI dataset covers 2016–2022. Using different sensors would not affect data analysis as the fourth MERIS reprocessing was focused to align with Sentinel-3/OLCI data processing chains to allow for continuity of the products derived from the instruments (Alhammoud and Meris Validation Team, 2019). Nonetheless, an oceanic site was selected in the northwest of Puerto Rico, where terrestrial input is unlikely to affect the continuity evaluation of the sensors (Supplementary Figure S1). The satellite imagery was processed using the SeNtinel Application Platform (SNAP), where a series of steps were built using the graph builder tool to allow for batch processing of the imagery (Figure 4). The pixel extraction of Kd490 values for each location within estuary and the additional oceanic site was done using the Kd fail mask as a filter to remove contaminated pixels, but any value higher than 1 m-1 was removed from the dataset before analysis because they corresponded to contaminated pixels, e.g., land contamination (Figure 4). Lastly, the Level-3 Binning tool was used to generate Kd490 averages for the before and after periods and the Band Maths tool was used to generate a difference map for our area of interest. The algorithm used to derive the Kd490 product was developed by Morel et al. (2007) and is suitable for non-turbid ocean waters (Kd490 < 0.3 m-1) as is the case in our area of study (Wang et al., 2009; García-Sais et al., 2017; Hernández et al., 2020; Ortiz-Rosa et al., 2020). It uses a ratio of the irradiance reflectance at 490 nm and 560 nm:
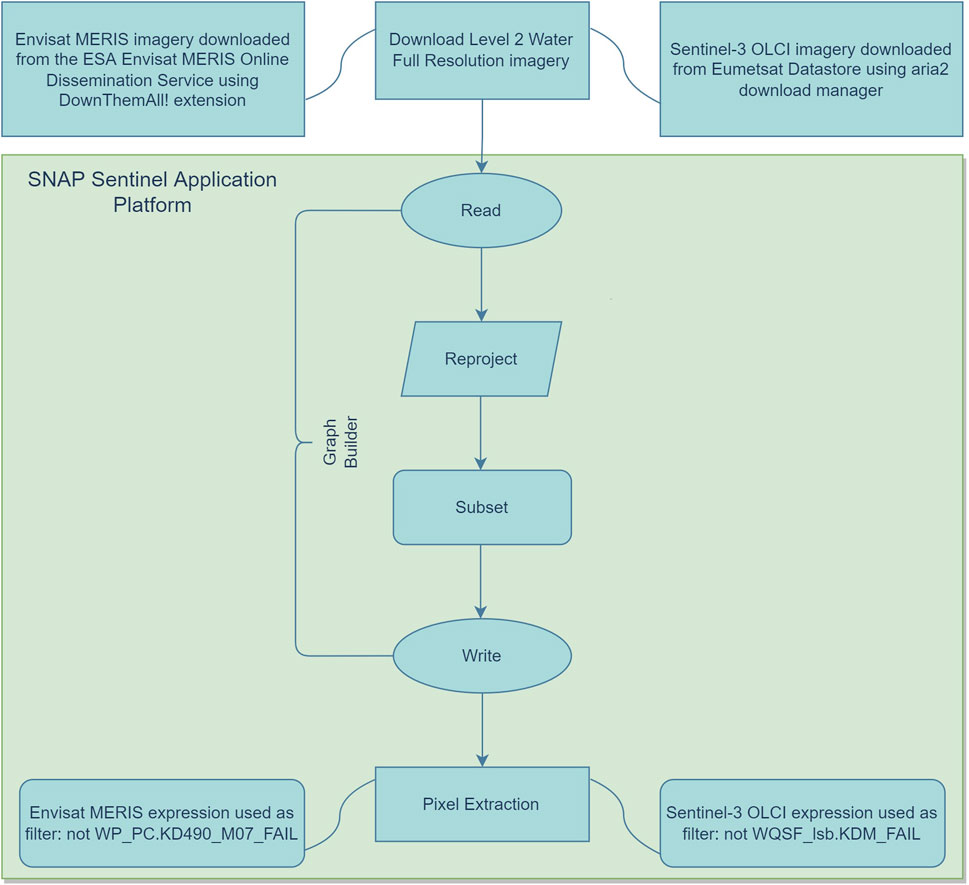
Figure 4. Workflow used to download and process satellite imagery using the SeNtinel Application Platform and to extract Kd490 values in southwest Puerto Rico.
A permutational multivariate analysis of variance (PERMANOVA) was used to analyze the reduced data for which a centroid was calculated for each season (wet and dry) per year using PRIMER v7 (Clarke and Gorley, 2015). This multivariate model considers that Kd490 can respond differently to multiple factors, specifically the condition of managed and not managed, localization of the estuary, and location/proximity to the mouth of the river within the estuary (Figure 2). The analyses used 9,999 permutations of the residuals under a reduced model to produce a null distribution (Anderson et al., 2008).
3 Results
3.1 Oceanic site
A total of 269 valid pixels of Kd490 estimates were extracted from the MERIS dataset (2002–2008) and a total of 1,276 estimates were extracted from the OLCI dataset (2016–2022) at the oceanic site to allow comparison between sensors. The analysis to evaluate the continuity of the sensors estimates in the oceanic site shows that there is not a significant change (p = 0.06) between sensor estimates (Table 1).

Table 1. Analysis of variance for the oceanic site of the diffuse attenuation coefficient at 490 nm (Kd490) values in the Before (2002–2008) and After period (2016–2022).
3.2 Patterns of Kd490 in the estuaries
As for the results of the main goal of this study, the PERMANOVA results indicated that interaction at the lowest level, year by estuary, is statistically significant (p = 0.001) (Table 2); indicating that the patterns of temporal variation were not the same for each estuary (Figure 5). Interaction at the second lowest level, period by location, was also significant (p = 0.0001); also indicating that temporal changes detected between periods were unique for each location within estuary. The interactions between year and condition, period by estuary, and period by condition were not statistically significant (p > 0.05).
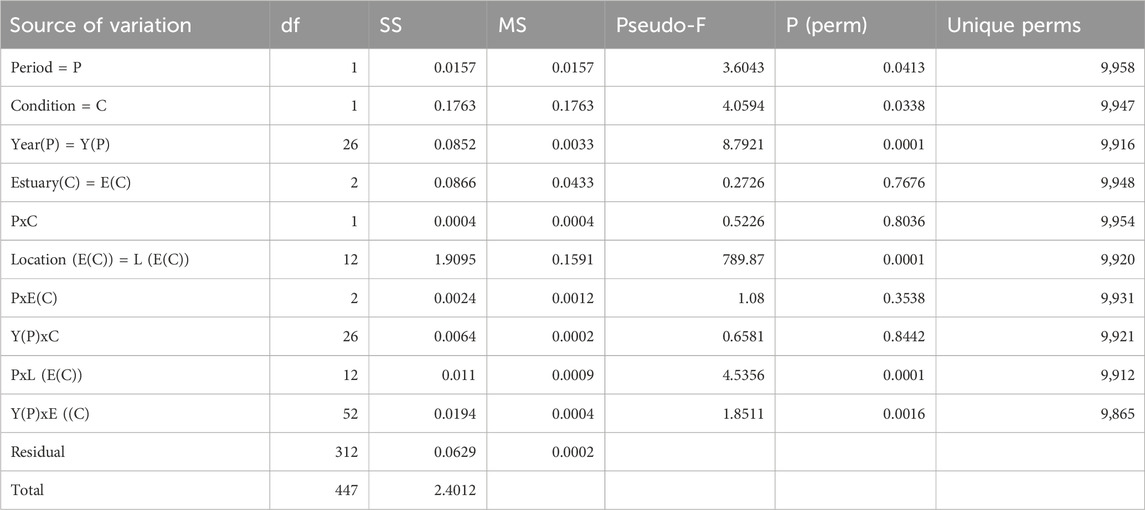
Table 2. Permutation multivariate analysis of variance on a mixed multifactorial model for the managed and not managed estuaries before and after the implementation of management actions.
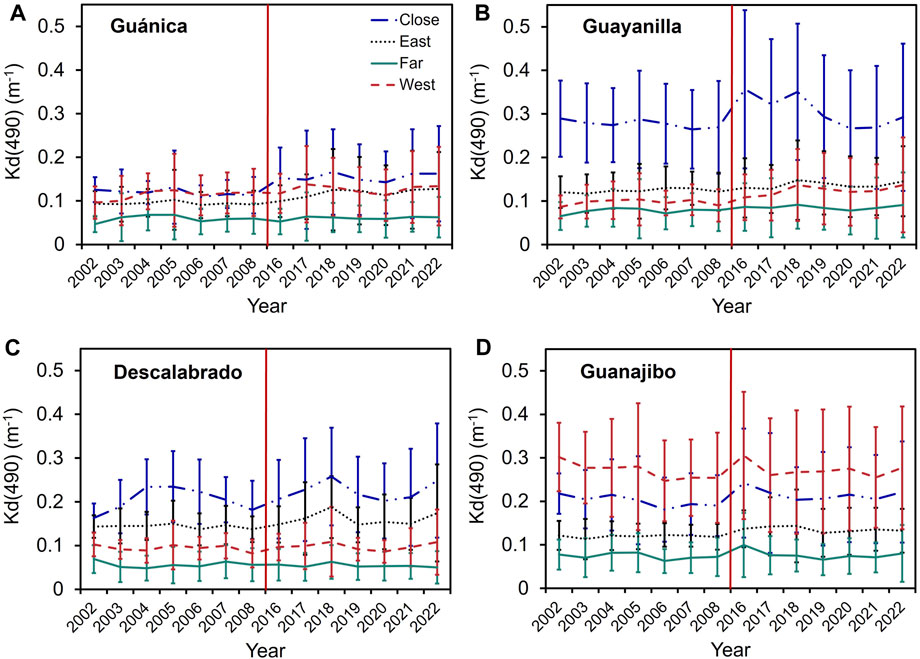
Figure 5. Kd490 distributions in (A) Guánica Bay, (B) Guayanilla Bay, (C) Descalabrado River, and (D) Guanajibo River. The legend to identify each location within estuary is in panel (A). The red line separates the before and the after periods.
Overall, the lowest Kd490 values were observed in Guánica, while the highest values were observed in Guayanilla Bay, particularly in the Close location (Figures 5A, B). In Guánica Bay, Kd490 values ranged from ∼0.05–0.15 m-1 and in Guayanilla ranged from ∼0.05–0.3 m-1 except for two anomalies in 2016 and 2018 (Figures 5A, B). In Descalabrado they ranged from ∼0.05–0.25 m-1 and in Guanajibo from ∼0.05–0.3 m-1 (Figures 5C,D). From Figures 5A–D a general pattern of Kd490 in the estuaries can be seen, which was the Far location having lower Kd490 values than other locations, as expected. Another generalized pattern was the Close location having the highest Kd490 values at all estuaries in the southern region, but this was not observed in the Guanajibo River, probably due to differences between wind patterns and that the West location was closest to land in this site. In the southern estuaries, a peak can be observed in 2018, the year after the passage of Hurricane Maria on the island, and a decrease in 2020, the year of the Covid-19 pandemic outbreak and a 6.4 magnitude earthquake offshore of southwest Puerto Rico, indicating less human impact during this year (Figures 5A, B, D). The averages from the before and after period showed Kd490 values ranging from 0.02 m-1–1 m -1 and the difference plot (After-Before) showed increasing values in the insular shelf and decreasing values in the open ocean (Figure 6).
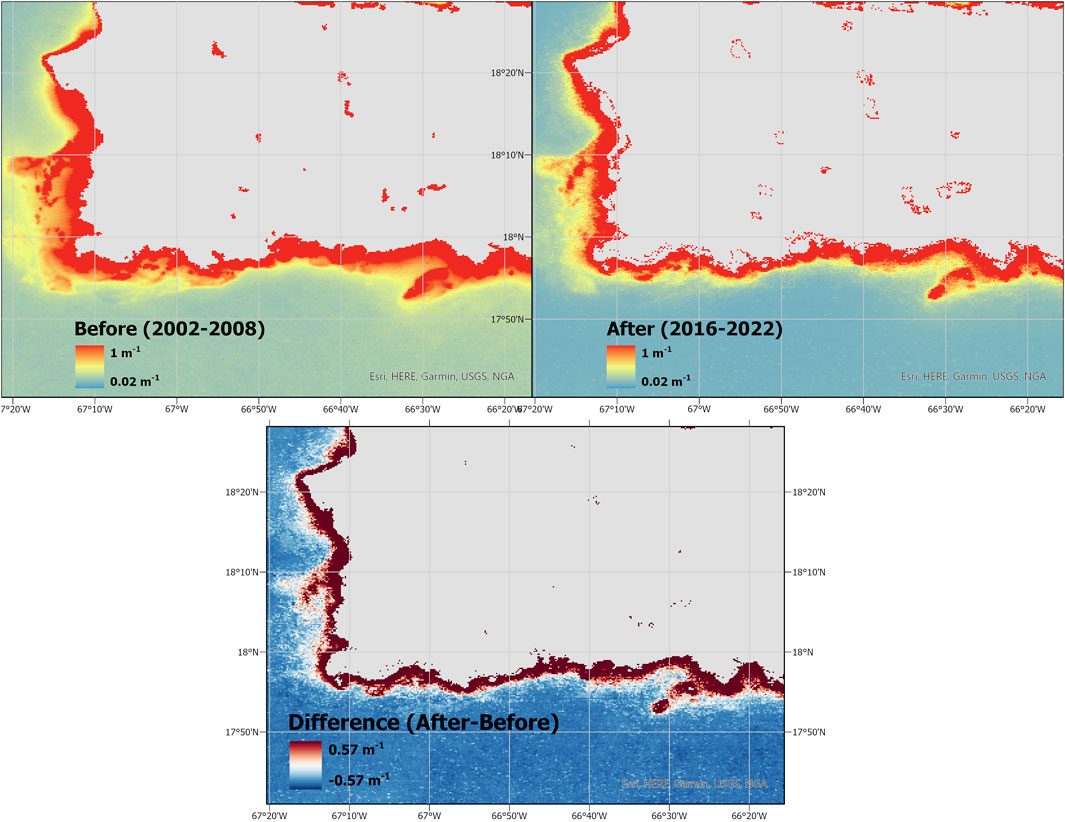
Figure 6. Maps of Southwest Puerto Rico showing the average Kd490 values for the before and after periods with the difference between periods (after-before).
3.2.1 Guánica Bay
The Guánica Bay estuary showed the least variability within locations of all estuaries, having Kd490 values close to 0.1 m-1 in all locations, except in the Far (offshore) location where values were closer to 0.05 m-1 (Figure 5A). Observations from the Before period showed steady trends, i.e., no increase or decrease of Kd490 at all locations, the West location being the most variable. In the After period, more variability and a subtle upward trend can be observed in all locations except for the Far location. The Close, East, and West locations followed similar patterns of temporal variability in the After period, more noticeably in the last 5 years. In these locations, an increase of Kd490 can be observed in the 2016–2018 period, and a decrease in the 2019–2020 period, after which it started to increase again.
3.2.2 Guayanilla Bay
Guayanilla Bay showed significantly higher Kd490 values, ∼0.3 m-1, in the Close location as compared to the rest of the locations where not much variability was observed (Figure 5B). In the Before period, all locations remained steady throughout time. The Close location had noticeable peaks in the 2016–2018 period, which showed a ∼0.05 m-1 increase when compared to the rest of the period. Similar patterns as those of Guánica Bay can be observed at all locations in the after period, where the 2016–2018 period followed an upward trend and the 2019–2020 period followed a downward trend after which it started to increase again. In the After period, the East and West location followed a more similar pattern and closer values than the Before period.
3.2.3 Descalabrado River
The second most variable estuary within locations was the Descalabrado River estuary ranging from ∼0.5–0.25 m-1 (Figure 5C). The Before period showed steady trends of Kd490 at the East, West, and Far locations while the Close location showed an upward trend up to 2005 after which it started to decrease. In the After period, similar patterns as the ones described for Guánica and Guayanilla Bays are observed in the Close, East, and West locations. The 2016–2018 period showed an upward trend and the 2019–2020 period showed a downward trend after which it started to increase. The Far location appeared to show a slight decrease of Kd490 for this period.
3.2.4 Guanajibo River
The Guanajibo River estuary showed the most variability of Kd490 within locations with a range of ∼0.5–0.3 m-1 (Figure 5D). All of them followed very similar patterns at different amplitudes. This estuary was the only where the West location reported the highest Kd490 values. The West and Close locations showed a downward trend in the Before period. In the After period, a peak can be observed in 2016, except for the East location where it is not noticeable, and no upward or downward trend can be observed.
4 Discussion
To evaluate the effectiveness of the Guánica management actions, it was hypothesized that Kd490 values would decrease in Guánica after the implementation of the management actions. The temporal distribution patterns observed were not consistent with an improvement, i.e., a decrease of Kd490, following the management actions, thus, the hypothesis is rejected. The results of the analysis indicated that there were differences between the before and after periods, but they were unique to each location within the estuary. This means that variability within the estuaries was high, and each location followed different patterns of temporal variability of Kd490 driven by conditions unique to the location. Even in the managed estuary, Guánica Bay, variability of turbidity between the locations (Close, East, West, and Far) was high. The oceanic continuity analysis indicated that there was not a significant difference between sensor estimates, which allows for the integration of the sensors estimates for long-term trend monitoring and time series analysis. Any differences between sensor estimates can be explained by the improvements applied in the OLCI sensor (mitigation of sun-glint, improved signal-to-noise ratio) and improved revisit times, thus being able to detect more variability and accurate estimates than the MERIS sensor (See Supplementary Figure S2 showing the increase in frequency sampling) (Nieke et al., 2015).
Waters in the estuaries can be considered non-turbid ocean waters (Kd490 < 0.3 m-1) (Wang et al., 2009). Generalized patterns were observed in the southern estuaries which showed the highest Kd490 values in the Close location and the lowest values in the Far location as expected. The Guanajibo River estuary, located in southwest Puerto Rico, followed different patterns which can be attributed by the difference in prevailing trade winds in this area causing higher resuspension rates near the shore (Miller and Cruise, 1995; Gómez-Gómez et al., 2014). The 2018 peak observed in the southern estuaries coincides with the fact that the impact of Hurricane Maria in September 2017 has been reported to have lasted up to 4 months after its passage through the island driven by mass wasting, sediment runoff, coastal turbidity, and bottom sediment resuspension (Takesue Sany et al., 2019). Keellings and Hernández Ayala, (2019) reported the extreme rainfall event associated with the hurricane while Bessette-Kirton et al. (2019) reported a low landside density (1–25 landslides/Km2) in the upland region of the Guánica Bay watershed. In terms of water quality, Ortiz-Rosa and colleagues (2020) reported that absorption of colored dissolved organic matter or detrital gelbstoff (aCDOM/ADG) was high (>0.13 m-1) in Guánica 4 months after the event as well as the coastal total suspended sediment (TSM) (∼5–6 mg/L) values as reported by Miller et al. (2019). Additionally, Hernández et al. (2020) reported high Kd490 (>0.1 m-1) and Chlorophyll-a concentration (>0.45 μg/L) values and Cheriton et al. (2019) reported increased concentrations of suspended particulate material (SPM) and polychlorinated biphenyls (PCBs), a highly toxic industrial contaminant, after the hurricane. This peak was not observed in the Guanajibo River which could be explained due to the low impact of the hurricane on the west side of the island and possibly to its difference in sediment type, i.e., mixed terrigenous and carbonate clastic. The COVID-19 lockdowns as well as a major earthquake offshore of southwest Puerto Rico in 2020 caused less anthropogenic disturbance to the coastal environment in this area, and therefore improved water quality, which is why a trough can be observed in 2020–2021 in some of the estuaries, a phenomenon also observed in other areas of the world (Supplementary Figure S2) (Callejas et al., 2021; Ormaza-González et al., 2021; Vijay Prakash et al., 2021).
This study is limited to changes observed in light attenuation and does not account for other coral reef threats such as increased seawater temperature and disease outbreaks (Rogers and Ramos-Scharrón, 2022). Events of resuspension, changes in precipitation, and tropical cyclone frequency and intensity cannot be eliminated as sources of change in Kd490 values. Furthermore, Guánica’s water discharge is managed and not direct and the primary discharge occurs in Guánica Bay and then flushes out to the south where our stations were located. Additionally, a recent study found that watersheds located east of Guánica are a predominant source of sediments to Guánica’s reefs, suggesting that management efforts should also be implemented in these watersheds (Takesue et al., 2021). In addition, the impact of the hurricanes on water clarity probably outweighed the efforts made in the watershed to improve water quality. It has also been of discussion that ecosystems follow complicated responses after restoration efforts because there can be a lag time between efforts and evidence of recovery as well as other environmental stressors, e.g., climate change, affecting water quality, resulting in shifting baselines (Jeppesen et al., 2005; Duarte et al., 2009).
This work is a preliminary assessment of the management actions implemented in the Guánica Bay Watershed by 2022, in which the two most important recommendations by the management plan, i.e., restoration of the Guánica Lagoon and construction of treatment wetlands, had not been completed (Viqueira Rios, 2021). Future assessments should be done after the implementation of these two management actions and a more comprehensive analysis that includes other remotely sensed water quality parameters and in situ measurements is recommended.
This study is the first attempt, to our knowledge, to evaluate the Guánica Bay Watershed Management Plan in terms of water quality. Results from this work could potentially contribute to determining whether current strategies in the Guánica Bay watershed should be continued, stopped, or modified. Other contributions could include the implementation of management actions in other watersheds with anthropogenic impacts like those of the Guánica Bay Watershed or the refinement of future watershed management plans developed to improve water quality in Guánica Bay and other areas around the world.
Data availability statement
The original contributions presented in the study are included in the article/Supplementary Material, further inquiries can be directed to the corresponding author.
Author contributions
AG-M: Conceptualization, Data curation, Formal Analysis, Investigation, Methodology, Visualization, Writing–original draft, Writing–review and editing. RA: Conceptualization, Funding acquisition, Resources, Supervision, Writing–review and editing. JC-M: Conceptualization, Formal Analysis, Methodology, Supervision, Visualization, Writing–review and editing. WH: Conceptualization, Funding acquisition, Resources, Supervision, Writing–review and editing.
Funding
The author(s) declare that financial support was received for the research, authorship, and/or publication of this article. This publication was made possible by the National Oceanic and Atmospheric Administration, Office of Education Educational Partnership Program under Agreement No. NA16SEC4810006. Any opinions, findings, conclusions, or recommendations expressed in this publication are those of the author(s) and do not necessarily reflect the view of the U.S. Department of Commerce, National Oceanic and Atmospheric Administration.
Acknowledgments
Thanks to colleagues Ángel A. Acosta-Colón for his valuable feedback during this research and Jenniffer Pérez Pérez for her guidance in image processing. Thanks to Isabel Ríos from the Information Technology Center at the University of Puerto Rico, Mayagüez Campus for her support with ArcGIS Pro software and to Clark E Sherman for his input to coastal sediment processes. Lastly, thanks to Michelle Tomlinson, Sachi Mishra, Andrew Meredith, Richard Stumpf, and the NCCOS team for their training and help during Kd490 evaluation efforts in Puerto Rico.
Conflict of interest
The authors declare that the research was conducted in the absence of any commercial or financial relationships that could be construed as a potential conflict of interest.
Publisher’s note
All claims expressed in this article are solely those of the authors and do not necessarily represent those of their affiliated organizations, or those of the publisher, the editors and the reviewers. Any product that may be evaluated in this article, or claim that may be made by its manufacturer, is not guaranteed or endorsed by the publisher.
Supplementary material
The Supplementary Material for this article can be found online at: https://www.frontiersin.org/articles/10.3389/frsen.2024.1404323/full#supplementary-material
References
Ahn, J. H., Grant, S. B., Surbeck, C. Q., Digiacomo, P. M., Nezlin, N. P., and Jiang, S. (2005). Coastal water quality impact of stormwater runoff from an urban watershed in Southern California. Environ. Sci. Technol. 39 (16), 5940–5953. doi:10.1021/es0501464
Alhammoud, B.MERIS Validation Team (2019). MERIS validation and algorithm 4th reprocessing –MERIS validation team (MVT) (final report). Available at: https://earth.esa.int/eogateway/documents/20142/37627/MERIS-Validation-Team-MVT-Final-Report.pdf.
Amirrezvani, A. (2016). Long-term trends in water quality parameters in coastal waters of Puerto Rico. Mayaguez: University of Puerto Rico.
Anderson, M. J., Gorley, R. N., and Clarke, K. R. (2008) PERMANOVA+ for PRIMER: guide to software and statistical methods. China: PRIMER-E Ltd, 1–214.
Bailey, S. W., and Werdell, P. J. (2006). A multi-sensor approach for the on-orbit validation of ocean color satellite data products. Remote Sens. Environ. 102 (1–2), 12–23. doi:10.1016/j.rse.2006.01.015
Bertocci, I., De Oliveira Martins, M. I., Meyer, H. S., Gómez, O. B., Maggi, E., and Arenas, F. (2021). Resurvey of sea urchins and mussels at protected and harvested shores a decade after: a beyond-BACI approach. Mar. Environ. Res. 169, 105347. doi:10.1016/j.marenvres.2021.105347
Bessette-Kirton, E. K., Cerovski-Darriau, C., Schulz, W. H., Coe, J. A., Kean, J. W., Godt, J. W., et al. (2019). Landslides triggered by hurricane Maria: assessment of an extreme event in Puerto Rico. GSA Today 29 (6), 4–10. doi:10.1130/GSATG383A.1
Bordalo, A. A. (2003). Microbiological water quality in urban coastal beaches: the influence of water dynamics and optimization of the sampling strategy. Water Res. 37 (13), 3233–3241. doi:10.1016/S0043-1354(03)00152-0
Callejas, I. A., Lee, C. M., Mishra, D. R., Felgate, S. L., Evans, C., Carrias, A., et al. (2021). Effect of COVID-19 anthropause on water clarity in the Belize coastal Lagoon. Front. Mar. Sci. 8, 490. doi:10.3389/fmars.2021.648522
Cardona-Maldonado, M. A. (2008). Assessment of coral reef community structure using water optical properties. Mayaguez: University of Puerto Rico.
Carriger, J. F., Fisher, W. S., Jr, T. B. S., and Sturm, P. E. (2013). Advancing the Guánica bay (Puerto Rico) watershed management plan. Coast. Manag. 41 (1), 37–41. doi:10.1080/08920753.2012.747814
Cheriton, O., Storlazzi, C., Rosenberger, K., and Sherman, C. (2019). Controls on sediment transport over coral reefs off southwest Puerto Rico: seasonal patterns and Hurricane Maria. Coast. Sediments, 903–915. doi:10.1142/9789811204487_0079
DNER (2016). Plan Integral de Recursos de Agua de Puerto Rico 2016. Available at: http://drna.pr.gov/documentos/plan-integral-de-recursos-de-agua-de-puerto-rico-revision-junio-2016/.
Duarte, C. M., Conley, D. J., Carstensen, J., and Sánchez-Camacho, M. (2009). Return to neverland: shifting baselines affect eutrophication restoration targets. Estuaries Coasts 32, 29–36. doi:10.1007/s12237-008-9111-2
Dwight, R. H., Caplan, J. S., Brinks, M. V., Catlin, S. N., Buescher, G., and Semenza, J. C. (2011). Influence of variable precipitation on coastal water quality in southern California. Water Environ. Res. 83 (12), 2121–2130. doi:10.2175/106143011x12928814444574
EUMETSAT (2017). OLCI level 2 ocean Colour Full resolution in NTC - sentinel-3, European organization for the exploitation of meteorological satellites. Available at: https://navigator.eumetsat.int/product/EO:EUM:DAT:SENTINEL-3:OL_2_WFR___NTC.
EUMETSAT (2022). OLCI level 2 ocean Colour Full resolution (version BC003) - sentinel-3 - reprocessed. Eur. Organ. Exploitation Meteorological Satell. doi:10.15770/EUM_SEC_CLM_0061
Freeman, L. A., Corbett, D. R., Fitzgerald, A. M., Lemley, D. A., Quigg, A., and Steppe, C. N. (2019). Impacts of urbanization and development on estuarine ecosystems and water quality. Estuaries Coasts 42 (7), 1821–1838. doi:10.1007/s12237-019-00597-z
Freitas, L. M., Oliveira, M. d. D. M., Leão, Z. M. A. N., and Kikuchi, R. K. P. (2019). Effects of turbidity and depth on the bioconstruction of the Abrolhos reefs. Coral Reefs 38 (2), 241–253. doi:10.1007/s00338-019-01770-3
García-Sais, J. R., Williams, S. M., and Amirrezvani, A. (2017). Mortality, recovery, and community shifts of scleractinian corals in Puerto Rico one decade after the 2005 regional bleaching event. PeerJ 5, e3611. doi:10.7717/peerj.3611
Gilbes, F., Armstrong, R. A., Webb, R., and Muller-Karger, F. (2001). SeaWiFS helps assess hurricane impact on phytoplankton in Caribbean Sea. EOS 82 (45), 529–533. doi:10.1029/01eo00314
Gómez-Gómez, F., Rodríguez-Martínez, J., and Santiago, M. (2014). Hydrogeology of Puerto Rico and the outlying islands of vieques, culebra, and mona. Available at: https://pubs.er.usgs.gov/publication/sim3296.
Green, R. H. (1979). Sampling design and statistical methods for environmental biologists. USA: John Wiley and Sons.
Halliday, E., and Gast, R. J. (2011). Bacteria in beach sands: an emerging challenge in protecting coastal water quality and bather health. Environ. Sci. Technol. 45 (2), 370–379. doi:10.1021/es102747s
Hernández, W. J., Ortiz-Rosa, S., Armstrong, R. A., Geiger, E. F., Eakin, C. M., and Warner, R. A. (2020). Quantifying the effects of hurricanes Irma and Maria on coastal water quality in Puerto Rico using moderate resolution satellite sensors. Remote Sens. 12, 964. doi:10.3390/rs12060964
Hernández Ayala, J. J., and Méndez Tejeda, R. (2023). Examining the spatiotemporal changes in the annual, seasonal, and daily rainfall climatology of Puerto Rico. Climate 11, 225. doi:10.3390/cli11110225
Hochberg, E. J., Peltier, S. A., and Maritorena, S. (2020). Trends and variability in spectral diffuse attenuation of coral reef waters. Coral Reefs 39, 1377–1389. doi:10.1007/s00338-020-01971-1
Hurlbert, S. H. (1984). Pseudoreplication and the design of ecological field experiments. Ecol. Monogr. 54 (2), 187–211. doi:10.2307/1942661
Jeppesen, R., Søndergaard, M., Jensen, J. P., Havens, K. E., Anneville, O., Carvalho, L., et al. (2005). Lake responses to reduced nutrient loading – an analysis of contemporary long-term data from 35 case studies. Freshw. Biol. 50, 1747–1771. doi:10.1111/j.1365-2427.2005.01415.x
Keellings, D., and Hernández Ayala, J. J. (2019). Extreme rainfall associated with Hurricane Maria over Puerto Rico and its connections to climate variability and change. Geophys. Res. Lett. 46, 2964–2973. doi:10.1029/2019GL082077
Khatri, N., and Tyagi, S. (2015). Influences of natural and anthropogenic factors on surface and groundwater quality in rural and urban areas. Front. Life Sci. 8 (1), 23–39. doi:10.1080/21553769.2014.933716
Lee, Z. P., Darecki, M., Carder, K. L., Davis, C. O., Stramski, D., and Rhea, W. J. (2005). Diffuse attenuation coefficient of downwelling irradiance: an evaluation of remote sensing methods. J. Geophys. Res. 110 (2), 1–9. doi:10.1029/2004JC002573
Li, J., Knapp, D. E., Lyons, M., Roelfsema, C., Phinn, S., Schill, S. R., et al. (2021). Automated global shallow water bathymetry mapping using google earth engine. Remote Sens. 13, 1469. doi:10.3390/rs13081469
Lin, L., Yang, H., and Xu, X. (2022). Effects of water pollution on human health and disease heterogeneity: a review. Front. Environ. Sci. 10. doi:10.3389/fenvs.2022.880246
Mallin, M. A., Williams, K. E., Esham, E. C., and Lowe, R. P. (2000). Effect of human development on bacteriological water quality in coastal watersheds. Ecol. Appl. 10 (4), 1047–1056. doi:10.1890/1051-0761(2000)010[1047:EOHDOB]2.0.CO;2
Miller, P. W., Kumar, A., Mote, T. L., Moraes, F. D. S., and Mishra, D. R. (2019). Persistent hydrological consequences of hurricane Maria in Puerto Rico. Geophys. Res. Lett. 46, 1413–1422. doi:10.1029/2018GL081591
Miller, R. L., and Cruise, J. F. (1995). Effects of suspended sediments on coral growth: evidence from remote sensing and hydrologic modeling. Remote Sens. Environ. 53 (3), 177–187. doi:10.1016/0034-4257(95)00081-B
Morais, P. (2008). Review on the major ecosystem impacts caused by damming and watershed development in an Iberian basin (SW-Europe): focus on the Guadiana estuary. Ann. Limnol. 44 (2), 105–117. doi:10.1051/limn:2008012
Morel, A., Huot, Y., Gentili, B., Werdell, P. J., Hooker, S. B., and Franz, B. A. (2007). Examining the consistency of products derived from various ocean color sensors in open ocean (Case 1) waters in the perspective of a multi-sensor approach. Remote Sens. Environ. 111, 69–88. doi:10.1016/j.rse.2007.03.012
Nalley, E. M., Tuttle, L. J., Barkman, A. L., Conklin, E. E., Wulstein, D. M., Richmond, R. H., et al. (2021). Water quality thresholds for coastal contaminant impacts on corals: a systematic review and meta-analysis. Sci. Total Environ. 794, 148632. doi:10.1016/j.scitotenv.2021.148632
Nieke, J., Mavrocordatos, C., Donlon, C., Berruti, B., Garnier, T., Riti, J.-B., et al. (2015). “Ocean and land color imager on sentinel-3,” in Optical payloads for Space missions. Editor S. E. Qian (Germany: Wiley). doi:10.1002/9781118945179.ch10
Ormaza-Gonzaìlez, F. I., Castro-Rodas, D., and Statham, P. J. (2021). COVID-19 impacts on beaches and coastal water pollution at selected sites in Ecuador, and management proposals post-pandemic. Front. Mar. Sci. 8. doi:10.3389/fmars.2021.669374
Ortiz-Rosa, S., Hernández, W. J., Williams, S. M., and Armstrong, R. A. (2020). Water quality anomalies following the 2017 hurricanes in southwestern Puerto Rico: absorption of colored detrital and dissolved material. Remote Sens. 12, 3596. doi:10.3390/rs12213596
Poortinga, A., Clinton, N., Saah, D., Cutter, P., Chishtie, F., Markert, K. N., et al. (2018). An operational before-after-control-impact (BACI) designed platform for vegetation monitoring at planetary scale. Remote Sens. 10 (5), 760. doi:10.3390/rs10050760
Richmond, R. H., Rongo, T., Golbuu, Y., Victor, S., Idechong, N., Davis, G., et al. (2007). Watersheds and coral reefs: conservation science, policy, and implementation. BioScience 57 (7), 598–607. doi:10.1641/B570710
Rogers, C. S., and Ramos-Scharrón, C. E. (2022). Assessing effects of sediment delivery to coral reefs: a caribbean watershed perspective. Front. Mar. Sci. 8. doi:10.3389/fmars.2021.773968
Sanger, D., Blair, A., DiDonato, G., Washburn, T., Jones, S., Riekerk, G., et al. (2013). Impacts of coastal development on the ecology of tidal creek ecosystems of the US southeast including consequences to humans. Estuaries Coasts 38 (1), 49–66. doi:10.1007/s12237-013-9635-y
Seger, K. D., Sousa-lima, R., Schmitter-Soto, J. J., and Urban, Jr. E. R. (2021). Editorial: before-after control-impact (BACI) studies in the ocean. Front. Mar. Sci. 8. doi:10.3389/fmars.2021.787959
Sidabutar, N. V., Namara, I., Hartono, D. M., and Soesilo, T. E. B. (2017). The effect of anthropogenic activities to the decrease of water quality. IOP Conf. Ser. Earth Environ. Sci. 67 (1), 012034. doi:10.1088/1755-1315/67/1/012034
Smith, A., Yee, S. H., Russell, M., Awkerman, J., and Fisher, W. S. (2017). Linking ecosystem service supply to stakeholder concerns on both land and sea: an example from Guánica Bay watershed, Puerto Rico. Ecol. Indic. 74, 371–383. doi:10.1016/j.ecolind.2016.11.036
Smokorowski, K. E., and Randall, R. G. (2017). Cautions on using the Before-After-Control- Impact design in environmental effects monitoring programs. Facets 2 (1), 212–232. doi:10.1139/facets-2016-0058
Stewart-Oaten, A., Murdoch, W. W., and Parker, K. R. (1986). Environmental impact assessment: “pseudoreplication” in time? Ecol. Soc. Am. 67 (4), 929–940. doi:10.2307/1939815
Takesue, R. K., Sherman, C., Ramirez, N. I., Reyes, A. O., Cheriton, O. M., Viqueira, R., et al. (2021). Land-based sediment sources and transport to southwest Puerto Rico coral reefs after Hurricane Maria, May 2017 to June 2018. Coast. Shelf Sci. 259 (June), 107476. doi:10.1016/j.ecss.2021.107476
Tavakoly Sany, S. B., Monazami, G., Rezayi, M., Tajfard, M., and Borgheipour, H. (2019). Application of water quality indices for evaluating water quality and anthropogenic impact assessment. Int. J. Environ. Sci. Technol. 16 (7), 3001–3012. doi:10.1007/s13762-018-1894-5
Tomlinson, M. C., Stumpf, R. P., and Vogel, R. L. (2019). Approximation of diffuse attenuation, Kd, for MODIS high-resolution bands. Remote Sens. Lett. 10 (2), 178–185. doi:10.1080/2150704X.2018.1536301
Underwood, A. J. (1994). On beyond BACI: sampling designs that might reliably detect environmental disturbances. Ecol. Soc. Am. 4 (1), 3–15. doi:10.2307/1942110
Vijay Prakash, K., Geetha Vimala, C. S., Preethi Latha, T., Jayaram, C., Nagamani, P. V., and Laxmi, C. N. V. (2021). Assessment of water quality along the southeast coast of India during COVID-19 lockdown. Front. Mar. Sci. 8. doi:10.3389/fmars.2021.659686
Viqueira Ríos, R. (2018). Implementation of the Guánica bay watershed management plan: integrated watershed management actions final report 2012-2017.
Viqueira Ríos, R. (2021). Implementation of the Guánica bay watershed management plan: integrated watershed management.
Viqueira Ríos, R., Meyer Comas, L., and Sturm, P. (2012). Lajas Valley agricultural reserve farm inventory of the Guánica Lagoon. El Anegado adjacent areas Guánica, P. R.
Wang, G., Mang, S., Cai, H., Liu, S., Zhang, Z., Wang, L., et al. (2016). Integrated watershed management: evolution, development and emerging trends. J. For. Res. 27, 967–994. doi:10.1007/s11676-016-0293-3
Wang, M., Son, S., and Jr, L. W. H. (2009). Retrieval of diffuse attenuation coefficient in the Chesapeake Bay and turbid ocean regions for satellite ocean color applications. J. Geophys. Res. 114 (July), 1–15. doi:10.1029/2009JC005286
Wang, Y., Lee, Z., Ondrusek, M., Li, X., Zhang, S., and Wu, J. (2022). An evaluation of remote sensing algorithms for the estimation of diffuse attenuation coefficients in the ultraviolet bands. Opt. Express 30 (5), 6640. doi:10.1364/oe.446114
Warne, A. G., Webb, R. M. T., and Larsen, M. C. (2005). “Water, sediment, and nutrient discharge characteristics of rivers in Puerto Rico, and their potential influence on coral reefs,” in Scientific investigations report 2005-5206.
Whitall, D., Bauer, L. J., Sherman, C., Edwards, K., Mason, A., Pait, T., et al. (2013). Baseline assessment of Guánica bay, Puerto Rico in support of watershed restoration. NOAA technical memorandum NOS NCCOS 176. Prepared by the NCCOS center for coastal monitoring and assessment biogeography Branch. Germany: Silver Spring, 169.
Keywords: Guánica Bay, beyond-BACI, OLCI, MERIS, ocean Color, remote sensing
Citation: Guzmán-Morales AL, Armstrong RA, Cruz-Motta JJ and Hernández WJ (2024) Spatial and temporal patterns of diffuse attenuation coefficient in Guánica, Puerto Rico: 15 years after development of a watershed management plan. Front. Remote Sens. 5:1404323. doi: 10.3389/frsen.2024.1404323
Received: 20 March 2024; Accepted: 30 May 2024;
Published: 19 June 2024.
Edited by:
Srinivas Kolluru, Florida Atlantic University, United StatesReviewed by:
Vassiliki Metheniti, Foundation for Research and Technology Hellas (FORTH), GreeceJessica S Turner, University of Connecticut, United States
Premkumar R., Indian National Centre for Ocean Information Services, India
Chandanlal Parida, Curtin University, Australia
Copyright © 2024 Guzmán-Morales, Armstrong, Cruz-Motta and Hernández. This is an open-access article distributed under the terms of the Creative Commons Attribution License (CC BY). The use, distribution or reproduction in other forums is permitted, provided the original author(s) and the copyright owner(s) are credited and that the original publication in this journal is cited, in accordance with accepted academic practice. No use, distribution or reproduction is permitted which does not comply with these terms.
*Correspondence: Alba L. Guzmán-Morales, alba.guzman2@upr.edu