- 1School of Architecture, Urban Planning and Construction Engineering (AUIC), Politecnico di Milano, Milan, Italy
- 2Department of Built Environment, Aalto University, Otakaari, Finland
- 3Department of Architecture, Built Environment and Construction Engineering (ABC), Politecnico di Milano, Milan, Italy
In Europe, buildings account for 40% of the energy consumption and produce 36% of CO2 emissions. Renovation could be a great tool to decarbonize the building stock since it allows for a decrease in the operational energy required for buildings and is less material-consuming than new construction. Further benefits are brought by the usage of bio-based insulation materials that can drastically reduce embodied emissions and transform structures into factual carbon sinks. This study focuses on a particular kind of biogenic material, mycelium-wood composites, consisting of organic matter bound by the root structure of fungal organisms. This innovative insulation material was compared with traditional ones for the renovation of the building stock, with a focus on vertical components like walls in the Helsinki metropolitan area. To characterize mycelium-wood composites, density and carbon content information were gathered from the samples realized in the Politecnico di Milano MaBa.SAPERLab, while the production processes were included in a SimaPro model to obtain the GWP value. Different scenarios were then defined by two variables: the renovation rate of the building stock and the market penetration of mycelium-wood composites. For each scenario, the overall GWP and CO2 stored values were calculated. Results show the great potential of the innovative material that grants carbon storage in the building stock that could even surpass the amount stored in the 32,500 ha of forest in the area. However, this possibility is heavily influenced by factors independent of the type of insulation used that should be further investigated.
1 Introduction
Global climate change is a present problem that must be addressed as the effects are becoming more and more evident (NASA, 2023). In Europe, buildings account for 40% of the energy consumption and produce 36% of CO2 emissions, representing a great potential for the objective of reducing GHG emissions by 80–95% by 2050 compared to 1990 (Artola et al., 2016). The European stock changes very slowly: 85% of the buildings existing today were built before 2001, and 85–95% of them will be standing in 2050 (European Commission, 2020). In Finland, 43% of the housing stock was built between the 1950s and 1970s (Holopainen et al., 2016), and their renovation, particularly in terms of energy retrofit, could be a great tool to decarbonize the building stock and reach the goal of carbon neutrality, which the country has set for 2035 (Ministry of Econimc Affairs and Employment Energy, 2022). As a matter of fact, by increasing the thermal performance of buildings, the operational energy, especially required for heating, would decrease and, accordingly, the derived greenhouse gas (GHG) emissions.
However, it must be considered that, in addition to operational emissions, there is a conspicuous amount of CO2 release coming from the production and deconstruction of building materials (Ibn-Mohammed et al., 2013). The share of these emissions, known as embodied emissions, has been growing and presented to be up to 50% of the total emissions (Röck et al., 2020), and addressing them could help to cut down even further emissions coming from the construction sector.
Since renovation requires less material than new construction, renovation represents a better option to reduce construction sector emissions. For example, Hasik et al. (2019) compared an adaptive reuse project with new construction in a case study through a life-cycle assessment (LCA) analysis focused on construction materials, therefore excluding installation, use, demolition, and operational energy (A5, B1, B6, B7, and C1, based on LCA categorization). Results show how renovation allows to avoid between 53 and 75% of the impact from new construction on six different impact categories, including global warming potential (GWP). Itard and Klunder (2007) showed how, even considering operational energy, renovation constitutes a more favorable option than new construction. In particular, the study indicates that transformation is the best alternative among different levels of renovation considered since it reaches a good compromise between material consumption and performance. Even broader research was led by the Preservation Green Lab, National Trust for Historic Preservation (2011), comparing renovation and new construction applied to six different building typologies in different climate zones over the course of 75 years. The renovation option grants savings between 4 and 46% in all the scenarios except for the ones that contemplate a change in the intended use since more material is needed to complete the transformation. The material choice could, therefore, help minimize the environmental impact, even if a more consistent quantity is needed to complete the renovation.
Multiple studies (e.g., Pittau et al., 2019a,b,c,d; Carcassi et al., 2020; Galimshina et al., 2022) have compared different biogenic materials with traditional insulation as a façade retrofit solution, applying dynamic life-cycle assessment (DLCA). Results show how insulation materials obtained from fast-growing plants such as straw or hemp constitute a better solution than wood-derived ones since the briefer rotation period allows them to store more carbon dioxide and reach carbon negativity just a few years after the installation. If massively used, strew, for example, could remove up to 100 Mt. of CO2 -eq in 30 years (Pittau et al., 2019c). The great amount of material needed to reach traditional insulation performances, which could represent a problem in terms of costs and supply (Galimshina et al., 2022), is compensated by the fact that these materials are usually by-products of other industries, locally available, and therefore they do not concur to land competition (Carcassi et al., 2020). Switching to bio-based insulation materials could thus make buildings even act as carbon sink (Habert et al., 2020).
Mycelium composites are a particular kind of biogenic material, especially suited for insulation purposes, that have attracted academic and commercial interests as a new form of low-energy bio-fabrication and waste upcycling (Jones et al., 2020). Mycelium is the root structure of fungal organisms (Carcassi et al., 2022), and while growing, it can bind together organic matter, such as forestry and agricultural by-products, creating a solid material that is biodegradable at the end of its life (Elsacker et al., 2021). Because of the air trapped between the growing root and the biomass, and since the substrate used already has decent thermal properties, thermal conductivity has shown the most promising results (Yang et al., 2017; Xing et al., 2018), classifying mycelium composites as an alternative to synthetic insulation materials (Jones et al., 2020). The production of the material from a living organism can achieve a great variety of technical and esthetic properties through slight variations in the fabrication process (Girometta et al., 2019). Different studies (e.g., Yang et al., 2017; Xing et al., 2018; Jones et al., 2020; Zhang et al., 2022) have analyzed how changing different aspects of the production process can affect the final results and what the technical properties of mycelium composites are. The substrate used is definitely an important factor in the property’s definition. Wood can be considered a high-grade substrate material since it is rich in the nutrients needed by the fungi for its growth (Jones et al., 2020). Therefore, using forestry by-products can enhance the quality of the composites, especially in terms of mechanical properties (Vašatko et al., 2022).
The aim of this research is to consider the potential reduction of emissions coming from the application of mycelium-wood insulation in the energy retrofit domain. For the purpose of visualizing large-scale impact, the mycelium-wood insulation was modeled for all residential buildings in the Helsinki metropolitan area. As shown in Figure 1, the technology applied is that of external wall insulation added to the already existing one, independently from the insulation system that currently exists in the buildings. This is a simplification that allows a preliminary calculation to define the potential of large-scale mycelium insulation application with the available data on existing housing stock. Roof insulation was not considered since water stagnation on the horizontal surface is more probable to occur and could damage the biogenic material. Horizontal surface application is necessary to guarantee a proper energy retrofit of the building, but further research on durability issues that go beyond the scope of this study is required.
2 Methodology
2.1 Research process
To fully understand the effects of mycelium-wood insulation application, the present insulation market status was first defined by choosing different categories to consider and characterize them in terms of performance and environmental impact. The same characterization was also conducted for the innovative mycelium-wood material, both from experimental lab work and literature. Through the analysis of the state-of-the-art building stock and by introducing the renovation rate and the mycelium-wood market penetration variables, 20 different scenarios were built. Finally, for each scenario, the insulation amount needed was estimated in addition to the global warming potential (GWP) and CO2 stored values.
In Figure 2, the different research steps are grouped into four macro categories: building stock modeling, insulation material definition, CO2 stored, and CO2-eq emissions. In the building stock modeling section, starting from the dataset and through calculation, the existing buildings’ wall surface was obtained. The surface value was then crossed with the renovation rate variable to define the yearly amount of wall surface renovated for the different scenarios. By introducing the gap between the building stock performances and the current energy requirements and then adding common insulation characterization, market share analysis, and the mycelium-wood market penetration variable, the amount of insulation for each material in the different scenarios was calculated. Finally, it was possible to define CO2-eq emissions and CO2 storage. To define emissions, GWP values of common insulation materials obtained from the Ecoinvent database were introduced. While for the innovative mycelium-wood composite, the production process was entered into a SimaPro model. This information, combined with the previously calculated insulation amounts, provided the CO2 emissions values for the different scenarios. Finally, to obtain the carbon content of the mycelium-wood composite insulation, wood substrate amount and material density data were gathered from the samples produced in the Milan Polimi SaperLab. Subsequently, the results were upscaled and combined with the insulation amounts to acquire the values of CO2 stored in each scenario.
2.2 Insulation materials’ GHG values
Thermal insulation materials can be classified into four main categories: inorganic mineral derived, organic fossil fuel derived, also known as synthetic, organic both from plants and animals, and innovative materials (Grazieschi et al., 2021). The last group was not considered in this study since materials such as aerogel and vacuum insulation panels are not widely used because of issues such as costs, uncertainty of service life, and poor mechanical strength (Schiavoni et al., 2016). For the first group, instead, performance and emissions data of glass and stone wool were gathered since they cover together 58% of the European market (Grazieschi et al., 2021). The synthetic insulation category was considered as the expanded and extruded polystyrene and polyurethane cover approximately 41% of the European market (Grazieschi et al., 2021). Finally, for the organic group, referred to as biogenic, which covers 1% of the remaining market, data on cellulose and wood fibers were collected since they are considered the most used and commercialized products.
The information on market share refers to Europe, but it can be assumed that the Finnish situation reflects the status of the European market: from the gathered case studies on a single detached house (Takano et al., 2015) and multi-story townhouse (Pal et al., 2017) in the Finnish context, the most common insulation materials in Finland are expanded polystyrene (EPS), stone wool and glass wool, cellulose fibers, and blown wool. The roadmap for thermal insulation by Ojanen et al. (2014) adds to the list extruded polystyrene (XPS), polyurethane, and wood fibers, while the reports on sustainable refurbishment of exterior walls and building facades by Häkkinen (2012) and Vares et al. (2012) confirm that these materials are employed in refurbishment.
The insulation materials’ thermal performance was obtained from both literature and Finnish companies’ EPDs. Table 1 shows the GWP values considered for the common insulation materials, gathered from the Ecoinvent database, and the CO2 stored by biogenic insulation, obtained from the KBOB 2022 database. The average value from the collected specific data was considered in the calculation.
2.3 Mycelium-wood GHG value
For the mycelium-wood insulation, samples were realized in the Milan Polimi SaperLab following the production method proposed by Carcassi et al. (2022). Since the examined area is situated in Finland, for the mycelium-wood composite substrate, sawdust and wood chips were used instead of bamboo particles compared to the study by Carcassi et al. (2022). With 86% of the country’s land covered by forest, in fact, the wood industry sector is one of the most important in the Finnish economy (Niinistö et al., 2021), and sawdust and wood chips constitute a largely available by-product. The samples realized were both rectangular (15 × 15 × 5 cm) and cylindrical (Φ 10.5 cm; h = 5 cm). Table 2 shows for each sample the weight measured after the mycelium deactivation and the resulting density. For this study, an average density value of 167.5 kg/m3 was considered.
The amount of wood substrate used in the process was considered for the calculation of CO2 stored in the mycelium-wood composite using the following equation:
where:
• WS is the wood substrate amount in kg/m3 with 70% humidity, namely, an average value of 267 kg/m3;
• CC is the carbon content of the biogenic substrate, namely, 50%;
• 3.67 is the molar weight ratio between CO2 and C.
As for the GHG emissions deriving from the production of the mycelium-wood composite, different stages were included in a cradle-to-gate SimaPro model. The model was obtained starting from the one used by Carcassi et al. (2022) but was adjusted to the wood substrate used in terms of density and environmental load. The samples obtained using sawdust and wood chips, in fact, present a volumetric mass of 167 kg/m3, lower than the MycoBamboo analyzed by Carcassi et al. (2022). Furthermore, the wood substrate used in this study was considered a pure waste of the timber production process with a 0% allocation. The model comprehends the energy-requiring steps of the lab production, namely biomass sterilization and inoculation and the sample deactivation obtained from drying the composite. In addition, the preparation and transportation of the grain spawn package were added to the model. The GWP of the innovative material was calculated considering the national energy mix, where the electrical energy needed is sourced from oil, natural gasses, coal, and wood fuels, and a second option, where energy is produced exclusively by renewable sources (REN). The model was implemented for a cylindrical sample with a diameter of 10 cm and a thickness of 3 cm, and the results were then upscaled to 1 kg of material. The values obtained were 2.13 kg CO2-eq/kg for the mycelium-wood composite produced with the national mix energy and 0.66 kgCO2-eq/kg for the REN production.
Thermal conductivity values for mycelium composites are highly affected by the measurement equipment used. To avoid having the calculation influenced by this factor, thermal characterization of the samples produced in the MaBa.SAPERLab was not conducted, and instead, different research on the thermal properties of mycelium composites was analyzed to define thermal behavior. Table 3 shows the various mycelium composites from which the estimated value of 0.06 W/mK used in this study was obtained. The mycelium-wood composite’s thermal characteristics are based on the volume of each ingredient for a unit of mycelium-wood composite.
2.4 Study area
The analysis focuses, as shown in Figure 3, on the Helsinki Metropolitan area, formed by the municipalities of Helsinki, Espoo, and Vantaa. With a population of over 1.2 million, the area is the most populous urban district in Finland (Statistics Finland, 2023a).
To define the building stock, only residential constructions, including single-family houses, detached and semi-detached houses, and blocks of flats, were considered, as they constitute 62% of the built environment in the Helsinki metropolitan area (Statistics Finland, 2023b), and data gathered by Aalto University were used. From the available specifics on every singular building, the construction footprint was calculated using the following formula:
where:
• GCA is the gross construction area of the building in m2;
• n floors is the number of floors of the building.
Finally, to obtain the wall’s surface values, Sw/Sf factor defined by Göswein et al. (2021) for the Geocluster 7 was applied to the buildings’ footprint, differentiating between single-family houses and multistorey buildings. Geoclusters are defined as groups of states with similar climate conditions, and Geocluster 7 comprehends Finland, Sweden, Estonia, and Latvia. The Sw/Sf factors were defined by considering the data obtained from the TABULA database, and for the geocluster, an average from the different countries was considered.
Different thermal performances were considered for the building according to their construction year. The time interval and U values assigned to the wall envelope were defined following the prescription of the Finnish Ministry of Environment for energy certifications (Ympäristöministeriö, 2017). Since, in the prescription, all the buildings before 1969 were considered in a single group, and a further division has been supposed to consider a linear variation from the 1969 U values. Constructions built after 2009 were not considered since they already meet the thermal requirements. Table 4 shows, for each time interval, the initial thermal resistance (RT0) and the necessary improvement (ΔR) to fulfill the current regulation in terms of energy efficiency (RTF). In addition, the necessary thermal insulation amount to achieve the new performance is shown. It is necessary to mention that we calculate the extra needed insulation, which is for fulfilling ΔR, and do not go through the type of current insulation of each building. It was calculated as a thickness made up of the different insulation categories, according to their market share, applying for each material the following equation:
where:
• MS is the market share of the insulation material category in percentage, namely, 58% for mineral insulation, 41% for synthetic insulation, and 1% for biogenic insulation;
• ΔR is the necessary thermal resistance improvement in m2K/W, as shown in Table 4;
• λ is the thermal conductivity of the insulation material category in W/mK, namely, 0.04 for mineral insulation, 0.032 for synthetic insulation, and 0.045 for biogenic insulation;
• ρ is the density of the insulation material category in kg/m3, namely, 78.3 for mineral insulation, 35.7 for synthetic insulation, and 74.4 for biogenic insulation.
2.5 Scenarios
Two variables were considered to define the different scenarios, i.e., the renovation rate (RR) of the building stock and the market penetration of the innovative mycelium-wood insulation material (MMP). As shown in Figure 4, for the renovation rate, four different options were examined. The base case was set at a steady growth of 1% a year, which corresponds to the present European average (European Commission, 2020). Another option was built considering the Renovation Wave stated by the European Commission, which has the objective of doubling the yearly percentage of buildings renovated, reaching 2% by 2030 and keeping the rate steady until 2050. An intermediate option between the above two was contemplated on the hypothesis that a renovation rate of 2% would be reached by 2050. Finally, a fourth and most optimistic alternative was examined, in which after accomplishing the goal of 2% in 2030, the rate keeps growing at the same pace until 2050. Every option starts from the present renovation rate of 1%, which means that 4.36E+05 m2 would be renovated in the first year. Table 5 shows, for each alternative, how much of the building surface renovated grows every year as compared to the previous one since the percentage increase of the renovation rate is linear for every option. It also presents the total façade surface that would be renovated by 2050 and is presented as the percentage of the total building stock surface value of 4.36E+07 m2. In every option, prioritization was considered, contemplating the renovation of the oldest building first and then moving gradually to the newer ones.
Figure 5 shows the five different alternatives analyzed for the mycelium-wood market penetration variable. The first one is the base case that describes the present state of a 0% market penetration. The four following options contemplate the possibilities of an increasing commercialization of the material by 2050: 25, 50, 75, and 100%. This means, for example, for the first option, that by 2050, 25% of the amount of insulation needed to renovate the residential building stock to meet current regulations will be made of mycelium-wood composites, while the rest of it will be supplied by common insulation materials in the shares presented in sub-section 2.2. The mycelium-wood market penetration percentages represent hypothetical options at this point since they do not take into account the insulation market adjustments that would be needed nor the production capacity, both of which would need further research.
Finally, the color characterization displayed in Table 6 explains how the different options assumed for the two variables, shown in Figures 4, 5, were combined to define 20 different scenarios.
3 Results
The research aim was to evaluate the possible benefits deriving from the application of mycelium-wood composites as external insulation in building renovation. In particular, the study focused on the Helsinki Metropolitan area, and therefore, wood sawdust and chips, largely locally available by-products, were considered as the composite substrate. The material lab production allowed us to gather information on production stages and organic matter amounts that were used in the calculation of both GWP values and CO2 storage. The mycelium-wood composite was compared with common insulations throughout 20 different scenarios, which were defined by making a hypothesis on the progressive increase of the renovation rate and the new material market penetration. Results were considered separately for the mycelium-wood composite production carried out with the national energy mix and with energy solely obtained from renewable sources (REN).
In both cases (Figures 6, 7), the scenarios form linear tendency lines as the mycelium-wood market penetration increases, while the space between the line shows the CO2 emissions rise caused by the growth of the renovation rate due to the greater amount of insulation required.
Figure 6 shows how, since the national energy mix relies heavily on fossil fuels, the composite usage affects mainly in terms of emissions’ increase, causing the tendency lines to have a 45° slope. In Figure 7, instead, the tendency lines trend suggests that the benefits coming from the CO2 absorption of the mycelium-wood composites are by far greater than the caused emission gain. It is still observable that a slight rise in the GWP value was caused by the growth of the mycelium-wood market penetration. Despite the mycelium-wood composite GWP REN unit value being lower than all the common insulation, the higher conductibility that characterizes the mycelium-wood insulation requires a higher amount of material to be used.
The same trend can be observed in the material flow shown in Figure 8. The total weight of insulation grows with the mycelium-wood market penetration. The composite has, in fact, higher conductivity than most of the common insulation considered, needing a larger thickness to achieve the same performance. In addition, with a registered average of 167.5 kg/m3 from the lab samples, the material is quite dense, increasing even more the total weight needed. These characteristics offer an advantage in terms of CO2 stored since greater material quantity means that more carbon dioxide can be cumulated. On the other hand, more weight could represent a challenge in terms of application, and the larger thickness would take up more space, increasing the wall width.
Finally, to better understand the benefits deriving from the mycelium-wood insulation application, a benchmark for the CO2 stored values was considered. As a reference, the amount of CO2 stored by 32,500 ha (City of Espo, 2023; City of Helsinki, 2023; Vantaa, 2023) of forest in the Helsinki metropolitan area in the 28 years’ time-lapse was calculated, referring to the forest land removal value of Statistics Finland (Niinistö and Piipatti, 2019). It is noticeable that the scenarios with the highest mycelium-wood market penetration rate can even exceed the benchmark’s values, both in the national mix and REN cases (Figures 6, 7).
4 Discussion and conclusion
Environment, as one of the sustainability features, plays a significant role in climate change mitigation, highlighting the need for careful consideration of material use for the purpose of building construction and renovation (Delavar et al., 2023). This research aimed at defining the possible benefits of the use of mycelium-wood and wood substrate composite insulation material in large-scale energy retrofit, using the Helsinki metropolitan area as the case study area. To characterize the innovative material, density and carbon content information were gathered from the samples realized in the Polimi SaperLab, while the production processes were included in a SimaPro model to obtain the GWP value of the mycelium-wood insulation. On the other hand, the GWP values for common insulation material in Europe were extracted from the literature. Different scenarios were then built, considering common insulation materials, current market shares, and assuming an increasingly mycelium-wood market penetration. A renovation rate variable was also considered, starting from the current situation and expecting a growth in the yearly amount of buildings renovated in the future. For each scenario, GWP and CO2 storage values were calculated, and they are shown in the results section.
Wood, as a low-carbon and carbon-storing material (see e.g., Amiri et al., 2020; Amiri, 2021; Talvitie et al., 2023), is one of the main components of mycelium-wood composite. It can be concluded that mycelium-wood composites represent a good possibility to make buildings act as carbon sinks and reduce emissions in the construction industry, but the innovative material usage alone is not sufficient. Even if the results show that by using mycelium-wood composite as insulation material, the amount of CO2 stored in the Helsinki metropolitan area building stock could equal and even, in some cases, surpass the carbon storage of forest in the same area, this possibility is heavily influenced by factors independent from the type of insulation used.
The first factor is the renovation rate, which highlights the necessity to increase the amount of buildings renovated yearly. Figure 9 shows for the different construction periods the amount of wall surface to be renovated and the necessary increase of thermal resistance to meet current energy regulations. It emerges how the most critical situation is the period before 1961 since it has the highest value both in terms of wall surface and ΔR. The periods between 1962 and 1977, even with a high ΔR value, present a considerably lower wall surface to be renovated and look, therefore, less concerning. Another critical period is the one between 1985 and 2002: even if the ΔR value has significantly improved as compared to 1961, the amount of wall surface is the second highest among the different periods. Finally, it can be noticed that even the buildings constructed between 2008 and 2009 need renovation to respect the performances required by current regulations.
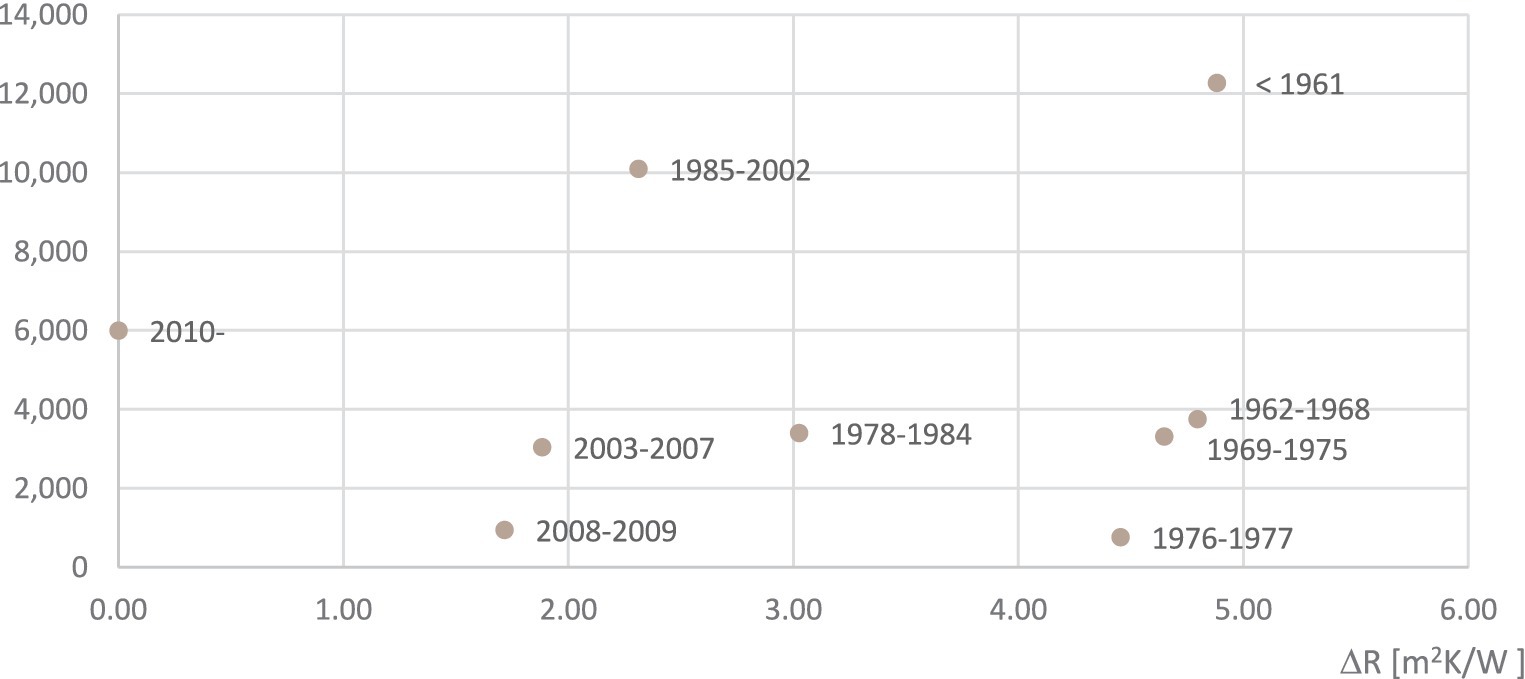
Figure 9. Building stock wall surface and necessary performance improvement by the construction period.
Table 7 shows how many of the existing buildings could be renovated in percentage in each scenario. In the scenarios with a 1% yearly renovation rate (BAU-S5), the building dated before 1961 would be completely renovated. This is possible thanks to the prioritization put in place in the application of the renovation rate variable, where it is assumed that older buildings are renovated first, moving gradually to the newer ones. The 1985–2002 period, instead, would be completely renovated only in the scenarios with the most optimistic renovation rate option (S16-20), which reaches values four times greater than the present rate. Even in these scenarios (S16-20), however, by 2050, only 47% of the constructions built between 2003 and 2007 would be renovated, and none of those built in the 2008–2009 period would undergo refurbishment.
Some consideration needs to be made also on the mycelium-wood composite production process and on the need to decarbonize energy production. The production stages included in the SimaPro model are, in fact, laboratory-scaled procedures; therefore, an optimization for larger-scale production could help to reduce the emissions caused by the innovative material manufacture. Furthermore, the development of industrialized processes would be necessary to ensure enough mycelium-wood insulation for large-scale applications. At the present market state, in fact, mycelium-wood composites are mostly still an experimental material. Even though many mycelium-related companies are springing up throughout Europe, such as Mogu, Myceen, and Mylab, their products are used mostly for niche applications and would not be able to support such a large-scale application as speculated in this study.
An even more significant impact was registered by the type of energy used for the production. The comparison between the results obtained with the national energy mix and with the energy derived from renewable sources underlines the importance of investments toward energy decarbonization. In the REN results, in fact, the small emissions increase due to the growth of the mycelium-wood market penetration is negligible when compared with the amount of CO2 absorbed by the material, while in the national mix case, the rise of the GWP value is significant enough to almost overrule the benefits of the carbon storage.
Finally, further considerations should be made on the wood substrate supplying. Even if the wood industry is one of the most important sectors of the Finnish economy and a consistent amount of manufacture by-products can be expected, at present, a conspicuous part of them is burned to obtain energy. Moreover, Finland aims to push even more in the future on this kind of energy production to replace fossil fuels. Massive demand for wood sawdust and chips for large-scale mycelium-wood composites production could, therefore, clash with the country’s plan and energy supply. Nevertheless, we excluded roof insulation from our study, as it plays a main role in the overall thermal performance of a building. This was because of moisture issues, which makes it necessary to research solutions to make it possible to use mycelium-wood composite in moisture conditions.
Data availability statement
The original contributions presented in the study are included in the article/supplementary material, further inquiries can be directed to the corresponding author.
Author contributions
AC: Conceptualization, Data curation, Formal analysis, Methodology, Validation, Visualization, Writing – original draft, Writing – review & editing. AA: Data curation, Formal analysis, Methodology, Validation, Writing – review & editing. SJ: Supervision, Validation, Writing – review & editing. FP: Conceptualization, Formal analysis, Funding acquisition, Methodology, Project administration, Supervision, Visualization, Writing – review & editing.
Funding
The author(s) declare that financial support was received for the research, authorship, and/or publication of this article. This study was funded by the Academy of Finland (13353847) and the Finnish Ministry of Environment (310371). In addition, we thank Erasmus+ for their exchange visit support.
Conflict of interest
The authors declare that the research was conducted in the absence of any commercial or financial relationships that could be construed as a potential conflict of interest.
Publisher’s note
All claims expressed in this article are solely those of the authors and do not necessarily represent those of their affiliated organizations, or those of the publisher, the editors and the reviewers. Any product that may be evaluated in this article, or claim that may be made by its manufacturer, is not guaranteed or endorsed by the publisher.
References
Amiri, A. How green building certificates fulfill the environmental goals in the buildings’ life cycle. (2021).
Amiri, A., Ottelin, J., Sorvari, J., and Junnila, S. (2020). Cities as carbon sinks—classification of wooden buildings. Environ. Res. Lett. 15:094076. doi: 10.1088/1748-9326/aba134
Artola, I., Rademaekers, K., Williams, R., and Yearwood, J., Boosting building renovation: What potential and value for Europe? (2016).
Carcassi, O. B., De Angelis, E., Iannaccone, G., Malighetti, L. E., Masera, G., and Pittau, F. (2020). “Bio-based materials for the Italian construction industry: buildings as carbon sponges” in Regeneration of the built environment from a circular economy perspective (Switzerland: SpringerOpen), 237–247.
Carcassi, O. B., Minotti, P., Habert, G., Paoletti, I., Claude, S., and Pittau, F. (2022). Carbon footprint assessment of a novel bio-based composite for building insulation. Sustain. For. 14:1384. doi: 10.3390/su14031384
City of Espo . (2023). Characteristics of Espoo forests. Available at: https://www.espoo.fi/en/sports-and-nature/nature-espoo/characteristics-espoo-forests (Accessed July 20, 2023).
City of Helsinki . (2023). Management of forests. Available at: https://www.hel.fi/en/urban-environment-and-traffic/general-maintenance/management-of-parks-and-green-spaces/management-of-forests (Accessed July 20, 2023).
Delavar, T., Amiri, A., Borgentorp, E., and Junnila, S. (2023). The prioritization of sustainability features of buildings from the viewpoint of experts. Buildings 13:3021. doi: 10.3390/buildings13123021
Elsacker, E., Søndergaard, A., Van Wylick, A., Peeters, E., and De Laet, L. (2021). Growing living and multifunctional mycelium composites for large-scale formwork applications using robotic abrasive wire-cutting. Constr. Build. Mater. 283:122732. doi: 10.1016/j.conbuildmat.2021.122732
Elsacker, E., Vandelook, S., Brancart, J., Peeters, E., and De Laet, L. (2019). Mechanical, physical and chemical characterisation of mycelium-based composites with differents types of lignocellulosic substrates. PLoS One 14:e0213954. doi: 10.1371/journal.pone.0213954
European Commission , Communication from the commission to the European Parliament, the council, the European economic and social committee and the Committee of the Regions: A renovation wave for Europe - greening our buildings, creating jobs, improving lives. (2020).
Galimshina, A., Moustapha, M., Hollberg, A., Padey, P., Lasvaux, S., Sudret, B., et al. (2022). Bio-based materials as a robust solution for building renovation: a case study. Appl. Energy 316:119102. doi: 10.1016/j.apenergy.2022.119102
Girometta, C., Picco, A. M., Baiguera, R. M., Dondi, D., Babbini, S., Cartabia, M., et al. (2019). Physico-mechanical and thermodynamic properties of mycelium-based biocomposites: a review. Sustain. For. 11:281. doi: 10.3390/su11010281
Göswein, V., Reichmann, J., Habert, G., and Pittau, F. (2021). Land availability in Europe for a radical shift toward bio-based construction. Sustain. Cities Soc. 70:102929. doi: 10.1016/j.scs.2021.102929
Grazieschi, G., Asdrubali, F., and Thomas, G. (2021). Embodied energy and carbon of building insulating materials: a critical review. Clean. Environ. Syst. 2:100032. doi: 10.1016/j.cesys.2021.100032
Habert, G., Röck, M., Steininger, K., Lupísek, A., Birgisdottir, H., Desing, H., et al. (2020). Carbon budgets for buildings: harmonising temporal, spatial and sectoral dimensions. Build. Cities 1, 429–452. doi: 10.5334/bc.47
Häkkinen, T. (2012). Sustainable refurbishment of exterior walls and building facades. VTT Technol. 30. Available at: https://www.osti.gov/etdeweb/biblio/22006349
Hasik, V., Escott, E., Carlisle, S., Faircloth, B., and Bilec, M. M. (2019). Comparative whole building life cycle assessment of renovation and new construction. Build. Environ. 161:106218. doi: 10.1016/j.buildenv.2019.106218
Holopainen, R., Milandru, A., Ahvenniemi, H., and Häkkinen, T. (2016). Feasibility studies of energy retrofits – case studies of nearly zero-energy building renovation. Energy Procedia 96, 146–157. doi: 10.1016/j.egypro.2016.09.116
Ibn-Mohammed, T., Greenough, R., Taylor, S., Ozawa-Meida, L., and Acquaye, A. (2013). Operational vs. embodied emissions in buildings - a review of current trends. Energ. Buildings 66, 232–245. doi: 10.1016/j.enbuild.2013.07.026
Itard, L., and Klunder, G. (2007). Comparing environmental impacts of renovated housing stock with new construction. Build. Res. Inform. 35, 252–267. doi: 10.1080/09613210601068161
Jones, M., Mautner, A., Luenco, S., Bismarck, A., and John, S. (2020). Engineered mycelium composite construction materials from fungal biorefineries: a critical review. Mater. Des. 187:108397. doi: 10.1016/j.matdes.2019.108397
Ministry of Econimc Affairs and Employment Energy , Carbon neutral Finland 2035 - national climate and energy strategy. (2022).
NASA . (2023). The effects of climate change. Available at: https://climate.nasa.gov/effects/.
Niinistö, T., Peltola, A., Räty, M., Sauvula-Seppälä, T., Torvelainen, J., Uotila, E., et al. (2021). Finnish statistical yearbook of forestry 2021. Helsinki: Luonnonvarakeskus.
Niinistö, S., and Piipatti, R., "Greenhouse gases 2017, land use, land use change and forestry," Statistics Finalnd (2019). [Online]. Available at: https://www.stat.fi/til/khki/2017/01/khki_2017_01_2019-01-15_tie_001_en.html#:~:text=According%20to%20Statistics%20Finland%27s%20preliminary,in%20the%20comparison%20year%201990 (Accessed July 20, 2023).
Ojanen, T., Pinto Seppä, I., Koukkari, H., and Nykänen, E. (2014). Roadmap for the thermal insulation solutions of future building envelopes. VTT Technology-series report. VTT Technical Research Centre of Finland, 114.
Pal, S. K., Takano, A., Alanne, K., Palonen, M., and Siren, K. (2017). A multi-objective life cycle approach for optimal building design: a case study in Finnish context. J. Clean. Prod. 143, 1021–1035. doi: 10.1016/j.jclepro.2016.12.018
Pittau, F., Carcassi, O. B., Servalli, M., Pellegrini, S., and Claude, S. (2022). Hygrothermal characterization of bio-based thermal insulation made of fibres from invasive alien lake plantsbounded with mycelium. Earth Environ. Sci. 1078:012069. doi: 10.1088/1755-1315/1078/1/012069
Pittau, F., Habert, G., and Iannaccone, G. (2019b). A life-cycle approach to building energy retrofitting: Bio˗Based technologies for sustainable urban regeneration. IOP Conf. Ser. Earth Environ. Sci. 290:012057. doi: 10.1088/1755-1315/290/1/012057
Pittau, F., Habert, G., and Iannaccone, G. (2019c). The renovation of the building stock in Europe: an essential opportunity to store carbon in buildings. Technol. Eng. Mater. Architect. 5,147–157. doi: 10.30682/tema0502l
Pittau, F., Iannaccone, G., Lumia, G., and Habert, G., “Towards a model for circular renovation of the existing building stock: a preliminary study on the potential for CO2 reduction of bio-based insulation materials”, In: Sustainable Built Environment Conference, Graz (2019d).
Pittau, F., Lumia, G., Heeren, N., Iannaccone, G., and Habert, G. (2019a). Retrofit as a carbon sink: the carbon storage potentials of the EU housing stock. J. Clean. Prod. 214, 365–376. doi: 10.1016/j.jclepro.2018.12.304
Preservation Green Lab, National Trust for Historic Preservation , The greenest building: quantifying the environmental value of building reuse. (2011).
Röck, M., Saade, M. R. M., Balouktsi, M., Rasmussen, F. N., Birgisdottir, H., Frischknecht, R., et al. (2020). Embodied GHG emissions of buildings – the hidden challenge for effective climate change mitigation. Appl. Energy 258:114107. doi: 10.1016/j.apenergy.2019.114107
Schiavoni, S., D'Alessandro, F., Bianchi, F., and Asdrubali, F. (2016). Insulation materials for the building sector: a review and comparative analysis. Renew. Sust. Energ. Rev. 62, 988–1011. doi: 10.1016/j.rser.2016.05.045
Statistics Finland , Population in the largest municipalities [Online]. (2023a). Available at: https://www.stat.fi/tup/suoluk/suoluk_vaesto_en.html#Population%20in%20the%20largest%20municipalities (Accessed July 20, 2023).
Statistics Finland . Number of buildings by intended use and year of construction, 2022 [Online]. (2023b). Available at: https://pxdata.stat.fi/PxWeb/pxweb/en/StatFin/StatFin__rakke/statfin_rakke_pxt_116g.px/ (Accessed July, 20, 2023).
Takano, A., Pal, S. K., Kuittinen, M., Alanne, K., Hughes, M., and Winter, S. (2015). The effect of material selection on life cycle energy balance: a case study on a hypothetical building model in Finland. Build. Environ. 89, 192–202. doi: 10.1016/j.buildenv.2015.03.001
Talvitie, I., Kinnunen, A., Amiri, A., and Junnila, S. (2023). Can future cities grow a carbon storage equal to forests? Environ. Res. Lett. 18:044029. doi: 10.1088/1748-9326/acc677
Vantaa . (2023). Metsänhoito. Available at: https://www.vantaa.fi/fi/asuminen-ja-ymparisto/ymparisto-ja-luonto/metsanhoito (Accessed July 20, 2023).
Vares, S., Pulakka, S., Toratti, T., Fulop, L., Hradil, P., Vesikari, E., et al. (2012). Sustainable refurbishment of exterior walls and building facades. Final report, part B – general refurbishment concepts. VTT Technol. 33.
Vašatko, H., Gosch, L., Jauk, J., and Stavric, M. (2022). Basic research of material properties of mycelium-based composites. Biomimetics 7:51. doi: 10.3390/biomimetics7020051
Xing, Y., Brewer, M., El-Gharabawy, H., Griffith, G., and Jones, P. (2018). Growing and testing mycelium bricks as building insulation materials. Earth Environ. Sci. 121:022032. doi: 10.1088/1755-1315/121/2/022032
Yang, Z., Zhang, F., Still, B., White, P., and Amistislavski, P. (2017). Physical and mechanical properties of fungal mycelium-based biofoam. J. Mater. Civ. Eng. 29:04017030. doi: 10.1061/(ASCE)MT.1943-5533.0001866
Ympäristöministeriö (2017). Laskennallisen Energiatehokkuuden Vertailuluvun (E-Luvun) Määrittäminen Energiatodistuksessa, 1048/2017, Liite 1. Helsinki.
Keywords: renovation, retrofit, low-carbon, greenhouse gas, carbon storage, global warming potential
Citation: Candido A, Amiri A, Junnila S and Pittau F (2024) Mycelium-wood composites as a circular material for building insulation. Front. Sustain. Cities. 6:1412247. doi: 10.3389/frsc.2024.1412247
Edited by:
Roberto Alonso González-Lezcano, CEU San Pablo University, SpainReviewed by:
Sofia Melero-Tur, CEU San Pablo University, SpainMaría Eugenia Maciá, CEU San Pablo University, Spain
Copyright © 2024 Candido, Amiri, Junnila and Pittau. This is an open-access article distributed under the terms of the Creative Commons Attribution License (CC BY). The use, distribution or reproduction in other forums is permitted, provided the original author(s) and the copyright owner(s) are credited and that the original publication in this journal is cited, in accordance with accepted academic practice. No use, distribution or reproduction is permitted which does not comply with these terms.
*Correspondence: Ali Amiri, YWxpLmFtaXJpQGFhbHRvLmZp