- 1Royal Belgian Institute of Natural Sciences (RBINS), Brussels, Belgium
- 2National Physical Laboratory (NPL), Teddington, United Kingdom
- 3CNR-ISMAR, Istituto di Scienze Marine, Consiglio Nazionale delle Ricerche, Rome, Italy
- 4Instituto de Astronomía y Física del Espacio, Consejo Nacional de Investigaciones Científicas y Técnicas (IAFE, CONICET/UBA, University of Buenos Aires), Buenos Aires, Argentina
- 5Laboratoire d’Océanographie de Villefranche (LOV), Sorbonne Université/CNRS, Villefranche-sur-Mer, France
- 6European Space Agency (ESA), Frascati, Italy
- 7Tartu Observatory, University of Tartu (UT), Tartu, Estonia
- 8Deutsches GeoForschungsZentrum (GFZ), Potsdam, Germany
- 9University of Maryland Baltimore County (UMBC), Baltimore, MD, United States
Satellites are now routinely used for measuring water and land surface reflectance and hence environmentally relevant parameters such as aquatic chlorophyll a concentration and terrestrial vegetation indices. For each satellite mission, radiometric validation is needed at bottom of atmosphere for all spectral bands and covering all typical conditions where the satellite data will be used. Existing networks such as AERONET-OC for water and RadCalNet for land provide vital information for validation, but (AERONET-OC) do not cover all spectral bands or (RadCalNet) do not cover all surface types and viewing angles. In this Perspective Article we discuss recent advances in instrumentation, measurement methods and uncertainty estimation in the field of optical radiometry and put forward the viewpoint that a new network of automated hyperspectral radiometers is needed for multi-mission radiometric validation of water and land surface reflectance. The HYPERNETS federated network concept is described, providing a context for research papers on specific aspects of the network. This network is unique in its common approach to both land and water surfaces. The common aspects and the differences between land and water measurements are explained. Based on early enthusiasm for HYPERNETS data from validation-oriented workshops, it is our viewpoint that this new network of automated hyperspectral radiometers will be useful for multi-mission radiometric validation of water and multi-angle land surface reflectance. The HYPERNETS network has strong synergy with other measurement networks (AERONET, AERONET-OC, RadCalNet, FLUXNET, ICOS, skycam, etc.) and with optional supplementary measurements, e.g., water turbidity and fluorescence, land surface temperature and soil moisture, etc.
1 Introduction
1.1 Motivation
Satellites are now routinely used for measuring water and land surface reflectance and hence parameters such as aquatic chlorophyll a concentration and terrestrial vegetation indices. Since these remotely sensed parameters may be significantly affected by errors in top of atmosphere calibration and atmospheric correction, radiometric validation at the bottom of atmosphere is needed for quality control, ensuring that data reaching end-users are of known quality and that quality issues are reported to satellite data providers for improvement.
For each satellite mission, radiometric validation is needed for all spectral bands and covering all typical conditions where the satellite data will be used, including various water/land types, sun angles, atmospheric conditions (aerosols, absorbing gases, scattered and semi-transparent clouds), surface altitudes, spatial heterogeneity, etc.
1.2 User needs
The users of in situ measurements of water and land surface reflectance are identified as:
• Satellite operators, including international and national space agencies and “Newspace” commercial data providers—see Supplementary Material S1 for a list of target missions.
• Developers of atmospheric correction algorithms and software.
• Environmental agencies and scientists using in situ measurements to monitor water or land surface properties.
The user requirements for in situ measurements of water and land surface reflectance are identified as (Goyens et al., 2018):
• Hyperspectral coverage 380–1020 nm for water and minimally 380–1700 nm but preferably 380–2500 nm for land.
• Spectral resolution of 5 nm Full Width at Half Maximum (FWHM) for 380–1020 nm and 10 nm FWHM for 1020–1700 nm.
• Measurements every 30 min during daylight.
• Data provided publicly in Near Real Time (e.g., <1 day) by web service.
• Fiducial Reference Measurement (FRM) quality, including full estimate of uncertainty.
• Full nadir/azimuth coverage up to 60° nadir for land surface reflectance.
In addition to the reflectance measurements, users request pictures from cameras and information on aerosols and direct/diffuse irradiance ratio.
Atmospheric applications are specifically excluded here because aerosol parameters are already well-measured by AERONET (Holben et al., 1998) and because measurement of absorbing atmospheric gases (Verhoelst et al., 2021) generally requires much finer spectral resolution, e.g., sub-nanometre.
1.3 Precursor networks
The user needs of Section 1.2 are partially met by the existing networks, AERONET-OC for water and RadCalNet for land.
The AERONET-OC 12 band instrument (Zibordi et al., 2021) matches well the spectral bands of “ocean colour” sensors, but less well the wide bands of “land” sensors such as Sentinel-2/MSI, Landsat-8/9, Planet Superdoves, etc.—see Figure 1. Validation of the new generation of hyperspectral sensors by multispectral ground measurements is also insufficient (Giardino et al., 2020; Braga et al., 2022). While aerosol correction can be assessed by a limited set of multispectral bands, the new potential of hyperspectral satellite data, e.g., spectral curvature/derivative algorithms (Dierssen et al., 2020; Lavigne et al., 2022), will require hyperspectral in situ data.
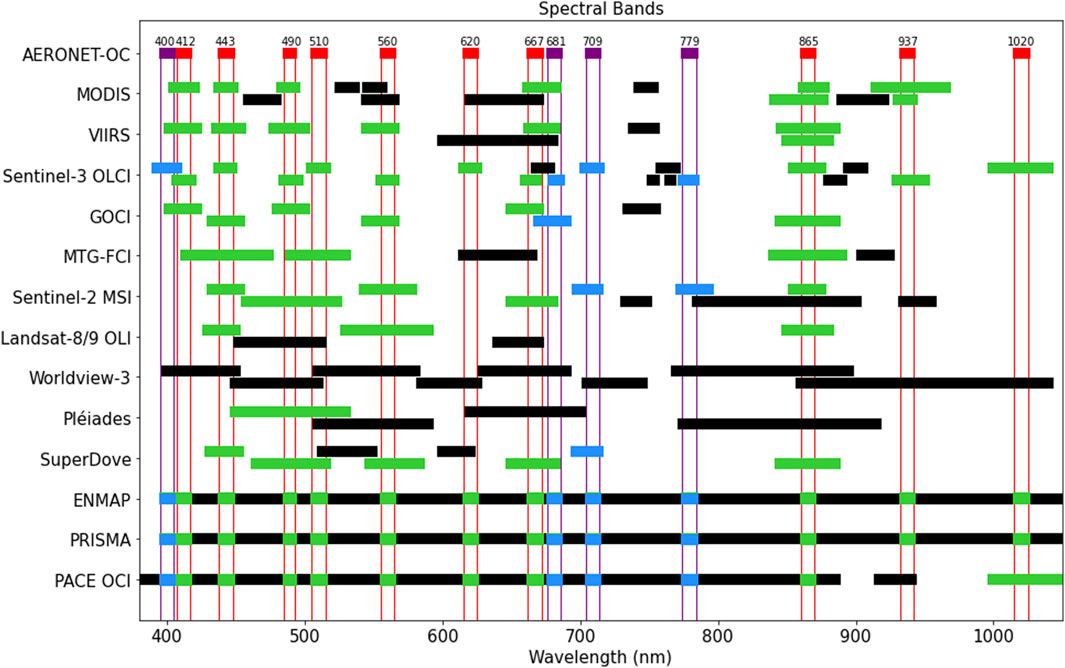
Figure 1. Spectral bands of typical satellite missions to be validated compared with (top row) the CIMEL CE318TV-12 used in AERONET-OC. The two versions of the CE318TV-12 instrument are displayed with common bands in red and optional bands in violet. The variant CE318TV-12-LC (“lake colour”) has bands 681 nm and 709 nm, while the CE318TV-12-OC (“ocean colour”) variant CE318TV-12-OC has bands 400 nm and 779 nm. The CE318-TU12 instrument used for multispectral land surface reflectance measurements (Meygret et al., 2011) has a different set of 9 or 12 spectral bands.1 Satellite bands with central wavelength falling inside a CE318TV-12 common/optional band are shown in green/blue respectively and those falling outside such bands are shown in black.
RadCalNet (Bouvet et al., 2019) is designed to provide hyperspectral radiance at top of atmosphere for the purpose of satellite vicarious (in-flight) calibration. RadCalNet sites are located in optimal locations with horizontal homogeneity of the surface and clear, stable atmosphere and do not cover the more difficult surfaces required for a complete sensor validation plan. Some sites do not use hyperspectral instruments. RadCalNet provides only nadir-viewing data.
While both AERONET-OC and RadCalNet must continue to provide vital data for calibration and validation of satellite missions, it is our viewpoint that a new in situ measurement network is needed to fully satisfy the user requirements for multi-mission water and land surface reflectance validation. This was the motivation for setting up HYPERNETS, a federated network of sites running autonomous hyperspectral radiometer systems to provide validation data at all spectral bands in the range 380–1700 nm for all satellite missions with a surface reflectance product.
1.4 The difference between land and water
The land and water optical remote sensing communities have traditionally operated very separately with different data processing chains, atmospheric correction algorithms, etc. This separation is driven by the different user groups and data products, and by different physical processes, particularly those relevant for atmospheric correction.
Water is generally much darker than land, exacerbating challenges for aerosol correction, adjacency effect correction, vicarious calibration, absorbing aerosol detection/correction, etc. Removal of air-water interface reflection is an additional challenge, particularly as regards sunglint removal for near nadir-viewing sensors. However, there are algorithms that can apply over both water and land surfaces, e.g., iCOR (Keukelaere et al., 2018) and ACOLITE/DSF (Vanhellemont, 2019).
Land surfaces may have higher spatial heterogeneity at short length scales (<30 m) and high angular but low temporal variability of upwelling radiance.
Despite these differences, the HYPERNETS consortium decided to develop instrumentation and processing for both water and land surfaces, benefitting from the economy of scale for radiometer, host system and processor development and offering a larger customer base for the new radiometer. The commonality in data processing and distribution for the land and water network will also facilitate validation of land and water surface reflectance simultaneously and open up new opportunities to study complex interactions between water and land environments.
1.5 Scope and overview of this Perspective Article
In this Perspective Article we discuss recent advances in optical radiometry and describe the HYPERNETS federated network concept (Figure 2). HYPERNETS integrates two branches for the different surface types: WATERHYPERNET (Ruddick et al., 2024) and LANDHYPERNET (De Vis et al., 2024a). Both branches use the newly developed HYPSTAR® radiometer system, which is the main focus of this article. The PANTHYR/TriOS radiometer system (Vansteenwegen et al., 2019) is also included in WATERHYPERNET and in the summary of results of Section 3.
2 Optical radiometry state of the art and HYPERNETS network design
2.1 Measurement method
For water reflectance measurements, automated above water radiometry (Zibordi et al., 2009) has proven to be cost-effective. HYPERNETS adopts the same approach as AERONET-OC for measurement of water-leaving radiance,
For land surface reflectance measurements, protocols for automated radiometry are less mature. Most measurements are supervised and use a reflectance based method with a reflectance standard as a reference (Slater et al., 1987). An autonomous multiangle surface reflectance protocol was developed by Meygret et al. (2011) for measurement of Hemispherical Conical Reflectance Factor (HCRF) with a multispectral instrument. This protocol could not be implemented by HYPERNETS due to the hyperspectral measurement time, power and data transfer requirements. HYPERNETS developed a sequential acquisition protocol (De Vis et al., 2024b), measuring
While the basic water and land reflectance measurement scenarios are sufficient, complementary measurements can be added, e.g., sunglint pointing for estimation of wave slope statistics (Goyens and Ruddick, 2023); direct sun radiance measurement, potentially supplemented with various sky radiance measurements, for comparison with the direct
2.2 Hyperspectral radiometers
The user requirements of Section 1.2, particularly the spectral requirements and the need for a pointable radiometer could not be met by any Commercial Off The Shelf (COTS) radiometer—see Kuusk et al. (2024). The HYPERNETS consortium therefore designed a new hyperspectral radiometer, the HYPSTAR®. This innovative design (Kuusk et al., 2024) is based on spectrometers covering 380–1020 nm at 3 nm FWHM with 0.5 nm sampling interval and (land units only) 1020–1680 nm at 10 nm FWHM and 3 nm sampling interval. The spectrometers are multiplexed between alternative optical paths, one for radiance and one for irradiance, reducing cost and minimising impact of thermal sensitivity and some absolute calibration uncertainties on reflectance products. One innovative feature of the HYPSTAR® radiometer design is the integration of an external LED source, which can be used at night for monitoring the long term stability of radiometric calibration. Another useful feature, first suggested in the OSPREY design (Hooker et al., 2012), is an embedded RGB camera. This camera has proven extremely useful for troubleshooting equipment failures and strange radiometric data—at some sites it is not unusual to see a bird sitting on the radiometer, partially obscuring the field of view!
2.3 Autonomous system
The HYPSTAR® system integrates the radiometer with a pointing system, a PC, a power source and data transmission hardware and associated software/firmware (Doxaran et al., 2024; Kuusk et al., 2024).
For autonomous systems deployed with minimal maintenance requirements, and in potentially hostile environments (hot, cold, wet, salty), remote connection capabilities should be ensured and all components must be selected for reliability. HYPSTAR® has only two moving parts, the radiometer multiplexer and the pointing system.
Over the last decade, technological improvements and mass COTS production have been significant for: pointing systems (now ubiquitous for security cameras), data transmission (4G), photovoltaic power and solid state drives.
The HYPSTAR® system (Kuusk et al., 2024), integrates a Will-Burt Bowler RX pointing system with a Cincoze rugged PC and a custom-made board integrating relays for efficient power management. Components were selected for an operating temperature range of −25°C to +45°C and housings are rated with Ingress Protection IP66, IP67 or IP68.
Power supply is site-specific, preferably grid power, but otherwise photovoltaic or wind power with associated battery and controller. Data transmission is generally by cellular or cabled internet, but may be manual for some locations, e.g., Gobabeb and Antarctica.
System components are attached to mounting structures, which vary from large platforms to standalone masts. The choice of platform and mounting location is important for data quality and should be made to limit optical perturbations of the radiometric targets by the structure and maximise acceptable viewing angles—see Section 3.3 of Ruddick et al. (2024).
2.4 Validation sites
A validation network should include sites covering a wide range of surface types and atmospheric conditions, in particular including the “difficult cases” where atmospheric correction may fail or produce poor results.
Water sites should cover a range of turbidity, phytoplankton biomass and species, Coloured Dissolved Organic Matter absorption, sun zenith angle, clouds, aerosols, etc. Sites at high altitude and with strong adjacency effects are also needed.
Land sites should cover a range of substrates and vegetation including non-vegetated, snow/ice, grassland, agricultural with various crops and practices, forest with various species, age and canopy cover, etc.
When comparing satellite and matchup in situ measurements it is necessary to consider additional uncertainties associated with the different wavelength, space, time and angular coordinates.
A key question for each validation site is the spatial heterogeneity. Each validation site should be characterised in terms of spatial variability to give the additional uncertainty involved in a matchup comparison as function of length scale, e.g., Dogliotti et al. (2015), Dogliotti et al. (2024), Doxaran et al. (2024) for water and Morris et al. (2024) for land.
Temporal variability at a validation site, also of importance in satellite/in situ matchups, can be characterised from time series of the in situ radiometer measurements (Doxaran et al., 2024).
For land sites, the angular variability of upwelling radiance is very high for many surface types, particularly for vegetated surfaces and shadowing surfaces. The BRDF needs to be modelled for each site (Schunke et al., 2023), using measurements at multiple sun and viewing zenith and azimuth angles. The separation of spatial and angular variability may be complex, but should be feasible if data are collected over sufficient time and for multiple sun and viewing angles.
The list of currently operated HYPERNETS validation sites is provided in Supplementary Material S2.
2.5 Data processing and quality control
Network data processing and quality control (De Vis et al., 2024a) must be centralised and automated to ensure efficient operations and reliability of data provided to users. Measurements are acquired at the validation sites every 15–30 min during daylight. Raw data and metadata are transmitted to computer servers, one for water and one for land processing.
Identical processing is applied for water and land sites up to the level of calibrated radiances and irradiances with associated uncertainties. The derivation of water and land surface reflectance then uses different measurement functions, but within a common software framework. This integration of land and water processing facilitates joint use of quality control tests, e.g., comparison of
2.6 Uncertainty estimation
An important feature of the HYPERNETS processor, of relevance to Fiducial Reference Measurements (Donlon and Zibordi, 2014; Goryl et al., 2023), is the propagation of measurement uncertainties from their sources to the final reflectance data using a Monte Carlo approach (International Standards Organisation ISO, 2008). The implementation, using the open source CoMet toolkit,2 preserves temporal and spectral uncertainty covariance. The covariance information (De Vis et al., 2024b) provided with the output reflectance ensures that the uncertainty of downstream products, such as Normalised Difference Vegetation Index (NDVI) or aquatic chlorophyll a concentration, can be correctly evaluated.
2.7 Data distribution and network user support
For optimal user uptake, data from the network will be publicly distributed through an interactive user interface, and an Application Programming Interface (API). Data are provided in NetCDF format with relevant metadata, following the INSPIRE directive, and are covered by a CC-BY-ND open data licence.
HYPERNETS provides support for both site operators and data users. Site operators receive installation and troubleshooting advice, share experiences on site operations and data exploitation in scientific discussions and receive radiometer calibration and characterisation services.
A common request from space agencies is for a list of validation site coordinates to be used in prioritising acquisitions, e.g., during a commissioning phase. The coordinates of HYPERNETS sites are provided in Supplementary Material S2.
3 Summary of demonstration results
In Supplementary Material S4, findings from exploitation of early prototype HYPERNETS data can be found. This includes both the new HYPSTAR® system and the PANTHYR system (Vansteenwegen et al., 2019), which is based on the mature COTS TriOS/RAMSES radiometer.
So far, PANTHYR (Vanhellemont, 2020; Vanhellemont and Ruddick, 2021; Braga et al., 2022; Vanhellemont, 2023; Ruddick et al., 2024) and HYPSTAR® (Dogliotti et al., 2024; Doxaran et al., 2024; Gonzalez Vilas et al., 2024; Ruddick et al., 2024) data have been used for validation of water reflectance from Landsat-8&9/OLI, Sentinel-2/MSI, Sentinel-3/OLCI, PRISMA, Aqua/MODIS, SNPP&JPSS1/VIIRS, and the constellation of PlanetScope Dove and Superdove satellite data, generally comparing different atmospheric correction algorithms.
HYPSTAR® data have been used for validation of land surface reflectance from Sentinel-2/MSI and Landsat-8&9/OLI for a deciduous forest site (Morris et al., 2024).
HYPERNETS data have also been used for monitoring of water quality and phytoplankton species (Goyens et al., 2022; Ruddick et al., 2024).
Finally, the HYPERNETS instrument system and data processing is also relevant for vicarious calibration of satellites at top of atmosphere if the deployment site is sufficiently spatially homogeneous and well-characterised. E.g., De Vis et al. (2024a) demonstrates use of the HYPSTAR® at Gobabeb (Namibia) and the Princess Elisabeth Antarctica base for vicarious calibration of Sentinel-2, Landsat-9 and PRISMA. The HYPSTAR®/Gobabeb site (Sinclair et al., 2023) has been submitted to the official application process to become a RadCalNet site.
4 Discussion
We suggest that a new network of automated hyperspectral radiometers is needed for multi-mission radiometric validation of water and land surface reflectance. The corresponding HYPERNETS federated network, now at the stage of demonstrated prototype, has been described.
A new hyperspectral radiometer has been designed, manufactured and tested. This radiometer provides high quality data according to user needs and will be commercialised by the new company RSware OÜ (https://hypstar.eu).
A host system, built from both COTS and customised components has been assembled and tested with improvements in reliability (uptime) over the successive prototype versions.
The new hyperspectral radiometer and associated host system have been deployed at 22 sites, of which 10 water and 8 land sites are expected to continue in 2024. The network is expected to expand slowly in 2024–26 by addition of new international partners.
Data are transmitted from validation sites to central servers, and are processed daily using automated routines. Data distribution will be public from web data portals. Pending completion of these data portals, prototype “beta-release” datasets have been publicly distributed via www.zenodo.org—see Supplementary Material S5.
Measurement uncertainties are propagated in data processing using Monte Carlo modelling. The current status includes uncertainties from replicate noise and radiometric calibration. Measurement uncertainties from the following processes are in preparation for future implementation: a) radiometer thermal sensitivity, straylight and angular response, b) mounting platform optical perturbations (shading/reflection) and, for water, c) air-water interface reflected light. Additional uncertainties relating to space and time differences when using HYPERNETS data for satellite validation are also being addressed (Dogliotti et al., 2024; Doxaran et al., 2024; Morris et al., 2024).
While HYPERNETS is designed as a standalone single measurand network there is a strong interest in collaboration with other networks. Some HYPERNETS sites are already co-located with sites from the AERONET, AERONET-OC, RadCalNet, FLUXNET, IR-ILICO, ICOS, ROMA3 and skycam networks, and co-location of one or more sites with the PANDONIA4 network is foreseen. The synergy between co-located networks provides both economic and scientific benefits: site infrastructure and maintenance visits can be combined for multiple instruments providing an economy of scale; data from HYPERNETS can be intercompared directly with similar measurands using different methods/instruments from other networks, e.g., surface reflectance from AERONET-OC and RadCalNet; HYPERNETS could benefit from information on aerosol properties from AERONET and atmospheric absorbing gases from PANDONIA4. HYPERNETS sites can be supplemented by other measurements, e.g., water turbidity and fluorescence, land surface temperature and soil moisture, etc.
Based on early enthusiasm from data users, HYPERNETS seems useful for radiometric validation of water and multi-angle land surface reflectance. The cost effectiveness of the approach is clearly demonstrated, e.g., validation of Landsat-8&9/OLI, Sentinel-2/MSI, Sentinel-3/OLCI, the PlanetScope/SuperDoves constellation, SNPP&JPSS1/VIIRS, AQUA/MODIS and PRISMA satellite data at the La Plata Estuary site (Dogliotti et al., 2024). Exploitation of HYPERNETS data for other applications, e.g., satellite vicarious calibration (De Vis et al., 2024b) and water quality monitoring (Goyens et al., 2022), is also promising.
Data availability statement
The original contributions presented in the study are included in the article/Supplementary Material, further inquiries can be directed to the corresponding author.
Author contributions
KR: Conceptualization, Funding acquisition, Methodology, Project administration, Writing–original draft, Writing–review and editing. AB: Conceptualization, Funding acquisition, Methodology, Project administration, Writing–review and editing. VB: Conceptualization, Funding acquisition, Methodology, Project administration, Writing–review and editing. PD: Conceptualization, Methodology, Writing–review and editing. AD: Conceptualization, Funding acquisition, Methodology, Project administration, Writing–review and editing. DD: Conceptualization, Funding acquisition, Methodology, Project administration, Writing–review and editing. PG: Conceptualization, Funding acquisition, Methodology, Project administration, Writing–review and editing. CG: Conceptualization, Funding acquisition, Methodology, Project administration, Writing–review and editing. JK: Conceptualization, Funding acquisition, Methodology, Project administration, Writing–review and editing. DS: Funding acquisition, Methodology, Project administration, Writing–review and editing. KT: Funding acquisition, Methodology, Project administration, Writing–review and editing. QV: Conceptualization, Methodology, Writing–review and editing.
Funding
The authors declare that financial support was received for the research, authorship, and/or publication of this article. This work was carried out in the context of: the H2020/HYPERNETS project funded by the European Union’s Horizon 2020 research and innovation programme (Grant agreement n◦ 775983), the HYPERNET-POP project funded by the European Space Agency (contract n◦ 4000139081/22/I-EF), by the PICT-2020/2636 project funded by the ANPCyT, and by NASA grant 80NSSC24K0479 in support of the Surface Biology and Geology mission development and a NASA grant in support of Plankton, Aerosol, Cloud, ocean Ecosystem (PACE) Mission Validation.
Acknowledgments
Over the last 6 years we have benefited from input, discussions and support from many colleagues. We thank all colleagues from the H2020/HYPERNETS and FRM4SOC projects as well as external experts and project advisers including particularly: Giuseppe Zibordi, Nigel Fox, Craig Donlon, Tania Casal, Erwin Goor, Miroslaw Darecki, Ewa Kwiatkowska, Juan Gossn, Kevin Alonso Gonzalez, Fabrizio Niro and Dimitry Van der Zande. Acknowledgement of these individuals does not constitute endorsement of or responsibility for the content of this work but is merely an expression of thanks for their suggestions and support. The Escuela Naval Militar (EsNM) and Servicio de Hidrografia Naval (SHN) are thanked for permission and access to the LPAR platform and installation support.
Conflict of interest
Co-authors from University of Tartu and Sorbonne University may benefit from future commercialisation of the HYPSTAR® radiometer and system. One author (PG) represents a funding agency. All other authors are likely to receive future research funding for HYPERNETS-related data acquisition and/or exploitation. The authors declare that these interests do not affect the statements and findings of this paper, which has been written according to the normal scientific ethics and values of the academic community.
The remaining authors declare that the research was conducted in the absence of any commercial or financial relationships that could be construed as a potential conflict of interest.
The authors declared that they were an editorial board member of Frontiers, at the time of submission. This had no impact on the peer review process and the final decision.
Publisher’s note
All claims expressed in this article are solely those of the authors and do not necessarily represent those of their affiliated organizations, or those of the publisher, the editors and the reviewers. Any product that may be evaluated in this article, or claim that may be made by its manufacturer, is not guaranteed or endorsed by the publisher.
Supplementary material
The Supplementary Material for this article can be found online at: https://www.frontiersin.org/articles/10.3389/frsen.2024.1372085/full#supplementary-material
Footnotes
1https://www.cimel.fr/solutions/ce318-t/#specifications
2https://www.comet-toolkit.org/
3https://aeronet.gsfc.nasa.gov/; https://aeronet.gsfc.nasa.gov/new_web/ocean_color.html; https://ceos.org/home-2/wgcv-radcalnet/; https://fluxnet.org/; https://www.ir-ilico.fr/; https://www.icos-cp.eu/; https://roma.conicet.gov.ar/
4https://www.pandonia-global-network.org/
References
Bouvet, M., Thome, K., Berthelot, B., Bialek, A., Czapla-Myers, J., Fox, N. P., et al. (2019). RadCalNet: a radiometric calibration network for earth observing imagers operating in the visible to shortwave infrared spectral range. Remote Sens. 11 (20), 2401. doi:10.3390/rs11202401
Braga, F., Fabbretto, A., Vanhellemont, Q., Bresciani, M., Giardino, C., Scarpa, G. M., et al. (2022). Assessment of PRISMA water reflectance using autonomous hyperspectral radiometry. ISPRS J. Photogrammetry Remote Sens. 192, 99–114. doi:10.1016/j.isprsjprs.2022.08.009
De Vis, P., Goyens, C., Hunt, S., Vanhellemont, Q., Ruddick, K. G., and Bialek, A. (2024a). Generating hyperspectral reference measurements for surface reflectance from the LANDHYPERNET and WATERHYPERNET networks. Front. Remote Sens. doi:10.3389/frsen.2024.1347230
De Vis, P., Howes, A., Vanhellemont, Q., Bialek, A., Morris, H., Sinclair, M., et al. (2024b). Feasibility of satellite vicarious calibration using HYPERNETS surface reflectances from Gobabeb and princess Elisabeth Antarctica sites. Front. Remote Sens. (submitted).
Dierssen, H., Bracher, A., Brando, V., Loisel, H., and Ruddick, K. (2020). Data needs for hyperspectral detection of algal diversity across the globe. Oceanography 33 (1), 74–79. doi:10.5670/oceanog.2020.111
Dogliotti, A. I., Gossn, J., Vanhellemont, Q., and Ruddick, K. G. (2015). Evaluation of two sites for ocean color validation in the Turbid waters of the rio de La Plata (Argentina) (2015) Proceedings of the Sentinel-3 for Science Workshop held in Venice-Lido, Italy, 2-5 June 2015, ESA Special Publication SP-734, Paris, France.
Dogliotti, A. I., Piegari, E., Rubinstein, L., Perna, P., and Ruddick, K. G. (2024). Using the automated HYPERNETS hyperspectral system for multimission Satellite Ocean Colour validation in the Río de La Plata, accounting for different spatial resolutions. Front. Remote Sens. doi:10.3389/frsen.2024.1354662
Donlon, C. J., and Zibordi, G. (2014). “In situ optical radiometry,” in Optical radiometry for ocean climate measurements (Amsterdam, Netherlands: Elsevier), 245–246.
Doxaran, D., Elkilani, B., Corizzi, A., and Goyens, C. (2024). Validation of satellite-derived water-leaving reflectance in contrasted French coastal waters based on HYPERNETS field measurements. Front. Remote Sens. 4, 1290110. doi:10.3389/frsen.2023.1290110
Giardino, C., Bresciani, M., Braga, F., Fabbretto, A., Ghirardi, N., Pepe, M., et al. (2020). First evaluation of PRISMA level 1 data for water applications. Sensors 20, 4553. doi:10.3390/s20164553
Gonzalez Vilas, L., Brando, V. E., Concha, J. A., Goyens, C., Dogliotti, A. I., Doxaran, D., et al. (2024). Validation of satellite water products based on HYPERNETS in situ data using a match-up Database file (MDB) structure. Front. Remote Sens. 5, 1330317. doi:10.3389/frsen.2024.1330317
Goryl, P., Fox, N., Donlon, C., and Castracane, P. (2023). Fiducial reference measurements (FRMs): what are they? Remote Sens. 15 (20), 5017. doi:10.3390/rs15205017
Goyens, C., Lavigne, H., Dille, A., and Vervaeren, H. (2022). Using hyperspectral remote sensing to monitor water quality in drinking water reservoirs. Remote Sens. 14 (21), 5607. doi:10.3390/rs14215607
Goyens, C., and Ruddick, K. (2023). Improving the standard protocol for above-water reflectance measurements: 1. Estimating effective wind speed from angular variation of sunglint. Appl. Opt. 62, 2442. doi:10.1364/AO.481787
Goyens, C., Ruddick, K., and Kuusk, J. (2018). “Spectral requirements for the development of a new hyperspectral radiometer integrated in automated networks - the HYPERNETS sensor,” in 2018 9th Workshop on Hyperspectral Image and Signal Processing: Evolution in Remote Sensing (WHISPERS), Amsterdam, Netherlands, September, 2018, 1–5. doi:10.1109/WHISPERS.2018.8747259
Holben, B. N., Eck, T. F., Slutsker, I., Tanré, D., Buis, J. P., Setzer, A., et al. (1998). AERONET—a federated instrument network and data archive for aerosol characterization. Remote Sens. Environ. 66 (1), 1–16. doi:10.1016/S0034-4257(98)00031-5
Hooker, S. B., Bernhard, G., Morrow, J. H., Booth, C. R., Comer, T., Lind, R. N., et al. (2012). “Optical sensors for planetary radiant energy (OSPREY): calibration and validation of current and next-generation NASA missions,” in NASA technical memorandum 2012–215872 (Greenbelt, MD, USA: NASA).
International Standards Organisation (ISO) (2008). Evaluation of measurement data - guide to the expression of uncertainty in measurement. JCGM 100:2008. Geneva, Switzerland: International Standards Organisation ISO.
Keukelaere, L. D., Sterckx, S., Adriaensen, S., Knaeps, E., Reusen, I., Giardino, C., et al. (2018). Atmospheric correction of Landsat-8/OLI and Sentinel-2/MSI data using iCOR algorithm: validation for coastal and inland waters. Eur. J. Remote Sens. 51 (1), 525–542. doi:10.1080/22797254.2018.1457937
Kuusk, J., Corizzi, A., Doxaran, D., Duong, K., Flight, K., Kivastik, J., et al. (2024). HYPSTAR: a hyperspectral pointable system for terrestrial and aquatic radiometry. Front. Remote Sens. (submitted).
Lavigne, H., Ruddick, K., and Vanhellemont, Q. (2022). Monitoring of high biomass Phaeocystis globosa blooms in the Southern North Sea by in situ and future spaceborne hyperspectral radiometry. Remote Sens. Environ. 282, 113270. doi:10.1016/j.rse.2022.113270
Meygret, A. R., Santer, P., and Berthelot, B. (2011). ROSAS: a robotic station for atmosphere and surface characterization dedicated to on-orbit calibration. in Proceedings of SPIE - the international society for optical engineering (San Diego, California, USA: SPIE). doi:10.1117/12.892759
Morris, H., Sinclair, M., De Vis, P., and Białek, A. (2024). Utilising LANDHYPERNETS data products over a deciduous broadleaf forest to validate Sentinel-2 and Landsat surface reflectance products. Front. Remote Sens., doi:10.3389/frsen.2024.1322760
Ruddick, K. G., Brando, V. E., Corizzi, A., Dogliotti, A. I., Doxaran, D., Goyens, C., et al. (2024). WATERHYPERNET: a prototype network of automated in situ measurements of hyperspectral water reflectance for satellite validation and water quality monitoring. Front. Remote Sens. (submitted).
Schunke, S., Leroy, V., and Govaerts, Y. (2023). Retrieving BRDFs from UAV-based radiometers for fiducial reference measurements: caveats and recommendations. Front. Remote Sens. 4. doi:10.3389/frsen.2023.1285800
Sinclair, M., Bialek, A., Vis, P. D., and Bouvet, M. (2023). “HYPERNETS land network: HYPSTAR®-XR deployment and validation in Namibia, Africa,” in IGARSS 2023 - 2023 IEEE International Geoscience and Remote Sensing Symposium, Pasadena, CA, USA, July, 2023, 4625–4627. doi:10.1109/IGARSS52108.2023.10282079
Slater, P. N., Biggar, S. F., Holm, R. G., Jackson, R. D., Mao, Y., Moran, M. S., et al. (1987). Reflectance- and radiance-based methods for the in-flight absolute calibration of multispectral sensors. Remote Sens. Environ. 22 (1), 11–37. doi:10.1016/0034-4257(87)90026-5
Vanhellemont, Q. (2019). Adaptation of the Dark Spectrum fitting atmospheric correction for aquatic applications of the Landsat and sentinel-2 archives. Remote Sens. Environ. 225, 175–192. doi:10.1016/j.rse.2019.03.010
Vanhellemont, Q. (2020). Sensitivity Analysis of the Dark Spectrum fitting atmospheric correction for metre- and decametre-scale satellite imagery using autonomous hyperspectral radiometry. Opt. Express 28 (20), 29948–29965. doi:10.1364/OE.397456
Vanhellemont, Q. (2023). Evaluation of eight band SuperDove imagery for aquatic applications. Opt. Express 31 (9), 13851–13874. doi:10.1364/OE.483418
Vanhellemont, Q., and Ruddick, K. (2021). Atmospheric correction of Sentinel-3/OLCI data for mapping of suspended particulate matter and chlorophyll-a concentration in Belgian turbid coastal waters. Remote Sens. Environ. 256, 112284. doi:10.1016/j.rse.2021.112284
Vansteenwegen, D., Ruddick, K., Cattrijsse, A., Vanhellemont, Q., and Beck, M. (2019). The pan-and-tilt hyperspectral radiometer system (PANTHYR) for autonomous satellite validation measurements – prototype design and testing. Remote Sens. 11 (11), 1360. doi:10.3390/rs11111360
Verhoelst, T., Compernolle, S., Pinardi, G., Lambert, J.-C., Eskes, H. J., Eichmann, K.-U., et al. (2021). Ground-based validation of the Copernicus Sentinel-5P TROPOMI NO2 measurements with the NDACC ZSL-DOAS, MAX-DOAS and pandonia global networks. Atmos. Meas. Tech. 14 (1), 481–510. doi:10.5194/amt-14-481-2021
Zibordi, G., Holben, B., Slutsker, I., Giles, D., D’Alimonte, D., Mélin, F., et al. (2009). AERONET-OC: a network for the validation of ocean color primary products. J. Atmos. Ocean. Technol. 26, 1634–1651. doi:10.1175/2009jtecho654.1
Keywords: satellite validation, hyperspectral reflectance, in situ measurements, automated network, radiometry
Citation: Ruddick KG, Bialek A, Brando VE, De Vis P, Dogliotti AI, Doxaran D, Goryl P, Goyens C, Kuusk J, Spengler D, Turpie KR and Vanhellemont Q (2024) HYPERNETS: a network of automated hyperspectral radiometers to validate water and land surface reflectance (380–1680 nm) from all satellite missions. Front. Remote Sens. 5:1372085. doi: 10.3389/frsen.2024.1372085
Received: 17 January 2024; Accepted: 14 March 2024;
Published: 10 April 2024.
Edited by:
Zhigang Cao, Chinese Academy of Sciences (CAS), ChinaReviewed by:
Joseph L. Garrett, NTNU, NorwayShun Bi, Helmholtz Association of German Research Centres (HZ), Germany
Copyright © 2024 Ruddick, Bialek, Brando, De Vis, Dogliotti, Doxaran, Goryl, Goyens, Kuusk, Spengler, Turpie and Vanhellemont. This is an open-access article distributed under the terms of the Creative Commons Attribution License (CC BY). The use, distribution or reproduction in other forums is permitted, provided the original author(s) and the copyright owner(s) are credited and that the original publication in this journal is cited, in accordance with accepted academic practice. No use, distribution or reproduction is permitted which does not comply with these terms.
*Correspondence: Kevin G. Ruddick, a3J1ZGRpY2tAbmF0dXJhbHNjaWVuY2VzLmJl