- Department of Geography and Urban Studies, Temple University, Philadelphia, PA, United States
Nature-based and energy transition strategies are amongst most considerable solutions that are gaining popularity in recent years, especially in global north. This review provides a new approach to link these apparently independent concepts to highlight the potentials of coupled systems. Exploring common boundary concepts such as energy infrastructure, natural capital, land sink, ecosystem services etc. in both energy and environmental geography fields can facilitate our understanding toward potential contributions of nature-based solutions and energy transition for efficiency and sustainability goals. The results of this review can help to further develop robust coupled systems to deal with urban societal and environmental challenges such as land and energy scarcity. This review presents a variety of theoretical foundations and the rationales behind nature-based solutions and energy transition including socio-technical transitions, socio-ecological-technological, land-energy transition, and circular economy scholarships. Finally, by proposing future research directions, the role of each boundary concept in coupled NbS-ET systems is shown.
Introduction
Over 4 billion people live in cities today, with 62% (5.4 billion) of the global population anticipated to dwell in cities by 2035 (UN-Habitat, 2020). Urban land area is predicted to triple from 2000 to 2030 (d'Amoura et al., 2017). The rapid urbanization, happening at a rate never seen before will heavily impact local and regional ecosystem functions, and exacerbate climate change and global warming (Venter et al., 2020; Zuniga-Teran et al., 2020). In the case of global environmental challenges, urban climate-induced greenhouse gas (GHG) emissions, sea level rise and extreme heat among others must be tackled to meet the aim of Paris Agreement to limit mean global temperature increase to 1.5°C (IPCC, 2019). To achieve this goal, as academics and non-academics have emphasized, applying green pathways such as renewable energies and nature-based solutions can help reduce dependence on fossil fuels and CO2 emissions, respectively.
One of the recent approaches to reduce the negative effects of climate change is the use of nature-based solutions that is gaining considerable popularity among policy makers, planners, and urban ecologists worldwide. Nature-based solutions (NbS) such as green roofs, green facades, community gardens, bioswales, urban forests, green infrastructure, urban wetlands, and unmanaged green sites are seen as important promises for climate change adaptation in cities (Van Oijstaeijen et al., 2020; Castellar et al., 2021). While the design and implementation of NbS that are incompatible with the natural environment may have negative consequences for the ecosystem (e.g., afforestation with non-native species), well-planned NbS support a wide range of ecosystem services at different spatial levels which are critical in resolving anthropogenic concerns and facilitating the planning of sustainable and resilient urban areas (Alves et al., 2019; Babí Almenar et al., 2021; Girardin et al., 2021; Pan et al., 2021). Moreover, transitioning from fossil fuel-based consumptions to renewable energies such as wind, solar and thermal is another example of natural solutions in which an integration of technological innovations and alternative energies can is used to prevent harming the environment. Energy transition can help societies achieve sustainable goals through providing a wide variety of ecosystem services such as food supply, energy poverty eradication, energy justice among many more (Kabeyi and Olanrewaju, 2022).
However, it is supposed that technological innovation, particularly in the realm of ET and NbS, should deal with some barriers and challenges such as land acquisition, geographical implications (e.g., transportation, location, and scale), techno-economic, and socio-technical barriers (Bardi, 2013; Bridge et al., 2013; Cherpp et al., 2018; Bogdanov et al., 2019). For example, although wind and photovoltaics are two modern renewables that produce electric power, these technologies are difficult to integrate into an urban energy system in which the concepts of flexibility, low cost, and transportability are fundamental. Also, as Scheidel and Sorman (2012) state, the land speculation and consequently land rush will happen due to the need of large ET sectors to be settled. The land scarcity is expected to be raised considering the growing demand for alternative energies expansion worldwide. Also, implementing NbS is sometimes associated with some serious barriers in different contexts such as the lack of budget or technical assistance which has made it very difficult to achieve the results in some circumstances. For example, Toxopeus and Polzin (2021) show that NbS implementation face two major financial barriers including a need for balancing and coordinating public and private financing for a specific urban NBS and determining how to evaluate and account for NBS's numerous benefits. Thus, dealing with technological and financial challenges needs an integrative and comprehensive approach regarding effective resource management.
Transiting from a fossil fuel-based economy toward net-zero carbon cities can be supported by using a coupled NbS-ET systems in which both renewable energies and nature-based solutions are integrated. This approach strongly supports a need for sustainable land management (SLM). Earth Summit (1992) defines SLM as “the use of land resources, including soils, water, animals and plants, for the production of goods to meet changing human needs, while simultaneously ensuring the long-term productive potential of these resources and the maintenance of their environmental functions” (Motavalli et al., 2013). Implementing an integrative natural-engineering systems can optimize SLM in such a rapid urbanization which help reduce air pollution, biodiversity loss, and heat island effects in cities. However, although a considerable number of studies have utilized NbS to facilitate climate change mitigation and adaptation policies (Depietri and McPhearson, 2017; Nesshöver et al., 2017; Seddon et al., 2020), focus on sustainability transition aspects of NBS with the purpose of understanding theoretical foundations and concepts driving the NbS-based transition in urban areas remain limited only to a few peer-reviewed articles (Frantzeskaki et al., 2017; van der Jagta et al., 2020). On the other hand, albeit due to the similarity and transparency of the word “energy transition” with “sustainability transition”, there are several studies that show the significance of ET for accelerating the latter through using alternative resources from solar, wind, water, geothermal, waste, and biomass (Chen et al., 2019; Pietrosemoli and Rodríguez-Monroy, 2019). Thus, one idea which is rapidly growing among academic and non-academic is combining both urban natural climate solutions (e.g., wetlands and gardens) with renewable energy technologies (e.g., solar photovoltaic) in urban areas, an approach which we call it as “coupled NbS-ET systems” in this review.
In this paper, I bridge this gap by reviewing the literature to explore the most important common concepts and theoretical foundations to link NbS and ET for conceptualizing “coupled NbS-ET systems” approach in cities. The aim of this review is exploring the common boundary concepts (CBC) as intermediaries by which both nature-based solutions and ET can be linked. Star and Griesemer (1989) suggest that “boundary concepts” can be used to manage both variety of understanding and cooperation to solve challenges and achieve common goals between different disciplines and stakeholders. Boundary concepts are defined as “plastic enough to adapt to the needs and constraints of the several parties that employ them, yet robust enough to maintain a (common) identity” (Star and Griesemer, 1989, p. 393). In our case, CBC as intermediaries between NbS and ET are useful themes which help facilitate sustainability transition and climate adaptation through transdisciplinary negotiations and collaborations amongst society, nature, and technology. For instance, the role of boundary concepts such as ecosystem services, socio-ecological concepts, and green infrastructure in creating common understanding among different fields of geography and planning has been articulated in some previous studies (Opdam et al., 2015; Razzaghi-Asl, 2015; Schleyer et al., 2017; Smets et al., 2020). This understanding can help clarify the NbS concept's applicability and capacity to address ET concerns in communities.
The objectives of this review are to: (i) explore the current debate of combined NbS and ET (ii) detect key mechanisms and CBC by which two approaches can be linked and (iii) suggest promising relationships to assess the implications of coupled NbS-ET systems approach. The additional goals of this review are to highlight the applicability of available concepts and theoretical foundations to highlight the importance of “coupled NbS-ET systems” in the face of climate change. This new approach may help planners and decision-makers find suitable and efficient ways to better utilize the combined alternatives of natural resources and renewable energy technologies in the case of land scarcity and growing human impacts on natural environment. It concludes by some important research gaps and questions.
An Overview of the State of the Art
Nature-Based Solutions
Nature-based solutions (NbS) are a relatively new notion used to support sustainable development and climate mitigation challenges, notably in cities (Nesshöver et al., 2017; van der Jagta et al., 2020). Despite some ambiguities in the definition of this concept (see also Castellar et al., 2021), NbS can be defined as “the potential power of nature and the keys it can provide to global challenges in fields such as climate change, food security, social and economic development” (IUCN, 2020). According to this definition, NbS or recently “natural climate solutions” can offer multiple benefits for both climate change adaptation and mitigation challenges specifically through reducing the exposure to natural hazards and GHG reduction, respectively (Chausson et al., 2020; Seddon et al., 2020; Girardin et al., 2021). For example, NbS can contribute around 11 Gt CO2 e year-1 which is equivalent to about 20% of the mitigation needed between now and 2050 to keep global warming below 2°C (Griscom et al., 2017).
Moreover, NbS are more complex natural solutions in comparison to other nature-derived solutions (e.g., solar panels) which have ability to store carbon and regulate water flow and help communities reach sustainability transition in a faster and more resilient way (Stefanakis et al., 2021). According to Girardin et al. (2021), the promise of NbS is to reduce GHG emission through protecting and restoring ecosystems as well as improving land management which in turn results in natural cooling of the earth. The land sector including forests, wetlands, agriculture, and other land uses is responsible for about 24% of annual CO2 emissions (IPCC, 2014), so that land-based ecosystems as a strong strategy of NbS alone play a very decisive role in the carbon sequestration process of the planet's climate (Keesstra et al., 2018).
Energy Transition
According to IRENA (2021a) energy transition is defined as “a pathway toward transformation of the global energy sector from fossil-based to zero-carbon by the second half of this century. At its heart is the need to reduce energy-related CO2 emissions to limit climate change”. Carbon dioxide (CO2) emissions account for two-thirds of all greenhouse gas emissions (IPCC, 2014; Asarpota and Nadin, 2020). It is the key driver of ET worldwide in the face of what Mártil de la Plaza (2021) calls “perfect storm” in which fossil fuel depletion and increased energy consumption due to population growth has occurred. There is a growing interest in using alternative energies (e.g., wind, solar, etc.) to shift from a fossil fuel-based systems to a more efficient low-carbon energy system (Hoppe and de Vries, 2018; Gielena et al., 2019). Renewable energies are known as an alternative powerful tool to moderate societal and environmental challenges. As a new report from International Energy Agency (IEA) shows, renewable energy has shown to be the most resistant to COVID19 lockdown tactics yet (IEA, 2020), meaning that renewable power has been mostly unchanged, although demand for other renewable energy sources has decreased. They promise a safe, dependable, economical, and quickly deployable path to a low-carbon future that can accomplish over 90% of the energy-related CO2 emission reductions required to fulfill climate targets according to the REmap (IRENA, 2021b). However, renewable energy sources should be sustainable because they are obtained organically from ongoing energy flows in our environment. Renewable energy must be infinite and provide non-harmful delivery of environmental products and services to be sustainable (Owusu and Asumadu-Sarkodie, 2016).
Coupled NbS-ET Systems
Since NbS and ET are relatively new ideas, growing interest in both by policy makers and planners compels reflection on the interplay of these concepts, even though they are seemingly viewed as disparate approaches. Recent research has focused on the combination of different renewable energy technologies with a wide variety of green spaces and green infrastructure which constitute NbS in such a way that it can be efficient and less harmful for the environment (Figure 1). Benefits provided by natural mechanisms such as evapotranspiration would be helpful to better utilize the ET technologies. For example, a study by Shafique et al. (2020) shows that the combination of solar cells in green roofs (PV-green roof) can increase the benefits of green roofs for residents as well as the environment. Enhancing the power output of PV as well as urban heat island mitigation are some benefits of such combined systems. Results of a study in Zurich, Switzerland reveals that, as compared to a normal roof, green roofs can boost yearly PV energy yield by 1.8%, while cool roofs (an integrated green roof and solar panels as a niche) can raise it by 3.4% for a flat rooftop PV installation (Battista Cavadini and Cook, 2021). Burney et al. (2018) indicate the suitability of solar market gardens in northeast Benin, West Africa. It is defined as a technology and management package for women's agricultural organizations in Sub-Saharan Africa engaged in hand-watered horticultural produce. In this case both renewable solar energy and community or village gardens can be combined in a coupled system in which both green infrastructure and PV are interlinked.
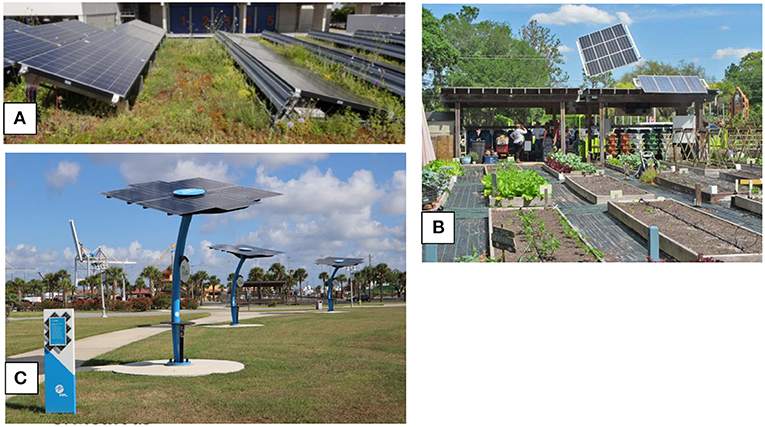
Figure 1. Diverse coupled nature-intensive systems. (A) PV-green roof (Baumann et al., 2016) (B) A local solar garden (sustany.org) (C) An urban park with solar panels (solarnow.fpl.com).
Moreover, O'Connor (2019) highlights the potential benefits of community solar gardens as a coupled NbS-ET systems for low-income communities through providing more equitable distribution of solar panels among these people. This approach is promising because it is crucial to increase the total land needed to install more solar panels as a reliable source of renewable energy in already constructed green spaces in neighborhoods or villages. In another technical study by Casierra-Martinez et al. (2020) indicates the usefulness of combining Solar photoreactor and constructed wetlands to enhance the wastewater treatment process. Also, as a study shows, it is possible to combine solar panels in urban green spaces to provide heating in cold days, cooling in summertime and lighting in night (Hakimizad et al., 2015). According to Randle-Boggis et al. (2020), solar parks provide ecological benefits, and they may assist ensure that the energy transition does not result in localized ecosystem damage and may even lead to increases in ecosystem health. However, there might be some tradeoffs between using combined systems. For example, Tasneem et al. (2020) investigate the suitability and efficiency of different models of urban wind farms and conclude that commercial wind farms cannot be replaced by urban wind farms. The performance of urban wind farms is limited by the chaotic and turbulent character of city wind. Our review shows that despite a growing literature on investigating about synergies and tradeoffs of coupled NbS-ET systems, there is a need to explore theoretical foundations and conceptual linkages to understand the real outcomes of such systems.
Methods
The literature review was mostly based on an inductive and snowballing approach (Badampudi et al., 2015), in which concepts and theoretical foundations linking NbS with ET in urban settings were found and categorized in the literature. A set of keywords was used to limit the search to studies that looked at NbS and ET in urban settings to create a “Start Set”. All searches were conducted using Scopus as the search engine, with the search field set to “keywords” and the document type set to article or review. To find relevant literature, five separate search queries were used, each with a different two-way and three-way combinations of (keyword category-related) search phrases (Query 1: Nature-based solutions AND Urban context; Query 2: Energy transition AND Urban context; Query 3: Nature-based solutions AND Energy transition; Query 4: Nature-based solutions AND Sustainability transition; Query 5: Nature-bases solutions AND Energy AND Climate change). Because the fields of NbS and ET have evolved mostly independently of one another, this technique was selected. Total 28 articles were chosen after an initial assessment of their relevance to our topic, to be further analyzed by author as start set. To consolidate and deepen the review's goal, the second round of searches was begun by setting search field to a two-way combination of (mechanism/nature-based solutions) OR (mechanism/energy transition) based on extracted mechanisms in the same database. As a result, further 39 articles were added and then examined. After the screening samples, total 67 articles were examined through qualitative content analysis (Mayring, 2000). Content analysis is a research approach for testing theoretical concerns and improving data comprehension in which a condensed number of concepts or categories characterizing a reality, a theory, or a study topic can be obtained (Elo and Kyngäs, 2008).
Results and Discussion
From the 68 articles it was possible to extract some basic theoretical concepts that define the mutual contribution of nature-based solutions to ET. To do this, an inductive approach to analyze different boundary concepts was applied to categorize some major theoretical foundations and frameworks as mechanisms by which we can realize the potential relationships between NbS and ET. The mechanisms and CBC intend to promote the use and development of coupled NbS-ET systems in different contexts. The mechanism identified in Table 1 are: socio-technical transition; land-energy relations; circular economy; and socio-ecological-technological systems. Table 1 intends to promote our knowledge about separates and quickly summarizes the mechanisms and CBC, which are described more below. “Mechanisms” are theoretical and conceptual foundations by which the two concepts can be implemented and linked. This is followed by more in-depth descriptions of the mechanisms and CBC, emphasizing the breadth of the literature, which spans several research traditions and epistemic logics. It is possible to identify socio-technical transitions and land-energy relations as the most frequently stated theoretical approaches in the literature by reviewing the results.
Socio-Technical Transition
As literature shows NbS and ET are innovative tools to address global societal and environmental challenges. Implementing these tools often is associated with urban transformation as a systematic social and technological change in planning and designing cities. In this regard, the concept of socio-technical transition is a relevant theory to conceptualize the potential contributions of both ET and NbS. As Lawhon and Murphy (2011) state through its multi-level conception of how societies might transition toward more sustainable futures, this theory provides a particularly valuable tool for geographers to address these challenges. Yet, socio-technical transition framework has been largely applied in the context of ET and less in NbS literature (Morgunova, 2021; Wu et al., 2021).
One of the most applicable frameworks that has been proposed in articulating socio-technical transition so far is the multi-level perspective (MLP). The MLP was originally created to explain and comprehend change processes (such as transition and transformation) in a complex system (Geels and Kemp, 2007). It is a framework for mapping and analyzing complex systems on three levels: socio-technical landscape (exogenous environment's pressure), socio-technical regime (a shared set of rules), and niche level (micro level innovations). For example, Morgunova (2021) shows that a greater attention on the socio-technical regime's capacities and traits can help with the transition to a more sustainable energy system. Likewise, using the multi-level approach as a framework for analysis in China, Xu (2021) concludes that coordination of interests during the ET, the rule of law in the energy sector, full life-cycle clean renewable energy generation, and the phase-out of excessive fossil fuel subsidies are central measures to sustainable ET. In another study done by Roesler (2018), MLP is applied to show the importance of community-led approach to energy transition projects such as bioenergy villages in Germany.
Secondly, Mitic-Radulovic and Lalovic (2021) have examined the suitability of MLP framework to analyze the application of co-created NbS in the urban planning process. They try to apply three components of MLP including urban planning systems as “socio-technical regime”, socio-political tensions and oppositions as “socio-technical landscape” and “linear parks” as niches in two case studies of Belgrade and Serbia. Dorst et al. (2021) conceptualizes the concept of “structural conditions” affecting the integration of NbS in urban development with a new framework “urban infrastructure regimes” adapted from “socio-political regime”. The findings of this study suggest that NBS necessitate a distinct understanding of socio-technical regimes more than other types of innovation. They propose that, in addition to the usual components of socio-technical regime frameworks, physical geographies be considered to better understand the hurdles to NBS development and prospects for enabling its wider application.
As literature indicates successful ET and cost-effective NbS are often associated with citizen participation (Van Ham and Klimmek, 2017; Ryghaug et al., 2018). Yet, as some studies state, emerging new technologies often face difficulties in gaining socio-cultural acceptability and deployment which need a social innovation approach or recently used term “sustainable innovation” notions (Hoppe and de Vries, 2018; Janssen et al., 2020). Desa and Jia (2020) define social innovation in relation to sustainability transition as “a set of multi-level mechanisms that facilitate sustainability transitions: across sectors, across social finance, across institutional structures, and through social ventures across geographic regions”. In this case, both ET and NbS as socio-technical innovations should involve people in co-creating and co-designing processes (Palomo et al., 2021). As several studies show, co-creation is one of the key sustainable principles for complex NbS and ET in which real environmental and societal challenges can be addressed by harnessing citizen participation (Mahmoud and Morello, 2018; IUCN, 2020; Ryszawska et al., 2021). Reviewing the current literature shows that co-creation is recognized as one of the most crucial parts of sustainability transition including both NbS implementation and ET planning. For instance, Sillak et al. (2021) propose a conceptual framework for assessing strategic co-creation in ET planning based on the role of actors (state, market, community, and third sector) in the various phases of co-creation, the use of activities (expectation alignment, social learning, resource acquisition, assessment, and evaluation) to foster transformative power; and the outcomes of co-creation (in terms of effectiveness, efficiency, and social acceptability). In addition, Co-creating of NbS and ET can help policy makers and planners achieve more resilient and sustainable urban areas aiming at reconnecting people with natural capitals or so-called “Living Labs (LLs)” due to its capacity to include different stakeholders' needs and expectations (Irene DeLosRíos-White et al., 2020). The European Commission defines Living Labs as “user-centered, open innovation ecosystems based on a systematic user co-creation approach integrating research and innovation processes in real life communities and settings. In practice, Living Labs place the citizen at the center of innovation, and have thus shown the ability to better mold the opportunities offered by new ICT concepts and solutions to the specific needs and aspirations of local contexts, cultures, and creativity potentials”.
Land-Energy Relations
The review found that the land scarcity appears to be one of the key drivers of ET and NbS implementation worldwide (Janssen et al., 2020). Due to a fast urban growth in many countries, public land in cities accounts for a very tiny proportion of total area in comparison to private property and it is suggested that many important issues, such as space for schools, hospitals, and recreation, should be considered for public land rather than NbS (Qiao et al., 2018). The large-scale NbS such as wetlands and detention basins need large swaths of land depending on their capacity (van de Ven et al., 2021). Renewable energy is scattered widely and unevenly throughout the land, and its ability to be harvested is largely dependent on specific physical, bio-physical, cultural, and social landscape qualities that may be significantly more prominent in some locations than in others (Nadaï and van der Horst, 2010; De Boer et al., 2018). As the solar power or other renewable energies development are rapidly growing, conflicts over land usage and environmental justice issues are gaining traction (Katkar et al., 2021; Sareen, 2021). The re-composition of socio-technical relations so-called “energy landscape” between landscape and energy might be viewed because of this spatial impact. As Silva and Sareen (2021) indicate in some cases in Portugal, multiple variables related to energy infrastructure changes, such as shifting land use, local cash flows for welfare functions, and altered landscapes, are important to local populations.
The transition from traditional fossil fuels or oil-based consumption to new alternative energies as well as the transformation of structural and gray infrastructure to NbS require a paradigm shift from producing “energy and services for landscape” to “energy and services from landscape”. Urban nature has strong potentials to support both above shifts through providing nature-intensive renewable energy sources and green large or small lands. As Thrän et al. (2020) state, new energy landscapes not only include traditional public or private lands but also contain new land potentials for cities such as contaminated lands, landfills, or brownfields. These sources of energy and ecosystem services can be appropriate for solar energy because they are not utilized and have no other productive purpose from a human perspective (EPA, 2012; van de Ven et al., 2021). For example, The U.S. Environmental Protection Agency (EPA) has provided a new plan called “RE-Powering” to turn contaminated lands, brownfields, and landfills to renewable energy developments (Figure 2). In the case of integrating renewable energy technologies with green vacant lands or grasslands as large scale NbS, a coupled NbS-ET can be imaginable.
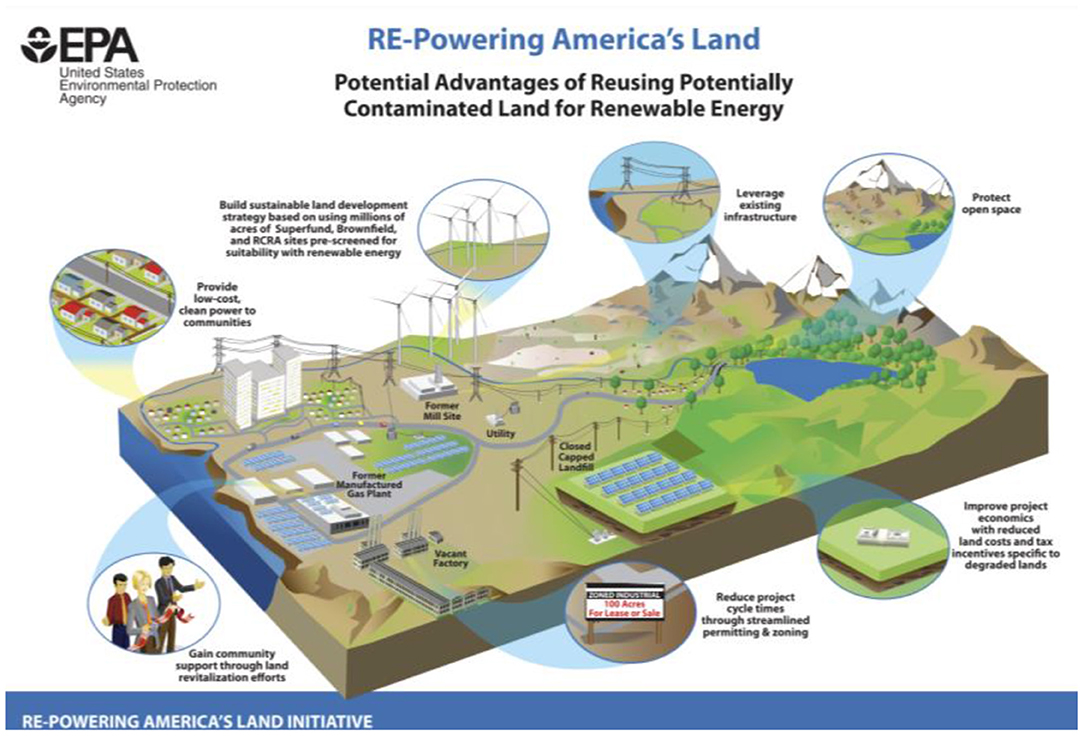
Figure 2. Schematic Re-powering America's land initiative (EPA, 2012).
Circular Economy
Circular economy (CE) or more recently “nature-based economy” (Rhodes et al., 2021) is defined as an “economic system that aims at minimizing waste and making the most of resources” (Langergraber et al., 2020). According to estimates, CE will reduce world energy production by 12.7%, assisting in the achievement of the Paris climate agreement (Chen and Kim, 2019). Indeed, at numerous levels and dimensions, energy transition and circular economic transition are inextricably linked. Circular economy, on the other hand, prioritizes renewable resources, such as renewable energy. For instance, the findings of a recent review in Africa show that a circular economy model could help with renewable energy adoption and transition (Mutezo and Muopo, 2021). Both energy transition and circular economy have common sustainability concern, creating two overlapping communities beneficial to other through creating pathways to a net-zero system. Circular economy can increase the efficient use of renewable energies and reduce the ecological footprint. It is noticeable that circular economy and ET models are considered as two pillars of resource management and low carbon (Okafor et al., 2021).
As this review shows, in a circular system, resource input and waste, emission, and energy leakage are minimized by slowing, closing, and narrowing energy and material loops (Pearlmutter et al., 2020). It is supposed that this approach can mitigate climate change through decarbonization, dematerialization and material management. In the framework of a green and circular economy, NbS can be considered as a reliable source for transiting from traditional carbon-based supply systems to a new renewable-oriented circular economy (Ramírez-Agudelo et al., 2021). Shifting to a circular economy with less waste, a more plant-based diet, and less resource overconsumption will free up land for carbon storage and biodiversity which can be facilitated by means of NbS (Seddon et al., 2020). NbS can supplement sector-level decarbonization efforts by neutralizing emissions that firms and organizations are unable to reduce due to technological constraints. In this regard, evidence-based outcomes show that NbS can help with the transition to a circular economy by offering extra benefits and ecosystem services in addition to more sustainable resource management (Stefanakis et al., 2021).
Natural and modified ecosystems as NbS can contribute significantly to climate change mitigation by acting as a “natural carbon sink”, absorbing and sequestering CO2 emissions (IUCN, 2020). Forests, wetlands, and oceans conservation, restoration, and sustainable ET play a key part in the proper functioning of the carbon cycle and the balanced regulation of the planet's climate. Some NbS solutions, like preserving existing wetlands, are primarily aimed at reducing greenhouse gas emissions. Others, such as regrowing clear-cut forests and restorative agriculture, actively remove CO2 from the sky. As some studies indicate, land-use and land-cover change, which includes deforestation and forest degradation, changes in agricultural practices, reforestation and afforestation, and changes in ecosystem management and fire control, is the most direct human cause of unbalanced land carbon (The Royal Society, 2001; Harper et al., 2018; Yue et al., 2020). NbS has the potential to expand the extent of land and ocean carbon sinks, as well as cut GHG emissions, making a significant contribution to climate change mitigation (Seddon et al., 2020). Land sinks are crucial resources for achieving a net-zero future due to their capacity to store and capture CO2 as they have eliminated 33% of anthropogenic emissions between 2009 and 2018 and they are also important for ET due to the strong demand for these technologies on land, as previously mentioned (Zhu et al., 2021). As a result, land may act as a potent carbon sink, absorbing carbon from the atmosphere and returning it to live flora and soils. Yet, although the possibility of nature-based solutions is exciting, it cannot be used as an urgent action to phase out of current energy practices or environmental damage in order to provide food and fuel.
Furthermore, one of the greatest contributions of circular economy to ET and NbS is dematerialization of natural resources in the face of “ecological overuse”. As Wackernagel et al. (2021) indicate, increased overuse has hastened the biosphere's ecological depletion, resulting in increased biodiversity loss, climate change, forest degradation, and freshwater scarcity. By progressively depleting natural capital, delayed impact and insufficient feedback aggravate future resource security issues. NbS can address these challenges through restoring and maintaining the water cycle, water and waste treatment, recovery and reuse, nutrient recovery and reuse, material recovery and reuse, food and biomass production, energy efficiency and recovery, and building system recovery. Moreover, although ET can help the dematerialization process through reducing a total resource flow for generating electricity (Watari et al., 2019), one of the most important unresolved issues of ET is waste management which could be facilitated by using similar natural climate solutions such as treatment wetlands. For example, as research show, the expansion of low-carbon energy generates new circular economy opportunities through waste-to-energy and the recycling of end-of-life energy items (e.g., retired photovoltaic panels), an action that requires enhanced resource governance approach (Watari et al., 2021). So, coupled NbS-ET technologies can facilitate the transition to circular economy with probably differing degrees and mechanisms that can be interlinked or mutually applicable.
Socio-Ecological-Technological Systems
As mentioned above, a symmetrical combination of society, technology and ecology's implications should be considered for conceptualizing the sustainability transition through NbS and ET concepts. Loorbach et al. (2017) distinguish three kinds of systems including socio-technical (e.g., energy), socio-economic (e.g., education) and socio-ecological (e.g., forestry). As Tzoulas et al. (2021) state NbS can be conceptualized as complex socio-ecological systems by which it can help understand of the importance of nature in urban areas. In this respect, the linkage between NbS as mainly socio-ecological systems and ET technologies as socio-technical systems can be investigated through an integrated framework called “socio-ecological-technical systems” (SETS). The urban SETS framework is a systems approach to urban constructed infrastructure that stresses the relevance of technology. This paradigm examines underlying social causes and acquired benefits in monitoring and intervention and conceptualizes nature-based projects as part of connected human and natural systems. Using the SETS paradigm to investigate the interactions between diverse green energy infrastructures and NbS in urban systems allows for equal attention on the coupling of social, ecological, and technical processes, which may aid in identifying barriers to and opportunities for urban sustainability transitions (McPhearson et al., 2016; Browna et al., 2021). For instance, turning vacant lots into solar farms need more attention when a network of green infrastructure such as urban wetlands are already present on the site. In this case, new renewable energy park can simultaneously benefit existing structures and facilities, nevertheless, it may harm urban ecology by producing unrecyclable materials.
Additionally, Keeler et al. (2019) propose a new framework to analyze the value of NbS including social factors (e.g., social norms, income, and demographics), ecological (e.g., vegetation structure, landscape position, and soil), and technological factors (e.g., existing infrastructure). This framework can help geographers, energy policy makers, and urban ecologists determine synergies and tradeoffs between potential NbS or ET services to optimize the final outcomes for urban nature. Yet, little research has been done on the application of this framework in the field of ET albeit with no direct SETS framing. For instance, Browna et al. (2021) propose a range of local strategies to reduce carbon emissions including rooftop solar and large-scale solar among others in consistent with our review, an analysis based on using SETS framework for localizing climate solutions. Also, it seems that this approach needs a transdisciplinary point of view to integrate not only different disciplines' aspects but also stakeholders' viewpoints in the transition process. For example, Park (2021) emphasizes on the role of social acceptance in this regard, with the increasing attention of policy makers and planners as well as NGOs to the application of NbS as facilitators of urban sustainability transition, adopting a transdisciplinary perspective has become one of the requirements for its realization which would be possible with defining practical CBC such as supporting ecosystem services.
Future Research Directions
Our comprehensive review reveals the most important theoretical foundations and common boundary concepts (CBC) to conceptualize coupled NbS-ET systems. Since this new approach has not been developed yet, its promise for a more sustainable and resilient natural infrastructure is remarkable. Our synthesis is aimed at exploring conceptual linkages between NbS and ET as two relatively new rapidly growing nature-intensive solutions that are supposed to be effective for climate change adaptation in cities. While the actual benefits of such systems limit to a few studies, theoretical evidence show that developing robust coupled systems (e.g., PV-green roofs) in urban areas can be significant. However, more investigation about potential synergies and tradeoffs between incorporating and co-locating NbS and ET types is needed. Also, as theories such as circular economy indicate, solar panels may have some detrimental effects in terms of waste disposal, it would be possible to further analysis the suitability of incorporating other renewable resources such as biomass and biothermal in nature-based solutions projects to create more sustainable coupled systems.
Several empirical and case study research on the potential of such systems for urban resilience and sustainability is needed. Table 2 shows some possible benefits may be addressed by coupled NbS-ET systems based on each CBC. When comparing different applicable CBC among the NbS and ET, they have a lot in common albeit with different processes and approaches. NbS can help to mitigate the negative effects of the energy industry by reducing energy use and capturing and storing carbon. Urban NbS such as urban parks, for example, might help to reduce an area's overall energy demand helping mitigate the 'urban heat island' effect. By shading building surfaces, deflecting solar radiation, and releasing moisture into the air, trees, green roofs, and other green NbS can help cool metropolitan areas (Menon and Sharma, 2021). NbS can also lower embodied emissions in urban development and infrastructure by employing renewable energies such as solar, wind or biothermal instead of using non-renewables materials like concrete and steel. Also, coupled NbS and ET systems may result in natural assets restoration and preservation through greenhouse gas (GHG) emissions reduction, and ecosystem protection.
Perhaps the lack of empirical and modeling research in the case of coupled systems is the most critical gap. So, it is recommended that the future research respond to some important questions regarding the applicability of coupled systems for sustainability transition and climate change mitigation. For example, can PV-green roofs help accelerate the sustainability transition in different socioeconomic conditions? To what extent such a system may contribute to air pollution or urban heat island effects? How can we prioritize co-locating the different types of renewable energies and ecosystem-based solutions to increase ecosystem services? Who will benefit from coupled systems for climate adaptation? What governance arrangements can be appropriate for coupled systems in different scales? Do scaling up and down of such systems contribute to more efficient and sustainable natural systems? This research calls for further research and investment in coupled NbS-ET systems to help determine the optimized and suitable models in different contexts and climate scenarios.
Conclusion
The current study has identified a set of CBC for conceptualizing the potential linkages between NbS and ET in urban areas based on a literature assessment on NbS and ET in cities. This approach certainly has its limits. It included only theoretical and conceptual frameworks as intermediaries between NbS and ET, nevertheless, there are some potential assessments (e.g., multi-scale integrated analysis of societal and ecosystem metabolism) or governance approaches (e.g., networked governance) which can be further investigated in this line of research.
Furthermore, the existing common frameworks and ideas of NbS and ET show striking similarities as well as significant distinctions. These findings focus on a need for applying different CBC based on empirical and modeling research for defining coupled NbS-ET systems rather than just analyses in each components' transition toward more low-carbon and nature-based cities. Clarifying the contributions of each concept for the other requires more in-depth analyses in terms of political economy and political ecology of nature-based energy systems as well as metabolic analysis of NbS in different urban areas and multi levels. However, both concepts have a remarkable contribution to climate change mitigation due to their potential to promote GHG and CO2 reduction as well as ecosystem restoration. Moreover, NbS and ETs are the kind of natural climate solutions by which policy makers and planners can achieve the Paris Agreement goal and sustainability transition notion. In addition, the contributions of this study would pave a new way to the combination of different types of ET and NbS in a time where interlinked social, ecological, and technological innovations require multifunctional and transdisciplinary solutions.
Author Contributions
The author confirms being the sole contributor of this work and has approved it for publication.
Conflict of Interest
The author declares that the research was conducted in the absence of any commercial or financial relationships that could be construed as a potential conflict of interest.
Publisher's Note
All claims expressed in this article are solely those of the authors and do not necessarily represent those of their affiliated organizations, or those of the publisher, the editors and the reviewers. Any product that may be evaluated in this article, or claim that may be made by its manufacturer, is not guaranteed or endorsed by the publisher.
References
Alves, A., Gersonius, B., Kapelan, Z., Vojinovic, Z., and Sanzhez, A. (2019). Assessing the Co-Benefits of green-blue-grey infrastructure for sustainable urban flood risk management. J. Environ. Manag. 239, 244–254. doi: 10.1016/j.jenvman.2019.03.036
Asarpota, K., and Nadin, V. (2020). Energy strategies, the urban dimension, and spatial planning. Energices 13, 3642. doi: 10.3390/en13143642
Atanasova, N., Castellar, J., Pineda-Martos, R., Elisabeth Nika, C., Katsou, E., Istenic, D., et al. (2021). Nature-based solutions and circularity in cities. Circ. Econ. Sustain. 1, 319–332. doi: 10.1007/s43615-021-00024-1
Babí Almenar, J., Elliot, T., Rugani, B., Philippe, B., Navarrete Gutierrez, T., Sonnemann, G., et al. (2021). Nexus between nature-based solutions, ecosystem services and urban challenges. Land Use Policy 100, 104898. doi: 10.1016/j.landusepol.2020.104898
Badampudi, D., Wohlin, C., and Petersen, K. (2015). “Experiences from using snowballing and database searches in systematic literature studies,” in EASE '15: Proceedings of the 19th International Conference on Evaluation and Assessment in Software Engineering, Vol. 17 (ACM), 1–10. doi: 10.1145/2745802.2745818
Bardi, U. (2013). The grand challenge of the energy transition. Front. Energy Res. 1, 2. doi: 10.3389/fenrg.2013.00002
Battista Cavadini, G., and Cook, L. M. (2021). Green and cool roof choices integrated into rooftop solar energy modelling. Appl. Energy 296, 117082. doi: 10.1016/j.apenergy.2021.117082
Baumann, T., Schär, D., Carigiet, F., and Dreisiebner, A. (2016). “Performance analysis of PV green roof systems,” in 32nd European Photovoltaic Solar Energy Conference and Exhibition (EU PVSEC 2016), Munich.
Bogdanov, D., Farfan, J., Sadovskaia, K., Aghahosseini, A., Child, M., Gulagi, A., et al. (2019). Radical transformation pathway towards sustainable electricity via evolutionary steps. Nat. Commun. 10, 1077. doi: 10.1038/s41467-019-08855-1
Bridge, G., Bouzarovski, S., Bradshaw, M., and Eyre, N. (2013). Geographies of ET: Space, place, and the low-carbon economy. Energy Policy 53, 331–340. doi: 10.1016/j.enpol.2012.10.066
Broto, V. (2017). Energy landscapes and urban trajectories towards sustainability. Energy Policy 108:755–764. doi: 10.1016/j.enpol.2017.01.009
Browna, M., Dwivedi, P., Mani, S., Matisoff, D., Mohan, J. E., Mullen, J., et al. (2021). A framework for localizing global climate solutions and their carbon reduction potential. PNAS 118, 31e2100008118. doi: 10.1073/pnas.2100008118
Burney, J., Phillips, S., and Lahl, J. (2018). Assessing the productivity and profitability of the Solar Market Garden. Dev. Eng. 3, 60–71. doi: 10.1016/j.deveng.2018.01.002
Calvert, K., Greer, K., and Maddison-MacFadyen, M. (2019). Theorizing energy landscapes for energy transition management: Insights from a socioecological history of energy transitions in Bermuda. Geoforum 102, 191–201. doi: 10.1016/j.geoforum.2019.04.005
Casierra-Martinez, H. A., Madera-Parraa, C. A., Vargas-Ramírezb, X. M., Caselles-Osorio, A., and Torres-López, W. A. (2020). Diclofenac and carbamazepine removal from domestic wastewater using a Constructed Wetland-Solar Photo-Fenton coupled system. Ecol. Eng. 153, 105699. doi: 10.1016/j.ecoleng.2019.105699
Castellar, J. A. C., Popartan, L. A., Pueyo-Ros, J., Atanasova, N., Langergraber, G., Säumel, I., et al. (2021). Nature-based solutions in the urban context: terminology, classification and scoring for urban challenges and ecosystem services. Sci. Total Environ. 779, 146237. doi: 10.1016/j.scitotenv.2021.146237
Chausson, A., Turner, B., Seddon, D., Chabaneix, N. A. J, Girardin, C., et al. (2020). Mapping the effectiveness of nature-based solutions for climate change adaptation. Global Change Biol. 26, 6134–6155. doi: 10.1111/gcb.15310
Chen, C., Xue, B., Cai, G., Thomas, H., and Stückrad, S. (2019). Comparing the energy transitions in Germany and China: synergies and recommendations. Energy Rep. 5, 1249–1260. doi: 10.1016/j.egyr.2019.08.087
Chen, W.-M., and Kim, C. (2019). Circular economy and energy transition: a nexus focusing on the non-energy use of fuels. Energy Environ. 30, 586–600. doi: 10.1177/0958305X19845759
Cherpp, A., Vinichenko, V., Jewell, J., Brutschin, E., and Sovacool, B. (2018). Integrating techno-economic, socio-technical, and political perspectives on national energy transitions: A meta-theoretical framework. Energy Res. Soc. Sci. 37, 175–190 doi: 10.1016/j.erss.2017.09.015
d'Amoura, C., Reitsma, F., Baiocchid, G., Barthele, S., Güneralp, B., Erb, K., et al. (2017). Future urban land expansion and implications for global croplands. PNAS 114, 8930–8944. doi: 10.1073/pnas.1606036114
De Boer, J., Zuidema, C., and Gugerell, K. (2018). New interaction paths in the energy landscape: the role of local energy initiatives. Landscape Res. 43, 489–502. doi: 10.1080/01426397.2018.1444154
Depietri, Y., and McPhearson, T. (2017). “Integrating the grey, green, and blue in cities: nature-based solutions for climate change adaptation and risk reduction,” in Nature-based Solutions to Climate Change Adaptation in Urban Areas, Theory and Practice of Urban Sustainability Transitions, eds N. Kabisch, H. Korn, J. Stadler, A. Bonn (Springer Open).
Desa, G., and Jia, X. (2020). Sustainability transitions in the context of pandemic: an introduction to the focused issue on social innovation and systemic impact. Agricul. Hum. Values 37, 1207–1215. doi: 10.1007/s10460-020-10129-2
Dodson, J. (2014). Suburbia under an energy transition: a socio-technical perspective. Urban Stud. 51, 1487–1505. doi: 10.1177/0042098013500083
Dorst, H., van der Jagta, A., Runhaarab, H., and Raven, R. (2021). Structural conditions for the wider uptake of urban nature-based solutions – a conceptual framework. Cities 116, 103283. doi: 10.1016/j.cities.2021.103283
Earth Summit. (1992). The Earth Summit: the United Nations Conference on Environment and Development (UNCED). Rio de Janeiro.
Elo, S., and Kyngäs, H. (2008). The qualitative content analysis process. J. Adv. Nurs. 62, 107–115 doi: 10.1111/j.1365-2648.2007.04569.x
EPA (2012). Re-Powering Program Overview. Available online at: https://19january2017snapshot.epa.gov/re-powering/re-powering-program-overview_.html (accessed May 4, 2022).
Frantzeskaki, N., Borgström, S., Gorissen, L., Egermann, M., and Ehnert, F. (2017). Nature-Based Solutions Accelerating Urban Sustainability Transitions in Cities: Lessons from Dresden. Genk; Stockholm: Springer Open.
Geels, F. W. (2019). Socio-technical transitions to sustainability: a review of criticisms and elaborations of the Multi-Level Perspective. Curr. Opin. Environ. Sustain. 39, 187–201. doi: 10.1016/j.cosust.2019.06.009
Geels, F. W., and Kemp, R. (2007). Dynamics in socio-technical systems: Typology of change processes and contrasting case studies. Technol. Soc. 29, 441–455. doi: 10.1016/j.techsoc.2007.08.009
Gielena, D., Boshella, F., Saygin, D., Bazilianc, M., Wagnera, N., and Gorini, R. (2019). The role of renewable energy in the global energy transformation. Energy Strat. Rev. 24, 38–50. doi: 10.1016/j.esr.2019.01.006
Girardin, C. A. J., Jenkins, S., Seddon, N., Allen, M., Lewis, S. L., Wheeler, C. E., et al. (2021). Nature-based solutions can help cool the planet - if we act now. Nature 593, 191–194. doi: 10.1038/d41586-021-01241-2
Griscom, B. W., Adams, J., Ellis, P. W., Houghton, R. A., Lomax, G. R., Miteva, D. A., et al. (2017). Natural climate solutions. PNAS 114, 11645–11650. doi: 10.1073/pnas.1710465114
Hakimizad, S., Razzaghi Asl, S., and Ghiai, M. (2015). A review on the design approaches using renewable energies in urban parks. Int. J. Renew. Energy Res. 5, 686–693. doi: 10.20508/ijrer.v5i3.2315.g6627
Hale, S. E., Folde, M., Unni, H. S., Sjødahl, E., Smebye, A., and Oen, A. (2021). From landfills to landscapes-Nature-based solutions for water management taking into account legacy contamination. Integr. Environ. Assess. Manage. 18, 99–107. doi: 10.1002/ieam.4467
Harper, A., Powell, T., Cox, P. M., House, J., Huntingford, C., Lenton, T. M., et al. (2018). Land-use emissions play a critical role in land-based mitigation for Paris climate targets. Nat. Commun. 9, 2938. doi: 10.1038/s41467-018-05340-z
Heaslip, E., and Fahy, F. (2018). Developing transdisciplinary approaches to community energy transitions: an island case study. Energy Res. Soc. Sci. 45, 153–163. doi: 10.1016/j.erss.2018.07.013
Holland, R. A., Beaumont, N., Hooper, T., Austen, M., Gross, R., Heptonstall, P., et al. (2018). Incorporating ecosystem services into the design of future energy systems. Appl. Energy 222, 812–822. doi: 10.1016/j.apenergy.2018.04.022
Hoppe, T., and de Vries, G. (2018). Social innovation and the energy transition. Sustainability 11, 141. doi: 10.3390/su11010141
IPCC (2014). “Climate change 2014: synthesis report,” in Contribution of Working Groups I, II and III to the Fifth Assessment Report of the Intergovernmental Panel on Climate Change, eds Core Writing Team, R. K. Pachauri and L. A. Meyer (Geneva: IPCC), 151.
IPCC (2019). Climate Change and Land: an IPCC Special Report on Climate Change, Desertification, Land Degradation, Sustainable Land Management, Food Security, and Greenhouse Gas Fluxes in Terrestrial Ecosystems, eds P. R. Shukla, J. Skea, E. Calvo Buendia, V. Masson-Delmotte, H.-O. Pörtner, D. C. Roberts, P. Zhai, R. Slade, S. Connors, R. van Diemen, M. Ferrat, E. Haughey, S. Luz, S. Neogi, M. Pathak, J. Petzold, J. Portugal Pereira, P. Vyas, E. Huntley, K. Kissick, M. Belkacemi, and J. Malley (IPCC).
IRENA (2021b). World Energy Transitions Outlook: 1.5°C Pathway. International Renewable Energy Agency: Abu Dhabi.
Irene DeLosRíos-White, M., Roebeling, P., Valente, S., and Vaittinen, I. (2020). Mapping the life cycle co-creation process of nature-based solutions for urban climate change adaptation. Resources 9, 39. doi: 10.3390/resources9040039
Janssen, N. G., Pereira Ramos, D., Linderhof, E. t., Polman, V., Laspidou, N., Fokkinga, C., et al. (2020). The climate, land, energy, water and food nexus challenge in a land scarce country: innovations in the netherlands. Sustainability 12, 10491. doi: 10.3390/su122410491
Kabeyi, M. J. B., and Olanrewaju, O. A. (2022). Sustainable energy transition for renewable and low carbon grid electricity generation and supply. Front. Energy Res. 9, 743114. doi: 10.3389/fenrg.2021.743114
Katkar, V. V, Sward, J. A., Worsley, A., and Zhang, K. M. (2021). Strategic land use analysis for solar energy development in New York State. Renew. Energy 173, 861–875. doi: 10.1016/j.renene.2021.03.128
Keeler, B. L., Hamel, P., McPhearson, T., Hamann, M. H., Donahue, M. L., Meza Prado, K. A., et al. (2019). Social-ecological and technological factors moderate the value of urban nature. Nat. Sustain. 2, 29–38. doi: 10.1038/s41893-018-0202-1
Keesstra, S., Nunes, J., Novara, A., Finger, D., Avelar, D., Kalantari, Z., et al. (2018). The superior effect of nature-based solutions in land management for enhancing ecosystem services. Sci. Total Environ. 610–611, 997–1009. doi: 10.1016/j.scitotenv.2017.08.077
Kim, H., and Park, H. (2018). PV waste management at the crossroads of circular economy and energy transition: the case of South Korea. Sustainability 10, 3565. doi: 10.3390/su10103565
Kisser, J., Wirth, M., De Gusseme, B., Van Eekert, M., Zeeman, G., Schoenborn, A., et al. (2020). A review of nature-based solutions for resource recovery in cities. Blue Green Syst. 2, 138–171. doi: 10.2166/bgs.2020.930
Langergraber, G., Pucher, B., Simperler, L., Kisser, J., Katsou, E., Buehler, D., et al. (2020). Nature-based solutions for creating a resourceful circular city. Blue-Green Syst. 2, 933. doi: 10.2166/bgs.2020.933
Lawhon, M., and Murphy, T. J. (2011). Socio-technical regimes and sustainability transitions: Insights from political ecology. Prog. Hum. Geograp. 36, 354–378. doi: 10.1177/0309132511427960
Loorbach, D., Frantzeskaki, N., and Avelino, F. (2017). Sustainability transition research: transforming science and practice for societal change. Annu. Rev. Environ. Resour. 42, 599–626. doi: 10.1146/annurev-environ-102014-021340
Lupp, G., Zingraff-hamed, A., Huang, J., Oen, A., and Pauleit, S. (2021). Living labs—a concept for co-designing nature-based solutions. Sustainability 13, 188. doi: 10.3390/su13010188
Mahmoud, I., and Morello, E. (2018). “Co-reaction pathway for urban nature-based solutions: testing a shared-governance approach in three cities and nine action labs,” in Smart and Sustainable Planning for Cities and Regions, eds A. Bisello D. Vettorato, D. Ludlow, and C. Baranzelli (Green Energy and Technology; Springer).
Mártil de la Plaza, I. (2021). The Energy Challenge: The Transition to a New Energy Model. Available online at: https://www.bbvaopenmind.com/en/science/environment/the-energy-challenge-the-transition-to-a-new-energy-model/#:~:text=Changing%20the%20energy%20model%20is,fossil%20fuel%2Dindependent%20energy%20mix
McPhearson, T., Pickett, S. T. A., Grimm, N. B., Niemela, J., Alberti, M., Elmqvist, T., et al. (2016). Advancing urban ecology toward a science of cities. BioScience 66, 3. doi: 10.1093/biosci/biw002
Menon, J., and Sharma, R. (2021). Nature-based solutions for co-mitigation of air pollution and urban heat in Indian cities. Front. Sustain. Cities 3, 705185. doi: 10.3389/frsc.2021.705185
Mitic-Radulovic, A., and Lalovic, K. (2021). Multi-level perspective on sustainability transition towards nature-based solutions and co-creation in urban planning of Belgrade, Serbia. Sustainability 13, 7576. doi: 10.3390/su13147576
Morgunova, M. (2021). The role of the socio-technical regime in the sustainable energy transition: a case of the Eurasian Arctic. .ive Indust. Soc. 8, 100939. doi: 10.1016/j.exis.2021.100939
Motavalli, P., Nelson, K., Udawatta, R., Jose, S., and Bardham, S. (2013). Global achievements in sustainable land management. Int. Soil Water Conserv. Res. 1, 1–10. doi: 10.1016/S2095-6339(15)30044-7
Mutezo, G., and Muopo, J. (2021). A review of Africa's transition from fossil fuels to renewable energy using circular economy principles. Renew. Sustain. Energy Rev. 137, 110609. doi: 10.1016/j.rser.2020.110609
Nadaï, A., and van der Horst, D. (2010). Introduction: landscapes of energies. Landscape Res. 35, 143–155. doi: 10.1080/01426390903557543
Nesshöver, C., Assmuth, T. N., Irvine, K., M., Rusch, G., Waylen, K. A., et al. (2017). The science, policy, and practice of nature-based solutions: an interdisciplinary perspective. Sci. Total Environ. 597, 1215–1227. doi: 10.1016/j.scitotenv.2016.11.106
O'Connor, M. (2019). All in the community: using community solar gardens to bring the benefits of renewable energy to low-income communities. Georgetown Environ. Law Rev. 31, 391–407.
Okafor, C., Madu, C., Ajaero, C., Ibekwe, J., and Otunomo, F. (2021). Situating coupled circular economy and energy transition in an emerging economy. AIMS Energy 9, 651–675 doi: 10.3934/energy.2021031
Opdam, P., Westernik, J., Vos, C., and De Vries, B. (2015). The role and evolution of boundary concepts in transdisciplinary landscape planning. Plan. Theory Pract. 16, 786. doi: 10.1080/14649357.2014.997786
Owusu, P. A., and Asumadu-Sarkodie, S. (2016). A review of renewable energy sources, sustainability issues and climate change mitigation. Cogent Eng. 3, 1167990. doi: 10.1080/23311916.2016.1167990
Palomo, I., Locatelli, B., Otero, I., Colloff, M., Crouzat, E., Cuni-Sanchez, A., et al. (2021). Assessing nature-based solutions for transformative change. One Earth 4, 730–741. doi: 10.1016/j.oneear.2021.04.013
Pan, H., Page, J., Cong, C., Barthel, S., and Kalantar, I. Z. (2021). How ecosystems services drive urban growth: Integrating nature-based solutions. Anthropocene 35, 100297. doi: 10.1016/j.ancene.2021.100297
Park, E. (2021). Social acceptance of renewable energy technologies in the post-fukushima era. Front. Psychol. 11, 612090. doi: 10.3389/fpsyg.2020.612090
Pearlmutter, D., Theochari, D., Nehls, T., Pinho, P., Piro, P., Korolova, A., et al. (2020). Enhancing the circular economy with nature-based solutions in the built urban environment: green building materials, systems, and sites. Blue-Green Systems 2, 46–72. doi: 10.2166/bgs.2019.928
Pietrosemoli, L., and Rodríguez-Monroy, C. (2019). The Venezuelan energy crisis: Renewable energies in the transition towards sustainability. Renew. Sustain. Energy Rev. 105, 415–426. doi: 10.1016/j.rser.2019.02.014
Qiao, X., Kristoffersson, A., and Randrup, B. T. (2018). Challenges to implementing urban sustainable stormwater management from a governance perspective: a literature review. J. Clean. Prod. 196, 943–952. doi: 10.1016/j.jclepro.2018.06.049
Ramírez-Agudelo, N. A., de Pablo, J., and Roca, E. (2021). Exploring alternative practices in urban water management through the lens of circular economy–a case study in the Barcelona metropolitan area. J. Clean. Prod. 329, 129565. doi: 10.1016/j.jclepro.2021.129565
Randle-Boggis, R. J., White, P. C. L., Cruz, J. G., Parker, H., Montag, J. M. O., and Scurlock, A. (2020). Armstrong Realizing co-benefits for natural capital and ecosystem services from solar parks: a co-developed, evidence-based approach. Renew. Sustain. Energy Rev. 125, 109775. doi: 10.1016/j.rser.2020.109775
Razzaghi-Asl, S. (2015). Boundary concepts in trans-disciplinary approach to environmental design in Iran, from the viewpoints of professors. Armanshahr 7, 211–219.
Rhodes, M., and McQuaid, S., Gemma Donnelly-Cox. (2021). Social innovation and temporary innovations systems (TIS): insights from nature-based solutions in Europe. Soc. Enterp. J. doi: 10.1108/SEJ-01-2021-0001. [Epub ahead of print].
Rink, D., and Schmidt, C. (2021). Afforestation of urban brownfields as a nature-based solution. Experiences from a Project in Leipzig (Germany). Land 10, 1–17. doi: 10.3390/land10090893
Roesler, T. (2018). Community resources for energy transition: implementing bioenergy villages in Germany. Area. 51, 268–276. doi: 10.1111/area.12444
Ryghaug, M., Moe Skjølsvold, T., and Heidenreich, S. (2018). Creating energy citizenship through material participation. Soc. Stud. Sci. 48, 283–303. doi: 10.1177/0306312718770286
Ryszawska, B., Rozwadowska, M., Ulatowska, R., Pierzchała, M., and Szymanski, P. (2021). The power of co-creation in the energy transition—DART model in citizen energy communities projects. Energies 14, 5266. doi: 10.3390/en14175266
Sareen, S. (2021). Energy infrastructure transitions and environmental governance. Local Environ. 26, 323–328. doi: 10.1080/13549839.2021.1901270
Scheidel, A., and Sorman, A. (2012). Energy transitions and the global land rush: Ultimate drivers and persistent consequences. Global Environ. Change 22, 588–595. doi: 10.1016/j.gloenvcha.2011.12.005
Schleyer, C., Lux, A., Mehring, M., and Görg, C. (2017). Ecosystem services as a boundary concept: arguments from social ecology. Sustainability 9, 1107. doi: 10.3390/su9071107
Seddon, N., Chausson, A., Berry, P., Girardin, C. A. J., Smith, A., and Turner, B. (2020). Understanding the value and limits of nature-based solutions to climate change and other global challenges. Phil. Trans. R. Soc. B 375, 20190120. doi: 10.1098/rstb.2019.0120
Shafique, M., Luo, X., and Zuo, J. (2020). Photovoltaic-green roofs: a review of benefits, limitations, and trends. Solar Energy 202, 485–497. doi: 10.1016/j.solener.2020.02.101
Sillak, S., Borch, K., and Sperling, K. (2021). Assessing co-creation in strategic planning for urban energy transitions. Energy Res. Soc. Sci. 74, 101952. doi: 10.1016/j.erss.2021.101952
Silva, L., and Sareen, S. (2021). Solar photovoltaic energy infrastructures, land use and sociocultural context in Portugal. Local Environ. 26, 347–363. doi: 10.1080/13549839.2020.1837091
Smets, J., De Blust, G., Verheyden, W., Wanner, S., Van Acker, M., and Turkelboom, F. (2020). Starting a participative approach to develop local green infrastructure, from boundary concept to collective action. Sustainability 12, 10107. doi: 10.3390/su122310107
Star, S. L., and Griesemer, J. (1989). Institutional ecology, ‘translations' and boundary objects: amateurs and professionals in Berkeley's Museum of vertebrate zoology, 1907-39. Soc. Stud. Sci. 19, 387–420. doi: 10.1177/030631289019003001
Stefanakis, A., Calheiros, S. C., and Nikolaou, I. C. (2021). Nature-based solutions as a tool in the new circular economic model for climate change adaptation. Circ. Econ. Sustain. 1, 303–318. doi: 10.1007/s43615-021-00022-3
Tasneem, Z., Al Noman, A., Das, S. K., Saha, K., Islam, R., Ali, F., et al. (2020). An analytical review on the evaluation of wind resource and wind turbine for urban application: Prospect and challenges. Dev. Built Environ. 4, 100033. doi: 10.1016/j.dibe.2020.100033
The Royal Society (2001). The Role of Land Carbon Sinks in Mitigating Global Climate Change. London: The Royal Society.
Thrän, D., Gawel, E., and Fiedler, D. (2020). Energy landscapes of today and tomorrow. Energ. Sustain. Soc. 10, 43. doi: 10.1186/s13705-020-00273-2
Toxopeus, H., and Polzin, F. (2021). Reviewing financing barriers and strategies for urban nature-based solutions. J. Environ. Manag. 289, 112371. doi: 10.1016/j.jenvman.2021.112371
Tzoulas, K., Galan, J., Venn, S., Dennis, M., Pedroli, B., Mishra, H., et al. (2021). A conceptual model of the social–ecological system of nature-based solutions in urban environments. Ambio 50, 335–345. doi: 10.1007/s13280-020-01380-2
van de Ven, D. J., Capellan Peréz, I., Arto, I., Cazcarro, I., de Castro, C., Patel, P., et al. (2021). The potential land requirements and related land use change emissions of solar energy. Sci. Rep. 11, 2907. doi: 10.1038/s41598-021-82042-5
van der Jagta, P. N., Ravena, R., Dorsta, H., and Runhaara, H. (2020). Nature-based innovation systems. Environ. Innov. Soc. Trans. 35, 202–216. doi: 10.1016/j.eist.2019.09.005
Van Ham, C., and Klimmek, H. (2017). “Partnerships for nature-based solutions in urban areas - showcasing successful examples,” in Nature-based Solutions to Climate Change Adaptation in Urban Areas, Theory and Practice of Urban Sustainability Transitions, eds N. Kabisch, H. Korn, J. Stadler, A. Bonn (Springer Open).
Van Oijstaeijen, W., Van Passel, S., and Cools, J. (2020). Urban green infrastructure: a review on valuation toolkits from an urban planning perspective. J. Environ. Manag. 267, 110603. doi: 10.1016/j.jenvman.2020.110603
Venter, Z. S., Hjertager Krog, N., and Barton, N. D. (2020). Linking green infrastructure to urban heat and human health risk mitigation in Oslo, Norway. Sci. Total Environ. 709, 136193. doi: 10.1016/j.scitotenv.2019.136193
Wackernagel, M., Hanscom, L., Jayasinghe, P., Lin, D., Murthy, A., Neill, A., et al. (2021). The importance of resource security for poverty eradication. Nat. Sustain. 4, 731–738. doi: 10.1038/s41893-021-00708-4
Watari, T., Benjamin, C., McLellan, G. D., Dominish, E., Yamasue, E., and Nansai, K. (2019). Total material requirement for the global energy transition to 2050: a focus on transport and electricity. Resour. Conserv. Recycl. 148, 91–103. doi: 10.1016/j.resconrec.2019.05.015
Watari, T., Nansai, K., Nakajima, K., and Giurco, D. (2021). Sustainable energy transitions require enhanced resource governance. J. Clean. Prod. 312, 127698. doi: 10.1016/j.jclepro.2021.127698
Wu, Z., Shao, Q., Su, Y., and Zhang, D. (2021). A socio-technical transition path for new energy vehicles in China: A multi-level perspective. Technol. Forecast. Soc. Change 172, 121007. doi: 10.1016/j.techfore.2021.121007
Xu, S. (2021). The paradox of the energy revolution in China: a socio-technical transition perspective. Renew. Sustain. Energy Rev. 137, 110469. doi: 10.1016/j.rser.2020.110469
Yue, C., Ciais, P., Houghton, R. A., and Nassikas, A. (2020). Contribution of land use to the interannual variability of the land carbon cycle. Nat. Commun. 11, 3170. doi: 10.1038/s41467-020-16953-8
Zhu, L., Ciais, P., Bastos, A., Ballantyne, A., Chevallier, F., Gasser, T., et al. (2021). Decadal variability in land carbon sink efficiency. Carbon Balance Manage 16, 15. doi: 10.1186/s13021-021-00178-3
Keywords: nature-based solutions, energy transition, sustainability transition, coupled systems, climate mitigation, common boundary concepts
Citation: Razzaghi Asl S (2022) Re-powering the Nature-Intensive Systems: Insights From Linking Nature-Based Solutions and Energy Transition. Front. Sustain. Cities 4:860914. doi: 10.3389/frsc.2022.860914
Received: 24 January 2022; Accepted: 26 May 2022;
Published: 22 June 2022.
Edited by:
Amalia Zucaro, Energy and Sustainable Economic Development (ENEA), ItalyReviewed by:
Marco Casazza, University of Salerno, ItalyFanxin Meng, Beijing Normal University, China
Copyright © 2022 Razzaghi Asl. This is an open-access article distributed under the terms of the Creative Commons Attribution License (CC BY). The use, distribution or reproduction in other forums is permitted, provided the original author(s) and the copyright owner(s) are credited and that the original publication in this journal is cited, in accordance with accepted academic practice. No use, distribution or reproduction is permitted which does not comply with these terms.
*Correspondence: Sina Razzaghi Asl, tuk98809@temple.edu