- 1Department of Community Medicine and School of Public Health, Post Graduate Institute of Medical Education and Research (PGIMER), Chandigarh, India
- 2Department of Environment Studies, Panjab University, Chandigarh, India
Indoor air quality (IAQ), specifically after the COVID-19 pandemic, has become an international issue, as humans spend 80–90% of their time in indoor microenvironments. Poor IAQ has been related to the sick-building syndrome, nasal and ocular irritations, allergies, and respiratory dysfunction, including premature deaths. Phytoremediation is a novel strategy to absorb, adsorb, assimilate or transfer/reduce air pollutants and improve the IAQ using plants. Hence, the current review aims to explore indoor plants' role in improving indoor air quality, including their purification capabilities. There is increasing evidence that various plant species (e.g., Ficus benjamina, Chlorophytum comosum, Draceana) or their parts can reliably reduce the concentration of numerous air pollutants in the indoor microenvironment and promote human wellbeing. However, the indoor air pollutants removal efficiency depends on the species of plant, various plant characteristics such as leaf size, thickness, area, photosynthetic activity, light intensity and part of plant involved, i.e., roots, leaves, wax, cuticle and stomata. Using indoor plants is one of the most cost-effective and reliable methods of making a healthier indoor environment. Better public health can be maintained at a lower cost, with less strain on the health care system, if more emphasis is placed on creating a biophilic atmosphere and increasing the use of indoor plants. However, there are no established criteria for the best indoor plants and the impact of indoor plants on various factors such as interior ventilation, temperature, humidity, etc. Therefore, further experimental research is needed that simulates the interior environment to monitor the impacts of indoor plants on factors such as humidity, temperature, ventilation, etc., in improving the microenvironment of a closed space/room.
Highlights
- Study examines the available evidence of the phytoremediation potential of indoor air pollutants.
- Indoor air pollutants removal efficiency depends on the parts of the plants involved: roots, leaves, wax, cuticle and stomata.
- Effect of plant-microbiome needs to be fully understood for indoor pollutants removal.
- Indoor plants offer an eco-friendly option for air purification with minimal energy consumption.
Introduction
Air quality is a crucial environmental factor affecting people's health, comfort and productivity. Air pollution has a negative impact on human health. Primary and secondary air pollutants like particulate matter (PM), volatile organic compounds (VOCs) such as benzene, toluene, ethylbenzene, xylene (BTEX), formaldehyde, polyaromatic hydrocarbons (PAHs), and inorganic pollutants (O3, NOx, CO2, SO2) remain present in ambient air in very high concentration specifically in lower and middle-income countries. There has been growing evidence that air pollutants are the root cause of the rise in the incidence of respiratory health issues in developing nations like China and India (Gulia et al., 2021; Zhang et al., 2022). These air pollutants could be present even at higher concentrations in indoor environments than outdoor ones (Myers and Maynard, 2005; Morawska et al., 2013).
In recent years, the energy crisis has led to the construction of air-tight, thermally insulated buildings, demanding less energy for heating. But in efforts to reduce energy consumption, less attention is paid to ventilation systems in the building. Due to reduced gaseous exchange, contaminants can accumulate up to toxic levels inside closed spaces leading to serious health concerns. Since most people in this modern time spend 85–90% time in indoor microenvironments such as schools, homes, or offices (Klepeis et al., 2001; Soreanu et al., 2013; Ravindra et al., 2020), the quality of indoor air become a major concern for better health.
Poor indoor air quality (IAQ) is known to be associated with the sick-building syndrome, which includes respiratory dysfunction, severe headache, ocular and cutaneous irritations, allergies, fatigue, and metabolic disorders (Brasche et al., 1999; Liu et al., 2016) and in some severe cases can lead to death (D'Amato et al., 2002). In addition to these health-related problems, indoor air pollution also decreases work efficiency and increases medical expenses, thus affecting the financially. According to the WHO report, indoor air contaminants accounted for 4.3 million premature deaths in 2012 (World Health Organization, 2014) and 3.8 million deaths in 2016, mainly in low- and middle-income countries (World Health Organization, 2016).
Pollutants present in indoor air come from different sources like outdoor air, fuel combustion, pets, furniture, paints, electronic equipment, etc. (Afshari et al., 2005; Yu et al., 2009; Clausen et al., 2011; Sharma et al., 2019; Ravindra et al., 2021a) as shown in Figure 1. Fungi and bacteria also contribute to lowering IAQ. Carbon dioxide (CO2) is a common indoor pollutant released by occupants' breathing activity. Its concentration can rise several times higher than the standard value in air-tight spaces. A high concentration of CO2 causes fatigue, drowsiness, headache, loss of concentration, nose and throat irritation, nasal discharge, cough and eye discharge (Seppanen et al., 2005; Llewellyn and Dixon, 2011; Ercan, 2012; Cetin and Sevik, 2016). Therefore, CO2 concentration is typically used as a parameter to check the ventilation rate in closed spaces (Zhang and Smith, 2003).
Besides CO2, PM and VOCs are the two primary pollutants in the indoor air. PM are hazardous as they can easily accumulate in the living cells and have high heavy metal content (Gawrońska and Bakera, 2015; Sonwani et al., 2022). Another class of pollutants, i.e., VOCs, are compounds with low molecular weight, such as aromatic, halogenated and oxygenated hydrocarbons with a 50–60°C boiling point at room temperature (Ravindra et al., 2008; Kim et al., 2018). In addition to other health-related issues, VOCs like and formaldehyde and benzene have also been reported to be carcinogenic (Nielsen and Wolkoff, 2010; Aydogan and Montoya, 2011). Prolonged exposure to these pollutants and their synergetic effect is the main reason behind health-related problems caused by poor IAQ (Soreanu et al., 2013).
Thus, maintaining an indoor environment could be an essential criterion for human health and comfort. Numerous modern techniques have been developed to maintain the air quality in closed spaces, but none seem promising in maintaining the level of pollutants. None of the present air purifiers can remove all the contaminants to match the standards fixed by World Health Organization (WHO) and they are very expensive. It has also been reported that some air purifiers emit ozone which is also toxic for humans if it accumulates beyond a certain level (Britigan et al., 2006). Hence, continuous efforts are being made to develop economical and sustainable air-purifying techniques. Growing decorative plants in indoor environments can provide a much more efficient and natural approach to abate the concentration of different air pollutants in ambient air. Han and Ruan (2020) conducted a systematic review and found that indoor plants have the potential to improve the air quality, followed by a decrease in temperature and an increase in humidity. In contrast, Susanto et al. (2021) suggested that indoor plants can be used as an alternative way to reduce indoor air pollution.
Indoor plants purify the air and positively affect the occupant's work productivity and mental health (Shibata and Suzuki, 2004; Han, 2009; Park and Mattson, 2009; Raanaas et al., 2011; Deng and Deng, 2018; Saxena and Sonwani, 2020). Over the past three decades, various studies have elaborated that indoor plants may dramatically reduce the majority of indoor air pollutants. In the post COVID-19, people are shifting toward traditional and greener options and reducing the usage of artificial purifiers. Therefore, appropriate low-cost methods of changing indoor air quality and microclimate conditions should be researched.
However, there have been no thorough assessments of the impact of indoor plants on air quality. To fill a gap in the literature, this study examines the existing literature to provide an overview of the effects of indoor plants on air quality, including identifying the main classes of indoor air pollutants and their impact on human health. The manuscript's key focus is to highlight the various mechanisms by which decorative plants take up indoor air pollutants and convert them into less toxic or harmless metabolites. The study also identifies the major plant species reported to be effective for indoor air phytoremediation and to regulate interior environmental comfort and air quality.
Methodology
This comprehensive literature review explores and discusses the role of indoor plants in improving indoor air quality for human health and comfort. The inclusion criteria for screening the existing literature were: (1) recent publication in an international peer-reviewed journal available on PubMed, Google Scholar, Scopus, or Science Direct database (irrespective of date); (2) emphasis on articles that review the main classes of indoor air pollutants and their impact on human health, plants involved in the uptake of indoor air pollutants and converting them into less toxic or harmless metabolites, related mechanism of action, parts of plants involved in improving air quality; (3) published in English (4) both in-vivo and in-vitro studies were also considered. The exclusion criteria include (1) thesis, dissertations, conference proceedings, letters to the editor and editorials; (2) language except for English. The keywords begin with “indoor air quality”, followed by the search words and their combinations: “air pollutants”, “phytoremediation”, “indoor plants”, “household air pollution” “indoor air quality” and “public health”. The search was performed using Boolean logic searching methods (AND/OR/NOT/()/“ ”). A manual search for relevant articles was also conducted in the bibliography section of each article.
Further, a bibliometric analysis was done to extract the snapshot of the research domain by objectively and statistically examining the bibliographic data. Swift collection of the database was done based on the keyword frequency using the dimensions website (https://www.dimensions.ai/). “Indoor air plants” AND “air pollution” AND “public health” AND “phytoremediation” were used as search terms to identify the most relatively matching publication. The retrieval was made on November 2, 2022. VOSviewer (version 1.6.10) was utilized to analyze citations and publications based on countries.
Bibliometric analysis
The global literature published between 1970 and 2021 on the influence of indoor plants on indoor air quality was analyzed through the dimensions database collection. The original number of published articles revealed that 1,174 documents are available between 1970 and 2021. The information for the articles that met the requirements was exported in CSV format and included publication year, language, journal, title, author, affiliation, keywords, document type, abstract, and citation counts. The network and density of the countries based on relevant documents pertaining minimum of 10 citations/10 documents from the different geographic areas were viewed using VOSviewer.
The table generated by the VOSviewer software shows the prominent countries that published articles on the topics of indoor air quality. Table 1 depicts top 20 countries based on total link strength. According to the VOSviewer manual, each link carries a strength, which is represented by a positive numeric value. The greater this value, the greater the link's strength. The total link strength reflects the number of occurrences of search keyword queries in published articles. China has a total link strength (621), followed by Australia (515), the United States (340), and then the United Kingdom (325). India is at fifth position with 288 link strength. The origin seems far more global, spanning Europe, Asia, and America.
Figure 2 illustrates the Bibliometric analysis of citations and publications by country. The size of each circle corresponds to the number of co-citations. The distance between the two circles represents their correlation. On the basis of citations, various colors represent distinct groups. Scientific research plays a vital role in understanding the effects of indoor plants on indoor air quality.
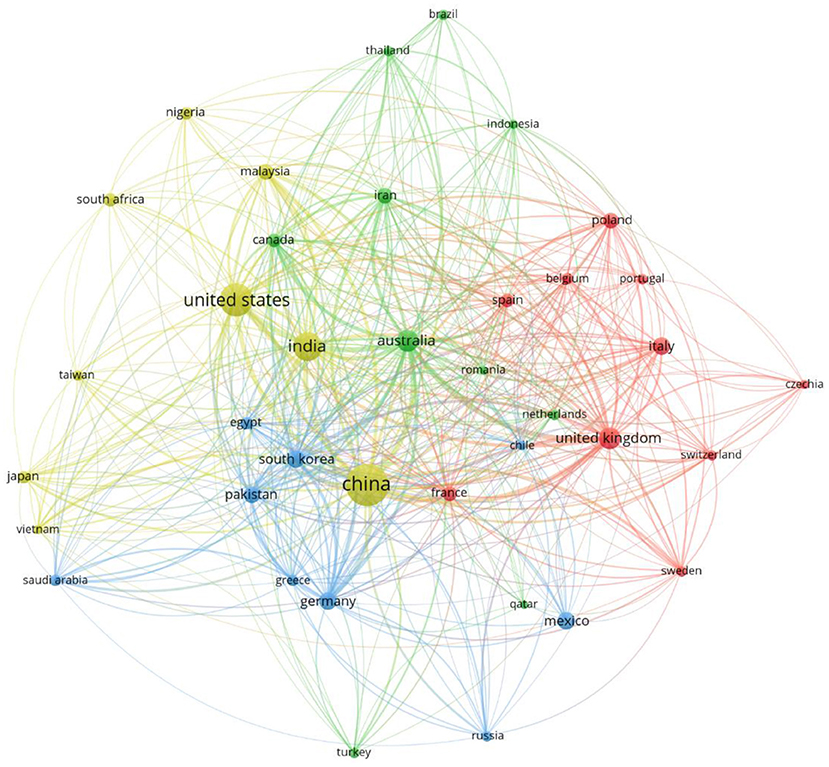
Figure 2. Countries' network and density based on relevant documents on phytoremediation of indoor air pollution using bibliographic analysis.
Indoor air quality
The air quality within and around buildings and other structures impacts the health of the occupants. In urban areas, mechanical heating and cooling air ventilation systems (known as HVAC) are used to maintain the temperature and IAQ of closed spaces like offices, schools, homes, etc. In order to lower energy utilization, buildings are made as air-tight as possible to prevent the infusion of unconditioned outside air with conditioned air inside. The construction of thermally insulated buildings comparatively requires less energy for heating and air conditioning, which has increased the problem of indoor pollution worldwide. Moreover, in lower and middle-income countries, most rural populations depend on solid biomass burning as their primary source of household energy for their daily needs, including cooking and residential heating (Ravindra et al., 2020, 2021b).
In 2015 emission from the incomplete combustion of solid biomass fuel was reported to be associated with over 2.9 million premature deaths on a global scale and ranked as the 8th major risk factor contributing to the global burden of disease (Ravindra et al., 2020). Thus, indoor air pollution has emerged as an essential consideration to minimize the preventable burden of death and disability to improve public health (World Health Organization, 2014, 2016). Particulate matter pollution, biological pollution (such as pollen, fungi, molds, dust mites, etc.), physical pollution caused by agents such as temperature and electromagnetic fields, and chemical pollution, which includes volatile organic compounds in addition to radon, carbon monoxide, and other non-organic chemicals, are the four categories that can be used to classify indoor air pollution (Kim et al., 2018).
VOCs are considered an extensively studied class of indoor air pollutants. Their indoor levels can rise up to 10 times higher than the outdoor air levels (Žuškin et al., 2009). They are released from various sources building materials, paints, adhesives, cosmetics, furniture, carpets, electronic equipment, cleaning products and occupant activities (Yu and Crump, 1998; Llewellyn and Dixon, 2011; Agarwal et al., 2019). The concentration of VOCs also varies with time, occupant activities and depending on the type of indoor environment. During winters, their indoor amount is notably higher as most windows remain closed. Various VOCs are present in the indoor environment, among which formaldehyde, benzene, toluene, ethylbenzene, xylene, and polyaromatic hydrocarbons (PAHs) are most widely studied due to their hazardous nature.
CO2 is a common indoor air pollutant, present in very high concentrations in poorly ventilated rooms and buildings due to continuous respiratory emissions by occupants (Llewellyn and Dixon, 2011; Kaur-Sidhu et al., 2020). Other sources of CO2 inside closed spaces include gas stoves, fireplaces, cigarette smoking and microbial activities. All these activities can increase CO2 concentration to a much higher level than its outside ambient environmental concentration. Usually, the average outdoor air concentration of CO2 is ~400ppm, as provided by World Meteorological Organization (WMO, 2018). However, this level may rise up to 2000-2500 ppm in crowded and under-ventilated rooms (Persily and Gorfain, 2004; Pettit et al., 2018). CO2 concentrations are generally used to measure the ventilation rate in buildings and levels below 1,000 ppm indicate an adequate fresh air supply in the room. They are frequently used to examine and investigate a building's ventilation capacity to remove indoor air contaminants.
Particulate matter is generally classified based on its aerodynamic diameter ranging from 0.001 to 10 μm. The smaller the size, the deeper it can penetrate the human respiratory system; thus, fine and ultrafine particles affect health more severely than coarse particles. Fine particles in indoor air have been reported to be the most hazardous fraction of air pollutants leading to various human health impacts (Pandey et al., 2021; Sonwani et al., 2021). Particulate matter is emitted into the indoor air during cooking, ironing, cleaning, smoking, and other occupant activities and remains suspended in the air for a long time (D'Amato et al., 2002; Afshari et al., 2005). PM10 and PM2.5 are the most extensively studied categories of particulate matter. There are various toxic components of air pollutants, such as heavy metals and organic compounds like PAHs, which remain absorbed/adsorbed on fine particles (Ravindra et al., 2001, 2006; Masiol et al., 2012). Further, environmentally persistent free radicals can also adhere to indoor particles, increasing their bio-availability and enhancing the accumulation of toxic fractions in the living cells (Saravia et al., 2013).
Health effects of indoor air pollutants
Several studies provide direct evidence of exposure to indoor fine particles and their association with various respiratory illnesses such as impaired lung function, asthma and chronic obstructive pulmonary disease (COPD) (Upadhyay et al., 2014; Wu et al., 2018; Shetty et al., 2021). It has been estimated that exposure to toxic indoor fine particles could contribute to around 10–30% of the global burden of disease (Morawska et al., 2013). Indoor air pollution has been related to various health effects, including irritation of the eyes and upper respiratory tract, chronic lung inflammation, cardiovascular disease, and, in extreme cases, cancer (Ravindra, 2019). Exposure to high particle concentration has been linked to increased morbidity and mortality due to cardiovascular, respiratory and venous thromboembolic disease (Bari et al., 2014; Ge et al., 2018).
Simoni et al. (2002) discovered a relationship between indoor PM2.5 exposure and the onset of bronchitis and asthma symptoms, particularly during the winter months, in northern-central Italy. Moreover, 51% of the global death ratio and 50% of disability-adjusted life years were reported to be linked with PM2.5 exposures in India and China (World Health Organization, 2016). In India, particulate matter was found to be responsible for 1.1 million premature deaths in 2015. Prolonged exposure to PM10 and PM2.5 can cause inflammation of the alveoli. This could lead to blood coagulation and the release of harmful inflammatory cytokines. Further, severe exposure to fine indoor particles has been reported to be associated with bronchitis, asthma and neuron damage, including myocardial infarction and ischemic heart disease (Rai, 2015; Manisalidis et al., 2020).
Moreover, most indoor VOCs are identified as human carcinogens and their exposure has been associated with respiratory problems, including asthma. Formaldehyde is a ubiquitous indoor pollutant and is classified as a Group I carcinogen by International Agency for Research on Cancer (IARC) (2006). There is sufficient epidemiological evidence that stipulates that formaldehyde causes cancer in humans. According to IARC, formaldehyde causes nasopharyngeal cancer in human beings. Among BTEX hydrocarbons, benzene is also reported to induce cancer and is considered one of the most toxic VOCs. It can potentially produce hematological illnesses such as acute and chronic lymphocytic leukemia, acute myeloid leukemia, non-lymphoma, Hodgkin's multiple myeloma, and aplastic anemia (Snyder, 2012). Further, various studies have linked VOC exposure to respiratory, hepatic, nervous and renal problems (Mendell, 2007; Sriprapat et al., 2014a; Mozaffar and Zhang, 2020).
Poor indoor air quality also causes sick building syndrome known as SBS, including multiple chemical sensitivity and new house syndrome (Shinohara et al., 2004). Indoor air pollutants can act as a carrier of allergens, respiratory and eye irritants, and toxicants (Bernstein et al., 2008; Ravindra, 2019; Ravindra et al., 2021a). The most common indicators of poor IAQ are ocular, nasal and cutaneous irritation, severe allergies, headache, fatigue and respiratory dysfunction, symptoms collectively known as SBS (Aydogan and Montoya, 2011; Lu et al., 2016).
Role of plants for absorption and removal of indoor pollutants
Plants kept indoors serve as a component of the three-dimensional environment and interact with humans in a wide variety of ways. Numerous studies discuss the different aspects of plant characteristics, such as leaf area, number, sunlight intensity, respiration, and photosynthesis, to remove air pollutants effectively. Irga et al. (2013) estimated that 57 m2 of leaf area would be able to absorb/remove around 13% of CO2 generated per person in an average room without ventilation. The greater the leaf area, the more CO2 is removed by indoor plants. Previous studies have also suggested that leaf characteristics significantly remove pollutants, as shown in Table 2. Various other leaf characteristics, i.e., leaf roughness, thickness and leaf villi, were also important aspects concerning dust retention ability (Jia et al., 2012; Lu et al., 2018; Jeong et al., 2020).
At the same time, Fujii et al. (2005) reported an increase in the removal efficiency of CO2 with increasing illuminance. Also, Burchett et al. (2011) found that low light intensities (~10 PAR μmol m−2 s−1) showed no or less CO2 removal. The removal rates were quite inadequate, even with a reasonable number of plants to improve indoor air quality. This lower efficiency of CO2 removal was due to the respiration process taken place by non-green parts of plants and pot microorganisms. Therefore, for potted plants, the increased light intensity with reduced pot mix microorganisms is required (Burchett et al., 2011). Hörmann et al. (2018) showed that the value of toluene varied between 3.4 and 5.7 L h−1 m−2 leaf area with no significant differences between selected plant and light conditions (light/dark). (Orwell et al., 2004) observed that the removal rate of benzene per pot ranged from 12 to 27 ppm d−1 and microorganisms of the potting mix rhizosphere were the primary agents of removal.
Some air-purifying houseplants are depicted in Figure 3. During photosynthesis, the photoelectric effect in the leaf produces a large number of negative air ions (NAIs), which is responsible for absorbing dust and improving air quality (Wu and Lee, 2004; Perez et al., 2013; Yan et al., 2015). Shiue et al. (2011) also reported that NAIs effectively reduce the aerosol pollutants in average-sized rooms. Moreover, the production of NAIs during photosynthesis is beneficial to the health of individuals. However, using plants to produce fresh air with abundant NAIs indoors is still a major challenge. Hence, there is a need to understand the optimization of the relationship between them.
Pathways and mechanisms for indoor air pollutants removal by plants
Phytoremediation strategies
Phytoremediation is a bioremediation technique using plants and associated microorganisms to remove, transfer, stabilize or destroy pollutants (Newman and Reynolds, 2004). Plants are well known for their ability to absorb and metabolize toxic compounds from air, water and soil. Using green plants to improve IAQ offers a comparatively less expensive and environmentally friendly option than modern high-energy consuming techniques. Indoor plants eliminate harmful pollutants and have a positive psychological effect on occupants, thus enhancing human health and wellbeing (Fjeld et al., 1998; Han, 2009). Several studies focus on describing the role of plants in remediating poor IAQ, such as Darlington et al. (2001), Wood et al. (2002), and Aydogan and Cerone (2021).
Plants can absorb and assimilate air pollutants through regular gas exchange via the stomatal opening. Some indoor contaminants are known to be removed by microorganisms associated with plants or through direct adsorption to the plant surface (Son et al., 2000; Orwell et al., 2004). There have been different techniques used for phytoremediation based on their physical properties, type of the plant and medium of remediation, i.e., Phytoextraction, Phyto filtration, Phyto stabilization, phytodegradation, rhizodegradation and phytovolatilization, shown in Figure 4 (Moya et al., 2019). All these techniques use different metabolic processes to filter the pollutants. Figure 5 shows the contribution of different plant parts in removing indoor pollutants from the surrounding air.
Entry of pollutants into the plants and mechanism of action
Photosynthesis is the primary process responsible for lowering the amount of indoor CO2. Plants uptake CO2 and releases O2 in the surrounding atmosphere. They utilize CO2 as a source of carbon to synthesize organic compounds by using sunlight and water as a source of energy. Hence, the rate of photosynthesis depends upon the conditions like availability of sunlight, amount of CO2 and temperature (Zeiger and Field, 1982; Cure and Acock, 1986). The rate of photosynthesis increases with the increase in the concentration of CO2 under vigorous light intensity, releasing more O2 into the air.
NASA conducted a series of experiments that proved that green plants could efficiently remove VOCs from the air (Wolverton et al., 1989). Plants remove toxicants from the air either through stomatal uptake (Newman and Reynolds, 2004) or by adsorbing them on their leaf surface (Orwell et al., 2004). Plants can metabolize these chemicals into non-toxic compounds, either utilized by them or released into the surrounding atmosphere (Newman and Reynolds, 2004; Liu et al., 2007). Transpiration is a normal process of water evaporation from plant parts, mainly leaves, that increase the level of humidity in closed spaces, thus making the indoor environment more comfortable for the occupants (Aydogan and Montoya, 2011; Llewellyn and Dixon, 2011; Kichah et al., 2012; Deng and Deng, 2018).
In plants, both the aboveground and belowground plant parts play a role in purifying indoor air. Stomata present on the leaf surface provide a natural gateway for gaseous pollutants into the plant body. The cuticle also plays an essential role in adsorbing air contaminants. For example, in Z. zamiifolia, cuticular absorption accounted for 20% of benzene, 23% of toluene, 25 % of ethylbenzene, and 26% of xylene uptake (Sriprapat and Thiravetyan, 2013). After entering into the leaf, contaminants can be degraded, retained, or excreted either at the uptake site or after translocation to other sections. Kondo et al. (1995) showed that increased stomatal conductance of Nerium Indicum was linearly related to the increased formaldehyde removal from the surrounding. Benzene can penetrate more quickly due to its hydrophobic nature toward the cuticle, while formaldehyde enters more rapidly through the stomatal opening as shown in Figure 6 (Cruz et al., 2014).
The actual pathway or uptake of indoor pollutants depends on their physicochemical properties. Hydrophilic pollutants (such as formaldehyde) cannot quickly diffuse/penetrate through the cuticle (as it is made up of lipids) and often enter through the stomatal openings. Roots are also involved in decontaminating the indoor air. Roots take up pollutants by themselves from the surrounding air and increase pollutant's bioavailability for the microorganisms (Wenzel, 2009). Once taken up by roots, pollutants are either metabolized or translocated to the aboveground parts for further processing or storage. Removal of aboveground parts affects the efficiency of the root zone for pollutant uptake. In the absence of photosynthesis, no root exudation occurs, which is mainly responsible for increasing the bioavailability of pollutants. Transpiration pull will also be lacking; therefore, absorbed pollutants cannot be transported to the other parts. These factors reduce the ability of roots to remove pollutants from the indoor air. Table 3 shows the list of indoor plants involved in air pollutant removal.
Several enzymes, such as formate dehydrogenase, cytochrome P450 monooxygenase, and dioxygenase, also play an essential role in plant cellular detoxification pathways (Rylott and Bruce, 2009). They are actively involved in the oxidation of the BTEX (xylene) group of pollutants. BTEX mainly enters the plant body through the stomata. After entering into the leaf, they are targeted to intracellular compartments for transformation. Cytochrome P450 enzymes are present on the membranes of the endoplasmic reticulum. Hydroxylation and cleavage of the aromatic ring are the main steps in BTEX transformation. Phenol is the first intermediate metabolite formed during benzene metabolism, which is then converted into catechol. Finally, cis-cis muconic acid is formed through the cleavage of an aromatic ring, with o-quinone formation, as an unstable intermediate. Muconic acid is the first organic acid formed during benzene metabolism, which can be further oxidized to fumaric acid.
Since fumaric acid is an intermediate of the Tricarboxylic Acid (TCA) cycle, it can directly enter into the TCA cycle. Studies using 14C-labeled benzene indicate that benzene degrades into both high and low-molecular-weight compounds. Still, the maximum radioactivity was found in low molecular weight molecules such as organic acids and amino acids, as shown in Figures 6, 7, which depict formaldehyde metabolism and pathway of benzene metabolism in plants.
Microflora and indoor air pollutants
Microflora associated with plant parts also plays a vital role in removing pollutants (Orwell et al., 2004; Weyens et al., 2015). Numerous bacteria and fungi have been found to be symbiotically associated with the roots (rhizosphere) and leaves (phyllosphere) of plants which help the host plant in nutrient and water uptake, provide protection against pathogens and withstand biotic and abiotic stress (Weyens et al., 2009, 2015; Bulgarelli et al., 2013). Plant microcosm also assists the host plant in detoxifying air by increasing the bioavailability of pollutants to different parts, thus removing them from the air (Claudio, 2011).
Plant-microbe interaction is critical in phytoremediation because it sequesters and degrades contaminants while promoting plant growth (Weyens et al., 2009, 2015). Microorganisms living in the rhizosphere and phyllosphere have the ability to absorb pollutants from the ambient atmosphere (Sorkhoh et al., 2011). In fact, the removal capacity of plants for VOCs is now mainly attributed to the activity of microflora associated with the roots of plants (Llewellyn and Dixon, 2011). However, the effect and complexity of the plant microbiome need to be fully understood to remove indoor pollutants effectively.
Future perspective
Scholarly articles published on indoor plants affecting air quality indicate IAQ is drawing more attention. Indoor plants are the best-suited eco-friendly approach toward air purification/remediation with minimal energy consumption. But more in-depth studies are required to quantify the reduction in the actual amount of energy consumption due to plants. Moreover, various plant species that are likely candidates for use as house plants further need to be identified. Therefore, there is a significant window of opportunity for researchers, botanists, environmentalists, health and medicine experts to collaborate and investigate a specific plant's species, its criteria for indoor plants and their benefits.
More empirical research is needed to determine how indoor plants mitigate pollutants like formaldehyde, benzene, and toluene that are not in the air. Furthermore, as a consistent benchmark for transparency, ease of replication, and direct comparison, more complete and accurate information should be provided regarding the experimental protocols, especially on acclimation, plant quantity, ventilation, climate conditions, sampling frequency or period, and replication. In order to compare plant performance in pollutant absorption, consistent experimental techniques and measurement units are required.
Further research is needed on plant characteristics such as leaf size, thickness, surface area, the efficacy of specific species, and the plant part involved in removing air pollutants. Future interventions for air pollutants must concentrate on human behavior in response to indoor plants and enhanced ventilation. Further, short- or long-term exposure to indoor pollutants may result in the development of possible allergies or diseases. Hence, there is a need to establish a safe list of plants that can be used to minimize indoor air pollutants.
Limitation
This study is predominately based on a scoping/narrative approach, but we aimed to comprehensively provide the role of indoor plants in reducing air pollution. However, systematic reviews detailing each aspect, e.g., Indoor air pollution and health risk, mechanism of phytoremediation, economic viability and various aspects of the built environment, could further help to explore the use of the plant in reducing the exposure of indoor air pollutants.
Conclusions
Indoor plants appear to show pronounced improvement in IAQ and promote human well-being. Numerous studies showed a substantial decline in the concentration of air pollutants in the presence of indoor plants. This review enhanced knowledge of the detoxification pathways involved in removing various pollutants (such as plant roots, effectively removing VOCs). The extent to which indoor plants mitigate or reduce the adverse effects of air pollution differs from species to species. Therefore, the plant microbiome's effect and complexity still need to be fully understood. Further, the removal capacity of indoor plants needs to be fully clarified to establish and understand the actual pollutant removal mechanism. Moreover, the ability to remove air pollutants needs to be quantitatively assessed based on research trials and actual modeled-based mechanisms. Especially in low- and middle-income countries, there are several challenges and stress in the field of the health sector. Shifting to indoor greenery and adapting a biophilic environment can help reduce the pressure on the health sector and help sustain better public health.
Author contributions
KR and SM conceptualized and co-designed the framework and reviewed and edited the manuscript. Both authors contributed equally and approved the final manuscript.
Conflict of interest
The authors declare that the research was conducted in the absence of any commercial or financial relationships that could be construed as a potential conflict of interest.
Publisher's note
All claims expressed in this article are solely those of the authors and do not necessarily represent those of their affiliated organizations, or those of the publisher, the editors and the reviewers. Any product that may be evaluated in this article, or claim that may be made by its manufacturer, is not guaranteed or endorsed by the publisher.
References
Afshari, A., Matson, U., and Ekberg, L. E. (2005). Characterization of indoor sources of fine and ultrafine particles: a study conducted in a full-scale chamber. Indoor Air. 15, 141–150. doi: 10.1111/j.1600-0668.2005.00332.x
Agarwal, P., Sarkar, M., Chakraborty, B., and Banerjee, T. (2019). “Phytoremediation of Air Pollutants,” in Phytomanagement of Polluted Sites. Elsevier. p. 221–241. doi: 10.1016/B978-0-12-813912-7.00007-7
Aydogan, A., and Cerone, R. (2021). Review of the effects of plants on indoor environments. Indoor Built Environ. 30, 442–460. doi: 10.1177/1420326X19900213
Aydogan, A., and Montoya, L. D. (2011). Formaldehyde removal by common indoor plant species and various growing media. Atmospheric Environment. 45, 2675–2682. doi: 10.1016/j.atmosenv.2011.02.062
Bari, M. A., MacNeill, M., Kindzierski, W. B., Wallace, L., Héroux, M.-È., and Wheeler, A. J. (2014). Predictors of coarse particulate matter and associated endotoxin concentrations in residential environments. Atmos. Environ. 92, 221–230. doi: 10.1016/j.atmosenv.2014.04.025
Bernstein, J. A., Alexis, N., Bacchus, H., Bernstein, I. L., Fritz, P., Horner, E., et al. (2008). The health effects of nonindustrial indoor air pollution. J. Allergy Clin. Immunol. 121, 585–591. doi: 10.1016/j.jaci.2007.10.045
Brasche, S., Bullinger, M., Gebhardt, H., Herzog, V., Hornung, P., Kruppa, B., et al. (1999). Factors determining different symptom patterns of sick building syndrome-results from a multivariate analysis. Proc. Indoor Air. 99, 402–407.
Britigan, N., Alshawa, A., and Nizkorodov, S. A. (2006). Quantification of ozone levels in indoor environments generated by ionization and ozonolysis air purifiers. J. Air Waste Manag. Assoc. 56, 601–610. doi: 10.1080/10473289.2006.10464467
Bulgarelli, D., Schlaeppi, K., Spaepen, S., van Themaat, E. V. L., and Schulze-Lefert, P. (2013). Structure and functions of the bacterial microbiota of plants. Annu. Rev. Plant Biol. 64, 807–838. doi: 10.1146/annurev-arplant-050312-120106
Burchett, M., Torpy, F., De Filippis, L., Brennan, J., and Irga, P. J. (2011). Indoor-Plant Technology for Health and Environmental Sustainability. Sydney, Australia: Horticulture Australia.
Cetin, M., and Sevik, H. (2016). Measuring the impact of selected plants on indoor CO2 concentrations. Pol. J. Environ. Stud. 25, 973–979. doi: 10.15244/pjoes/61744
Claudio, L. (2011). Planting Healthier Indoor Air, Vol. 119. National Institute of Environmental Health Sciences, United States. doi: 10.1289/ehp.119-a426
Clausen, G., Bek,ö, G., Corsi, R. L., Gunnarsen, L., Nazaroff, W. W., Olesen, B. W., et al. (2011). Reflections on the state of research: indoor environmental quality: reflections on the state of research. Indoor Air. 21, 219–230. doi: 10.1111/j.1600-0668.2010.00706.x
Cornejo, J. J., Munoz, F. G., Ma, C. Y., and Stewart, A. J. (1999). Studies on the decontamination of air by plants. Ecotoxicology. 8, 311–320. doi: 10.1023/A:1008937417598
Cruz, M. D., Christensen, J. H., Thomsen, J. D., and Müller, R. (2014). Can ornamental potted plants remove volatile organic compounds from indoor air?—a review. Environ. Sci. Pollut. Res. 21, 13909–13928. doi: 10.1007/s11356-014-3240-x
Cure, J. D., and Acock, B. (1986). Crop responses to carbon dioxide doubling: a literature survey. Agri. Forest Meteorol. 38, 127–145. doi: 10.1016/0168-1923(86)90054-7
D'Amato, G., Liccardi, G., D'Amato, M., and Cazzola, M. (2002). Outdoor air pollution, climatic changes and allergic bronchial asthma. Eur. Res. J. 20, 763–776. doi: 10.1183/09031936.02.00401402
Darlington, A. B., Dat, J. F., and Dixon, M. A. (2001). The biofiltration of indoor air: air flux and temperature influences the removal of toluene, ethylbenzene, and xylene. Environ. Sci. Technol. 35, 240–246. doi: 10.1021/es0010507
Deng, L., and Deng, Q. (2018). The basic roles of indoor plants in human health and comfort. Environ. Sci. Pollut. Res. 25, pp.36087–36101. doi: 10.1007/s11356-018-3554-1
Dingle, P., Tapsell, P., and Hu, S. (2000). Reducing formaldehyde exposure in office environments using plants. Bull. Environ. Contam. Toxicol. 64, 2. doi: 10.1007/s001289910044
Ercan, M. S. (2012). Your Compass Green “Environmental Indicator,” in X. International HVAC Technology Symposium. p. 30.
Fjeld, T., Veiersted, B., Sandvik, L., Riise, G., and Levy, F. (1998). The effect of indoor foliage plants on health and discomfort symptoms among office workers. Indoor and Built Env. 7, 204–209. doi: 10.1177/1420326X9800700404
Fujii, S., Cha, H., Kagi, N., Miyamura, H., and Kim, Y. S. (2005). Effects on air pollutant removal by plant absorption and adsorption. Build. Environ. 40, 105–112. doi: 10.1016/j.buildenv.2004.04.015
Gawrońska, H., and Bakera, B. (2015). Phytoremediation of particulate matter from indoor air by Chlorophytum comosum L. plants. Air Qual. Atmos. Health. 8, 265–272. doi: 10.1007/s11869-014-0285-4
Ge, E., Lai, K., Xiao, X., Luo, M., Fang, Z., Zeng, Y., et al. (2018). Differential effects of size-specific particulate matter on emergency department visits for respiratory and cardiovascular diseases in Guangzhou, China. Environ. Pollut. 243, 336–345. doi: 10.1016/j.envpol.2018.08.068
Giese, M., Bauer-Doranth, U., Langebartels, C., and Sandermann, H. Jr (1994). Detoxification of formaldehyde by the spider plant (Chlorophytum comosum L.) and by soybean (Glycine max L.) cell-suspension cultures. Plant Physiol. 104, 1301–1309. doi: 10.1104/pp.104.4.1301
Gulia, S., Shukla, N., Padhi, L., Bosu, P., Goyal, S. K., and Kumar, R. (2021). Evolution of air pollution management policies and research gap assessment in India. Int. J. Environ. Probl. 100431. doi: 10.1016/j.envc.2021.100431
Han, K.-T. (2009). Influence of limitedly visible leafy indoor plants on the psychology, behavior, and health of students at a junior high school in Taiwan. Environ. Beh. 41, 658–692. doi: 10.1177/0013916508314476
Han, K. T., and Ruan, L. W. (2020). Effects of indoor plants on air quality: a systematic review. Environ. Sci. Pollut. Res. 27, 16019–16051. doi: 10.1007/s11356-020-08174-9
Hörmann, V., Brenske, K. R., and Ulrichs, C. (2018). Assessment of filtration efficiency and physiological responses of selected plant species to indoor air pollutants (toluene and 2-ethylhexanol) under chamber conditions. Environ. Sci. Pollut. Res. 25, 447–458. doi: 10.1007/s11356-017-0453-9
International Agency for Research on Cancer (IARC) (2006). Formaldehyde, 2-Butoxyethanol and 1-tert-Butoxypropanol-2-ol. IARC Monographs on the Evaluation of Carcinogenic Risks to Humans, Vol. 88. Lyon: World Health Organization. p. 39–325.
Irga, P. J., Torpy, F. R., and Burchett, M. D. (2013). Can hydroculture be used to enhance the performance of indoor plants for the removal of air pollutants?. Atmos. Environ. 77, 267–271. doi: 10.1016/j.atmosenv.2013.04.078
Jeong, N. R., Kim, K. J., Yoon, J. H., Han, S. W., and You, S. (2020). Evaluation on the potential of 18 species of indoor plants to reduce particulate matter. J. People Plants Environ 23, pp.637–646. doi: 10.11628/ksppe.2020.23.6.637
Jia, Y., Wu, C., Dong, C. F., Li, C. P., and Liao, H. M. (2012). Measurement on ability of dust removal of seven green plants at micro-conditions. J. Cent. South Univ. Technol. 43, 4547–4553.
Kaur-Sidhu, M., Ravindra, K., Mor, S., and John, S. (2020). Emission factors and global warming potential of various solid biomass fuel-cook stove combinations. Atmos. Pollut. Res. 11, 252–260. doi: 10.1016/j.apr.2019.10.009
Kichah, A., Bournet, P.-E., Migeon, C., and Boulard, T. (2012). Measurement and CFD simulation of microclimate characteristics and transpiration of an Impatiens pot plant crop in a greenhouse. Biosyst. Eng. 112, 22–34. doi: 10.1016/j.biosystemseng.2012.01.012
Kim, K., and Kim, H. (2008). Development of model and calculating equation for rate of volatile formaldehyde removal of indoor plants. Hortic. Environ. Biotechnol. 49, 155–161. Available online at: https://www.dbpia.co.kr/Journal/articleDetail?nodeId=NODE01013352
Kim, K. J., Khalekuzzaman, M.d., Suh, J. N., Kim, H. J., Shagol, C., Kim, H.-H., et al. (2018). Phytoremediation of volatile organic compounds by indoor plants: a review. Hortic. Environ. Biotechnol. 59, 143–157. doi: 10.1007/s13580-018-0032-0
Klepeis, N. E., Nelson, W. C., Ott, W. R., Robinson, J. P., Tsang, A. M., Switzer, P., et al. (2001). The national human activity pattern survey (NHAPS): a resource for assessing exposure to environmental pollutants. J. Expo. Sci. Environ. Epidemiol. 11, 231–252. doi: 10.1038/sj.jea.7500165
Kondo, T., Hasegawa, K., Uchida, R., Onishi, M., Mizukami, A., and Omasa, K. (1995). Absorption of formaldehyde by oleander (Nerium indicum). Environ. Sci. Technol. 29, 2901–2903. doi: 10.1021/es00011a030
Liu, C., Colón, B. C., Ziesack, M., Silver, P. A., and Nocera, D. G. (2016). Water splitting–biosynthetic system with CO 2 reduction efficiencies exceeding photosynthesis. Science. 352, 1210–1213. doi: 10.1126/science.aaf5039
Liu, Y.-J., Mu, Y.-J., Zhu, Y.-G., Ding, H., and Crystal Arens, N. (2007). Which ornamental plant species effectively remove benzene from indoor air? Atmospheric Environ. 41, 650–654. doi: 10.1016/j.atmosenv.2006.08.001
Llewellyn, D., and Dixon, M. (2011). “Can Plants Really Improve Indoor Air Quality?,” in Comprehensive Biotechnology, Vol. 4, ed M.-Y. Murray (Amsterdam: Elsevier), 331–338. doi: 10.1016/B978-0-08-088504-9.00325-1
Lu, C., Deng, Q., Li, Y., Sundell, J., and Norbäck, D. (2016). Outdoor air pollution, meteorological conditions and indoor factors in dwellings in relation to sick building syndrome (SBS) among adults in China. Sci. Total Environ. 560, 186–196. doi: 10.1016/j.scitotenv.2016.04.033
Lu, S., Yang, X., Li, S., Chen, B., Jiang, Y., Wang, D., et al. (2018). Effects of plant leaf surface and different pollution levels on PM2. 5 adsorption capacity. Urban Forestry Urban Green. 34, 64–70. doi: 10.1016/j.ufug.2018.05.006
Manisalidis, I., Stavropoulou, E., Stavropoulos, A., and Bezirtzoglou, E. (2020). Environmental and health impacts of air pollution: a review. Front. Public Health. 8, 14. doi: 10.3389/fpubh.2020.00014
Masiol, M., Hofer, A., Squizzato, S., Piazza, R., Rampazzo, G., and Pavoni, B. (2012). Carcinogenic and mutagenic risk associated to airborne particle-phase polycyclic aromatic hydrocarbons: a source apportionment. Atmos. Environ. 60, 375–382. doi: 10.1016/j.atmosenv.2012.06.073
Mendell, M. J. (2007). Indoor residential chemical emissions as risk factors for respiratory and allergic effects in children: a review. Indoor Air. 17, 259–277. doi: 10.1111/j.1600-0668.2007.00478.x
Morawska, L., Afshari, A., Bae, G. N., Buonanno, G., Chao, C. Y. H., Hänninen, O., et al. (2013). Indoor aerosols: from personal exposure to risk assessment. Indoor Air. 23, 462–487. doi: 10.1111/ina.12044
Moya, T. A., van den Dobbelsteen, A., Ottele, M., and Bluyssen, P. M. (2019). A review of green systems within the indoor environment. Indoor Built Environ. 28, 298–309. doi: 10.1177/1420326X18783042
Mozaffar, A., and Zhang, Y. L. (2020). Atmospheric volatile organic compounds (VOCs) in China: a review. Curr. Pollut. Rep. 1–14. doi: 10.1007/s40726-020-00149-1
Myers, I., and Maynard, R. L. (2005). Polluted air—outdoors and indoors. Occup. Med. 55, 432–438. doi: 10.1093/occmed/kqi137
Newman, L. A., and Reynolds, C. M. (2004). Phytodegradation of organic compounds. Curr. Opin. Biotechnol. 15, 225–230. doi: 10.1016/j.copbio.2004.04.006
Nielsen, G. D., and Wolkoff, P. (2010). Cancer effects of formaldehyde: a proposal for an indoor air guideline value. Archives Toxicol. 84, 423–446. doi: 10.1007/s00204-010-0549-1
Orwell, R. L., Wood, R. A., Burchett, M. D., Tarran, J., and Torpy, F. (2006). The potted-plant microcosm substantially reduces indoor air VOC pollution: II. laboratory study. Water, Air, and Soil Pollution. 177, 59–80. doi: 10.1007/s11270-006-9092-3
Orwell, R. L., Wood, R. L., Tarran, J., Torpy, F., and Burchett, M. D. (2004). Removal of benzene by the indoor plant/substrate microcosm and implications for air quality. Water, Air, and Soil Pollution. 157, 193–207. doi: 10.1023/B:WATE.0000038896.55713.5b
Pandey, A., Brauer, M., Cropper, M. L., Balakrishnan, K., Mathur, P., Dey, S., et al. (2021). Health and economic impact of air pollution in the states of India: the Global Burden of Disease Study 2019. Lancet Planetary Health. 5, e25–e38. doi: 10.1016/S2542-5196(20)30298-9
Park, S.-H., and Mattson, R. H. (2009). Ornamental indoor plants in hospital rooms enhanced health outcomes of patients recovering from surgery. J. Altern Complement Med. 15, 975–980. doi: 10.1089/acm.2009.0075
Perez, V., Alexander, D. D., and Bailey, W. H. (2013). Air ions and mood outcomes: a review and meta-analysis. BMC Psychiatry 13, 1–20. doi: 10.1186/1471-244X-13-29
Persily, A. K., and Gorfain, J. (2004). Analysis of ventilation data from the US Environmental Protection Agency building assessment survey and evaluation (BASE) study. US Department of Commerce, Technology Administration, National Institute of Standards and Technology, Building and Fire Research Laboratory, NIST Interagency/Internal Report (NISTIR), Report Number 7145-R. Available online at: https://www.nist.gov/publications/analysis-ventilation-data-us-environmental-protection-agency-building-assessment-0?pub_id=100966
Pettit, T., Irga, P. J., and Torpy, F. R. (2018). Towards practical indoor air phytoremediation: a review. Chemosphere. 208, 960–974. doi: 10.1016/j.chemosphere.2018.06.048
Raanaas, R., Evensen, K., Rich, D., Sjøstrøm, G., and Patil, G. (2011). Benefits of indoor plants on attention capacity in an office setting. J. Environ. Psychol. 31, 99–105. doi: 10.1016/j.jenvp.2010.11.005
Rai, P. K. (2015). “Multifaceted health impacts of particulate matter (PM) and its management: an overview,” in Environmental Skeptics and Critics. p. 4, 1.
Ravindra, K. (2019). Emission of black carbon from rural households kitchens and assessment of lifetime excess cancer risk in villages of North India. Environ. Int. 122, 201–212. doi: 10.1016/j.envint.2018.11.008
Ravindra, K., Bencs, L., Wauters, E., De Hoog, J., Deutsch, F., Roekens, E., et al. (2006). Seasonal and site-specific variation in vapour and aerosol phase PAHs over Flanders (Belgium) and their relation with anthropogenic activities. Atmos. Environ. 40, 771–785. doi: 10.1016/j.atmosenv.2005.10.011
Ravindra, K., Goyal, A., Kumar, S., Aggarwal, A., and Mor, S. (2021a). Pollen Calendar to depict seasonal periodicities of airborne pollen species in a city situated in Indo-Gangetic plain, India. Atmos. Environ. 118649. doi: 10.1016/j.atmosenv.2021.118649
Ravindra, K., Kaur-Sidhu, M., and Mor, S. (2020). “Air pollution in rural households due to solid biomass fuel use and its health impacts,” in Indoor Environmental Quality. Singapore: Springer. p. 27–33. doi: 10.1007/978-981-15-1334-3_4
Ravindra, K., Kaur-Sidhu, M., Mor, S., Chakma, J., and Pillarisetti, A. (2021b). Impact of the COVID-19 pandemic on clean fuel programmes in India and ensuring sustainability for household energy needs. Environ. Int. 147, 106335. doi: 10.1016/j.envint.2020.106335
Ravindra, K., Mittal, A. K., and Van Grieken, R. (2001). Health risk assessment of urban suspended particulate matter with special reference to polycyclic aromatic hydrocarbons: a review. Rev. Environ. Health 16, 169–190. doi: 10.1515/REVEH.2001.16.3.169
Ravindra, K., Sokhi, R., and Van Grieken, R. (2008). Atmospheric polycyclic aromatic hydrocarbons: source attribution, emission factors and regulation. Atmos. Environ. 42, 2895–2921. doi: 10.1016/j.atmosenv.2007.12.010
Rylott, E. L., and Bruce, N. C. (2009). Plants disarm soil: engineering plants for the phytoremediation of explosives. Trends Biotechnol. 27, 73–81. doi: 10.1016/j.tibtech.2008.11.001
Saravia, J., Lee, G. I., Lomnicki, S., Dellinger, B., and Cormier, S. A. (2013). Particulate matter containing environmentally persistent free radicals and adverse infant respiratory health effects: a review: PM, EPFRS, and infant respiratory health. J. Biochem. Mol. Toxicol. 27, 56–68. doi: 10.1002/jbt.21465
Saxena, P., and Sonwani, S. (2020). Remediation of ozone pollution by ornamental plants in indoor environment. Glob. J. Environ. Sci. Manag. 6, 497–508. doi: 10.22034/gjesm.2020.04.06
Seppanen, O., Fisk, W. J., and Lei, Q. H. (2005). Ventilation and Work Performance in Office Work. doi: 10.1111/j.1600-0668.2005.00394.x
Sharma, K., Ravindra, K., Mor, S., Kaur-Sidhu, M., and Sehgal, R. (2019). Detection and identification of dust mite allergens in the air conditioning filters in Chandigarh, India. Environ. Sci. Pollut. Res. 26, 24262–24271. doi: 10.1007/s11356-019-05574-4
Shetty, B. S. P., D'Souza, G., and Padukudru Anand, M. (2021). Effect of indoor air pollution on chronic obstructive pulmonary disease (COPD) deaths in southern asia—a systematic review and meta-analysis. Toxics. 9, 85. doi: 10.3390/toxics9040085
Shibata, S., and Suzuki, N. (2004). Effects of an indoor plant on creative task performance and mood. Scandinavian J. Psychol. 45, 373–381. doi: 10.1111/j.1467-9450.2004.00419.x
Shinohara, N., Mizukoshi, A., and Yanagisawa, Y. (2004). Identification of responsible volatile chemicals that induce hypersensitive reactions to multiple chemical sensitivity patients. J. Expo. Sci. Environ. Epidemiol. 14, 84–91. doi: 10.1038/sj.jea.7500303
Shiue, A., Hu, S. C., and Tu, M. L. (2011). Particles removal by negative ionic air purifier in cleanroom. Aerosol Air Qual. Res. 11, 179–186. doi: 10.4209/aaqr.2010.06.0048
Simoni, M., Carrozzi, L., Baldacci, S., Scognamiglio, A., Pede, F., di, Sapigni, T., et al. (2002). The Po River Delta (north Italy) indoor epidemiological study: effects of pollutant exposure on acute respiratory symptoms and respiratory function in adults. Arch. Environ. Health: An Int. J. 57, 130–136. doi: 10.1080/00039890209602928
Snyder, R. (2012). Leukemia and benzene. Int. J. Environ. Res. Public Health. 9, 2875–2893. doi: 10.3390/ijerph9082875
Son, K., Lee, S., Seo, S., and Song, J. (2000). Effects of foliage plants and potting soil on the absorption and adsorption of indoor air pollutants. J. Korean Society Horticultural Sci. 41, 305–310. Available online at: https://www.cabdirect.org/cabdirect/abstract/20000314569
Sonwani, S., Madaan, S., Arora, J., Suryanarayan, S., Rangra, D., Mongia, N., et al. (2021). Inhalation exposure to atmospheric nanoparticles and its associated impacts on human health: A review. Front. Sustain Cities. 3, 690444. doi: 10.3389/frsc.2021.690444
Sonwani, S., Saxena, P., and Khillare, P. S. (2022). Profile of atmospheric particulate PAHs near busy roadway in tropical megacity, India. Inhalat. Toxicol. 34, 39–50. doi: 10.1080/08958378.2022.2030442
Soreanu, G., Dixon, M., and Darlington, A. (2013). Botanical biofiltration of indoor gaseous pollutants – a mini-review. Chem. Eng. J. 229, 585–594. doi: 10.1016/j.cej.2013.06.074
Sorkhoh, N. A., Al-Mailem, D. M., Ali, N., Al-Awadhi, H., Salamah, S., Eliyas, M., et al. (2011). Bioremediation of volatile oil hydrocarbons by epiphytic bacteria associated with American grass (Cynodon sp.) and broad bean (Vicia faba) leaves. Int. Biodeteriorat. Biodegradat. 65, 797–802. doi: 10.1016/j.ibiod.2011.01.013
Sriprapat, W., Boraphech, P., and Thiravetyan, P. (2014a). Factors affecting xylene-contaminated air removal by the ornamental plant Zamioculcas zamiifolia. Environ. Sci. Pollut. Res. 21, 2603–2610. doi: 10.1007/s11356-013-2175-y
Sriprapat, W., Suksabye, P., Areephak, S., Klantup, P., Waraha, A., Sawattan, A., et al. (2014b). Uptake of toluene and ethylbenzene by plants: removal of volatile indoor air contaminants. Ecotoxicol. Environ. Saf. 102, 147–151. doi: 10.1016/j.ecoenv.2014.01.032
Sriprapat, W., and Thiravetyan, P. (2013). Phytoremediation of BTEX from indoor air by Zamioculcas zamiifolia. Water Air Soil Pollut. 224, 1482. doi: 10.1007/s11270-013-1482-8
Susanto, A. D., Winardi, W., Hidayat, M., and Wirawan, A. (2021). The use of indoor plant as an alternative strategy to improve indoor air quality in Indonesia. Rev. Environ. Health 36, 95–99. doi: 10.1515/reveh-2020-0062
Tani, A., and Hewitt, C. N. (2009). Uptake of aldehydes and ketones at typical indoor concentrations by houseplants. Environ. Sci. Technol. 43, 8338–8343. doi: 10.1021/es9020316
Treesubsuntorn, C., and Thiravetyan, P. (2012). Removal of benzene from indoor air by Dracaena sanderiana: effect of wax and stomata. Atmos. Environ. 57, 317–321. doi: 10.1016/j.atmosenv.2012.04.016
Upadhyay, S., Ganguly, K., and Stoeger, T. (2014). Inhaled ambient particulate matter and lung health burden. Eur. Medical J. Available online at: http://Emjreviews.Com/ [Pada 21 Juni, 2017].
Wenzel, W. W. (2009). Rhizosphere processes and management in plant-assisted bioremediation (phytoremediation) of soils. Plant and Soil. 321, 385–408. doi: 10.1007/s11104-008-9686-1
Weyens, N., Thijs, S., Popek, R., Witters, N., Przybysz, A., Espenshade, J., et al. (2015). The role of plant-microbe interactions and their exploitation for phytoremediation of air pollutants. Int. J. Molecular Sci. 16, 25576–25604. doi: 10.3390/ijms161025576
Weyens, N., van der Lelie, D., Taghavi, S., Newman, L., and Vangronsveld, J. (2009). Exploiting plant-microbe partnerships to improve biomass production and remediation. Trends in Biotechnol. 27, 591–598. doi: 10.1016/j.tibtech.2009.07.006
WMO (2018). WMO Greenhouse Gas Bulletin (GHG Bulletin) - No. 14: The State of Greenhouse Gases in the Atmosphere Based on Global Observations through 2017. WMO.
Wolverton, B. C., Johnson, A., and Bounds, K. (1989). Interior Landscape Plants for Indoor Air Pollution Abatement (No. NASA-TM-101766). Technical Memorandum (TM). Available online at: https://ntrs.nasa.gov/citations/19930073077
Wood, R. A., Orwell, R. L., Tarran, J., Torpy, F., and Burchett, M. (2002). Potted-plant/growth media interactions and capacities for removal of volatiles from indoor air. J. Hortic. Sci. Biotechnol. 77, 120–129. doi: 10.1080/14620316.2002.11511467
World Health Organization (2014). WHO Guidelines for Indoor Air Quality: Household Fuel Combustion. World Health Organization.
World Health Organization (2016). Ambient Air Pollution: A Global Assessment of Exposure and Burden of Disease. Available online at: https://apps.who.int/iris/handle/10665/250141
Wu, C. C., and Lee, G. W. (2004). Oxidation of volatile organic compounds by negative air ions. Atmos. Environ. 38, 6287–6295. doi: 10.1016/j.atmosenv.2004.08.035
Wu, W., Jin, Y., and Carlsten, C. (2018). Inflammatory health effects of indoor and outdoor particulate matter. J. Allergy Clin. Immunol. 141, 833–844. doi: 10.1016/j.jaci.2017.12.981
Xu, Z., Wang, L., and Hou, H. (2011). Formaldehyde removal by potted plant–soil systems. J. Hazard. Mater. 192, 314–318. doi: 10.1016/j.jhazmat.2011.05.020
Yan, X., Wang, H., Hou, Z., Wang, S., Zhang, D., Xu, Q., et al. (2015). Spatial analysis of the ecological effects of negative air ions in urban vegetated areas: a case study in Maiji, China. Urban Forestry Urban Green. 14, 636–645. doi: 10.1016/j.ufug.2015.06.010
Yang, D. S., Pennisi, S. V., Son, K.-C., and Kays, S. J. (2009). Screening indoor plants for volatile organic pollutant removal efficiency. HortScience. 44, 1377–1381. doi: 10.21273/HORTSCI.44.5.1377
Yu, B. F., Hu, Z. B., Liu, M., Yang, H. L., Kong, Q. X., and Liu, Y. H. (2009). Review of research on air-conditioning systems and indoor air quality control for human health. Int. J. Refrigerat. 32, 3–20. doi: 10.1016/j.ijrefrig.2008.05.004
Yu, C., and Crump, D. (1998). A review of the emission of VOCs from polymeric materials used in buildings. Build. Environ. 33, 357–374. doi: 10.1016/S0360-1323(97)00055-3
Zeiger, E., and Field, C. (1982). Photocontrol of the functional coupling between photosynthesis and stomatal conductance in the intact leaf: Blue light and PAR-dependent photosystems in guard cells. Plant Physiol. 70, 370–375. doi: 10.1104/pp.70.2.370
Zhang, J. J., and Smith, K. R. (2003). Indoor air pollution: a global problem. Br. Med. Bull. 68, 209–225. doi: 10.1093/bmb/ldg029
Zhang, Q., Meng, X., Shi, S., Kan, L., Chen, R., and Kan, H. (2022). Overview of particulate air pollution and human health in China: evidence, challenges, and opportunities. The Innovation. 100312. doi: 10.1016/j.xinn.2022.100312
Zhou, J., Qin, F., Su, J., Liao, J., and Xu, H. (2011). Purification of formaldehyde-polluted air by indoor plants of Araceae, Agavaceae and Liliaceae. J. Food Agri. Environ. 9, 1012–1018. Available online at: https://www.researchgate.net/profile/Hui-Lian-Xu/publication/231184098_Purification_of_formaldehyde-polluted_air_by_indoor_plants_of_Araceae_Agavaceae_and_Liliaceae/links/0fcfd5065a101d697c000000/Purification-of-formaldehyde-polluted-air-by-indoor-plants-of-Araceae-Agavaceae-and-Liliaceae.pdf
Žuškin, E., Schachter, E. N., Mustajbegović, J., Pucarin-Cvetković, J., Doko-Jelinić, J., et al. (2009). Indoor air pollution and effects on human health. Period. Biol. 111, 37–40. Available online at: https://hrcak.srce.hr/en/file/56767
Keywords: indoor air quality (IAQ), air pollutants, removal efficiency, indoor plants, air remediation
Citation: Ravindra K and Mor S (2022) Phytoremediation potential of indoor plants in reducing air pollutants. Front. Sustain. Cities 4:1039710. doi: 10.3389/frsc.2022.1039710
Received: 08 September 2022; Accepted: 11 November 2022;
Published: 25 November 2022.
Edited by:
Prashant Rajput, Banaras Hindu University, IndiaReviewed by:
Pallavi Saxena, University of Delhi, IndiaSarkawt Hama, University of Surrey, United Kingdom
Copyright © 2022 Ravindra and Mor. This is an open-access article distributed under the terms of the Creative Commons Attribution License (CC BY). The use, distribution or reproduction in other forums is permitted, provided the original author(s) and the copyright owner(s) are credited and that the original publication in this journal is cited, in accordance with accepted academic practice. No use, distribution or reproduction is permitted which does not comply with these terms.
*Correspondence: Khaiwal Ravindra, a2hhaXdhbCYjeDAwMDQwO3lhaG9vLmNvbQ==; a2hhaXdhbC5yYXZpbmRyYSYjeDAwMDQwO3BnaW1lci5lZHUuaW4=; Suman Mor, c3VtYW5tb3ImI3gwMDA0MDt5YWhvby5jb20=
†These authors have contributed equally to this work