- 1Department of Biology, Saint Louis University, St. Louis, MO, United States
- 2Department of Biology, University of Missouri–St. Louis, St. Louis, MO, United States
Green infrastructure on rooftops in urban areas can enhance important ecosystem services. In addition to mitigating water runoff and regulating building temperatures, green roofs can provide food and nesting resources for wildlife. Rooftop gardens can also be utilized to cultivate food crops, giving them the potential to attenuate instances of food insecurity which are commonplace in many urban areas. Given that many crops depend on bee pollination, it is imperative to characterize the rooftop bee community. Therefore, we sampled three urban food roofs near downtown St. Louis, MO during the 2017 and 2018 growing seasons. We found 38 bee species utilizing the rooftop farms. We then compared the rooftop bee communities to those of two nearby ground-level community gardens. The roofs had lower abundances of Bombus species than the ground-level sites while non-native cavity nesters in the genus Megachile were overrepresented. These results indicate that the rooftop bee communities represent a nested subset of the species found at ground level. This has important implications for the management of green roofs for growing crops, as this shift in bee community structure may play a role in determining which crops can be adequately supported with pollination services.
Introduction
Cities aiming to combat the negative impacts of impervious surfaces, including stormwater runoff, polluted water and air, and the urban heat island effect employ green infrastructure methodologies that marry engineering solutions with natural processes (Parker and Zingoni de Baro, 2019). One cost-effective solution, green roofs, can mitigate flooding events, help regulate building temperature, and lessen the impact of pollutants entering storm drains (Getter and Rowe, 2006). Green roofs also improve the aesthetics of urban landscapes, and can serve as public recreational spaces as seen from New York City's High Line, and Denmark's CopenHill. Additionally, green roofs have been found to provide habitat to a variety of birds (Wang et al., 2017; Belcher et al., 2018), arthropods (Madre et al., 2013; Wooster et al., 2022), and pollinators (Colla et al., 2009; Tonietto et al., 2011).
Although there have been many investigations into plants suitable for green roofs (e.g., Dvorak and Volder, 2010), a review of the literature examining bees on green roofs shows a relative paucity of research (Hofmann and Renner, 2018). Furthermore, most of the literature focuses on managed honeybees, with only a third of the papers (n = 8) focusing on wild bee species diversity. A positive conclusion by Hofmann and Renner (2018) was that green roofs may have the potential to host a large diversity of wild bee species. Primarily, the bee species found on green roofs tend to be pollen generalists, cavity nesters, and medium sized (MacIvor and Lundholm, 2011; Tonietto et al., 2011). Alternatively, in a more recent study conducted in Geneva, Switzerland, Passaseo et al. (2021) found the functional trait diversity among green roof bees was representative of the ground-level bee community. More research on a variety of green roof habitats could further elucidate the extent to which these spaces are being used by bees, and how their management can contribute to pollinator conservation.
The bee community on roofs is also influenced by the characteristics of the roofs themselves. In a study of wild bee diversity of green roofs in Vienna, Austria, bee diversity and abundance were positively correlated with percent of flower coverage (Kratschmer et al., 2018). Bee diversity also increased with the overall flower diversity, and as with other studies, cavity nesting bees were dominant. However, ground nesting bees increased in abundance and diversity as the amount of fine substrate soil on the roof increased (Kratschmer et al., 2018).
An emerging trend in green roof management is growing food crops (Cristiano et al., 2021). These so-called “food roofs” could be a tool to address food security via urban agriculture (Specht et al., 2014). Additionally, in many cities the soil is contaminated with harmful compounds such as lead, making certain crops grown in these soils unsafe to eat (Byers et al., 2020). Thus, importing clean soil to structures like roofs to grow crops can ensure that the food is safe to consume (Brown and Jameton, 2000).
The success of food roofs will depend in part on whether insect pollinators that visit the crops are both diverse and abundant enough to provide adequate pollination services. Previous research has suggested that green roof arthropod communities exhibit lower abundances and species richness when compared to nearby ground-level habitats (Wang et al., 2022). Thus, it is possible that decreased bee diversity may result in diminished pollination rates for animal-pollinated crops on green roofs (Ksiazek et al., 2012). Many crops, such as members of the families Cucurbitaceae (e.g., melon, squash, cucumber) and Solanaceae (e.g., tomato, pepper, eggplant), require insect pollination for fruit set (Stoner, 2020; Cooley and Vallejo-Marín, 2021). Most Solanaceae also require sonication for pollen release, which can only be performed by certain bee taxa including Bombus and some Halictidae (Cardinal et al., 2018). This will be critical in highly urbanized areas where the amount of impervious surface around roof gardens is extensive and therefore the distances between potential source habitats are greater. Furthermore, for potential pollinators to find these green roofs they must also be capable of ascending to high elevations. This represents a type of environmental filtering that selects for stronger flyers, which tend to be larger bodied bees like members of the genera Bombus and Xylocopa (Wojcik and McBride, 2012).
The goals of this study were 2 fold: (1) to document the bee community diversity of three urban food roofs in downtown St. Louis, MO; and (2) to compare the bee diversity of food roofs to nearby community gardens with similar crop diversity.
Materials and methods
Study sites
The three roof sites were in the downtown area of St. Louis, Missouri, USA (Figure 1). These rooftop farms were installed and maintained by the non-profit organization Urban Harvest STL. Food Roof Farm (FRF) was established in 2015 and featured a greenhouse, vegetated walls, and raised garden beds. The building is two stories (9 m), with a roof footprint of 790 m2. The surrounding 500 m is 87% impervious surface at 1-meter resolution (East-West Gateway Council of Governments, 2017). During the time of study, FRF housed a large mix of native and non-native flowering plants including edible herbs, legumes, vegetables, and Missouri natives including Echinacea spp., Asclepias spp., and Rudbeckia spp.
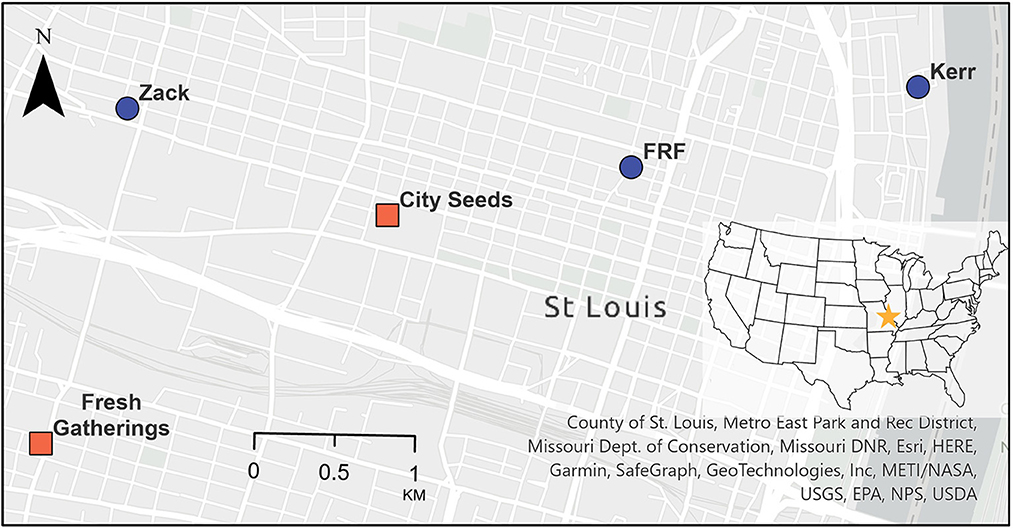
Figure 1. Map of collection locations in downtown/midtown St. Louis, Missouri. Food roofs sites are represented as blue circles, and ground-level community gardens are represented as orange squares.
The Kerr location was established in 2006 as a green roof using sedum Green Roof Blocks™, which are small self-contained units prefilled with growing substrate. Later, Urban Harvest STL partially converted the space into a food roof with the addition of modular Smart Pots®, which are circular fabric pots. The building is one story (5 m) and the roof footprint is 165 m2. It is adjacent to the Mississippi river (35% water within 500 m) and is surrounded by abandoned industrial buildings and overgrown lots. The surrounding 500 m is 50% impervious surface at 1-meter resolution. During the time of study, Kerr was growing vegetables such as squashes, tomatoes, and peppers, and herbs including basil and thyme, while still maintaining large amounts of sedum.
The Zack location was established in 2018 using Recover Aerated Media Modules (RAMMs), which are portable fabric pots filled with growing media that are supported by plastic crates. The building is four stories (16 m) and the footprint is 315 m2. The surrounding 500 m is 70% impervious surface at 1-meter resolution. The sampling of this food roof was done during its first active year, during which it was used to grow many herbs and vegetables, a few natives including Asclepias spp., and a variety of non-native ornamental flowers.
Sampling methodology
In 2017, we sampled FRF and Kerr 10 times between May and August. In 2018, each of the three roofs were sampled eight times each between June and August. Sampling occurred roughly weekly between the hours of 9:30 and 14:00 on days that ranged from sunny to partially cloudy. All pollinator sampling was performed via targeted aerial netting. FRF was sampled for 90 min during each visit whereas Kerr and Zack were sampled for 60 min. Sampling effort was comparable given the area covered by each garden. Honeybees, Apis mellifera, were not intentionally collected and were excluded from analyses. Bees were identified to species level by Nina Fogel and Michael Arduser utilizing regional taxonomic keys (Arduser, 2020; Ascher and Pickering, 2021). All specimens were pinned, labeled, processed, and housed in the insect collection of the biology department at Saint Louis University (catalog numbers jkr0001-jkr0889).
Rooftop species diversity analysis
To determine if there was a difference in species richness between the roofs, we utilized the “iNext” package (Hsieh et al., 2016) in the R computational environment (R Core Team, 2022). We used coverage-based rarefaction curves (Chao and Jost, 2012) to interpolate and extrapolate the data to obtain relevant metrics utilizing Hill numbers (Roswell et al., 2021). For Hill numbers (denoted as q), q = 0 is richness, q = 1 is the Shannon–Weiner diversity index, and q = 2 is equivalent to Simpson's diversity index (Roswell et al., 2021).
We used coverage-based extrapolation in the rarefaction analysis with 95% confidence intervals to determine differences among sites. Since we lacked sampling data from Zack in 2017, we only compared specimens from 2018. An analysis of both years for the other two sites is provided in Supplementary material.
Comparison to ground-level sites
To determine if the roof community was a subset of the ground-level community, we compared the aggregated roof data to that of the two nearest community gardens. The community gardens, City Seeds (planted area ~2,300 m2) and Fresh Gatherings (planted area ~550 m2), are located an average distance of 1.7 and 3.2 km away from the food roofs, respectively (Figure 1). Both ground locations contained comparable crops to those found in the food roofs. They also have similar surrounding impervious surface cover at 500 m using 1-meter resolution; 82% for City Seeds, and 62% for Fresh Gatherings. The sites were sampled weekly during the summer months in 2015 and 2016 following the same protocol as the roofs. All ground and roof sites were located in the contiguous downtown/midtown area of St. Louis city. Furthermore, all sites were at least 1 km apart and therefore the data are spatially independent due to the small foraging distance of most bees (Greenleaf et al., 2007). Because the abundances of individual species vary year-over-year, and the sampling years differ from the roof and ground locations, we focused on the relative abundances at the genus level for our ground to roof comparisons. Singletons and doubletons were removed. A correlation test, using Kendall's tau, was used to determine similarity between the pooled ground and pooled roof locations.
Additionally, we created a dendrogram of Euclidean distance and Ward's clustering using the “hclust” and “dist” functions in the vegan package (Oksanen et al., 2019). The general expectation is that if roofs are not a challenge for bees to reach, then dissimilarities should be based on random sampling error, and cluster distances should be similar among all locations. Alternatively, if some bee taxa lack the ability to reach the roofs (or locate the resources on them), then dissimilarities will emerge, and food roofs should cluster together.
Results
There was variation in the bee abundance and community composition between the roofs. We collected a total of 889 individuals, from 17 genera and 38 species (Table 1). FRF had 273 collected individuals in 2017 and 255 in 2018; Kerr had 77 collected individuals in 2017 and 162 in 2018; and Zack had 107 collected individuals from 2018. Six introduced cavity nesting species accounted for 40% of the collected roof specimens. Of the 60% of collected bees that are native, 22% (n = 117) nest above ground.
In 2018, the year that we have data for all three sites, there were differences in the species composition for the three roofs (Figure 2). We used diversity indices estimates based on coverage following Roswell et al. (2021). We found FRF had 26 observed species (q = 0), a q = 1 of 13.92 ± 0.91, and q = 2 of 9.6 ± 0.83. Kerr had 22 observed species (q = 0), a q = 1 of 8.55 ± 0.93 and q = 2 of 4.3 ± 0.61. Zack had the lowest diversity, with 13 observed species (q = 0), a q = 1 of 8.26 ± 0.71, and q = 2 of 6.78 ± 0.65. However, it is a possibility that the low diversity on Zack is because we sampled during the first season it was constructed, and thus there was low colonization, as compared to innate characteristics of the location.
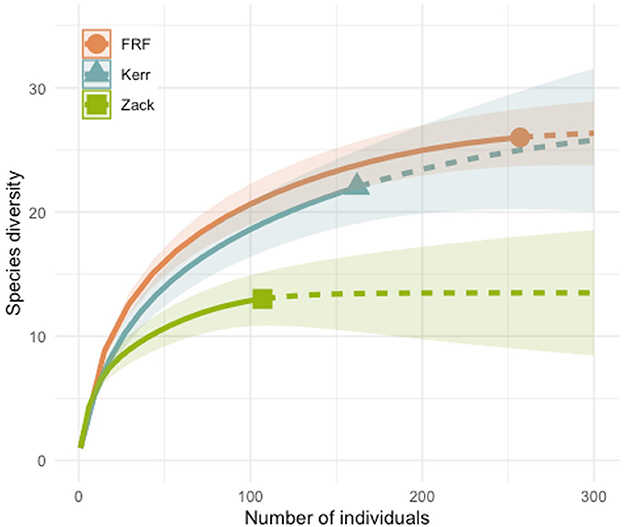
Figure 2. Species accumulation curves for the three roof sites for 2018. The solid lines denote rarefaction whereas the dotted lines are extrapolation. Confidence intervals are 95%.
The rooftop bee community differed significantly from the ground-level community (tau = 0.415, z = 2.488, p = 0.012). Roofs had a greater percentage of leaf-cutter bees (Megachile spp.), and decreased abundances of large bees including Bombus spp., Melissodes spp., and Xylocopa virginica, as well as fewer sweat bees in the genus Halictus (Figure 3). Additionally, the roof community was lacking the Cucurbitaceae specialists Peponapis pruinosa and Xenoglossa strenua, which were both present in the nearby ground sites. Only 12.6% of the ground specimens were introduced species. Cluster analysis further corroborated these results, with the two ground sites forming a cluster separate from the three roof sites (Figure 2). Furthermore, FRF and Zack were more similar to each other in community composition than they were to Kerr.
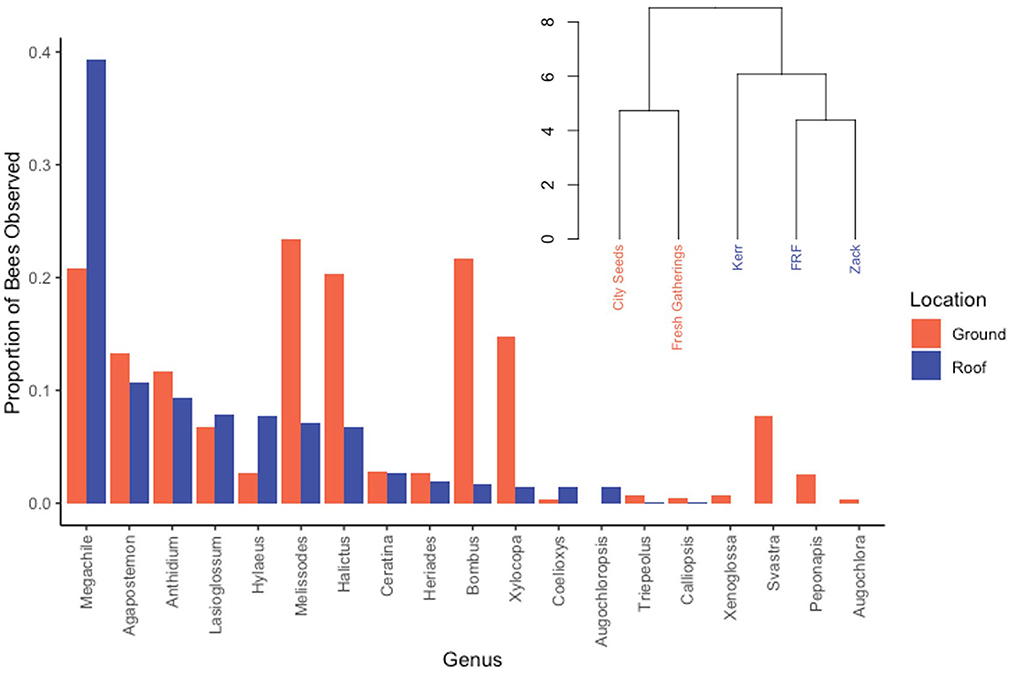
Figure 3. A comparison of community makeup at ground-level and roof-level by genus. The dendrogram indicates that the sites are grouped distinctly into two communities based on site type. The disparity in proportions of Megachile and several Apidae genera are the largest contributors to the difference in community composition.
Discussion
The overall community structure of the food roofs does not represent a random assortment drawn from the city's species pool (Camilo et al., 2017). The high abundance and diversity of non-native species is not surprising given that previous research has found urbanization to be positively correlated with introduced species (Fitch et al., 2019; Gruver and CaraDonna, 2021). Additionally, green roofs have been found to have a higher percentage of non-native cavity nesting species than nearby ground-level habitats (Tonietto et al., 2011), which is consistent with our findings. It has been posited that cavity-nesting bees may be pre-adapted to flying at greater heights due to their nest searching behaviors (MacIvor, 2016), which may help explain why these species are more commonly observed in rooftop gardens.
Most non-native species tend to be generalists that can exploit a broad range of resources. Thus, the traits they exhibit allow them to take advantage of human-dominated landscapes (Russo et al., 2021). Introduced species can outperform natives' physiological thermal maxima allowing them to exploit resources when the natives cannot (da Silva et al., 2021). Thus, it is possible that the non-native species observed in the roof gardens can outperform the natives through an enhanced ability to ascend to rooftop elevations or tolerate the novel conditions located therein.
It is unclear from the literature whether green roofs filter for bees with larger or smaller body sizes. In this case, elevation does seem to represent a challenge. In our study, the food roof bee community differs greatly from the ground-level sites due in part to the absence of large bees. Large-bodied members of the family Apidae, especially members of the genera Bombus, Svastra, and Xylocopa were absent or had decreased abundances compared to nearby ground-level gardens. MacIvor et al. (2015) found that smaller bodied bees were significantly less common than medium to large-bodied bees on Sedum planted roofs and found the two most abundant species were Megachile rotundata and Bombus griseocollis. Alternatively, Ksiazek et al. (2012) found that bees with smaller body sizes dominated the specimens collected on Chicago green roofs. Determining which specific environmental filters and/or species traits are being acted upon will require further investigation.
The managers of the food roofs reported no observed pollination deficits. However, while it is easy to determine if pollination is grossly insufficient (due to low yield or misshapen fruits), it can be harder to assess if yields could further improve with additional pollination services (Webber et al., 2020). The roofs had a paucity of Bombus and Xylocopa, which are thought to be the main pollinators of many crops in the region because of their large size and ability to sonicate flowers (Cooley and Vallejo-Marín, 2021). However, other sonicating genera such as Melissodes, Agapostemon and the large, non-sonicating Megachile texana may be filling the gap (Cardinal et al., 2018). Many of the crops present were obligate outcrossers that require bees for pollination. Some crops, like those in the family Solanaceae, require specialized pollinators, while others require a great number of individual visits. Thus, the amounts and types of pollination deficits, as well as the need for specific types of pollinators must be addressed in future research.
As the number of green roof managers opting to grow bee-pollinated crops increases, understanding the potential limitations to attracting sufficient bee diversity becomes more relevant. It is important for organizations seeking to grow crops on roofs to understand that there may be pollination deficits, especially in dense urban areas. Thus, green roof managers should ensure that there are resources for pollinators all season, especially when crops are not in bloom.
Data availability statement
The original contributions presented in the study are included in the article/Supplementary material, further inquiries can be directed to the corresponding author.
Author contributions
JR and GC conceptualized the experiment. JR and JH performed fieldwork and processed specimens. NF curated the data. NF and GC did the analyses. All authors worked on the writing and editing of the draft. All authors contributed to the article and approved the submitted version.
Funding
JR was supported through Saint Louis University's Knoedler undergraduate research fund.
Acknowledgments
We wish to thank Urban Harvest STL and their staff, especially Mary Ostafi, for providing access to the locations. Josh Marino assisted with fieldwork. Emily Morris, Rena Schmidt, and Rebecca Tonietto provided valuable comments on the manuscript.
Conflict of interest
The authors declare that the research was conducted in the absence of any commercial or financial relationships that could be construed as a potential conflict of interest.
Publisher's note
All claims expressed in this article are solely those of the authors and do not necessarily represent those of their affiliated organizations, or those of the publisher, the editors and the reviewers. Any product that may be evaluated in this article, or claim that may be made by its manufacturer, is not guaranteed or endorsed by the publisher.
Supplementary material
The Supplementary Material for this article can be found online at: https://www.frontiersin.org/articles/10.3389/frsc.2023.1100470/full#supplementary-material
References
Arduser, M. (2020). Identification and ecology of tallgrass prairie bees. In: Handbook for Workshop Held January 6–10, 2020. St. Louis: Saint Louis University.
Ascher, J. S., and Pickering, J. (2021). Discover Life Bee Species Guide and World Checklist (Hymenoptera: Apoidea: Anthophila). Available online at: https://www.discoverlife.org/mp/20q?search=Apoidea
Belcher, R. N., Fornasari, L., Menz, S., and Schroepfer, T. (2018). Birds use of vegetated and non-vegetated high-density buildings—a case study of Milan. J. Urban Ecol. 4, juy001. doi: 10.1093/jue/juy001
Brown, K. H., and Jameton, A. L. (2000). Public health implications of urban agriculture. J. Public Health Pol. 21, 20–39. doi: 10.2307/3343472
Byers, H. L., McHenry, L. J., and Grundl, T. J. (2020). Increased risk for lead exposure in children through consumption of produce grown in urban soils. Sci. Total Environ. 743, 140414. doi: 10.1016/j.scitotenv.2020.140414
Camilo, G. R., Muñiz, P. A., Arduser, M. S., and Spevak, E. M. (2017). A checklist of the bees (Hymenoptera: Apoidea) of St. Louis, Missouri, USA. J. Kansas Entomol. Soc. 90, 175–188. doi: 10.2317/0022-8567-90.3.175
Cardinal, S., Buchmann, S. L., and Russell, A. L. (2018). The evolution of floral sonication, a pollen foraging behavior used by bees (Anthophila). Evolution 72, 590–600. doi: 10.1111/evo.13446
Chao, A., and Jost, L. (2012). Coverage-based rarefaction and extrapolation: standardizing samples by completeness rather than size. Ecology 93, 2533–2547. doi: 10.1890/11-1952.1
Colla, S., Willis, E., and Packer, L. (2009). Can green roofs provide habitat for urban bees (Hymenoptera: Apidae)? Cities Environ. 2, 1–12. doi: 10.15365/cate.2142009
Cooley, H., and Vallejo-Marín, M. (2021). Buzz-pollinated crops: a global review and meta-analysis of the effects of supplemental bee pollination in tomato. J. Econ. Entomol. 114, 505–519. doi: 10.1093/jee/toab009
Cristiano, E., Deidda, R., and Viola, F. (2021). The role of green roofs in urban Water-Energy-food-ecosystem nexus: a review. Sci. Total Environ. 756, 143876. doi: 10.1016/j.scitotenv.2020.143876
da Silva, C. R. B., Beaman, J. E., Dorey, J. B., Barker, S. J., Congedi, N. C., Elmer, M. C., et al. (2021). Climate change and invasive species: a physiological performance comparison of invasive and endemic bees in Fiji. J. Exp. Biol. 224, jeb230326. doi: 10.1242/jeb.230326
Dvorak, B., and Volder, A. (2010). Green roof vegetation for North American ecoregions: a literature review. Landsc. Urban Plan. 96, 197–213. doi: 10.1016/j.landurbplan.2010.04.009
East-West Gateway Council of Governments. (2017). One Meter Urban Land Cover. Available online at: https://www.ewgateway.org/research-center/gis-maps/ (accessed November 11, 2022).
Fitch, G., Wilson, C. J., Glaum, P., Vaidya, C., Simao, M.-C., and Jamieson, M. A. (2019). Does urbanization favour exotic bee species? Implications for the conservation of native bees in cities. Biol. Lett. 15, 20190574. doi: 10.1098/rsbl.2019.0574
Getter, K. L., and Rowe, D. B. (2006). The role of extensive green roofs in sustainable development. Hort. Sci. 41, 1276–1285. doi: 10.21273/HORTSCI.41.5.1276
Greenleaf, S. S., Williams, N. M., Winfree, R., and Kremen, C. (2007). Bee foraging ranges and their relationship to body size. Oecologia 153, 589–596. doi: 10.1007/s00442-007-0752-9
Gruver, A., and CaraDonna, P. (2021). Chicago bees: urban areas support diverse bee communities but with more non-native bee species compared to suburban areas. Environ. Entomol. 50, 982–994. doi: 10.1093/ee/nvab048
Hofmann, M. M., and Renner, S. S. (2018). Bee species recorded between 1992 and 2017 from green roofs in Asia, Europe, and North America, with key characteristics and open research questions. Apidologie 49, 307–313. doi: 10.1007/s13592-017-0555-x
Hsieh, T. C., Ma, K. H., and Chao, A. (2016). iNEXT: an R package for rarefaction and extrapolation of species diversity (Hill numbers). Methods Ecol. Evol. 7, 1451–1456. doi: 10.1111/2041-210X.12613
Kratschmer, S., Kriechbaum, M., and Pachinger, B. (2018). Buzzing on top: linking wild bee diversity, abundance and traits with green roof qualities. Urban Ecosyst. 21, 429–446. doi: 10.1007/s11252-017-0726-6
Ksiazek, K., Fant, J., and Skogen, K. (2012). An assessment of pollen limitation on Chicago green roofs. Landsc. Urban Plan. 107, 401–408. doi: 10.1016/j.landurbplan.2012.07.008
MacIvor, J. S. (2016). Building height matters: nesting activity of bees and wasps on vegetated roofs. Israel J. Ecol. Evol. 62, 88–96. doi: 10.1080/15659801.2015.1052635
MacIvor, J. S., and Lundholm, J. (2011). Insect species composition and diversity on intensive green roofs and adjacent level-ground habitats. Urban Ecosyst. 14, 225–241. doi: 10.1007/s11252-010-0149-0
MacIvor, J. S., Ruttan, A., and Salehi, B. (2015). Exotics on exotics: pollen analysis of urban bees visiting Sedum on a green roof. Urban Ecosyst. 18, 419–430. doi: 10.1007/s11252-014-0408-6
Madre, F., Vergnes, A., Machon, N., and Clergeau, P. (2013). A comparison of 3 types of green roof as habitats for arthropods. Ecol. Eng. 57, 109–117. doi: 10.1016/j.ecoleng.2013.04.029
Oksanen, J., Blanchet, F. G., Friendly, M., Kindt, R., Legendre, P., McGlinn, D., et al. (2019). Vegan: Community Ecology Package. Available online at: https://cran.r-project.org/web/packages/vegan/index.html
Parker, J., and Zingoni de Baro, M. E. (2019). Green infrastructure in the urban environment: a systematic quantitative review. Sustainability 11, 3182. doi: 10.3390/su11113182
Passaseo, A., Rochefort, S., Pétremand, G., and Castella, E. (2021). Pollinators on green roofs: diversity and trait analysis of wild bees (Hymenoptera: Anthophila) and hoverflies (Diptera: Syrphidae) in an urban area (Geneva, Switzerland). CATE 14, 1–22. doi: 10.15365/cate.0.2021.140201
R Core Team (2022). R: A Language and Environment for Statistical Computing. R Foundation for Statistical Computing, Vienna, Austria. Available online at: https://www.R-project.org/ (accessed November 11, 2022).
Roswell, M., Dushoff, J., and Winfree, R. (2021). A conceptual guide to measuring species diversity. Oikos 130, 321–338. doi: 10.1111/oik.07202
Russo, L., de Keyzer, C. W., Harmon-Threatt, A. N., LeCroy, K. A., and MacIvor, J. S. (2021). The managed-to-invasive species continuum in social and solitary bees and impacts on native bee conservation. Curr. Opin. Insect Sci. 46, 43–49. doi: 10.1016/j.cois.2021.01.001
Specht, K., Siebert, R., Hartmann, I., Freisinger, U. B., Sawicka, M., Werner, A., et al. (2014). Urban agriculture of the future: an overview of sustainability aspects of food production in and on buildings. Agric. Hum. Values 31, 33–51. doi: 10.1007/s10460-013-9448-4
Stoner, K. A. (2020). Pollination is sufficient, even with low bee diversity, in pumpkin and winter squash fields. Agronomy 10, 1141. doi: 10.3390/agronomy10081141
Tonietto, R., Fant, J., Ascher, J., Ellis, K., and Larkin, D. (2011). A comparison of bee communities of Chicago green roofs, parks and prairies. Landsc. Urban Plan. 103, 102–108. doi: 10.1016/j.landurbplan.2011.07.004
Wang, J. W., Poh, C. H., Tan, C. Y. T., Lee, V. N., Jain, A., and Webb, E. L. (2017). Building biodiversity: drivers of bird and butterfly diversity on tropical urban roof gardens. Ecosphere 8, e01905. doi: 10.1002/ecs2.1905
Wang, L., Wang, H., Wang, Y., Che, Y., Ge, Z., and Mao, L. (2022). The relationship between green roofs and urban biodiversity: a systematic review. Biodivers. Conserv. 31, 1771–1796. doi: 10.1007/s10531-022-02436-3
Webber, S. M., Garratt, M. P. D., Lukac, M., Bailey, A. P., Huxley, T., and Potts, S. G. (2020). Quantifying crop pollinator-dependence and pollination deficits: the effects of experimental scale on yield and quality assessments. Agric. Ecosyst. Environ. 304, 107106. doi: 10.1016/j.agee.2020.107106
Wojcik, V. A., and McBride, J. R. (2012). Common factors influence bee foraging in urban and wildland landscapes. Urban Ecosyst. 15, 581–598. doi: 10.1007/s11252-011-0211-6
Keywords: green roofs, food security, biodiversity, Hill numbers, pollination services
Citation: Riehn JK, Fogel NS, Hathaway JN and Camilo GR (2023) Bee diversity on urban rooftop food gardens. Front. Sustain. Cities 5:1100470. doi: 10.3389/frsc.2023.1100470
Received: 16 November 2022; Accepted: 10 January 2023;
Published: 03 February 2023.
Edited by:
Léo Correia da Rocha-Filho, Federal University of Uberlandia, BrazilReviewed by:
Leigh Whittinghill, Connecticut Agricultural Experiment Station, United StatesAnita Judit Grossmann, Technical University of Berlin, Germany
Copyright © 2023 Riehn, Fogel, Hathaway and Camilo. This is an open-access article distributed under the terms of the Creative Commons Attribution License (CC BY). The use, distribution or reproduction in other forums is permitted, provided the original author(s) and the copyright owner(s) are credited and that the original publication in this journal is cited, in accordance with accepted academic practice. No use, distribution or reproduction is permitted which does not comply with these terms.
*Correspondence: Nina S. Fogel, nina.fogel@slu.edu