- Environmental Technology and Management, Department of Management and Engineering, Linköping University, Linköping, Sweden
Multi-utility tunnel (MUT) have received increasing attention as an alternative method for installing subsurface infrastructure for the distribution of electricity, telecommunications, water, sewage and district heating. MUTs are described as a potentially more sustainable technology than conventional open-cut excavation (OCE), especially if the entire life cycle of these cable and pipe networks is taken into account. Based on an extensive review of the academic literature, this article aims to identify and critically examine claims made about the pros and cons of using MUT for the placement of subsurface infrastructure. Identified claims are mapped, and their validity and applicability assessed. These claims are then analyzed from a sustainability perspective, based on the three sustainability dimensions and a life cycle perspective. The results show that a variety of advantages and disadvantages of using MUTs for subsurface infrastructure are highlighted by the articles, but several of these are without any empirical support. When some form of empirical support is presented, it usually comes from case-specific analyses of MUTs, and the applicability in other MUT projects is seldom discussed. Economic performance is the sustainability dimension that has received the most attention, while environmental performance has not been analyzed in the reviewed literature, which is a major limitation of the current knowledge. In summary, the knowledge about the sustainability performance of using MUTs for subsurface infrastructure is still limited and incoherent. In order to increase the knowledge, this article points out the importance of new case studies, in which the sustainability consequences of using MUTs for subsurface infrastructure are mapped and evaluated by combining both quantitative and qualitative assessment methods.
Introduction
In order to meet global challenges such as climate change and resource scarcity, it has become increasingly urgent to develop cities in a more sustainable direction. An important part of such development that seldom receives attention concerns the management of their underground (Hunt et al., 2014). This subsurface space is used for hosting a range of different constructions such as cellars, garages, subways, road tunnels and utilities. In cities, the subsurface utility infrastructure often takes up most of this space and these cables and pipes for distribution of utilities such as electricity, telecommunications, water, sewage and district heating constitutes the technical backbone of the city (Halfawy et al., 2008; Hojjati et al., 2017). Without sufficient and continuous flows of these utilities, many of the everyday activities in the city will not function properly. Careful planning and development of these infrastructures are therefore fundamental to achieve sustainable urban development (Pandit et al., 2017; Chester, 2019).
Due to the adoption of short-term planning cycles and requirements for the lowest initial construction costs, the most common technology to install subsurface infrastructure is open-cut excavation (OCE) or trenching (Canto-Perello and Curiel-Esparza, 2013). OCE involves the excavation of a shaft in which the different cables and pipes are placed before the soil masses are refilled and the site restored. The main advantage of this technique is that it has been used for a long time, and therefore, there is an elaborate workflow for this type of job, including well-established procedures for planning and installation (Canto-Perello and Curiel-Esparza, 2006). One major disadvantage of OCE, however, is that it leads to low accessibility to the cable and pipe infrastructure as they are buried directly in the ground. Any need for inspection, maintenance or renewal, therefore, relies on excavation to access the networks. This recurrent need for excavation during the lifetime of the infrastructure is costly, puts constraints on urban land use, disturbs city life, traffic and businesses and generates environmental impacts related to the use of machinery, transports, handling of excavated masses, site restoration and re-asphalting (Hunt et al., 2014).
Another problem with OCE is that the city underground gradually gets crowded with different cables and pipes, especially since the network parts taken out of use are often left behind in their subsurface location (Krook and Baas, 2013). This is often referred to as “the spaghetti problem”, and over time it makes it increasingly costly and difficult to access the networks for maintenance or renewal (Luttikhuis, 1992). The placement of cables and pipes directly in the soil also leads to significant wear due to corrosion and ground movements, which potentially shortens their service lifetime and causes increased risks for acute disruptions in the distribution of their essential utilities.
These problems with OCE have contributed to the development of alternative methods in terms of so-called trenchless technologies (TTs) (Ariaratnam et al., 1999; Tighe et al., 2002). TT is a collective name for techniques where the subsurface infrastructure is installed or maintained without any need for excavation. One type of TT is to build a tunnel of concrete, steel or plastic in which the utility pipes and cables are placed. The multi-utility tunnel, or MUT, is one of several different names for such tunnels, where the networks for distribution of water, sewage, electricity, communication, and district heating are collocated in a protected indoor environment. In a general sense, MUT is defined as “any system of underground structure containing one or more utility service which permits the placement, renewal, maintenance, repair or revision of the service without the necessity of making excavation: this implies that the structures are traversable by people and, in some cases, traversable by some vehicle as well” (Hunt et al., 2014, p.18). Such a definition signifies that there is a great variation between different types of MUTs in terms of their size, construction, functions and utilities (Luo et al., 2020). This article, however, focuses on MUTs particularly used for subsurface infrastructure and as an alternative to the conventional technology of OCE. For such applications of MUTs, the collocation of several cable and pipe networks is essential to effectively reduce the total need for excavation work in relation to maintenance and renewal of the city's subsurface infrastructures (Hunt et al., 2014).
Beyond that the utilization of such MUTs reduces the need for excavation in urban areas; they can also potentially address several other sustainability challenges of OCE. Since the cables and pipes are placed in a protected indoor-like environment, their service life can be expected to increase, and the collocation of many subsurface infrastructures in such a defined and traversable space can both limit constraints on urban land use and solve “the spaghetti problem”.
The previous research on MUTs for subsurface infrastructure consists of scattered studies from all over the world that focus on different aspects of using this technology in varying contexts and project settings (e.g., Riera and Pasqual, 1992; Hunt et al., 2014; Zhang et al., 2016; Valdenebro and Gimena, 2018). These studies often present MUT as a more sustainable alternative than OCE for managing the city's subsurface infrastructure:
“The utility tunnel is potentially one of the most sustainable urban underground infrastructures and must, therefore, be taken into account in urban planning…” (Cano-Hurtado and Canto-Perello, 1999, p.388).
“The use of urban utility tunnels (UUTs) is, without a doubt, the most sustainable solution to face a changing future as a result of the digital revolution in which we live.” (Valdenebro et al., 2019, p. 39).
There are many similar statements about MUTs' sustainability performance in the literature. Such statements claiming positive impact of products or processes on at least one of the three pillars of sustainability (economic, social and environmental) are often called sustainability claims. Such claims are increasingly addressed in sustainability, food and marketing research (see e.g. Cummins et al., 2014; Alevizou et al., 2015; Gupta and Chauhan, 2021; Lucas et al., 2021; van Doorn et al., 2021). So far, the support for the different sustainability claims made for MUTs, and whether this technology is better than OCE for placement of subsurface infrastructure, have not been systematically examined. This makes it difficult to identify limitations and gaps in current knowledge and leads to difficulties in deciding which aspects should be particularly focused by future research in order to improve the understanding of MUTs' sustainability performance and thus be able to strengthen the sustainability claims. It is indeed a complex task to evaluate the sustainability of such technologies for the placement of subsurface infrastructure. This since the choice between MUT or OCE does not only affect the owners of the cable and pipe networks and their installation, maintenance and renewal activities but has much broader consequences on the surrounding city life and community (Hojjati et al., 2018). Furthermore, the long service life of such infrastructures also adds significant uncertainties regarding their sustainability as many things may happen and change during their life cycle (Frangopol et al., 2004).
This article aims to assess the knowledge contributions and limitations of previous research regarding the sustainability of using MUTs as an alternative to OCE for subsurface infrastructure in urban areas. It does this by identifying the different sustainability claims that have been raised in the scientific literature and evaluating their validity and applicability. It then categorizes the knowledge contributions and limitations of these claims in relation to the three sustainability dimensions and the different life cycle phases of subsurface infrastructure. Based on the findings of this analysis, essential research areas and needs for enhancing the current knowledge level about the sustainability performance of MUTs as an alternative to OCE for placement of subsurface utility infrastructure are discussed.
Methods
The study was performed in two steps. The first step consisted of the selection of literature for the review, including the identification of sustainability claims in these studies (Figure 1). The second step involved mapping and further analysis of the identified sustainability claims in terms of their validity and applicability. This assessment formed the basis for the analysis of MUT sustainability and a discussion on how future research on the topic can be developed to fill the identified knowledge gap.
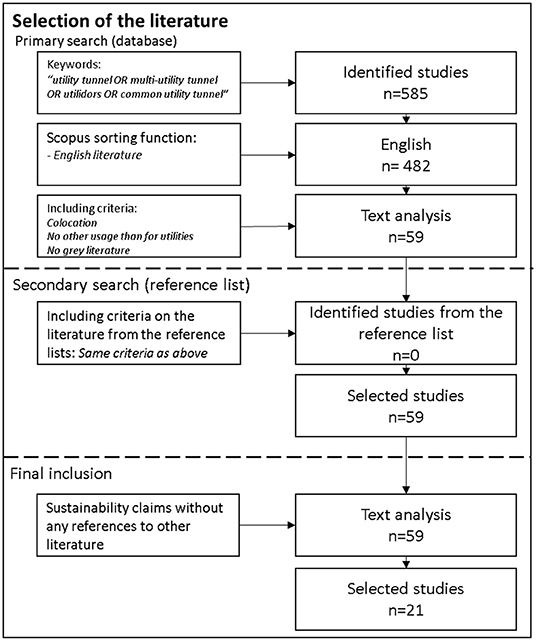
Figure 1. Flow diagram describing the literature search and selection procedure. “n” is the number of articles selected in every step.
Selection of the Literature
The selection of the relevant literature for the analysis was based on steps following different selection criteria (Figure 1).
The primary search consisted of three steps identifying publications that focused on MUTs and then excluded those that were not written in English, those that were not scientific publications and those that did not primarily focus MUTs for collocation of several wires- and pipe-based infrastructure systems. Scopus, a multi-disciplinary database for scientific articles published after 1960, was used for the primary search and the literature search was conducted in the spring of 2020. The used search bar was “utility tunnel OR multi-utility tunnel OR utilidors OR common utility tunnel”, which reflects that a MUT can be referred to in different ways. Common synonyms are “utilidors”, and “common utility tunnel”, but sometimes only “utility tunnel” is used. This search yielded 585 publications in total. This was followed by the exclusion of all non-English literature, achieved with the help of the language sorting mechanism of Scopus and resulting in the exclusion of 103 articles.
The secondary search consisted of reading the abstracts, keywords and conclusions of the articles to decide if they should be included in the review. To be included, the publications had to be scientific (journal articles, conference papers, books or book articles) and focus on MUTs for subsurface infrastructure. Given the fundamental importance of collocation to effectively avoid excavation during maintenance and renewal of the city's different cable and pipe networks (Hunt et al., 2014), only articles on MUTs for more than one type of utility were included. Based on these criteria, 59 articles were selected for the in-depth text analysis. Inspired by Pinior et al. (2017), an additional literature search was then done based on the reference lists from the selected literature, but it did not yield any additional articles to include in the review as the additional papers were either already identified in the primary search or excluded because of the selection criteria. The excluded articles are either about MUT that only contains one type of subsurface infrastructure or articles about tunnels used for other applications than the one focused on in this study. Numerous of the yielded articles focused on tunnels for car traffic or subways, and they were excluded as such tunnels do not constitute a viable alternative to using OCE for the placement of subsurface infrastructure. These types of tunnels also have completely different effects on society and are considerably larger than tunnels designed for hosting different cable and pipe networks. Among the excluded articles were also some studies on MUTs that were installed using a tunnel boring machine. Such tunnels are much larger and used for applications other than subsurface infrastructure such as transportation and are thereby also not a viable alternative to OCE.
The final inclusion step was based on the analysis of the 59 articles, where sustainability claims in this analysis defined as “a concrete statement about the sustainability consequences of using MUT instead of OCE” were sought. The occurrence of this type of claim was used as the final selection criterion, which led to exclusion of articles that focused on soil conditions (Il'ichev et al., 2011), BIM technologies (Wu et al., 2018; Ge and Xu, 2019; Hu and Zhang, 2019) and seismic performance (Chen et al., 2010, 2018; Jiang et al., 2010; Wang et al., 2017) of using MUTs without discussing the implications on sustainability. After this final selection stage, 21 articles remained for further analysis.
Analytical Approach
The analysis was performed in two steps (Figure 2), the first step consisted of mapping and categorization of the sustainability claims followed by an analysis of their validity and applicability and started with sorting the different sustainability claims in the articles to produce an overview. They were sorted into three categories, “additional underground construction”, “access without excavations” and “indoor-like environment”, which reflect fundamental traits of MUTs. This proved a practical way of sorting the different aspects raised in the literature and useful for presenting the claims in a systematic manner. Despite the relatively distinct character of these categories, some overlaps occurred between them.
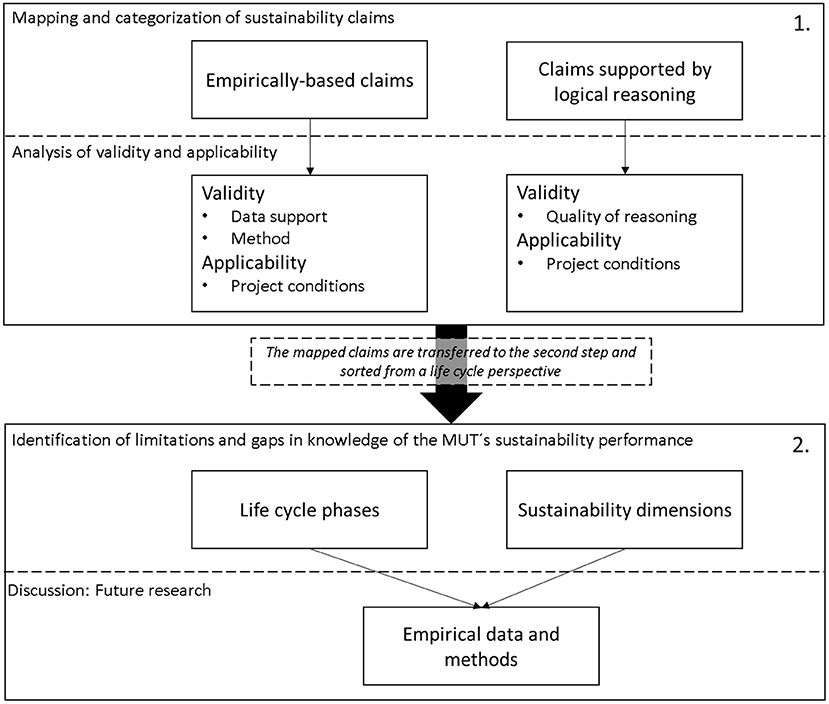
Figure 2. Scheme for the analytical approach of the review. The analysis consisted of two steps, where the first (1) focused on the validity and applicability of identified claims in the articles, and the second (2) on which life cycle phases and sustainability dimensions the previous claims addressed.
The identified sustainability claims in the articles were presented with different degrees of support. For reflecting these differences, the claims were sorted into claims that had been empirically studied to some extent, and claims only supported by the authors' logical reasoning. There were several types of logical reasoning in the analyzed articles. One of the most common ways of reasoning about the MUT's benefits was to describe the reported problems with using OCE and then claim that the MUT will solve some of these problems (e.g., excavations in the urban area). Other ways included applying observations in studies in related areas (e.g., working in a confined space) to MUTs, and just presenting the authors' thoughts without any empirical support. The validity, meaning that the claims are well-grounded, sound and correct, was investigated through an assessment of the support presented in the articles in terms of the applied data, methods and analytical approaches. Claims that had not been empirically studied but were based on different types of reasoning could, however, not be assessed in this way due to the lack of data and method descriptions. For such claims, the assessment of the validity instead focused on the authors' ability to present convincing arguments for their statements.
The applicability, the extent to which these claims are valid in other cases, was then addressed. These types of infrastructure projects are very sensitive to site and project-specific conditions such as soil conditions, the surrounding built environment, capacity needs, traffic intensity and surface layer (Curiel-Esparza and Canto-Perello, 2012). This means that a claim can be valid in one specific case but not in others. There can also be significant differences in the management and regulation of infrastructure utilities in different regions, which can affect the applicability of the claim in question (Hojjati et al., 2017). Therefore, it is useful to assess the general applicability of the claims and to what extent the presented empirical support or reasoning is dependent on such site, project or regionally contingent conditions.
In the second step of the analysis, the contributions of the claims to knowledge and understanding of MUT's sustainability performance were addressed. The claims were synthesized and assessed from both a life cycle perspective and in relation to the three pillars of sustainability (Basiago, 1999), which both are well-established frameworks for sustainability assessment. The three interconnected sustainability pillars, the economic, the social and the environmental, sometimes referred to as dimensions (Stirling, 1999) or stool legs (Dawe and Ryan, 2003) is the dominating framework for sustainability assessment (Purvis et al., 2019), while Life cycle assessment (LCA) was originally developed as an environmental assessment tool, but broader Life cycle sustainability assessments (LCSA) including economic and social consequences have gradually become common.
In practice, it can often be difficult to consistently separate these sustainability dimensions from each other and a particular quality of MUTs can generate impacts within all three dimensions. This categorization was done based on what specific consequences were studied or pronounced in the reviewed articles. For instance, the case when it comes to the potential of avoiding excavation work in urban areas through the use of MUTs. Excavation in the urban area have impacts on all the three sustainability dimensions but the selected articles do not address all the potential impact.
The Selected Literature
The frequency of published articles with sustainability claims for MUTs has increased in recent years. Most of the selected articles are published after 2010, but the oldest is from 1992. The majority of the studies is from Spain (15 articles), and only two other countries are represented in the selection, three articles from the UK and three from China. The narrow geographical scope of the articles identified during the selection process indicates that MUTs' sustainability performance is not a well-researched area. A number of MUT projects carried out around the world are reported, but sustainability performance has not been evaluated in the majority of these cases. MUTs can have different shapes, and most studied tunnels are rectangular and built-in concrete. The type and number of utilities placed in the MUT also differ in various cases. Some applications only host telecommunications and electrical infrastructures, while others involve multiple pipes and wires to accommodate, for example, water, sewage, electricity, telecommunications and district heating.
The 21 selected articles have different types of articles and topics (Table 1). Five major study focus areas can be identified: method development, working environment and safety, describing the MUT concept, sustainable infrastructure and case descriptions. Six articles focus on method development, of which three deal with methods for facilitating decisions on the type of technology to use for the installation of underground utilities, while the other articles focus on risk assessment or methods for valuing underground space. Five articles focus on the working environment and safety aspects of using MUTs, particularly the risks of working with cable- and pipe-based infrastructure in such confined spaces. These articles address which aspects should be considered to facilitate the work in the MUT and which safety risks should be taken into account.
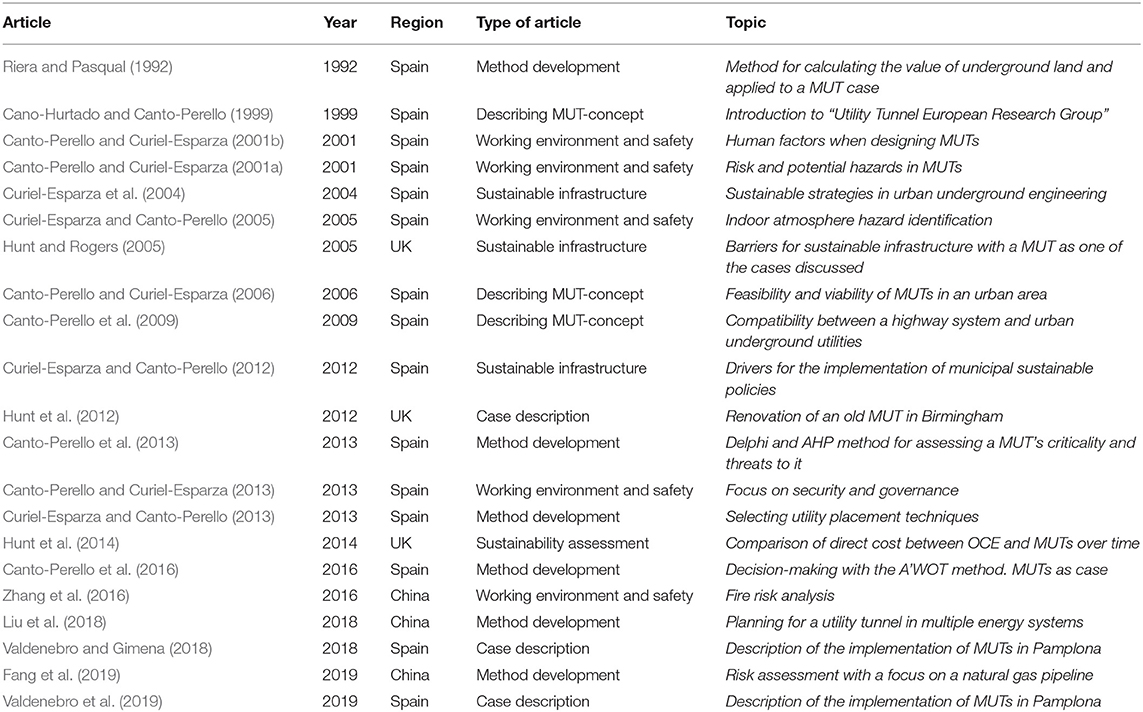
Table 1. Overview of the selected literature for this review with a presentation of the year of publication, the origin of the university for the main author, type of article and topic.
The MUT is a seldom-used technology compared to OCE, and, therefore, few articles (4) present general descriptions and discussions of this technology. These articles address, for example, the feasibility of using MUTs in an urban area and the increased use of MUTs in China. Three of the selected articles have a strong focus on sustainable infrastructure and use MUTs as a case to discuss general sustainability issues related to urban underground or subsurface infrastructure management. They all discuss sustainability and, to some extent, the MUT's performance, but only one study attempts to assess its sustainability performance.
Finally, three articles focus on specific cases where MUTs have been used. Two of these address a MUT construction in Pamplona, Spain, where this technology was used in a major revitalization/renovation project that ran from 2001–2009 (Valdenebro and Gimena, 2018; Valdenebro et al., 2019), while the third focuses on the renovation of a 50-year-old MUT placed in a university campus in Birmingham (Hunt et al., 2012). In addition to these two cases, the MUT installation that was part of a major urban transformation project before the 1992 Olympics in Barcelona has been studied (Riera and Pasqual, 1992).
MUT Sustainability Claims
The reading of the selected articles provided a gross list of claims concerning the MUT's sustainability performance. In Figure 3, the identified claims in the articles are sorted into three main categories: “additional underground construction”, “access without excavations” and “indoor environment”. Additional underground construction refers to sustainability claims related to the construction and installation of the tunnel used for the utilities. This construction is not used for OCE since the utilities are placed directly in the soil. In total, eight of the reviewed articles have contributed to this category.
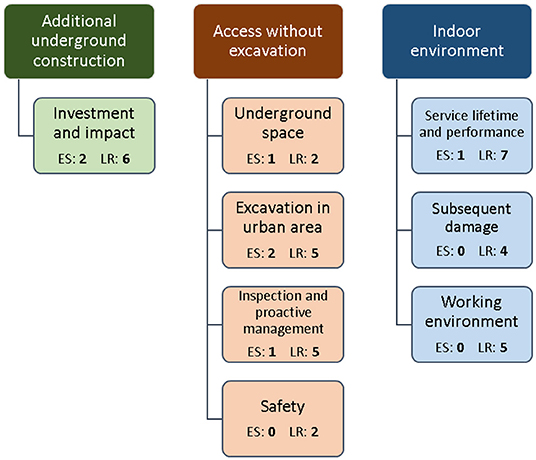
Figure 3. Sustainability claims sorted into three categories. ES is the number of articles that present empirical support for their claims, and LR is the number of articles that support their claims with logical reasoning.
The secondary category of claims, access without excavation, is connected to how the tunnel construction gives the utility owners access to the pipes and wires without any need for excavations. This accessibility potentially leads to a variety of pros and cons, and therefore the different claims are further divided into the subcategories of underground space (three articles), excavation in urban area (7 articles), inspection and proactive management (six articles) and safety (two articles). Underground space refers to the potential space-saving feature of MUTs. Since the utilities can be placed more densely in a MUT, the underground space can be used for other purposes. Excavation in the urban area includes claims of additional benefits owing to the reduced need for excavations in connection with maintenance when using MUTs. The increased accessibility to the utilities in MUTs is also central for the claims in the subcategory inspection and proactive management. When the utilities are placed in an accessible space as in a MUT, inspection becomes much easier by making it possible to continuously assess the quality of pipes and wires and manage the utilities more proactively. This accessibility is, however, not only a positive feature of MUTs as the risk for different types of sabotage can become higher than in connection with OCE, which is a claim that is categorized under safety.
The third category, indoor environment, includes claims connected to how when using MUTs, the utilities are placed in an indoor-like environment inside the tunnel and not directly in the soil as for OCE. This category has three subcategories: service lifetime and performance (eight articles), subsequent damage (four articles) and working environment (five articles). The indoor-like environment of the utilities affects their durability and performance over time, and claims linked to this are categorized under service lifetime and performance. Claims dealing with increased risks for damage of the utilities when placing them in the same confined space are categorized as subsequent damage. If, for instance, a water main starts leaking, it may also negatively impact the other utilities in the MUT. Some articles also stress that conducting maintenance and renewal of cable and pipe based infrastructure in confined spaces such as in a MUT can be a risk for the workers, and these claims are categorized in working environment.
Additional Underground Construction
In total, eight articles present claims connected to differences in construction costs between MUT and OCE. Only two of these have used empirical support (ES) for their reasoning (Figure 3), while the rest base their claims on some kind of logical reasoning (LR). However, regardless of the support for these different claims, there seems to be a consensus that using MUTs to install cable- and pipe-based infrastructure is more costly than using traditional technologies such as OCE (Riera and Pasqual, 1992; Cano-Hurtado and Canto-Perello, 1999; Canto-Perello and Curiel-Esparza, 2006, 2013; Canto-Perello et al., 2009; Curiel-Esparza and Canto-Perello, 2013; Liu et al., 2018; Valdenebro and Gimena, 2018). Riera and Pasqual (1992) and Valdenebro and Gimena (2018) are the only articles that present empirical support for such estimates from real cases of MUTs installed in Barcelona and Pamplona, respectively. According to Riera and Pasqual, a MUT is 80% more expensive than OCE, while Valdenebro and Gimena find it to be 50% more expensive. How these estimates have been made, however, is not well described in these studies, and the transparency regarding the employed data and assessment methods can thus be characterized as low. The authors appear to have followed their respective cases in detail, and thus it is reasonable to believe that they have had access to the costs for the MUT installations. Both these studies compared the costs for MUTs to OCEs, but the hypothetical OCE cases that have been used for comparison are not described at all. Given that the costs for such infrastructure projects can vary significantly depending on project-specific conditions (Kleiner et al., 2001; Rogers et al., 2012), this lack of detail makes it difficult to know how the cost differences presented in these studies would apply to other cases where other conditions prevail.
A distinct difference between the MUT installations in Barcelona and Pamplona is that the Barcelona construction was part of a major urban transformation project, while the Pamplona installation was connected to a renovation of existing networks. This difference is important since installation in the existing urban environment requires that the work must adapt to minimize disruptions to the functioning of the city, in contrast to larger conversion projects that do not have to make such considerations and therefore can be carried out with fewer disturbances. Building in an existing environment also demands temporary solutions to ensure a continuous supply of infrastructure services during the entire construction process. The relative cost of using MUT instead of OCE in Barcelona was higher compared to Pamplona despite the complexity of doing construction of utilities in existing environment. Project-specific conditions is not described enough in the articles to explain this cost difference.
Access Without Excavation
One distinct difference between MUTs and OCEs concerns the accessibility of the utilities without the need for excavation. Riera and Pasqual (1992), Cano-Hurtado and Canto-Perello (1999) and Valdenebro and Gimena (2018) all claim that underground space can be saved by using MUTs, and if this space is monetized, the MUT's competitiveness will be significantly improved, but only Riera and Pasqual (1992) present empirical support for this claim. Their study uses a new method for assessing and implementing the value of underground space for projects in an urban setting. The MUT construction in Barcelona was used as a case to test this valuation method and assess the economic performance of a project. The authors calculated how much space could be saved with a MUT, but the description of how the calculations were done lacks detail. This makes it difficult to assess the claim's credibility, and since it is only built upon one case, it is also difficult to understand its applicability in other cases of MUT constructions. Furthermore, it is not clear how much underground space the alternative OCE placement has been assumed to occupy.
The avoidance of excavations in urban areas not only brings the potential benefit of saving underground space; it can also reduce costs for maintenance and limit other negative consequences of excavation such as disturbances in the city life via traffic shutdowns, reduced mobility, wear on paved surfaces and reduced accessibility to businesses and stores (Cano-Hurtado and Canto-Perello, 1999; Canto-Perello et al., 2009, 2016; Curiel-Esparza and Canto-Perello, 2012, 2013; Liu et al., 2018; Valdenebro et al., 2019). There are seven articles that discuss this claim, but only Hunt et al. (2014) and Canto-Perello et al. (2016) present some empirical support for it. Canto-Perello et al. (2016) support the claim that using MUTs reduces the traffic shutdowns due to the avoidance of excavations in the urban area using the results from a survey involving 10 experts. However, the selection of these experts is not presented and motivated, and it is, therefore, hard to assess their knowledge and experience of MUTs. This leads one to question both the validity and applicability of this claim. Hunt et al. (2014), on the other hand, compared the life cycle costs of MUTs and OCEs, including the higher cost for installation of MUTs and the costs related to the current need for excavation work connected to OCE placements. The analysis of the latter was based on a simplified approach based on the costs related to different numbers of excavations during the life cycle of the cable and pipe based infrastructure. The study concluded that the MUT has good economic performance when used in the right locations (dense urban areas) and hosting as many types of utilities as possible. The disadvantage of this simplified analytical approach is that the actual amount of excavation that can be expected during the life cycle of utilities in OCE placements is very uncertain and can vary widely from case to case.
Higher levels of accessibility can also change the utility owners' ability to monitor, maintain and renew the pipe and wire infrastructures. This access to the infrastructure means that they, at a low cost, can continuously inspect and perform proactive maintenance (Cano-Hurtado and Canto-Perello, 1999; Canto-Perello et al., 2009, 2013, 2016; Valdenebro and Gimena, 2018; Valdenebro et al., 2019). The articles that bring up this benefit only present it as a potential one that may increase the security of the distribution and do not discuss its further implications for the management of the infrastructures. Canto-Perello et al. (2016) is the only article that presents empirical support for the benefits of proactive management in terms of the above-mentioned expert judgments. In essence, proactive management is mainly described as a benefit for the utility owners' ability to manage the networks, but that this can also lead to a more secure distribution when positive consequences for the inhabitants are not addressed.
Increased accessibility to the utilities may not only be positive; there is also a risk that unauthorized people gain access to the networks, and this may lead to sabotage, vandalism or other undesirable impacts on the utilities (Canto-Perello and Curiel-Esparza, 2013; Canto-Perello et al., 2013). This claim is only presented as a potential risk in the articles, without any kind of empirical support regarding the probability or frequency of such undesirable consequences.
Indoor Environment
When using MUT, the cables and pipes are placed in an indoor environment, unlike OCE, where the networks are surrounded by soil. According to Cano-Hurtado and Canto-Perello (1999), Canto-Perello and Curiel-Esparza (2013) and Canto-Perello et al. (2016), the surrounding soil means that the pipes are subject to wear and risk damage from soil movements and excavations, causing needs for maintenance and repair. For MUTs, the number of maintenance interventions is claimed to decrease by 70–80% (Riera and Pasqual, 1992; Curiel-Esparza and Canto-Perello, 2012). However, how this estimate has been done or where it comes from is not presented. According to Hunt et al. (2012), it is not only the number of emergency maintenance interventions that decrease with the use of MUTs; the lifetime of the utilities can also be extended. Although it is reasonable to believe that the durability of the utilities is improved in such a protected indoor environment, this claim lacks empirical support, and there are no experiences of how much the service life can actually be increased. Only Canto-Perello et al. (2016) present some empirical support in the form of the earlier discussed expert opinions.
The literature does not only claim that the placement of the networks in an indoor environment is entirely positive, but it also brings up that such a common space for the utilities can pose risks to the cables and pipes (Curiel-Esparza et al., 2004; Canto-Perello and Curiel-Esparza, 2006; Valdenebro and Gimena, 2018; Fang et al., 2019). For example, if a water line breaks, there is a risk that the water will damage other utilities. This could potentially knock out several types of infrastructure services at the same time. In the literature, such events are described as a potential risk, but none of the studies has investigated how important this risk is nor its potential consequences. Similar risks also exist for cable and pipes that are placed with OCE, where a continuously leaking water pipe can cause flooding and sinkholes as well as damage to other utilities in the proximity (Kumar et al., 2018).
Another risk presented in the literature is linked to the working environment in MUTs. Canto-Perello and Curiel-Esparza (2001b); Curiel-Esparza and Canto-Perello (2005), Zhang et al. (2016) and Fang et al. (2019) claim that, depending on the types of utilities placed in a MUT, it can bring different types of risks for workers. Examples of risks include gas pipelines that leak that can lead to explosions, leakage of wastewater that can cause harmful gases or spread diseases to workers, electrical pipes that can start fires and leaking district heating pipes with very hot water that can cause devastating consequences. These risks are brought up by different articles (e.g., Canto-Perello and Curiel-Esparza, 2001a; Curiel-Esparza and Canto-Perello, 2005; Zhang et al., 2016; Fang et al., 2019), but they do not present any empirical support for these risks and only base their suggestions on logical reasoning and experiences of working in other similar confined spaces.
The MUT's Sustainability Performance
All of the presented claims about MUT's pros and cons have, more or less, clear implications for the sustainability performance of the technology. However, the literature does not provide a comprehensive picture of the sustainability of MUTs as the different claims are scattered among various aspects and parts of the life cycle. This situation makes it difficult to provide any well-grounded conclusions regarding the sustainability of this technology and its performance in comparison to conventional alternatives for installing cable- and pipe-based infrastructure such as OCE.
Among the reviewed literature, studies that consider sustainability aspects throughout the entire MUT life cycle are lacking, as shown in Figure 4. Instead, the presented claims typically only target certain aspects of either the construction or use phase, while the end-of-life phase has not been addressed at all. This final life cycle phase involves activities that take place when the MUT is taken out of use. Such removal and waste treatment of large infrastructures in urban areas presumably have important impacts related to all three sustainability dimensions that should be considered. When it comes to the addressed sustainability impacts in the literature, most of the studies focus on economic performance. For the construction phase of the MUT, this is the only aspect that is analyzed despite that such a resource-intensive and invasive installation process also most likely has significant environmental and social consequences. The studies dealing with the use phase of MUTs entail, in general, a broader sustainability perspective by covering both economic and social aspects. However, none of the reviewed studies assesses environmental impacts related to the utilization of MUTs.
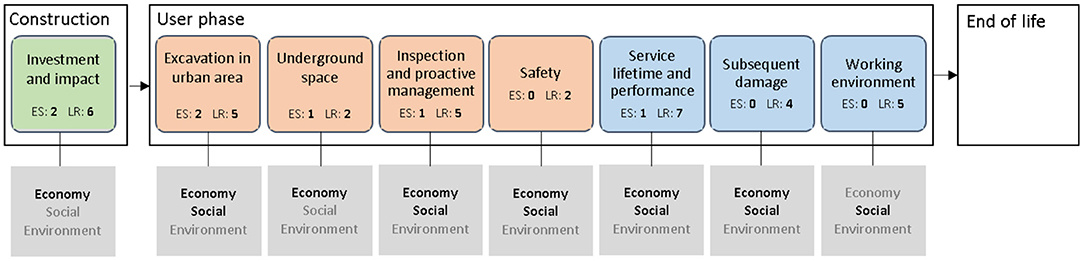
Figure 4. The earlier mapped and assessed sustainability claims presented along the MUT life cycle with the types of sustainability dimensions they are connected to (bold font). The economic dimension is the most studied and has implications for all claims except “working environment”. Social impact has been discussed for impact from the use phase, while the environmental one has only been discussed for “excavations in urban area”.
Beyond a limited scope on sustainability and the MUT life cycle, most of the studies present claims without sufficient empirical support and descriptions of the cases, projects and conditions considered in their analysis, which raises concerns about the validity and applicability of the study results. The few real-life projects that have been studied also indicate a lack of knowledge about what activities and processes take place along the life cycle of a MUT. Without such fundamental knowledge about the occurrence of different types of activities related to installation, maintenance, renewal, and waste management, any type of sustainability assessment of infrastructure technology becomes difficult. For MUTs, this deficiency in knowledge is most evident in the use and end-of-life phases. This is, however, not just a shortcoming that concerns MUTs but also OCEs, where it is often difficult to obtain credible estimates on the extent and frequency of different activities and processes along the life cycle (Kleiner and Rajani, 2001).
In the following part of this section, the current knowledge levels about the different sustainability dimensions of MUTs are discussed based on economic, social and environmental performance.
Economic Performance
In the studies on the economics of MUTs, performance is typically addressed by considering the costs for the construction and use phases and then comparing these to the corresponding ones for OCE. In essence, the installation of a MUT is described as more expensive, while the cost for the use phase is claimed to be lower than for OCE. Although it is only the construction cost for MUTs that has been empirically studied, there are reasons to believe that this claim is justified. Since the installation of a MUT involves a more invasive and resource-intense construction process during the use phase, the maintenance and renewal of the cable- and pipe-based infrastructure can essentially be done without costly excavations. However, the studies provide little guidance on what project-specific and management conditions and activities significantly influence these costs and thus in which situations the higher installation costs for MUTs will pay off.
An important reason why these studies fail to contribute to such a systemic understanding of the economics of MUTs is their lack of transparent descriptions of the project context they study or refer to. When it comes to cable- and pipe-based infrastructure, both site- and project-specific conditions affect the costs for the activities and processes during the entire life cycle, which means that the economic performance can vary widely between different projects (Francisque et al., 2014, 2017). Examples of such conditions that can differ from case to case are geological conditions, street surface layers, building density and traffic intensity, type of project (new development or location in the existing environment) and capacity needs from residents and businesses. Beyond such project-specific conditions, the reviewed studies lack detailed descriptions of what activities and processes have been accounted for and how their costs have been estimated. If such project-specific settings and methodological procedures are not clearly presented, it becomes difficult to understand under what conditions the presented results prevail and to what extent they are applicable in other cases (van der Giesen et al., 2020). In addition, this lack of transparency in different studies limits the possibility of reviewing and comparing different projects to identify conditions and activities of high importance for the economic performance of MUTs.
Another major limitation of these studies is that they only present superficial results concerning the economic performance of MUTs. For instance, the cost differences between MUTs and OCEs for the construction and use phases are typically only described as total costs or cost differences expressed in percentages. This lack of detailed and systematic assessments of how different project conditions and infrastructure management activities and processes contribute to the net outcome or estimated cost difference between MUTs and OCEs limits both the validity and usefulness of the results (Swarr et al., 2011; Esguerra et al., 2019). Given that any sustainability assessment of the life cycle performance of subsurface infrastructure technologies is inherently uncertain, the credibility of the results largely relies on how such uncertainties are analyzed and clearly communicated (Laner et al., 2015). Furthermore, without a fine-grained understanding of what conditions and activities build up the economic outcome of such technologies, the opportunity to provide guidance on how the economic performance can be improved is to some extent lost (Hetherington et al., 2014). Which are the critical conditions and settings that make projects suitable for MUTs, and how could the performance of MUTs be further improved in terms of material choices, changed the design or tailored installation processes? These are examples of some essential and forward-looking questions that the conducted studies on the economy of MUTs have so far failed to address.
The lack of a systemic and in-depth understanding of the economic performance of MUTs is, however, not the only limitation of the reviewed studies, and analysis of the involved actors is also missing (Hojjati et al., 2018). In MUT projects, several different utilities are placed in the same confined space and depending on the form of ownership (e.g., public or private) and type of utility, the incentives of their owners can be quite different (Wang and Fang, 2017). Understanding each owner's costs when choosing MUTs and OCEs is, therefore, a prerequisite for being able to develop tailored business models where the costs can be distributed in a fair way between the different utility owners involved (Alaghbandrad and Hammad, 2018). It is not only the construction cost that should be specified for each actor but also how the costs for the management of the different networks change over the entire life cycle if a MUT is used. The costs for maintenance and renewal may differ substantially between different utility networks. Water pipes, for example, are usually placed at a greater depth than electricity wires when OCE is used, meaning that the avoided costs for excavations in MUT projects are often significantly higher for such pipe networks than for power lines. The economic influence on other actors such as residents, landowners and business owners affected by the infrastructure location and management (Hojjati et al., 2017) is viewed here as part of the social sustainability performance.
Social Performance
The actor perspective is a way to make extensive impacts on society more specific and tangible (Weingaertner and Moberg, 2014). The social dimension involves the impact on both actors involved in managing the infrastructure, such as maintainers and other stakeholders in society (Hojjati et al., 2017). Examples of other important stakeholders are public transport, road users, residents and businesses/companies. The reviewed literature highlights several different types of social impacts from both MUTs and OCEs, but the actors are not identified and presented in a comprehensive manner. The social aspect that has received the most attention is the impact of excavation. Different types of potential impacts on traffic, business and many other activities in the city environment are outlined, but just as for economic performance, there is a lack of detailed knowledge of how important these impacts are and to what extent they are likely to occur. This knowledge deficit is of central importance for both OCE and MUT placements.
One particular aspect that we know distinguishes MUTs and OCEs is the work environment for maintenance and renewal, but the analyzed literature has only outlined potential risks when working in MUTs without making comparisons with OCE. Even if the risks in connection with the working environment of a MUT are well described, it is difficult to say anything about which alternative is preferable from the work environment perspective without detailed comparisons. It is definitely not riskless to work in an open shaft and be surrounded by soil masses, heavy working vehicles and sometimes intensive traffic flows (cf. Matthews et al., 2015).
Environmental Performance
Knowledge about the MUT's environmental performance is very undeveloped. The only certain thing is that the construction, user phase and end of life will have several different types of impacts on the environment. The most common type of environmental impact found in the analysis of infrastructure projects is the climate impact, often expressed as emissions of carbon dioxide equivalents. Hojjati et al. (2018) describe, in addition to climate change, that air quality, use of energy, materials and water, waste generation and management, as well as various impacts on biodiversity and soil and land, are aspects that all should be a part of a comprehensive environmental analysis. However, the comparison between the environmental impact of MUTs and OCEs would probably have strong similarities with the analysis of the economic performance in the reviewed studies. It will most likely be limited to the construction and user phases. The construction of a MUT will probably have a higher environmental impact due to the additional tunnel construction, but during the user phase, there will be a lower impact on the environment as excavation is avoided, the life of the subsurface infrastructure increases and the need for maintenance decreases.
Discussion
As demonstrated by this study, the knowledge about the MUT and its sustainability consequences is still incoherent and limited. Several epistemological and methodological challenges need to be considered in future research to improve the understanding of such multifaceted impacts related to infrastructure technologies. These challenges and how increased knowledge from case studies and the use of methods for assessing sustainability can be used are discussed below.
Addressing Knowledge Limitations About Infrastructure Management Practices
Although the MUT has been used occasionally since the mid-19th century, it is still an emerging technology with relatively few reported real-life projects and records of accomplishment compared to OCE (Zhang and Fang, 2018). From an epistemic point of view, there is a general lack of know-how and experience about which management activities and processes can be expected to occur along its life cycle. In combination with the long service life of infrastructure technologies, such a knowledge deficit creates large uncertainties regarding the long-term management needs and how they will affect different functions in society (Kleiner and Rajani, 2001; Shahata and Zayed, 2008). Facilitating reliable sustainability assessments of the MUT, therefore, relies on the development of more detailed knowledge about the activities and processes involved in its different life cycle phases (Hoogmartens et al., 2014).
Without such detailed knowledge, the validity and usefulness of sustainability assessments become limited. An illustrative example is a comparison by Hunt et al. (2014) of the costs for the construction and use phases of MUTs and OCEs. This type of study is valuable for pointing out potential pros and cons of MUTs compared to OCEs, but the usefulness of the results is limited without knowledge of how many excavations can be expected in real-life cases under different situations and settings. Developing such an understanding of the type, extent and frequency of different management activities and processes in individual cases thus facilitates and increases the credibility of sustainability assessments. However, to be able to provide more generic contributions to the performance of this technology, knowledge is required about the project conditions and settings in question and how they influence different sustainability consequences.
So, how can these knowledge deficits be addressed? One way to learn more about these management activities and processes is to further exploit the knowledge and experiences achieved in past MUT projects. Although the use of MUT is still limited, Luo et al. (2020) show that there are significantly more MUTs installed than the two described in this article (Pamplona and Barcelona), but their sustainability performance is still to be assessed. This can be done by collecting the available documentation from the different actors involved. To carefully map, document and compare different MUT projects is in itself an important research task.
When studying previous MUT projects, there is, however, a risk that information about project-specific conditions is missing and that the available documentation of different management activities and processes lacks important details. One way to achieve a greater understanding of such essential issues is to combine the documented information with interviews with the actors who have participated in the installation and management of the MUTs. Such interviews can add important information about the projects and their context and provide a better understanding of the various conditions that influence the type, extent and frequency of different utility maintenance and renewal needs. If both the collection of documentation and supplementary interviews are done systematically for several projects, there are opportunities for comparative analyses that can result in an improved understanding of similarities and differences between different cases. This allows building up more general knowledge regarding recurrent conditions and settings of importance for the performance of MUTs than the thus far common studies that only analyze individual cases.
Another way to gather more information about infrastructure management activities and processes is to continuously follow new MUT-projects over long period of time to gain additional knowledge of the life cycle activities. This increases the possibilities to tailor the data collection toward information needed to perform credible sustainability assessments. However, a challenge is that it is very difficult or even impossible to follow new projects in the long term within normal research projects. Nevertheless, if a research project has systematically documented the construction and installation phase of a MUT, this provides a good basis for later follow-up studies that focus primarily on the management of the system during the user phase and eventually the end of life. In any case, there are good reasons to encourage closer collaboration between researchers and practitioners on subsurface infrastructure to ensure that relevant and useful information and data are continuously documented during the management of the systems (Evans and Marvin, 2006; Wender et al., 2014). Carrying out this documentation is not only important for MUTs but also for OCEs because without corresponding knowledge about such activities and processes for conventional infrastructure placement, it is difficult to evaluate a MUT's relative sustainability performance.
Sustainability Assessment
The knowledge of which activities and processes take place along the life cycle for both MUTs and OCEs is necessary in order to evaluate the sustainability performance of the technologies. But to determine sustainability performance, the impact on the economy, environment and society of these activities must first be mapped.
The selection of which sustainability aspects to include in the assessment is decisive for the results and, therefore, the selection must be conducted in a systematic and transparent manner. What is accounted for as well as what aspects are not included and why need to be clearly explained. Infrastructure technologies and their management may cause a host of different economic, environmental and social consequences. However, as this study clearly demonstrates, the current knowledge about what specific sustainability impacts actually occur and which of them are most relevant for comparing different infrastructure technologies is still limited. Without such an overview, there is an obvious risk that important interconnections between infrastructure management activities and different sustainability impacts are neglected and trade-offs are missed, particularly when one technology has positive effects regarding one aspect but negative for others (Akhtar et al., 2015). There is a need to map cause-effect relationships between infrastructure management activities and various economic, environmental and social impacts. One way to initiate such knowledge development is through qualitative and participatory approaches, involving interviews and workshops with the stakeholders involved in and influenced by the management of infrastructure technologies (Buchholz et al., 2009; Diaz-Balteiro et al., 2017). As demonstrated by previous research in other fields (Einhäupl et al., 2019; Lindfors et al., 2020), the knowledge, experiences and perspectives of such stakeholders can be systematically compiled and analyzed to provide a gross overview of various sustainability consequences and related cause-effect relationships. Such an overview makes it possible to prioritize among the most relevant aspects to consider and perform qualitative evaluations of selected impacts. However, given that the results from such studies are based on the actors' knowledge and subjective values and that infrastructure management can affect sustainability in many ways, it is important to select a wide range of stakeholders with different expertise and perspectives. Examples of central actors are system owners, infrastructure contractors, road managers, business owners, residents and representatives from municipalities (Canto-Perello et al., 2016; Alaghbandrad and Hammad, 2018).
While such qualitative and participatory approaches are well suited for developing a broad overview of the economic, environmental and social consequences of infrastructure management, they typically provide little in-depth knowledge about specific sustainability impacts. For such purposes, quantitative assessment methods are needed. Various such methods are available and applied in other fields (Finnveden and Moberg, 2005, p. 2; Ahlroth et al., 2011) but when it comes to long-lived infrastructure networks particularly, life-cycle costing (LCC) and life-cycle assessments (LCA) are two examples of useful methods (Carlsson Reich, 2005). Beyond economic and environmental impacts, recent developments in LCC and LCA methodology also involve the possibility to include selected social impacts (Hoogmartens et al., 2014; Guinée, 2016). Examples of other potentially useful methods to specifically quantify societal and social impacts of technology choices related to subsurface infrastructure are discrete choice experiments (DCE) (Kessels et al., 2011) or contingent valuation methods (CVM) (Marella and Raga, 2014).
Up to now, however, there are only a few examples of quantitative assessments of the sustainability impact of MUT for subsurface infrastructure, and they only consider the economic performance of the construction phase compared to OCEs. This type of quantitative assessment thus needs to be developed for other sustainability impacts and life cycle phases. Another key limitation of the existing studies is that it only provides aggregated results in terms of total cost estimates. Furthermore, the identified absence of uncertainty and sensitivity analyses reduces the validity of the results and makes it difficult to understand how the performance can be influenced and improved.
Regardless of which methods will be used in the future to evaluate MUTs, the current state of knowledge should be taken into account. When it comes to such emerging technologies, which still are in an early phase of development, a learning-oriented approach to sustainability assessments has increasingly been advocated in the literature (Fleischer et al., 2005; Wender et al., 2014; Cucurachi et al., 2018). In contrast to decision-oriented studies, which mainly target net performance, the aim of such assessments is to develop a systematic understanding of the relevant sustainability aspects and how they are influenced by various conditions and settings. This is needed to avoid disqualifying such emerging concepts too early and, above all, to provide knowledge of fundamental importance for facilitating the further development of the technology in question.
Conclusions
Despite that the reviewed articles discuss several advantages and disadvantages of MUTs, it can be concluded that the level of knowledge about their sustainability performance is rather undeveloped. While previous research mainly deals with separate and isolated aspects, there is no comprehensive understanding of the sustainability consequences that occur during the life cycle of a MUT. In addition, most of the pros and cons highlighted in the literature are presented without any empirical support, making it difficult to assess their validity. The statements involving some form of empirical support are, on the other hand, highly case-specific, and the applicability of their results in other cases of MUTs are generally not discussed. Furthermore, the economic dimension of sustainability has received the most attention, particularly in relation to the installation phase of the MUT, while selected social aspects only have been discussed for the user phase. The environmental performance of MUTs has not been systematically evaluated in any study, which is a major shortcoming in the current knowledge.
In order to increase the knowledge about the performance of MUTs, there is a need for employing a life cycle perspective and a learning-oriented approach to sustainability assessments. Given the yet emerging character of MUTs, such learning-oriented studies should particularly focus on increasing the knowledge about the different management activities that occur along its life cycle. These studies can make use of both past and new projects but should pay more attention to the importance of project-specific conditions and details to provide a better ground for comparison and generalization. This demands more diverse and detailed data collection, which in most cases can only be achieved via close collaboration with stakeholders. Sustainability assessment methods such as LCA or LCC can provide guidance on data collection and knowledge development regarding infrastructure management activities. By combining both qualitative and quantitative assessment methods, cause-effect relationships between different types of management activities and sustainability consequences can be mapped and potentially critical factors and project conditions influencing the magnitude of these consequences identified. Such systemic knowledge facilitates more focused and tailored data collection, targeting the most influential management activities and project conditions for the sustainability performance of MUTs.
So far, the MUT has mainly been used in isolated projects and lacks the benefits of learning curves and the emergence of specialized actors and optimized working methods. It is likely that its performance will gradually improve with an increasing number of projects and subsequent development of the technology, know-how and more established practice. In order to support such development and facilitate the identification of promising paths for improvement, we argue that the use of learning-oriented sustainability assessments can play an important role.
Data Availability Statement
The original contributions presented in the study are included in the article/supplementary material, further inquiries can be directed to the corresponding author/s.
Author Contributions
FB: writing–original draft, investigation, and conceptualization. SA, JK, and NS: writing–review and editing, supervision, and conceptualization. All authors contributed to the article and approved the submitted version.
Funding
This study had received funding from the Kamprad Family Foundation for Entrepreneurship, Research & Charity (Grant No: 20180218).
Conflict of Interest
The authors declare that the research was conducted in the absence of any commercial or financial relationships that could be construed as a potential conflict of interest.
Publisher's Note
All claims expressed in this article are solely those of the authors and do not necessarily represent those of their affiliated organizations, or those of the publisher, the editors and the reviewers. Any product that may be evaluated in this article, or claim that may be made by its manufacturer, is not guaranteed or endorsed by the publisher.
References
Ahlroth, S., Nilsson, M., Finnveden, G., Hjelm, O., and Hochschorner, E. (2011). Weighting and valuation in selected environmental systems analysis tools – suggestions for further developments. J. Clean. Prod. 19, 145–156. doi: 10.1016/j.jclepro.2010.04.016
Akhtar, S., Reza, B., Hewage, K., Shahriar, A., Zargar, A., and Sadiq, R. (2015). Life cycle sustainability assessment (LCSA) for selection of sewer pipe materials. Clean Technol. Environ. Policy. 17, 973–992. doi: 10.1007/s10098-014-0849-x
Alaghbandrad, A., and Hammad, A. (2018). PPP Cost-Sharing of Multi-purpose Utility Tunnels. In: Smith, I.F.C., Domer, B. (Eds.), Advanced Computing Strategies for Engineering. Springer International Publishing, Cham, pp. 554–567. doi: 10.1007/978-3-319-91635-4_29
Alevizou, P., Oates, C., and McDonald, S. (2015). The well(s) of knowledge: the decoding of sustainability claims in the UK and in Greece. Sustainability. 7, 8729–8747. doi: 10.3390/su7078729
Ariaratnam, S. T., Lueke, J. S., and Allouche, E. N. (1999). Utilization of trenchless construction methods by canadian municipalities. J. Constr. Eng. Manage. 125, 76–86. doi: 10.1061/(ASCE)0733-9364(1999)125:2(76)
Basiago, A. D.. (1999). Economic, social, and environmental sustainability in development theory and urban planning practice. Environmentalist. (1998) 19, 145–161. doi: 10.1023/A:1006697118620
Buchholz, T., Rametsteiner, E., Volk, T. A., and Luzadis, V. A. (2009). Multi Criteria Analysis for bioenergy systems assessments. Energy Policy. 37, 484–495. doi: 10.1016/j.enpol.2008.09.054
Cano-Hurtado, J. J., and Canto-Perello, J. (1999). Sustainable development of urban underground space for utilities. Tunnell. Underground Space Technol. 14, 335–340. doi: 10.1016/S0886-7798(99)00048-6
Canto-Perello, J., and Curiel-Esparza, J. (2001a). Risks and potential hazards in utility tunnels for urban areas. Proc. Inst. Civil Eng. 156, 6. doi: 10.1680/muen.2003.156.1.51
Canto-Perello, J., and Curiel-Esparza, J. (2001b). Human factors engineering in utility tunnel design. Tunnel. Undergr. Space Technol. 16, 211–215. doi: 10.1016/S0886-7798(01)00041-4
Canto-Perello, J., and Curiel-Esparza, J. (2006). An analysis of utility tunnel viability in urban areas. Civil Eng. Environ. Syst. 23, 11–19. doi: 10.1080/10286600600562129
Canto-Perello, J., and Curiel-Esparza, J. (2013). Assessing governance issues of urban utility tunnels. Tunnel. Undergr. Space Technol. 33, 82–87. doi: 10.1016/j.tust.2012.08.007
Canto-Perello, J., Curiel-Esparza, J., and Calvo, V. (2009). Analysing utility tunnels and highway networks coordination dilemma. Tunnel. Undergr. Space Technol. 24, 185–189. doi: 10.1016/j.tust.2008.07.004
Canto-Perello, J., Curiel-Esparza, J., and Calvo, V. (2013). Criticality and threat analysis on utility tunnels for planning security policies of utilities in urban underground space. Expert. Syst. Appl. 40, 4707–4714. doi: 10.1016/j.eswa.2013.02.031
Canto-Perello, J., Curiel-Esparza, J., and Calvo, V. (2016). Strategic decision support system for utility tunnel's planning applying A'WOT method. Tunnel. Undergr. Space Technol. 55, 146–152. doi: 10.1016/j.tust.2015.12.009
Carlsson Reich, M.. (2005). Economic assessment of municipal waste management systems—case studies using a combination of life cycle assessment (LCA) and life cycle costing (LCC). J. Clean. Prod. 13, 253–263. doi: 10.1016/j.jclepro.2004.02.015
Chen, J., Shi, X., and Li, J. (2010). Shaking table test of utility tunnel under non-uniform earthquake wave excitation. Soil Dyn. Earthquake Eng. 30, 1400–1416. doi: 10.1016/j.soildyn.2010.06.014
Chen, Z., Liang, S., and He, C. (2018). Effects of different coherency models on utility tunnel through shaking table test. J. Earthquake Eng. 24, 579–600. doi: 10.1080/13632469.2018.1452807
Chester, M. V.. (2019). Sustainability and infrastructure challenges. Nat. Sustain. 2, 265–266. doi: 10.1038/s41893-019-0272-8
Cucurachi, S., van der Giesen, C., and Guinée, J. (2018). Ex-ante LCA of emerging technologies. Proc. CIRP 69, 463–468. doi: 10.1016/j.procir.2017.11.005
Cummins, S., Reilly, T. M., Carlson, L., Grove, S. J., and Dorsch, M. J. (2014). Investigating the portrayal and influence of sustainability claims in an environmental advertising context. J. Macromarket. 34, 332–348. doi: 10.1177/0276146713518944
Curiel-Esparza, J., and Canto-Perello, J. (2005). Indoor atmosphere hazard identification in person entry urban utility tunnels. Tunnel. Undergr. Space Technol. 20, 426–434. doi: 10.1016/j.tust.2005.02.003
Curiel-Esparza, J., and Canto-Perello, J. (2012). Understanding the major drivers for implementation of municipal sustainable policies in underground space. Int. J. Sustain. Develop. World Ecol. 19, 506–514. doi: 10.1080/13504509.2012.732973
Curiel-Esparza, J., and Canto-Perello, J. (2013). Selecting utilities placement techniques in urban underground engineering. Arch. Civil Mech. Eng. 13, 276–285. doi: 10.1016/j.acme.2013.02.001
Curiel-Esparza, J., Canto-Perello, J., and Calvo, M. A. (2004). Establishing sustainable strategies in urban underground engineering. Sci. Eng. Ethics. 10, 523–530. doi: 10.1007/s11948-004-0009-5
Dawe, N. K., and Ryan, K. L. (2003). The faulty three-legged-stool model of sustainable development. Conserv. Biol. 17, 1458–1460. doi: 10.1046/j.1523-1739.2003.02471.x
Diaz-Balteiro, L., González-Pachón, J., and Romero, C. (2017). Measuring systems sustainability with multi-criteria methods: a critical review. Eur. J. Oper Res. 258, 607–616. doi: 10.1016/j.ejor.2016.08.075
Einhäupl, P., Krook, J., Svensson, N., Van Acker, K., and Van Passel, S. (2019). Eliciting stakeholder needs – An anticipatory approach assessing enhanced landfill mining. Waste Manag. 98, 113–125. doi: 10.1016/j.wasman.2019.08.009
Esguerra, J. L., Krook, J., Svensson, N., and Van Passel, S. (2019). Assessing the economic potential of landfill mining: review and recommendations. Detritus. 8, 125–140. doi: 10.31025/2611-4135/2019.13883
Evans, R., and Marvin, S. (2006). Researching the sustainable city: three modes of interdisciplinarity. Environ. Plann. 38, 1009–1028. doi: 10.1068/a37317
Fang, W., Wu, J., Bai, Y., Zhang, L., and Reniers, G. (2019). Quantitative risk assessment of a natural gas pipeline in an underground utility tunnel. Process Safety Progress. doi: 10.1002/prs.12051
Finnveden, G., and Moberg, Å. (2005). Environmental systems analysis tools – an overview. J. Clean. Prod. 13, 1165–1173. doi: 10.1016/j.jclepro.2004.06.004
Fleischer, T., Decker, M., and Fiedeler, U. (2005). Assessing emerging technologies—Methodological challenges and the case of nanotechnologies. Technol. Forecast Soc. Change. 72, 1112–1121. doi: 10.1016/j.techfore.2004.10.005
Francisque, A., Shahriar, A., Islam, N., Betrie, G., Binte Siddiqui, R., Tesfamariam, S., et al. (2014). A decision support tool for water mains renewal for small to medium sized utilities: a risk index approach. J. Water Supply. 63, 281–302. doi: 10.2166/aqua.2013.305
Francisque, A., Tesfamariam, S., Kabir, G., Haider, H., Reeder, A., and Sadiq, R. (2017). Water mains renewal planning framework for small to medium sized water utilities: a life cycle cost analysis approach. Urban Water J. 14, 493–501. doi: 10.1080/1573062X.2016.1223321
Frangopol, D. M., Kallen, M. J., and van Noortwijk, J. M. (2004). Probabilistic models for life-cycle performance of deteriorating structures: review and future directions. Progr. Struct. Eng. Mater. 6, 197–212. doi: 10.1002/pse.180
Ge, F., and Xu, X. (2019). Research report on the Utility Tunnel Engineering based on BIM technology. J. Phys. 1176, 042028. doi: 10.1088/1742-6596/1176/4/042028
Guinée, J.. (2016). Life cycle sustainability assessment: what is it and what are its challenges? In: Clift, R., Druckman, A. (Eds.), Taking Stock of Industrial Ecology. Springer International Publishing, Cham, p. 45–68. doi: 10.1007/978-3-319-20571-7_3
Gupta, P., and Chauhan, S. (2021). Mapping intellectual structure and sustainability claims of sharing economy research – a literature review. Sustain. Prod. Consumpt. 25, 347–362. doi: 10.1016/j.spc.2020.09.006
Halfawy, M. R., Dridi, L., and Baker, S. (2008). Integrated decision support system for optimal renewal planning of sewer networks. J. Comput. Civil Eng. 22, 360–372. doi: 10.1061/(ASCE)0887-3801(2008)22:6(360)
Hetherington, A. C., Borrion, A. L., Griffiths, O. G., and McManus, M. C. (2014). Use of LCA as a development tool within early research: challenges and issues across different sectors. Int. J. Life Cycle Assess. 19, 130–143. doi: 10.1007/s11367-013-0627-8
Hojjati, A., Jefferson, I., Metje, N., and Rogers, C. D. F. (2017). Embedding sustainability criteria into pre-appraisal of underground utility for future cities. Proc. Inst. Civil Eng. 170, 258–271. doi: 10.1680/jurdp.17.00023
Hojjati, A., Jefferson, I., Metje, N., and Rogers, C. D. F. (2018). Sustainability assessment for urban underground utility infrastructure projects. Proc. Inst. Civil Eng. 171, 68–80. doi: 10.1680/jensu.16.00050
Hoogmartens, R., Van Passel, S., Van Acker, K., and Dubois, M. (2014). Bridging the gap between LCA, LCC and CBA as sustainability assessment tools. Environ. Impact Assess. Rev. 48, 27–33. doi: 10.1016/j.eiar.2014.05.001
Hu, C., and Zhang, S. (2019). Study on BIM technology application in the whole life cycle of the utility tunnel. In: Zeng, X., Xie, X., Sun, J., Ma, L., Chen, Y. (Eds.), International Symposium for Intelligent Transportation and Smart City (ITASC) 2019 Proceedings. Springer Singapore, Singapore, p. 277–285. doi: 10.1007/978-981-13-7542-2_27
Hunt, D. V. L., Jefferson, I., Drinkwater, N. K., and Rogers, C. D. F. (2012). Sustainable Utility Placement for University Campuses. In: GeoCongress 2012. Presented at the GeoCongress 2012, American Society of Civil Engineers, Oakland, California, United States. p. 4309–4318. doi: 10.1061/9780784412121.443
Hunt, D. V. L., Nash, D., and Rogers, C. D. F. (2014). Sustainable utility placement via Multi-Utility Tunnels. Tunnel. Undergr. Space Technol. 39, 15–26. doi: 10.1016/j.tust.2012.02.001
Hunt, D. V. L., and Rogers, C. D. F. (2005). Barriers to sustainable infrastructure in urban regeneration.pdf. In: Proceedings of the Institution of Civil Engineers-Engineering Sustainability. Vol. 158, pp. 67–81. doi: 10.1680/ensu.2005.158.2.67
Il'ichev, V. A., Nikiforova, N. S., and Tupikov, M. M. (2011). Deformation of soil masses during construction of shallow utility tunnels. Soil. Mech. Found. Eng. 48, 94–104. doi: 10.1007/s11204-011-9135-7
Jiang, L., Chen, J., and Li, J. (2010). Seismic response of underground utility tunnels: shaking table testing and FEM analysis. Earthquake Eng. Eng. Vibr. 9, 555–567. doi: 10.1007/s11803-010-0037-x
Kessels, R., Jones, B., Goos, P., and Vandebroek, M. (2011). The usefulness of Bayesian optimal designs for discrete choice experiments: bayesian optimal designs for choice experiments. Appl. Stochastic Models Bus. Ind. 27, 173–188. doi: 10.1002/asmb.906
Kleiner, Y., Adams, B. J., and Rogers, J. S. (2001). Water distribution network renewal planning. J. Comput. Civil Eng. 15, 15–26. doi: 10.1061/(ASCE)0887-3801(2001)15:1(15)
Kleiner, Y., and Rajani, B. (2001). Comprehensive review of structural deterioration of water mains: statistical models. Urban Water. 3, 131–150. doi: 10.1016/S1462-0758(01)00033-4
Krook, J., and Baas, L. (2013). Getting serious about mining the technosphere: a review of recent landfill mining and urban mining research. J. Clean. Prod 55, 1–9. doi: 10.1016/j.jclepro.2013.04.043
Kumar, A., Rizvi, S. A. A., Brooks, B., Vanderveld, R. A., Wilson, K. H., Kenney, C., et al. (2018). Using machine learning to assess the risk of and prevent water main breaks. arXiv:1805.03597. doi: 10.1145/3219819.3219835
Laner, D., Rechberger, H., and Astrup, T. (2015). Applying fuzzy and probabilistic uncertainty concepts to the material flow analysis of palladium in austria: uncertainty analysis of austrian palladium budget. J. Indus. Ecol. 19, 1055–1069. doi: 10.1111/jiec.12235
Lindfors, A., Gustafsson, M., Anderberg, S., Eklund, M., and Mirata, M. (2020). Developing biogas systems in Norrköping, Sweden: an industrial symbiosis intervention. J. Clean. Prod. 277, 122822. doi: 10.1016/j.jclepro.2020.122822
Liu, S., Yao, Y., Ren, L., Zhu, T., Ren, J., Xu, J., et al. (2018). Combination weight and radar chart based evaluation method for utility tunnel planning in multiple energy systems. In: 2018 2nd IEEE Conference on Energy Internet and Energy System Integration (EI2). IEEE, Beijing, p. 1–6. doi: 10.1109/EI2.2018.8581925
Lucas, S., Soler, L.-. G, and Revoredo-Giha, C. (2021). Trend analysis of sustainability claims: the European fisheries and aquaculture markets case. Food Policy. 104, 102141. doi: 10.1016/j.foodpol.2021.102141
Luo, Y., Alaghbandrad, A., Genger, T. K., and Hammad, A. (2020). History and recent development of multi-purpose utility tunnels. Tunnel. Undergr. Space Technol. 103, 103511. doi: 10.1016/j.tust.2020.103511
Luttikhuis, E. H. J. O.. (1992). “Underground space for utilities,” in Proceedings of the International Conference on Underground Space and Earth Sheltered Structures (Delft: Delft University Press), 3–5.
Marella, G., and Raga, R. (2014). Use of the Contingent Valuation Method in the assessment of a landfill mining project. Waste Manage. 34, 1199–1205. doi: 10.1016/j.wasman.2014.03.018
Matthews, J. C., Allouche, E. N., and Sterling, R. L. (2015). Social cost impact assessment of pipeline infrastructure projects. Envirorn. Impact Assess. Rev. 50, 196–202. doi: 10.1016/j.eiar.2014.10.001
Pandit, A., Minn,é, E. A., Li, F., Brown, H., Jeong, H., James, J. A. C., et al. (2017). Infrastructure ecology: an evolving paradigm for sustainable urban development. J. Clean. Prod. 163, S19–S27. doi: 10.1016/j.jclepro.2015.09.010
Pinior, B., Firth, C. L., Richter, V., Lebl, K., Trauffler, M., Dzieciol, M., et al. (2017). A systematic review of financial and economic assessments of bovine viral diarrhea virus (BVDV) prevention and mitigation activities worldwide. Prev. Vet. Med. 137, 77–92. doi: 10.1016/j.prevetmed.2016.12.014
Purvis, B., Mao, Y., and Robinson, D. (2019). Three pillars of sustainability: in search of conceptual origins. Sustainability Sci. 14, 681–695. doi: 10.1007/s11625-018-0627-5
Riera, P., and Pasqual, J. (1992). The importance of urban underground land value in project evaluation: a case study of Barcelona's utility tunnel. Tunnel. Undergr. Space Technol. 7, 243–250. doi: 10.1016/0886-7798(92)90005-3
Rogers, C. D. F., Hao, T., Costello, S. B., Burrow, M. P. N., Metje, N., Chapman, D. N., et al. (2012). Condition assessment of the surface and buried infrastructure - A proposal for integration. Tunnell Underground Space Technol. 28, 202–211. doi: 10.1016/j.tust.2011.10.012
Shahata, K., and Zayed, T. (2008). Simulation as a tool for life cycle cost analysis. In: 2008 Winter Simulation Conference. Presented at the 2008 Winter Simulation Conference (WSC), IEEE, Miami, FL, USA. p. 2497–2503. doi: 10.1109/WSC.2008.4736359
Stirling, A.. (1999). The appraisal of sustainability: some problems and possible responses. Local Environ. 4, 111–135. doi: 10.1080/13549839908725588
Swarr, T. E., Hunkeler, D., Klöpffer, W., Pesonen, H-. L., Ciroth, A., Brent, A. C., et al. (2011). Environmental life-cycle costing: a code of practice. Int. J. Life Cycle Assess. 16, 389–391. doi: 10.1007/s11367-011-0287-5
Tighe, S., Knight, M., Papoutsis, D., Rodriguez, V., and Walker, C. (2002). User cost savings in eliminating pavement excavations through employing trenchless technologies. Canad. J. Civil Eng. 29, 751–761. doi: 10.1139/l02-071
Valdenebro, J. V., and Gimena, F. N. (2018). Urban utility tunnels as a long-term solution for the sustainable revitalization of historic centres: the case study of Pamplona-Spain. Tunnel. Undergr. Space Technol. 81, 228–236. doi: 10.1016/j.tust.2018.07.024
Valdenebro, J. V., Gimena, F. N., and López, J. J. (2019). Construction process for the implementation of urban utility tunnels in historic centres. Tunnel. Undergr. Space Technol. 89, 38–49. doi: 10.1016/j.tust.2019.03.026
van der Giesen, C., Cucurachi, S., Guinée, J., Kramer, G. J., and Tukker, A. (2020). A critical view on the current application of LCA for new technologies and recommendations for improved practice. J. Clean. Prod. 259, 120904. doi: 10.1016/j.jclepro.2020.120904
van Doorn, J., Risselada, H., and Verhoef, P. C. (2021). Does sustainability sell? The impact of sustainability claims on the success of national brands' new product introductions. J. Business Res. 137, 182–193. doi: 10.1016/j.jbusres.2021.08.032
Wang, F., Jiang, X., and Niu, J. (2017). The large-scale shaking table model test of the shallow-bias tunnel with a small clear distance. Geotech. Geol. Eng. 35, 1093–1110. doi: 10.1007/s10706-017-0166-3
Wang, W., and Fang, J. (2017). Study on the risk evaluation model of utility tunnel project under a PPP Mode. In: ICCREM 2017. American Society of Civil Engineers, Guangzhou, China. p. 371–381. doi: 10.1061/9780784481059.039
Weingaertner, C., and Moberg, Å. (2014). Exploring social sustainability: learning from perspectives on urban development and companies and products: exploring social sustainability. Sustain. Develop. 22, 122–133. doi: 10.1002/sd.536
Wender, B. A., Foley, R. W., Hottle, T. A., Sadowski, J., Prado-Lopez, V., Eisenberg, D. A., et al. (2014). Anticipatory life-cycle assessment for responsible research and innovation. J. Respons. Innovat. 1, 200–207. doi: 10.1080/23299460.2014.920121
Wu, C., Liu, H., Huang, L., Lin, J., and Hsu, M. (2018). Integrating BIM and IoT technology in environmental planning and protection of urban utility tunnel construction. In: 2018 IEEE International Conference on Advanced Manufacturing (ICAM). IEEE, Yunlin. p. 198–201. doi: 10.1109/AMCON.2018.8615004
Zhang, X., and Fang, J. (2018). Systematic Model Study on the Investment Influencing Factors of Utility Tunnel Based on PPP Mode. In: ICCREM 2018. American Society of Civil Engineers, Charleston, South Carolina. p. 56–63. doi: 10.1061/9780784481752.008
Keywords: multi-utility tunnel, subsurface infrastructure, sustainability assessment, urban underground, cable and pipe networks
Citation: Bergman F, Anderberg S, Krook J and Svensson N (2022) A Critical Review of the Sustainability of Multi-Utility Tunnels for Colocation of Subsurface Infrastructure. Front. Sustain. Cities 4:847819. doi: 10.3389/frsc.2022.847819
Received: 03 January 2022; Accepted: 25 January 2022;
Published: 17 February 2022.
Edited by:
Md Maruf Mortula, American University of Sharjah, United Arab EmiratesCopyright © 2022 Bergman, Anderberg, Krook and Svensson. This is an open-access article distributed under the terms of the Creative Commons Attribution License (CC BY). The use, distribution or reproduction in other forums is permitted, provided the original author(s) and the copyright owner(s) are credited and that the original publication in this journal is cited, in accordance with accepted academic practice. No use, distribution or reproduction is permitted which does not comply with these terms.
*Correspondence: Filip Bergman, ZmlsaXAuYmVyZ21hbkBsaXUuc2U=