- 1Laboratorio de Interacciones y Procesos Ecológicos, Departamento de Ecología y Recursos Naturales, Facultad de Ciencias, Universidad Nacional Autónoma de México, Ciudad Universitaria, Mexico City, Mexico
- 2Laboratorio de Restauración Ecológica, Departamento de Zoología, Tercer Circuito Exterior, Instituto de Biología, Universidad Nacional Autónoma de México, Ciudad Universitaria, Mexico City, Mexico
- 3Instituto de Investigaciones en Ecosistemas y Sustentabilidad, Universidad Nacional Autónoma de México campus Morelia, Morelia, Mexico
As cities overgrow, the need for ecological restoration is becoming increasingly urgent, especially in densely populated areas. Urban ecological restoration represents the best approach to undertake damages to restore native ecosystem remnants fulfilling biodiversity but also social goals in dense urban settings such as Mexico City. The feasibility of restoring unique xerophytic scrub vegetation in lava field remnants was evaluated in a portion of a university campus in Mexico City. Here we present an index (Need and Feasibility of Restoration Index, NFRI) for such purpose. The NFRI was designed through multicriteria analysis and considered ecological, economic, and social indicators. Cluster and principal components analysis were carried out to identify different groups of lava field remnants with similar characteristics and to point out critical variables that in turn would support management strategies. The outcomes made evident the necessity of restoring native vegetation for all of the evaluated remnants; however, the group containing the largest ones obtained the highest values for restoration feasibility and NFRI. The recovery of the rest of the remnants is critical to support the ecological restoration of the area as this may provide connectivity with better-preserved ecosystem remnants. When the restoration is unaffordable due to financial constraints, it is highly recommended to direct efforts towards ecological rehabilitation actions. The establishment of native xerophytic gardens is promoted when remnants cannot support a self-sustainable plant community. It is crucial to include the diversity of views and values of the community and the economic and ecological aspects to guarantee the sustainability of the landscape, especially in the urban context. The latter can provide better planning and design processes, ensuring benefits for humans and nature.
Introduction
Around 70% of the human population will live in urban areas in 2050 (WHO, 2020). Cities are the places where people frequently experience nature through urban green spaces; nevertheless, the more people living in cities, the less room for nature and opportunities to be in contact with it and its multiple benefits (Cox et al., 2017). There is a dramatic tendency to a lower perceived value of green space in cities, threatening provision and its use (Kruize et al., 2019).
Some cities can host native ecosystem remnants within their urban matrix or surroundings. Compared to conventional urban green spaces like parks and gardens, native ecosystem remnants can provide specific ecosystem services (Carlucci et al., 2020) and host greater biodiversity including endangered plant species (Ives et al., 2016), animal species that require non-disturbed habitats (Kang et al., 2015) which also promote considerable psychological benefits for urban dwellers (Fuller et al., 2007). Moreover, these sites offer opportunities for people to develop awareness about the original ecosystem and contribute to the development of values and attitudes favoring biological conservation at a local and global scale (Olive, 2014).
Despite their importance, urban native ecosystem remnants are subjected to severe anthropogenic disturbances, posing a risk of its prevalence (Natale et al., 2015). Under an urban context, ecological restoration arises as a solution for conserving biodiversity, connecting people to nature (Standish et al., 2013), and strengthening s substantial nexus between human and ecological health (Zhou and Li, 2020; Reaser et al., 2021). Ecological restoration is the process by which the recovery of a damaged, degraded, or destroyed site is assisted considering the characteristics of a reference ecosystem (Gann et al., 2019). Social, economic, and cultural dimensions should be followed up carefully in each part of the restoration process to minimize conflicts and to reach the goals effectively (Christian-Smith and Merenlender, 2010).
While the ecological restoration of each of the damaged urban ecosystem remnants within a particular area is desirable, it might be unfeasible when severe financial constraints exist (Aguirre-Salado et al., 2017). Selecting the sites where the success of the restoration is elevated secures the goal's achievement and the maximizes benefits (Orsi and Geneletti, 2010). Furthermore, success probabilities may increase if expectations and direct involvement of stakeholders are considered throughout the designing and decision-making processes (Gann et al., 2019). Therefore, restoration objectives should consider the perceptions and values of communities, particularly in areas with high population density (Hychka and Druschke, 2017).
Restoration priority identification is seen as a multi-objective planning task in which social and economic aspects should be included (Guida-Johnson and Zuleta, 2017). A frequently used method for this purpose is multicriteria analysis (MCA). In this analysis, ecological, economic, and social variables are evaluated and used to calculate a value representing the suitability and priority for restoring a site (Orsi et al., 2011). This paper aims to present a case study highlighting the importance of planning and designing ecological restoration projects in densely populated urban areas. Specifically, the study used an index built through MCA to evaluate the need and the feasibility for restoring native vegetation in Mexico City, one of the biggest and crowded cities in the world. Our model comprised some of the lava field remnants and green spaces within the main campus of the National Autonomous University of Mexico (UNAM). These sites are outside a university's protected area (see below). Therefore, their restoration is crucial for increasing buffer zones, creating inter-connection corridors among remnants, improving habitat quality, and making this ecosystem closer to the university community to receive benefits and to contribute to its conservation (Camarena, 2010; Lara-Deras, 2016). Moreover, it is expected that this study may contribute to urgently recover and keeping the linkages between human and ecosystems health (Reaser et al., 2021) and to guarantee the sustainability of landscape, especially in urban contexts.
Methods
Study Site
The main campus of the UNAM hosts the Ecological Reserve of the Pedregal of San Angel (REPSA, for its acronym in Spanish) which protects 237.3 ha (Peralta-Higuera and Prado-Molina, 2009) of one of the last native vegetation relicts within Mexico City. These correspond to xerophytic scrub that grows on a lava field, and this unique urban ecosystem also exists outside the reserve. The substrate of the native ecosystem corresponds to basaltic rocks produced by the eruption of the Xitle volcano occurred in the year 280 ± 35 c.e. (Siebe, 2000). The complex topography of this substrate generates numerous microhabitats, and its porosity produces edaphic aridity, promoting the establishment of the xerophytic scrub (Peralta-Higuera and Prado-Molina, 2009). The sites under study are around the Olympic Stadium at the northwestern portion of the campus (Figure 1). Thirty-nine lava field remnants (7.16 ha) associated with green spaces were included in the analysis. These are located between 19°20'09” and 19°19'43” N and 99°11'23” and 99°11'52” W, at 2250 m a.s.l. The weather is temperate sub-humid with mean annual temperature of 15.6°C. The total mean annual precipitation is around 833 mm, and the rainy season is between June and October (Zambrano, 2016).
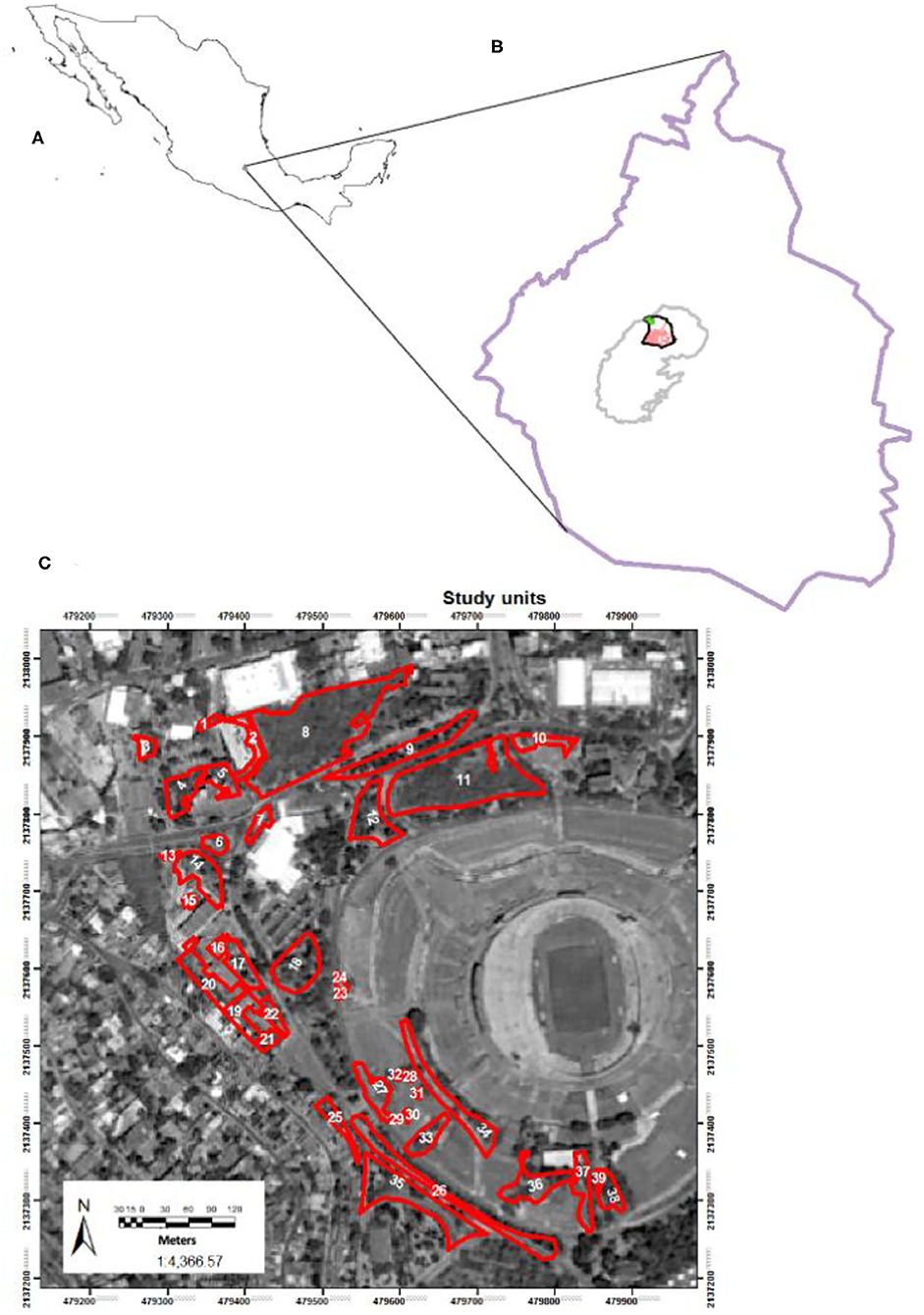
Figure 1. Study area and location of study sites. Location of Mexico City in Mexico (A); location of the lava field of San Ángel (grey polygon), University campus (black polygon), the Ecological Reserve of the Pedregal of San Ángel (red polygon) and the study area (green polygon) within Mexico City (purple polygon) (B); location of the 39 study sites (in red) around the University Olympic Stadium. This map was elaborated from a GIS archive of lava field remnants at the main campus provided in 2017 by the Department of Cartography and Geographic Information Systems of the Executive Secretary of the Ecological Reserve of the Pedregal of San Angel.
Survey
The degree to which a human community gives value to the biodiversity in a specific place can impact the success of environmental management actions and, therefore, restoration feasibility. This value can be economical, socio-cultural, or ecological. The way to evaluate the degree in which communities value biodiversity is context dependent (Laurila-Pant et al., 2015). In this study, a survey was conducted to document the opinion of the university community members regarding the priority of conservation and restoration actions, the conflicts between different types of land uses, and to know if people consider that the conservation of the native ecosystem may offer benefits to the community. See Supplementary Materials 1, 2 to consult the details for preparation and development of the survey and the survey formats, respectively.
Multicriteria Analysis Design
The design of the MCA used to build the index to evaluate the need and feasibility of restoration included conceptual considerations and methods from Orsi et al. (2011), Lithgow et al. (2015) and Tobón et al. (2017). The need and the feasibility of restoration, the main factors in the MCA design (Table 1) were evaluated through ecological criteria, and feasibility was also assessed with economic and social criteria. The criteria were identified through literature review and the expertise of people involved in vegetation restoration in lava fields within the campus. Indicators of specific aspects observed in the field and the literature were employed to evaluate criteria numerically between 0 and 10. Indicators were considered positive if the values in their original measuring scale had a direct relationship with the value assigned to the criteria and negative if they had an inverse relationship. See Supplementary Material 3 to review the methods used to evaluate the criteria and indicators of the MCA.
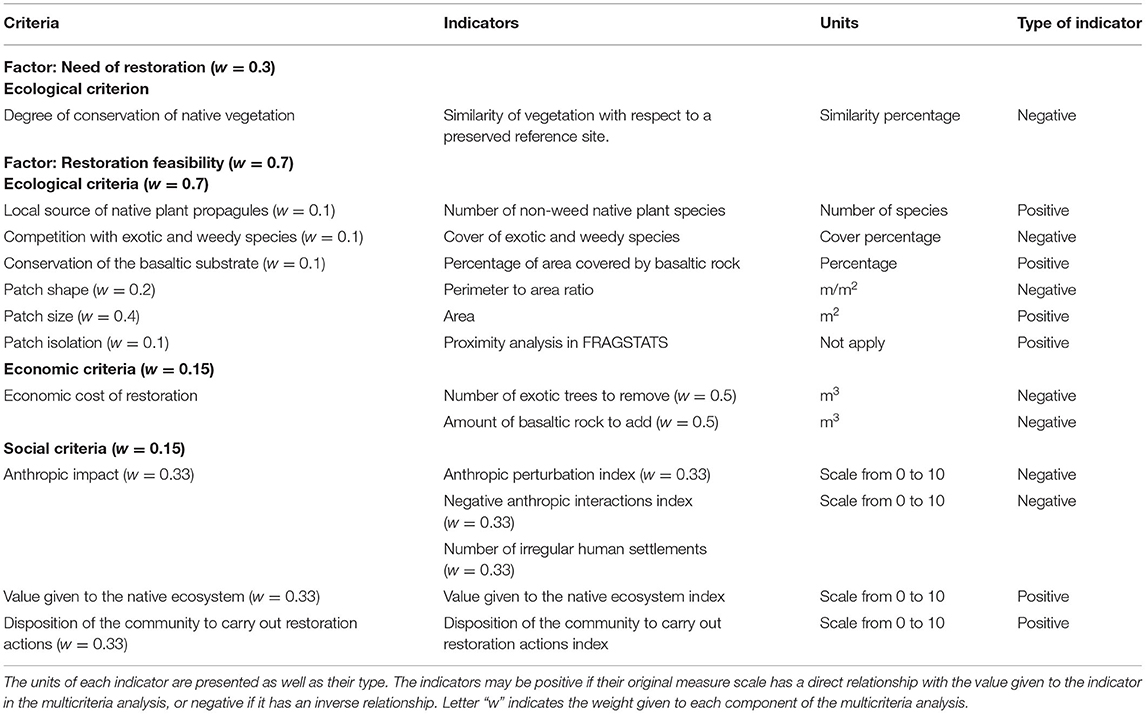
Table 1. Ecological, economic, and social criteria and indicators to evaluate the need and feasibility.
Each one of the factors was weighted (Table 1). Weights assigned to factors, type of restoration feasibility, criteria, and indicators (MCA elements) are denoted with the letter w. The weighing value was multiplied by the original value of the different MCA elements. Since patch size and shape (part of ecological feasibility) are important to determine the number and type of species a remnant can support (Forman, 1995) and might influence self-sustainability of the sites (Clewell and Aronson, 2013) different weights were assigned to each one of them (w = 0.4 and w = 0.2, respectively). The economic indicators received the same weight since collaboration with university entities involved in the quotation of restoration actions in monetary terms was not fruitful. Thus, it was unfeasible to distinguish the actions having significant importance in determining the total cost of the project. Social feasibility indicators and criteria also received equal weights.
The weighing method used by Tobón et al. (2017) was employed to assign different scores between ecological and socioeconomic feasibility. Mean values for both types of restoration feasibility were plotted for different weighing scenarios, and two lines were obtained; the point of interception corresponded to the optimal scenario (Figure 2A). A weight of 0.7 was assigned to ecological feasibility and of 0.3 to socioeconomic feasibility (Figure 2A). The latter was divided into equal parts (w = 0.15) between social and economic feasibility. The same method was applied to determine the weight assigned to the need (w = 0.3) and the feasibility factors (w = 0.7) (Figure 2B).
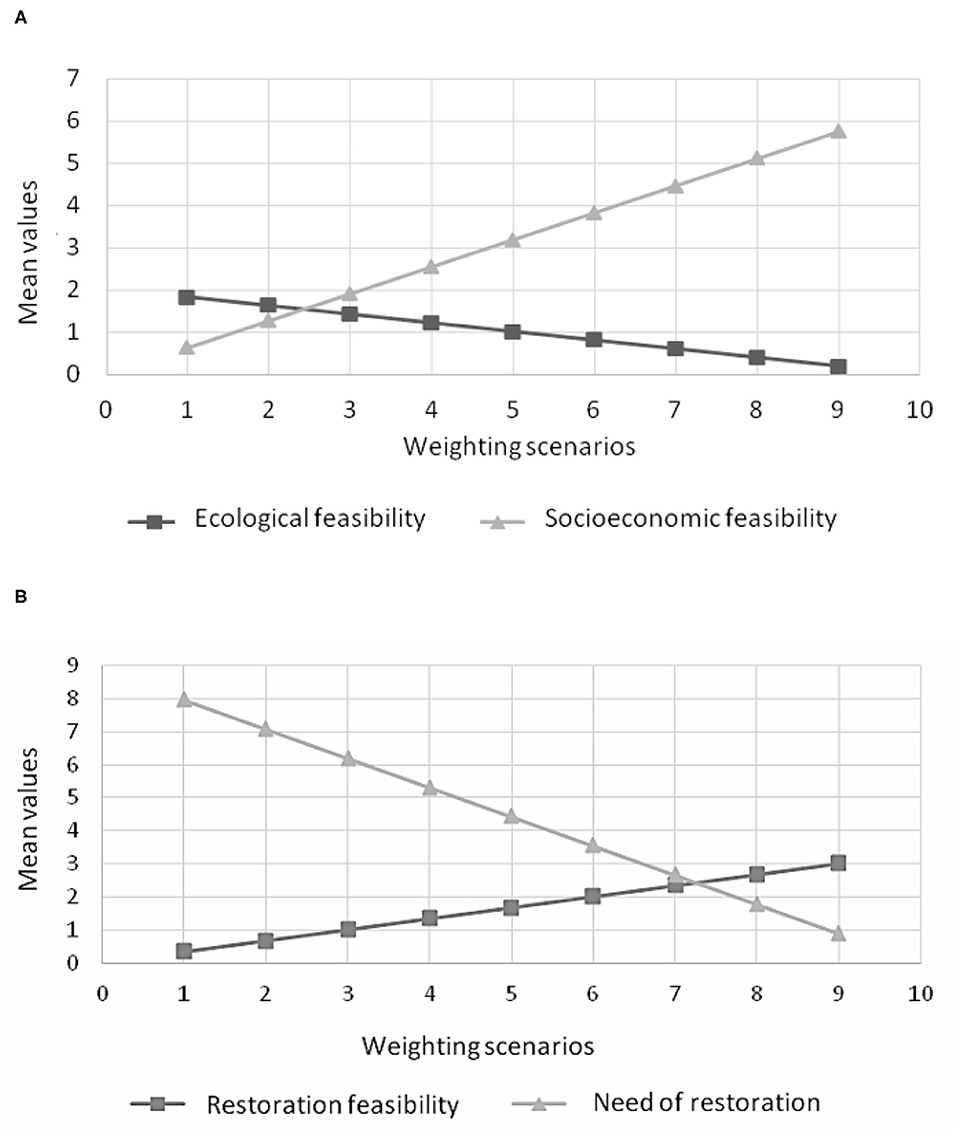
Figure 2. Graphs representing the mean values of the two factors of the multicriteria analysis and two types of restoration feasibility along different weighting scenarios. Graph for ecological and socioeconomic feasibility (A); graph for need and feasibility of restoration (B). Weighting scenario 1 starts with a weight of 0.1 and 0.9 to each variable respectively and scenario 9 correspond to a weight of 0.9 and 0.1 for the variables. In each scenario weights vary by 0.1 units.
To calculate the NFRI value to each site under study, we used the following equation:
where: N = value obtained for the need of restoration factor, wn = weight assigned to the need of restoration factor, wi = weight assigned to each type i of restoration feasibility (ecological, economic, or social feasibility), Fi = value obtained for each type i of restoration feasibility, wf = weight assigned to each f restoration feasibility factor, and m = number of types of restoration feasibility and respective weights.
The index and indicators criteria, types of restoration feasibility, and factors of the MCA were standardized from zero to ten. Then, the sites with the highest values of the NFRI have elevated priority and highest feasibility of restoration. This index is intended for use in sites with need of restoration greater than zero. In addition, ArcGIS10 (ESRI Inc., Redlands, CA, USA) was employed to build maps to visualized MCA results geographically. The coordinate system WGS 1984 UTM Zone 14N and Transverse Mercator Projection were used for all the maps presented.
Cluster and Principal Components Analysis
Standardized data of the MCA elements (Table 1) were used to implement a cluster analysis by the calculation of Euclidean distances and use complete linkage together with a principal component analysis (PCA) in R version 3.5.2 (R Core Team, 2018). The code and database used to conduct such analyses can be consulted in Supplementary Materials 4, 5, respectively. The complete linkage clustering method was chosen since it showed a high correlation (0.92, p-value <0.001) between calculated Euclidean distances and cophenetic distances (Legendre and Legendre, 2012). The dendrogram was cut to get four groups of study sites represented in the biplot obtained with the PCA. The statistical significance of the PC was tested by following the Broken stick method (Legendre and Legendre, 2012). The first and second PC were significant, making possible, their interpretation. These analyses were conducted to identify groups of sites with similar characteristics in terms of the MCA elements and where similar management strategies can be applied. The analyses were also used to identify MCA elements critical to determine variability between groups and define the NFRI.
Results
Index Values
An index (Need and Feasibility of Restoration Index, NFRI) based on multicriteria analysis, considering ecological, economic, and social indicators was designed and applied to assess the need and feasibility of restoring native vegetation in lava field remnants in Mexico City. Thirty-nine remnants at the main campus of the UNAM were evaluated; the outcomes indicated that all of them have a high need for restoration, but only some have high feasibility. For each site, values for MCA elements were obtained (Table 2). Regarding the need for restoration factor, the sites with values above six have priority to be restored (Figure 3A) since their vegetation structure differs more than 60% from reference sites. Most of the sites showed the need for restoration values between nine and 10 (Table 2), indicating that their xerophytic scrub native vegetation is highly degraded. Sites 8 and 11 had the lowest values of the need of restoration. However, these two sites showed the highest values (8.2 and 6.71, respectively) for the restoration feasibility factor, while the rest of the sites had values under three (Figure 3B). Moreover, for the NFRI of the native vegetation, it was also the site 8 the one with the highest value (Figure 3C), whereas the 13 showed the lowest value of all the 39 sites (see Table 2). Results for the different types of restoration feasibility are showed in Supplementary Material 6.
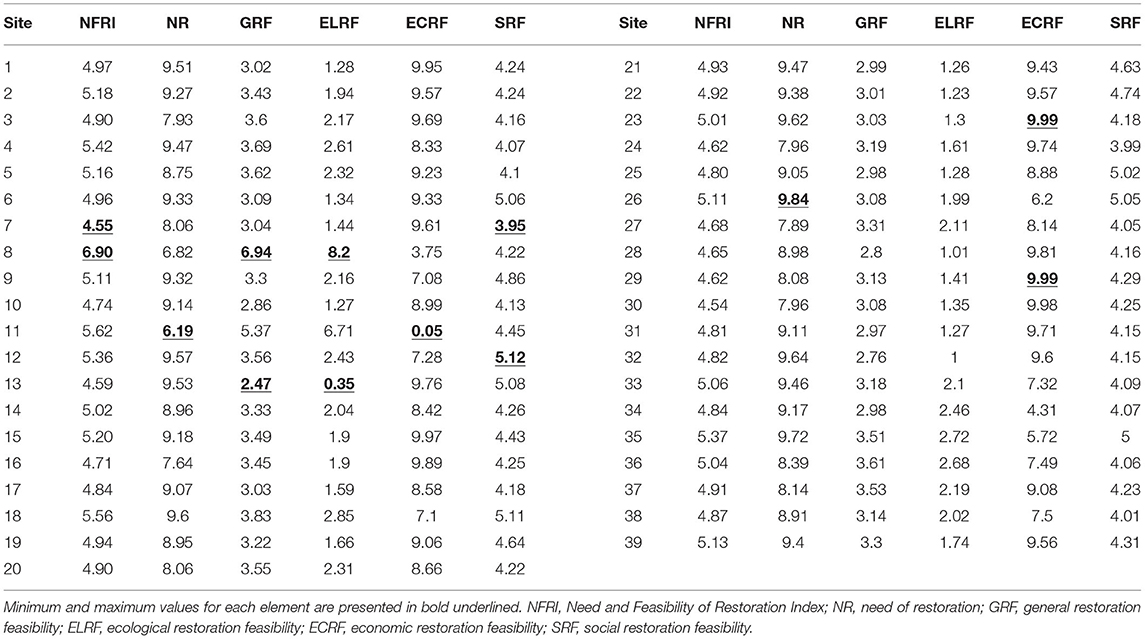
Table 2. Values obtained for the 39 study sites for the different elements of the multicriteria analysis.
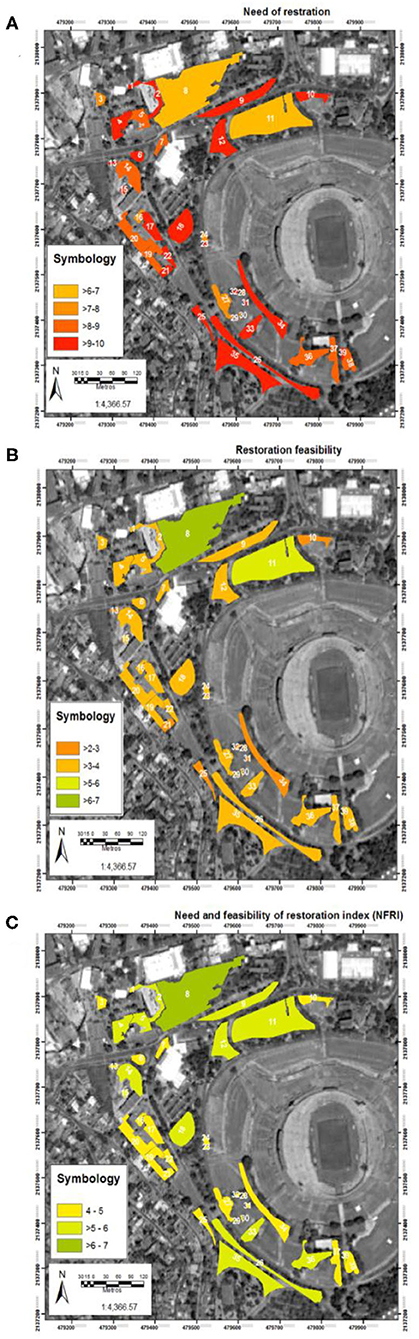
Figure 3. Maps showing the main results of the multicriteria analysis for the study sites around the University Olympic Stadium. Need of restoration (A); Restoration feasibility (B); Need and feasibility of restoration index (NFRI) (C).
Cluster and Classification Groups
The cluster and PCA showed that the PC1 and PC2 explained 68% of the total variance. Pearson correlation coefficients between the PC's are presented in Table 3. MCA elements that showed higher correlation with PC1 (>0.6, p < 0.001) are related to ecological and economic restoration feasibility, and particularly to the size and shape of the sites, number of native plant species, amount of basaltic rock to be added to and volume of exotic trees to be removed. For the social feasibility, the indicator of the number of irregular human settlements showed a high correlation (−0.901, p < 0.001) with PC1 since solely the most extensive sites (8 and 11) had irregular settlements. NFRI also showed a high correlation with PC1 (0.819, p < 0.001). MCA elements that showed a higher correlation with PC2 (>0.65, p < 0.001) mainly correspond to social feasibility criteria such as disposition of the local community and value of the native ecosystem. Competition with exotic and weed plant species and the degree of conservation of the basalt substrate criteria were also correlated to PC2.
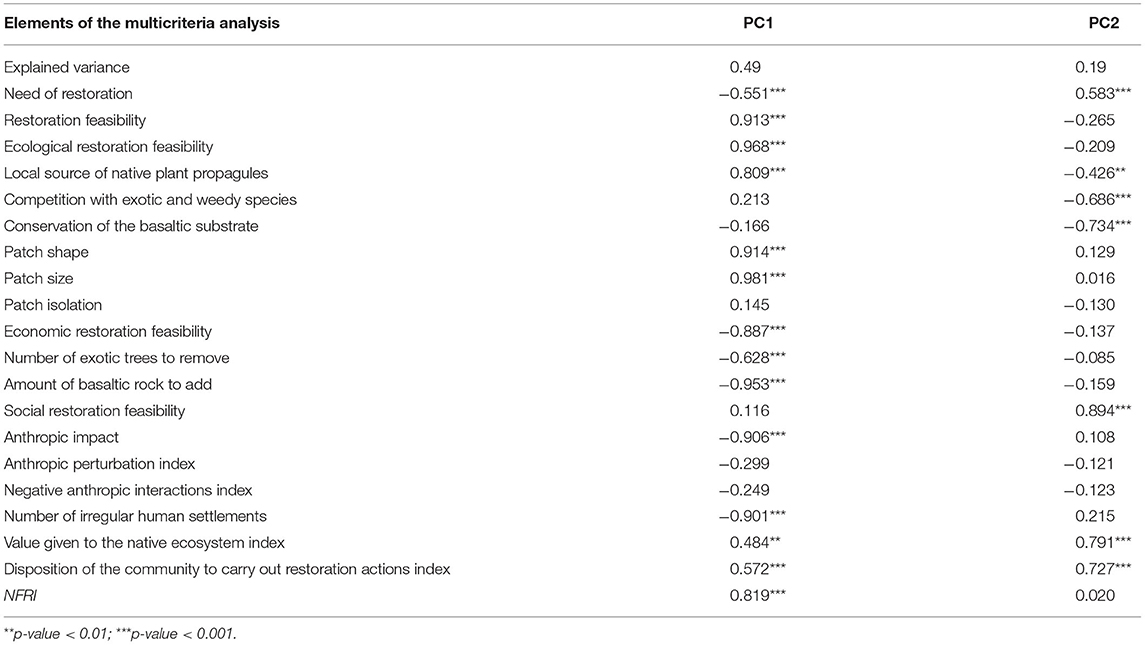
Table 3. Proportion of variance explained by the first two principal components (PC) and Pearson correlation of PC's with each element of the multicriteria analysis.
Through the analysis of classification group, the study sites were clustered in four groups. Figure 4 represents the results of cluster and PC analyses. Group 1 includes sites located within the university entities or in the stadium parking lot. In contrast with sites from group 3, these sites have better-preserved basalt substrate, and the dominance of exotic and weed plant species is lower than in the rest of the groups. Concerning social feasibility, sites from group 1 have lower values than group 3 because of the different opinions (in favor and against restoration) of the community with respect to the value given to the native ecosystem and disposition to carry out restoration actions. The sites conforming the group 2 (within the university entities and in the stadium parking lot) are relatively small and have a rugged topography preventing the access and reducing anthropic disturbance. The basalt substrate is relatively well-preserved and because of the small area few exotic trees should be removed and only added small volume of basaltic rocks. Study sites from group 3 are located outside university entities and in the stadium parking lot. The social feasibility value is also high since gardeners and street sweepers (actors closely related to these sites) thoroughly support the restoration and the rescue of lava field remnants. The economic restoration feasibility values for these sites go from high to intermediate, while the values for the ecological feasibility go from low to intermediate. This group has relatively low values for the degree of conservation of the basalt substrate, and the presence of exotic and weed plant species is evident. Group 4, comprising the largest and closer to each other sites with low isolation values, is distinguishable from the other three groups because of the high NFRI value due in part to the higher ecological feasibility.
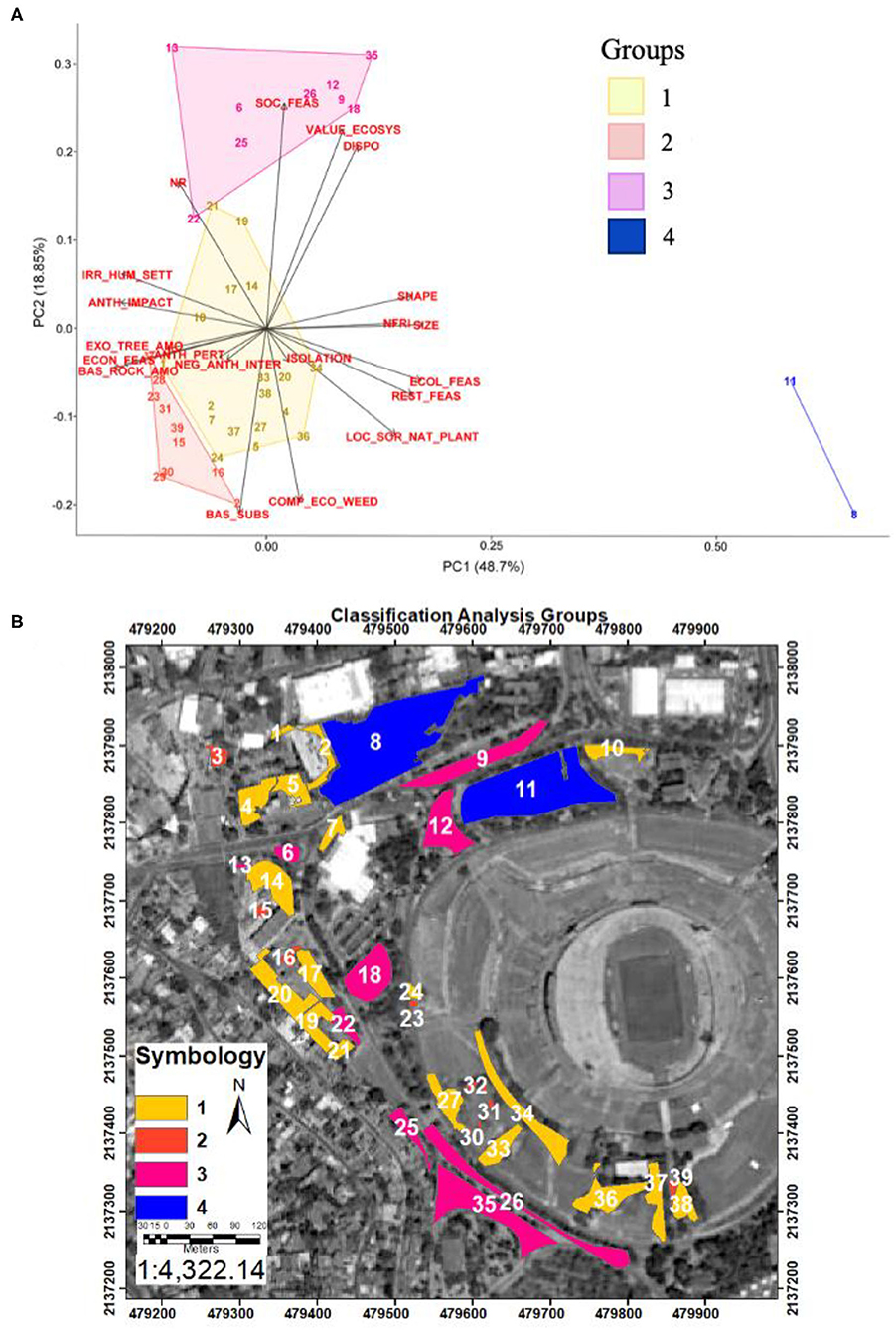
Figure 4. Classification and principal components analysis results. (A) biplot of the principal component analysis where the different colors of the study site numbers represent the four groups obtained in the classification analysis. NR, need of restoration; LOC_SOR_NAT_PLANT, local source of native plant propagules; COMP_ECO_WEED, competition with exotic and weedy species; SHAPE, patch shape; SIZE, patch size; ISOLATION, patch isolation; BAS_SUBS, conservation of the basaltic substrate; ECOL_FEAS, ecological restoration feasibility; BAS_ROCK_AMO, amount of basaltic rock to add; EXO_TREE_AMO, number of exotic trees to remove; ECON_FEAS, economic restoration feasibility; ANTH_PERT, anthropic perturbation index; NEG_ANTH_INTER, negative anthropic interactions index; IRR_HUM_SETT, number of irregular human settlements; ANTH_IMPACT, anthropic impact; VALUE_ECOSYS, value given to the native ecosystem index; DISPO, disposition of the community to carry out restoration actions index; SOC_FEAS, social feasibility; REST_FEAS, restoration feasibility; NFRI, Need and Feasibility of Restoration Index. (B) map showing the groups obtained in the classification analysis.
Discussion
This work relied on the design and application of a multicriteria analysis index (Need and Feasibility of Restoration Index, NFRI) to assess the need and the feasibility of restoring native vegetation in the lava field remnants in Mexico City. Ecological, economic, and social indicators were included for the design, and then it was applied in several lava field remnants within a campus university in Mexico City. The findings pointed out the urgent need to restore the native vegetation of the 39 remnants understudy and the importance of linking landscape to social-ecological indicators to conserve biodiversity and people's well-being.
Degradation of the native vegetation in the study sites around the university stadium is due mainly to anthropic perturbations. These sites are under severe pressure because of the urban matrix in which are immersed, and where impacts can be grievous concerning other types of landscape matrices (Vilà and Ibáñez, 2011). Some of the perturbations include change of the hydric regime by watering of green spaces, general removal of plant cover, deposition of organic and inorganic waste, the introduction of exotic species, and trampling of sites. Furthermore, the fragmentation of the ecosystem may have led to changes in the microclimatic conditions within the remnant patches by border effect that have facilitated the invasion of exotic and weed plant species, reducing native species richness (Saunders et al., 1991). In tropical forests, it is known that the border effect has negative impacts on the whole area of patches of <10 ha (Ceccon, 2013). For the xerophytic scrub at the study site, exotic invasive plant species associated with borders such as Cenchrus clandestinum can be found within the first 41 m from borderline of the REPSA core zones (unpublished data). It is desirable to conduct further studies in the campus to evaluate the border effect on xerophytic scrub vegetation and to identify the minimal size a patch should have to preserve the vegetation structure of reference sites. The largest remnants (approximately one ha) were already affected by exotic and weed plant species. Their vegetation structure differed as much as 60% concerning reference sites located within the core zones of the REPSA.
Large remnants showed less need for restoration since the vegetation community structure tends to be similar to well-preserved areas. In contrast, small patches, subjected to a greater border effect, had more significant vegetation degradation. This phenomenon may also be associated to relatively higher ecological restoration feasibility for larger remnants since the border effect is expected to be lower and native species could recolonize the sites. Sites 8 and 11 obtained the highest values for the NFRI (6.9 and 5.62, respectively; Table 2) mainly due to their high ecological restoration feasibility; hence, their recovery should be a priority. The ecological feasibility of these sites can be explained by their larger size and lower perimeter to area ratio that may reduce border effects and favor the presence of native species to recolonize degraded portions.
The recovery of the sites from group 4 could be economically expensive because of the loss of large portions of basalt substrate and the removal of countless exotic trees. Restoration actions in all of the sites with values of economic feasibility higher than 9 (53.8% of the sites) would be more affordable than restoration in only sites 8 and 11 due that 143 exotics trees need to be removed against 86 of the rest of the sites, whereas 47,873 m3 of rocks are to be added versus 18,069 m3. Moreover, the removal of irregular human settlements in group 4 sites could cause social conflicts.
In this complicated social-ecological scenario for large remnants, costly restoration actions could be reduced by directing efforts in areas that still maintain their original rocky substrate and conducting ecological rehabilitation in areas where the rocky substrate has been lost. This type of restoration implies removing inorganic wastes, protecting sites to avoid human incursions and their associated perturbation, establishing native plants that do not require a rocky substrate to thrive, and the control of exotic invasive species. Previous ecological restoration studies (see Estañol-Tecuatl and Cano-Santana, 2017) found that the addition of basaltic rocks does not necessarily promote the establishment of a xerophytic scrub; instead, it may favor the establishment of an arboreal stratum that is rooted in the ground under the rocks and preventing the establishment of exotic and weed species. Therefore, ecological rehabilitation represents an alternative for severely degraded areas of large remnants. Those whose ecological characteristics and degree of perturbation prevent the restoration of the original xerophytic scrub ecosystem.
In ecological terms, restoration or rehabilitation of small remnants could be a significant challenge due to the border effect and the high degradation of the native vegetation. Recovery strategies such as xeriscaping (replacing grassy lawns with soil, rocks, and native plant species) could be applied. Recovery actions include establishing and maintaining xerophytic gardens designed with native species, constant monitoring, and control of exotics (Camarena, 2010; Lara-Deras, 2016). This management may also positively affect the recovery of large remnants, for instance, eliminating invasive exotic species sources (Vilà and Ibáñez, 2011). Since several small remnants are located within university buildings, the xerophytic gardens can underscore the importance of the xerophytic scrub ecosystem to the community members. It is also an opportunity to promote community labor, participation, and the recovery of social bonds by developing gardening and conservation activities (Lara-Deras, 2016).
Actions of restoration, rehabilitation, or establishment of xerophytic gardens require to consider the diversity of opinions of the university community (Nassour et al., 2020) as this was reflected in the intermediate values obtained for the criteria of value given to it and the disposition to implement restoration actions (Table 2). These opinions sometimes seem contradictory because of the different potential uses people consider appropriate for green spaces in and around the university entities. This social-ecological problem could be addressed by providing more information about the historical and ecological importance of the native xerophytic scrub ecosystem and its conservation. Furthermore, spaces for reflection and discussion, especially between group of people with opposed views, should be promoted by the university authorities to address the problematics posed by the growth of infrastructure in the campus and the desire of specific groups to preserve the last remnants of natural ecosystems.
Conclusions
The need and the feasibility of restoring unique xerophytic scrub vegetation in lava field remnants were evaluated in a portion of a university campus in Mexico City. We develop an index (Need and Feasibility of Restoration Index, NFRI) through multicriteria analysis and considering ecological, economic, and social indicators. It was found that urban ecological restoration represents the best approach to undertake damages to restore native ecosystem remnants fulfilling biodiversity but also social goals in dense urban settings such as Mexico City. The outcomes made evident the necessity of restoring native vegetation for all the remnants considered in this study. However, the group containing the two largest remnants obtained the highest values for restoration feasibility and NFRI. The recovery of the rest of the remnants is critical to support the ecological restoration of the area as this may provide connectivity with better-conserved ecosystem remnants. In addition, when the restoration is unaffordable because of financial resources constraints, it is highly recommended to direct efforts towards ecological rehabilitation actions. In this study, the establishment of native xerophytic gardens is promoted when remnants cannot support a self-sustainable plant community.
Finally, it is essential to note the limitations of this study. On the one hand, one of the main limitations reside in the obtention of social indicators. For instance, data considering nocturnal activities of the university community were not assessed. Some individuals from specific institutions were reluctant to answer the survey, therefore, information associated with these study sites was poorly represented. In addition, some of the remnants, especially those near the Olympic Stadium and closer to open areas, present severe difficulties for restoration. In those areas where the community has total access, the restoration process will slow down, and a possible rehabilitation process or any simple action will be stopped at all.
On the other hand, concerning the methods, the main limitation was the definition of the weighting of the multicriteria analysis. A small group of experts did this definition; however, by considering a larger group of experts or other stakeholders, the model could increase its robustness. Finally, although different kinds of variables were integrated into the analysis, some others including the legal status of the sites, the presence of trained security personnel, the degree of conservation and mobility of the fauna, the presence of geologically relevant elements, and the quality of the urban matrix were missing and yet crucial to contribute on determining the feasibility of restoring the lava field remnants and their conservation.
Data Availability Statement
The original contributions presented in the study are included in the article/Supplementary Material, further inquiries can be directed to the corresponding author/s.
Ethics Statement
The studies involving human participants were reviewed and approved by Comité Académico del Posgrado en Ciencias Biológicas, Universidad Nacional Autónoma de México. Written informed consent for participation was not required for this study in accordance with the national legislation and the institutional requirements.
Author Contributions
MB and ZC-S conceived and designed the research. MB carried out field work and data analysis. MB and DA-L wrote the manuscript. AC, LZ, and DA-L revised the concept and design of the research and offered constructive critics to improve it. ZC-S, AC, LZ, and DA-L revised and edited the manuscript. All authors contributed to the article and approved the submitted version.
Funding
The study was financially supported by the program PAPIIT IV200117. The Consejo Nacional de Ciencia y Tecnología, CONACYT, granted the scholarship 629340 to MB.
Conflict of Interest
The authors declare that the research was conducted in the absence of any commercial or financial relationships that could be construed as a potential conflict of interest.
Publisher's Note
All claims expressed in this article are solely those of the authors and do not necessarily represent those of their affiliated organizations, or those of the publisher, the editors and the reviewers. Any product that may be evaluated in this article, or claim that may be made by its manufacturer, is not guaranteed or endorsed by the publisher.
Acknowledgments
We thank the support of the Postgraduate Program of Biological Science, the program PAEP, and the Faculty of Science, UNAM. We also thank to CONACYT for the scholarship 629340 to MB, and programs PAEP and PAPIIT IV200117 that funded the research. We are grateful with Iván Castellanos Vargas for the comments on the manuscript and the expertise of Yuriana Martínez and Ramiro Cruz on the identification of botanical samples. To the university and REPSA authorities who granted access to the sites and core zones and who provided spatial information. Also thank the people who participated in the fieldwork.
Supplementary Material
The Supplementary Material for this article can be found online at: https://www.frontiersin.org/articles/10.3389/frsc.2021.709357/full#supplementary-material
References
Aguirre-Salado, C., Miranda-Aragón, L., Pompa-García, M., Reyes-Hernández, H., Soubervielle-Montalvo, C., Flores-Cano, J. A., et al. (2017). Improving identification of areas for ecological restoration for conservation by integrating USLE and MCDA in a GIS-environment: a pilot study in a priority region Northern Mexico. ISPRS Int. J. Geo Inf. 6:262. doi: 10.3390/ijgi6090262
Camarena, P. (2010). Xerojardinería. Guía para el diseño de los jardines de Ciudad Universitaria. Secretaría Ejecutiva REPSA, Coordinación de la Investigación Científica. Mexico City: UNAM.
Carlucci, M. B., Brancalion, P. H. S., Rodrigues, R. R., Loyola, R., and Cianciaruso, M. V. (2020). Functional traits and ecosystem services in ecological restoration. Restor. Ecol. 28, 1372–1383. doi: 10.1111/rec.13279
Ceccon, E. (2013). Restauración en bosques tropicales: fundamentos ecológicos, prácticos y sociales. Mexico City: CRIM UNAM-Díaz de Santos.
Christian-Smith, J., and Merenlender, A. M. (2010). The disconnect between restoration goals and practices: a case study of watershed restoration in the Russian River Basin, California. Restor. Ecol. 18, 95–102 doi: 10.1111/j.1526-100X.2008.00428.x
Clewell, A. F., and Aronson, J. (2013). Ecological Restoration. Principles, Values, and Structure of an Emerging Profession. Washington, DC: Island Press. doi: 10.5822/978-1-59726-323-8_2
Cox, D. T. C., Hudson, H. L., Shanahan, D. F., Fuller, R. A., and Gaston, K. (2017). The rarity of direct experiences of nature in an urban population. Landsc. Urban Plan. 160, 79–84. doi: 10.1016/j.landurbplan.2016.12.006
Estañol-Tecuatl, F., and Cano-Santana, Z. (2017). Recovery of basalt substrate for xeric scrub restoration in a lava field in Mexico City. Ecol. Restor. 35, 41–51. doi: 10.3368/er.35.1.41
Forman, R. T. T. (1995). Land Mosaics: The Ecology of Landscapes and Regions. Cambridge, MA: Cambridge University Press. doi: 10.1017/9781107050327
Fuller, R. A., Irvine, K., Devine-Wright, P., Warren, P., and Gaston, K. (2007). Psychological benefits of greenspace increase with biodiversity. Biol. Lett. 3, 390–394. doi: 10.1098/rsbl.2007.0149
Gann, G. D., McDonald, T., Walder, B., Aronson, J., Nelson, C. R., Jonson, J., et al. (2019). International principles and standards for the practice of ecological restoration. Second edition. Restor. Ecol. 27, S1–S46. doi: 10.1111/rec.13035
Guida-Johnson, B., and Zuleta, G. A. (2017). Riparian rehabilitation planning in an urban-rural gradient: integrating social needs and ecological conditions. Ambio 46, 578–587. doi: 10.1007/s13280-016-0857-7
Hychka, K., and Druschke, C. G. (2017). Adaptive management of urban ecosystem restoration: learning from restoration managers in Rhode Island, U.S.A. Soc. Nat. Res. 30, 1358–1373. doi: 10.1080/08941920.2017.1315653
Ives, C. D., Letini, P. E., Threlfall, C. G., Ikin, K., Shanahan, D. F., Garrad, G. E., et al. (2016). Cities are hotspots for threatened species. Global Ecol. Biogeogr. 25, 117–126. doi: 10.1111/geb.12404
Kang, W., Minor, E. S., Park, C. R., and Lee, D. (2015). Effects of habitat structure, human disturbance, and habitat connectivity on urban forest bird communities. Urban Ecosyst. 18, 857–870. doi: 10.1007/s11252-014-0433-5
Kruize, H., Vliet, N. V., Staatsen, B., Bell, R., Chiabai, A., Muiños, G., et al. (2019). urban green space: creating a triple win for environmental sustainability, health, and health equity through behavior change. Int. J. Environ. Res. Public Health Rev. 16, 1–22. doi: 10.3390/ijerph16224403
Lara-Deras, R. (2016). Difusión cultural del Pedregal de San Angel en Ciudad Universitaria a través de la xerojardinería con base en la vegetación nativa (Thesis dissertation). Universidad Nacional Autónoma de México, Mexico City, Mexico.
Laurila-Pant, M., Lehikoinen, A., Uusitalo, L., Venesjärvi, R. (2015). How to value biodiversity in environmental management? Ecological Indicators. 55, 1–11. doi: 10.1016/j.ecolind.2015.02.034
Lithgow, D., Martínez, M. L., and Gallego-Fernández, J. B. (2015). The “ReDune” index (Restoration of coastal Dunes Index) to assess the need and viability of coastal dune restoration. Ecol. Indic. 49, 178–187. doi: 10.1016/j.ecolind.2014.10.017
Nassour, J., Leykin, D., Elhadad, M., and Cohen, O. (2020). Computational text analysis of a scientific resilience management corpus: environmental insights and implications. J. Environ. Inform. 36, 24–32.
Natale, E., Villalba, G., Junquera, J. E., and Zalba, S. M. (2015). Assessment of the conservation status of natural and semi-natural patches associated with urban areas through habitat suitability indices. Int. J. Environ. Res. 9, 495–504. doi: 10.3808/jei.201900423
Olive, A. (2014). Urban awareness and attitudes toward conservation: a first look at Canada's cities. Appl. Geogr. 54, 160–168. doi: 10.1016/j.apgeog.2014.08.002
Orsi, F., and Geneletti, D. (2010). Identifying priority areas for forest landscape restoration in chiapas (Mexico): an operational approach combining ecological and socioeconomic criteria. Landsc. Urban Plan. 94, 20–30. doi: 10.1016/j.landurbplan.2009.07.014
Orsi, F., Geneletti, D., and Newton, A. C. (2011). Towards a common set of criteria and indicators to identify forest restoration priorities: an expert panel-based approach. Ecol. Indic. 11, 337–347. doi: 10.1016/j.ecolind.2010.06.001
Peralta-Higuera, A., and Prado-Molina, J. (2009). “Los límites y la cartografía,” in Biodiversidad del Pedregal de San Ángel, eds A. Lot and Z. Cano-Santana (Mexico City: UNAM), 27–42.
R Core Team (2018). R: A Language and Environment for Statistical Computing. Vienna: The R Foundation for Statistical Computing. Available online at: https://www.R-project.org/
Reaser, J. K., Witt, A., Tabor, G. M., Hudson, P. J., and Plowright, R. K. (2021). Ecological countermeasures for preventing zoonotic disease outbreaks: when ecological restoration is a human health imperative. Restor. Ecol. 29:e13357. doi: 10.1111/rec.13357
Saunders, D. A., Hobbs, R. J., and Margules, C. R. (1991). Biological consequences of ecosystem fragmentation: a review. Conserv. Biol. 5, 18–32. doi: 10.1111/j.1523-1739.1991.tb00384.x
Siebe, C. (2000). Age and archaeological implications of Xitle volcano, southwestern Basin of Mexico-City. J. Volcanol. Geothermal Res. 104, 45–64. doi: 10.1016/S0377-0273(00)00199-2
Standish, R. J., Hobbs, R. J., and Miller, J. R. (2013). Improving city life: options for ecological restoration in urban landscapes and how these might influence interactions between people and nature. Landsc. Ecol. 28, 1213–1221. doi: 10.1007/s10980-012-9752-1
Tobón., W., Urquiza-Haas, T., Koleff, P., Schröter, M., Ortega-Álvarez, R., Campo, J., et al. (2017). Restoration planning to guide Aichi targets in a megadiverse country. Conserv. Biol. 31, 1086–1097. doi: 10.1111/cobi.12918
Vilà, M., and Ibáñez, I. (2011). Plant invasions in the landscape. Landsc. Ecol. 26, 461–472. doi: 10.1007/s10980-011-9585-3
WHO (2020). World Health Organization. Available online at: https://www.who.int (accessed May 12, 2020)
Zambrano, L., et al. (2016). Ecológica del Pedregal de San Ángel: Atlas de riesgos. Secretaría Ejecutiva de la Reserva Ecológica del Pedregal de San Ángel. Mexico City: UNAM.
Keywords: urban ecological restoration, multicriteria analysis, priority of restoration, restoration feasibility, xerophytic scrub, lava field, Mexico City
Citation: Bonilla-Rodríguez M, Arroyo-Lambaer D, Castillo A, Zambrano L and Cano-Santana Z (2021) Urban Ecological Restoration: Setting Priorities for Restoring Native Vegetation in Lava Field Remnants in Mexico City. Front. Sustain. Cities 3:709357. doi: 10.3389/frsc.2021.709357
Received: 13 May 2021; Accepted: 29 July 2021;
Published: 24 August 2021.
Edited by:
Zoe Li, McMaster University, CanadaCopyright © 2021 Bonilla-Rodríguez, Arroyo-Lambaer, Castillo, Zambrano and Cano-Santana. This is an open-access article distributed under the terms of the Creative Commons Attribution License (CC BY). The use, distribution or reproduction in other forums is permitted, provided the original author(s) and the copyright owner(s) are credited and that the original publication in this journal is cited, in accordance with accepted academic practice. No use, distribution or reproduction is permitted which does not comply with these terms.
*Correspondence: Denise Arroyo-Lambaer, ZGVuaXNlLmFycm95b0BzdC5pYi51bmFtLm14