- Department of Civil Engineering, Division of Global Architecture, Graduate School of Engineering, Osaka University, Osaka, Japan
A variety of transport options must be utilized to enhance public transportation sustainability. Previous studies have rarely developed quantitative methods for evaluating the performance of transport diversity. Thus, this study proposes measurement indicators to evaluate transport diversity on the basis of biodiversity indicators. For the hierarchy of public transport networks, we considered that the public transport system is analogous to ecosystems with a taxonomic hierarchy. The number of operation stations, route length, and operating frequency were used to evaluate the spatial and temporal coverage of the transport services. We analyzed the latent correlation between transport diversity and sustainable transport indicators and then examined the spatial distribution of transport diversity. By visualizing the spatial distribution of transport diversity, we found that high-speed railway stations are instrumental in the hierarchy of public transport networks. Furthermore, improving diversity would increase the modal share of public transport, which is considered crucial for developing sustainable transport, especially in the period of depopulation. Finally, this paper presents a demonstration experiment conducted in a regional city that introduced a variety of transport services integrated on a MaaS platform, attempting to provide a multimodal and seamless mobility service in a weak transport demand area.
Introduction
Owing to the accelerated development of aging and shrinking population in Japan, the age composition of the people in the country has drastically changed. The problem of protracted and significant population loss makes the provision of public transport increasingly difficult, especially in rural areas. A heady discussion relates the concept of sustainable development to transportation (Ministry of Land, Infrastructure, Transport and Tourism, 2020a). According to the (Ministry of Land, Infrastructure, Transport and Tourism, 2020b,c), the efficient resource allocation of various transport modes can reduce operating costs, accommodate diverse needs and preferences of users, and encourage the maintenance of a wider public transport network. Increasing diversity in transport systems by providing a variety of transport options supports the development of sustainable transport. Despite the need for evaluation measures, the literature has rarely developed measurement indicators that can assess the performance of transport diversity.
Thus, this study attempts to establish quantitative indicators that can be deployed to evaluate the diversity of urban transport systems in consideration of transport sustainability. To examine transport diversity, it is important to identify the role and characteristics of each transport mode in the network, rather than counting the number of modes present. For instance, intercity rail services cover longer distances than intracity transit, and personalized transport has higher mobility than mass transit, whereas mass transit has a better capacity. In this research, we characterized the hierarchical structure of public transport as analogous to the ecological hierarchy, aiming to concretize and quantify the concept of a hierarchy of transport diversity.
Literature Review
Measuring Diversity and Sustainability in a Public Transport Network
Though the common identified definition of sustainability is not available, there is growing interest in the concepts of sustainable development, indicators and measures for sustainable transport planning (Litman, 2016). Transportation Research Board (1997) interpreted sustainable transport as “it is about how environmental, economic, and social systems interact to their mutual advantage or disadvantage at various space-based scales of operation.” To access sustainability of urban transport systems, Doi and Kii (2012) conducted an impact analysis of public transport and land use strategies for all of 269 urban areas in Japan. The influence of compact city planning on the sustainability of public transport was evaluated by indicators, including financial balance of public transport operation, user benefits, and CO2 emissions reduction. Despite the results obtained from quantitative analysis, the essence of public transport was ignored as the simplifying objects were applied. Public transport consists of a variety of modes and services with different characteristics and functionalities. The public transport service is interdependent with other infrastructure and provides a critical connection for residential and business activities in an urban context. For sustainable transport assessment, it is necessary to further characterize the transport system by considering all modes.
Providing public transport service with diverse options extends citizens' freedom of choice. With the aim to broaden citizens' freedom of choice in mobility, United Nations (2007) emphasized the development of a wide range of policies and opportunities for sustainable transport. More choices lead to better choices, being considered an important device to increase well-being (Weikard, 1996). Sustainable transport is expected to not only cater diverse mobility needs of a society but also improve the welfare of people and their communities. Mobility users ought to be able to select their optimal mobility from among several alternatives. A diverse transport system promotes freedom of choice, enabling better mobility choices of users by providing better access to other services and opportunities. The transport system consists of various modes referred to as multimodal transport. A multimodal system enables efficient usage of public transport services, adds value across multiple perspectives, and enriches collaboration in the system.
There is a limited number of studies specifically focusing on the transport diversity as a measure to evaluate urban transport systems (Pareekh et al., 2017). Many studies have been conducted on transport network performance measurements (Nakagawa et al., 1994; Aratani and Todoroki, 2010; Nezu et al., 2013). Most of these studies aimed to derive the effects of infrastructure investment, focusing on its impact on regions. For example, Nakagawa et al. (1994) examined railway network performance from the perspective of accessibility. With the indicator “staying time” used, the level of social interaction between cities was compared to their railway networks. In addition, the benefits of a high-speed rail (HSR) system on the regional economy and urban mobility were highlighted (Aratani and Todoroki, 2010; Nezu et al., 2013). Network performance measurements have mainly been conducted on railway services in previous studies. Nonetheless, the evaluation should be conducted at the network level, as transport is provided by an integrated system. Despite the evaluations of the social impact of infrastructure, the examination of the public transport system itself has rarely been discussed.
Litman (2017) defines Transport Diversity as “the variety of mobility and accessibility options available in a particular situation, including various modes, services, and destinations.” A variety of transport options help cities with diverse populations to cater to their diverse mobility needs, supporting the realization of sustainable urban transport. Increasing diversity in public transport has not only contributed to the availability of transport services but also increased system efficiency, equity, and resilience (Litman, 2017; Rahimi-Golkhandan et al., 2019). Several studies have attempted to approach the concept of diversity in public transport in recent years. Feng and Hsieh (2009) developed a conceptual framework to explore the relationships among transport diversity, sustainability, and quality of life. Evaluating diversity from stakeholders' perspectives, it was suggested that diversity could assist planners in resource allocation to improve sustainability and quality of life by investing the infrastructure or service in areas with the least diversity. For a better understanding of transport diversity, Pareekh et al. (2017) employed an exploratory factor analysis to reveal the latent structure underlying this concept. With a sample of 51 cities with various demographic characteristics, factors such as public transport modal share, city population, and smart infrastructure were proven to positively influence transport diversity. However, there remains a need for multiple quantitative and qualitative approaches to measure transport diversity (Pareekh et al., 2017).
A few studies have linked ecological diversity to transport diversity (Feng and Hsieh, 2009; Amoaning-Yankson and Amekudzi-Kennedy, 2017; Hatoko, 2019; Rahimi-Golkhandan et al., 2019). For resilience assessment of transport infrastructure, Amoaning-Yankson and Amekudzi-Kennedy (2017) confirmed the similarity among ecological, social and economic systems, applying the ecological concepts to transport system resilience. Hatoko (2019) employed a biodiversity index to evaluate diversity in regional functionalities. The relationship between various regional characteristics (e.g., industrial composition and employment opportunities) and the level of community interaction represented in the transport network (e.g., passenger volumes of intercity transits) were examined. However, this research focused on the diversity of regional functionalities without assessing transport diversity. Feng and Hsieh (2009) adapted the ecological diversity concepts of richness and evenness to characterize transport diversity in their conceptual framework. To improve the resilience of transport infrastructure under increasing demand resulting from rapid urbanization, Rahimi-Golkhandan et al. (2019) further explored these two measures of functional richness and functional evenness and translated them to transport infrastructure as a predictor of system resilience. Nonetheless, there is a lack of studies to characterize and quantify transport diversity with concrete and well-defined metrics. With the aim to bridge the gap, this research seeks to conduct a complete study of both quantitative measures with the numerical usage data and qualitative insights on the nature of various public transport modes.
Taxonomic Diversity Indices
Research on diversity has long been of interest in the field of biology. With numerous measurements of biodiversity having been developed, we adopted the interpretation of Ohgaki (2008) as a reference. The traditional diversity indices, such as Shannon entropy and Simpson's index, are computed using the number of species and their proportions. Nevertheless, these indices cannot account for taxonomic relatedness between species. Consider the following examples: (1) There are savanna, forest, Indian, and Ceylon elephants. (2) There are elephants, zebras, tigers, and lions. For both cases, the degrees of diversity were both evaluated as four species. Given the hierarchical structure of ecosystems, different weights should be assigned corresponding to their similarity.
Warwick and Clarke (1995) proposed new biodiversity indices. As shown in Figure 1, the new concept captures the structure of not only the distribution of abundances among species but also their taxonomic relatedness. The taxonomic distance between species i and j is defined as:
0, if they are the same species.
1, if they are different species within the same genus.
2, if they are of different genera within the same family.
3, if they are of different families within the same order.
The biodiversity index (Δ*) defined by Clarke and Warwick (1998) is expressed as follows:
where S is the total number of species present; ni and nj are the total number of individuals in the samples of species i and j, respectively; and wij indicates the “taxonomic distances” given to the path length linking species i and j in the hierarchical classification. For the double summation, i and j range over the S species; it can be reduced to the same statistic as S(S − 1)/2. In Clarke and Warwick's index, the expected path length between two species reflects their similarity, giving a weight (wij) in the formulation.
Materials and Methods
Applying Taxonomic Hierarchy to Public Transport Classification
To present the abundance of all transport modes in the community, diversity was assessed based on their functionalities. Diverse transport modes have different functionalities. For example, railways demonstrate the advantages of high speed and punctuality, despite that most users find it easier to access bus stops than rail stations. A public transport network comprising a variety of modes contributes to a high overall functional performance level of the services. In this study, we applied a taxonomic hierarchy to classify public transport modes based on their characteristics and functionalities.
As mentioned previously, we first considered the classification of all public transport modes. In comparison with the taxonomic hierarchy, which today has eight ranks from specific to general, (species, genus, family, order, etc.), the hierarchy of transport modes has not been clearly defined. Figure 2A illustrates the conceptual model for classifying the public transport. Transport modes can be grouped into three broad categories: land, water, and air transport. Rail transit composed of a traced path was distinguished from road transport. For intercity transport, modes were further divided depending on their operating speed. In intracity transport systems, the right-of-way and node size in the network were further considered. The right-of-way establishes the right in terms of priority to a traffic signal or road space. For instance, the bus rapid transit (BRT) systems with dedicated median lanes or exclusive bus lanes have a higher right-of-way than conventional urban bus service. The definition of a four-level hierarchy and taxonomic distances of public transport classification is shown in Figure 2B.
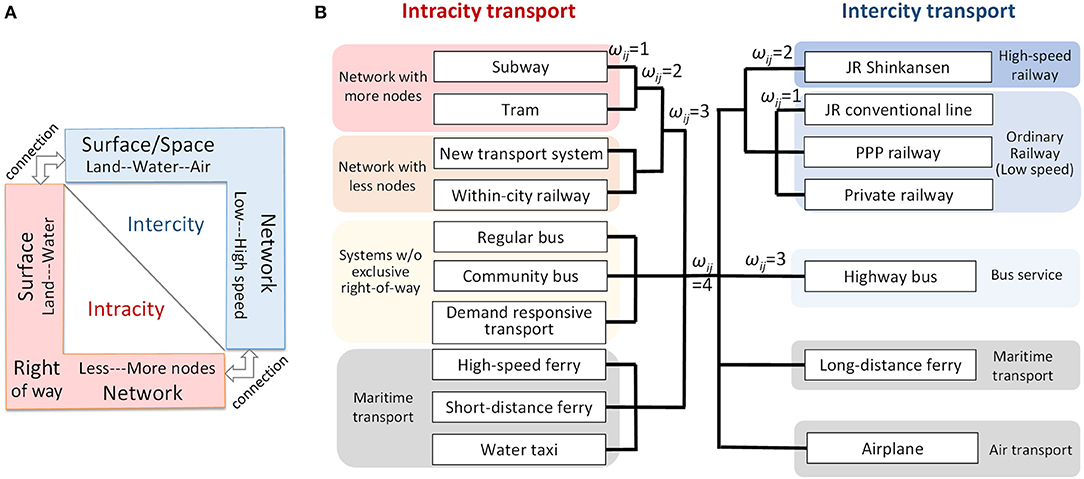
Figure 2. (A) Conceptual model to classify public transport. (B) Definition of taxonomic distance in a hierarchical structure of public transport.
Initially, from a transport planning perspective, intercity transport and intracity transport differ significantly in their functional characteristics. The first level and the most general rank were thus defined as intercity and intracity networks. Next, in the second level corresponding to family in taxonomic hierarchy, transport modes were divided depending on whether they were rail transit for both intercity and intracity networks. In intracity transport systems, the BRT system, which is referred to as the new transport system (NTS) in Japan, was also divided into the category of rail transit due to its exclusive right-of-way. Finally, the third level defined herein was also the most specific rank applied to our classification. For intracity transport, transport modes were further classified depending on whether an efficient network could be formed within a city. In other words, the node size of each transport mode, which indicates the number of stations, was examined. Additionally, for intercity rail systems, we distinguished the high-speed railway (Shinkansen) from the others. The bus services were categorized into the same group in both intercity and intracity networks. Highway infrastructure was not discussed and included in the figures since this research focused on mobility measures.
Applying Biodiversity Index to Measure Transport Diversity
As discussed in the previous section, this section clarifies how biodiversity indices can be applied to evaluate transport diversity. This study adopted a measure developed by Warwick and Clarke (Δ*), which was introduced in section Taxonomic diversity indices. In Warwick and Clarke's formulation, the measure of diversity considers the number of individuals per species to represent species evenness. The number of entities and operators involved in public transport might be used to calculate the number of individuals of transport services. However, natural monopolies are common phenomena in the markets, except for some metropolitan areas, as transport is a capital-intensive industry. There remain questions regarding whether a transport service is expected to be offered by multiple operators.
This study interpreted the evenness in transport diversity as the spatial and temporal extent of public transport services. The spatial extent consists of the number of operation stations and route length of transport services. An increase in the number of stations in an area makes the service more available and easier to access. As continuous data, route length has advantages in terms of precision and accuracy. In addition, for some long-distance bus routes operated in barely inhabited areas such as mountains, using both variables can avoid overestimation compared with using route length only.
The temporal extent refers to the operating frequency of a service, which is reflected in the timetable. For instance, the Midosuji Line (Osaka Metro) in Osaka has a minimum headway between 3 and 4 min. In contrast, the Geibi Line (JR conventional line) in Hiroshima provides only three trains a day inbound to Bingo-Ochiai. As shown above, there is a sharp contrast in the operating frequency of transport services provided in different regions. The operating frequency of each service corresponds to the number of individuals per mode from the viewpoint of temporal coverage.
The number of operation stations, route length, and operating frequency (the spatio-temporal data) are employed to measure transport diversity in this paper. Nevertheless, some characteristics of the different transport systems differ markedly. For example, stations in subway networks may be much more than high-speed railway stations. To prevent the case mentioned and place different variables on the same scale, we first standardized the data. The standardized spatial and temporal coverage of the transport service (ε) is defined as:
To start the formulation illustration, we set up aix as the spatio-temporal data of transport mode i in city x. The number of stations, route length, and number of trains (or buses) departing per hour in one direction were inputted separately. For each transport mode, aimax, aimin, and aiave are the maximum, minimum, and average values, respectively. The service performances in the three aspects are calculated as εstation, εroute, and εdepart. The overall spatial and temporal coverage of the transport mode in each city is evaluated as:
Thus, the spatial and temporal weights are balanced. The difference in the spatial and temporal extents of each public transport service is well-reflected in the index (εix). We set up the diversity index of public transport modes (Diptm) as:
where S is the total modes of public transport present in city x and wij indicates the taxonomic distances between modes x and j in the hierarchical classification. Compared with Warwick and Clarke's formulation (Δ*), the transport diversity index is not standardized by the number of species. This can be explained by the fact that transport diversity is based on a complete survey, rather than the sampling methods applied to investigate biodiversity. In addition, spatial and temporal coverage was defined as a standardized indicator. In terms of the scale of each variable in Diptem, w is on a scale of 1–4, while ε ranges from 0 to 2. Accordingly, both the features of “hierarchy structure” and “species abundance” are not only well-reflected but also weighted equally.
Analytical Framework
Sections Introduction and Literature Review provided the broad background and the problem statement of this study. The illustration of the approach to public transport diversity was given in the last two sections. This section elaborates on the analytical framework developed to address the research questions and the data analysis plan (Figure 3).
To empirically quantify the concept of hierarchical transport diversity, a measure of Diptm was applied to a study area consisting of cities with diverse population size and a wide variety of public transport options. For a better understanding of the spatial distribution of diversity, we firstly visualized the calculated diversity value of each city. The potential influence of the HSR network in a hierarchy of public transport was explored. Next, a correlation analysis was conducted to examine the association among transport diversity, human population, and public transport usage in the cities. To capture the features of metropolises and weak demand areas, the cluster analysis was performed. Cluster analysis is an effective and widely used method for analyzing multivariate data. It is an exploratory analysis used to describe a family of statistical procedures specifically designed to discover classifications within complex data sets (Tinsley and Brown, 2000).
Aside from a city-based analysis, this study evaluated transport diversity from the viewpoint of metropolitan areas, considering the influence of a central city on the suburban area. The degree of dependence of a UEA's transport diversity on its central city was examined.
Study Area
The study area is located in western Japan, including the Kansai (excluding Mie Prefecture), Shikoku, and the Chugoku regions. The diverse population of cities in selected areas forms city clusters with diverse transport needs. For instance, there are central metropolises in the Kansai region—Osaka, the conurbation—Kyoto, Osaka, and Kobe, regional central cities with a population of over 500 thousand, and the regional core cities with a population of over 200 thousand. The sharp contract between the growth in city centers (especially in Tokyo) and shrinking suburbs has become more noticeable in the recent decade. The population decline is supposed to result in a weak transport demand in the selected area, making it increasingly difficult to provide public transport services in the future.
The air and maritime transport are excluded from the subject of this study since both of them are used relatively little for passenger transport and accounted few for daily number of passenger journeys in the study area. Despite that a discussion of maritime and air transport has not been included in the main body, they were also contained in the hierarchical structure owing to the potential for future research.
Due to a relaxation of land use restriction, the urban areas are formed beyond jurisdictional boundaries in many Asian cities such as Japan. This study evaluated the hierarchical diversity and sustainability of public transport from the perspectives of both cities and urban employment areas (UEA). The concept of UEA was proposed by Kanemoto and Tokuoka (2001), in which an area consists of a central city and its associated outlying municipalities (suburbs) with a certain number of commuters. A central city was defined as a municipality with at least 10,000 densely inhabited district population. Municipalities with more than 10% of the population commuting to the central city were defined as suburbs. In this study, 399 cities and 48 UEA were examined. The difference between central cities and suburbs related to public transport systems were taken into consideration.
Data Collection Method
The data used for the study included the number of stations, route length, and timetable of each public transport service. The route length and station/stop information of rail and bus services were obtained from the Japanese National Land Numerical Information (Ministry of Land, Infrastructure, Transport and Tourism, 2017, 2019a,b) and administrative boundaries dataset (Official Statistics of Japan, 2019). The timetables of each station were collected from the Yahoo Japan Transit information. As the data were not available online for part of community bus services and demand responsive transport, the average value of 1 was assigned to their spatial and temporal coverage.
Results and Analysis
Role of High-Speed Railway in Hierarchy of Public Transport
Figure 4A shows the spatial distribution of the diversity of public transport in cities, and Figure 4B depends on the UEA. The visualization of spatial data illustrated that cities situated on the HSR corridor tended to have a higher diversity in their transport networks. To verify the influence of the HSR network on the hierarchical diversity of urban transport, we reevaluated the diversity without considering Shinkansen. The findings showed that despite the exclusion of the Shinkansen data from the calculation, the cities where Shinkansen stations still had a higher level of diversity (Figure 5). Accordingly, the Shinkansen can not only strengthen the hierarchy of public transport networks but also improve the performance of other transport services in the city.
Analysis on Transport Diversity, Population, and Public Transport Usage
With the aging society and population decline in Japan, it has become difficult to maintain public transport in areas with weak transport demand. Improving sustainability in a declining population is essential to ensure that older people can still enjoy a high quality of life. To investigate whether public transport diversity differed between cities and UEA with different population size, a correlation analysis was conducted. Population size data were based on the 2015 National Population Census (Statistics Bureau of Japan, 2016). A Pearson correlation showed that diversity had a significant positive relationship with the city population, with a correlation coefficient (r) of 0.935. Similarly, a strong positive relationship was observed between the UEA population and diversity (r = 0.959). The results suggest that the diversity of public transport is linked to human population size. That is, a population decline may result in a reduction in diversity.
Modal share is regarded as a critical component in the development of sustainable transport within a city. Regardless of a declining population hitting especially hard in rural areas, the growth of public transport use could be promoted by providing effective and efficient services. To explore whether the modal share in a city was affected by diversity in public transport, a correlation analysis was conducted. A sample of 23 cities was collected from the 2015 Nationwide Person Trip Survey (Ministry of Land, Infrastructure, Transport and Tourism, 2016). A Pearson correlation analysis indicated that there was no significant relationship between modal share and diversity (r = 0.649).
We examined the relationship between diversity with city population and the number of public transport users (modal share times city population). In Figure 6, Osaka was set to 1, and the relative proportions for other cities were estimated. For cities with larger populations and higher diversity, compared with Osaka and Kobe, there is a tendency for less public transport use to result from a lower level of diversity in Kyoto, Hiroshima, and Sakai (Figure 6A). In addition, cities with small populations and limited diversity tend to underperform in terms of public transport usage (Figure 6B).
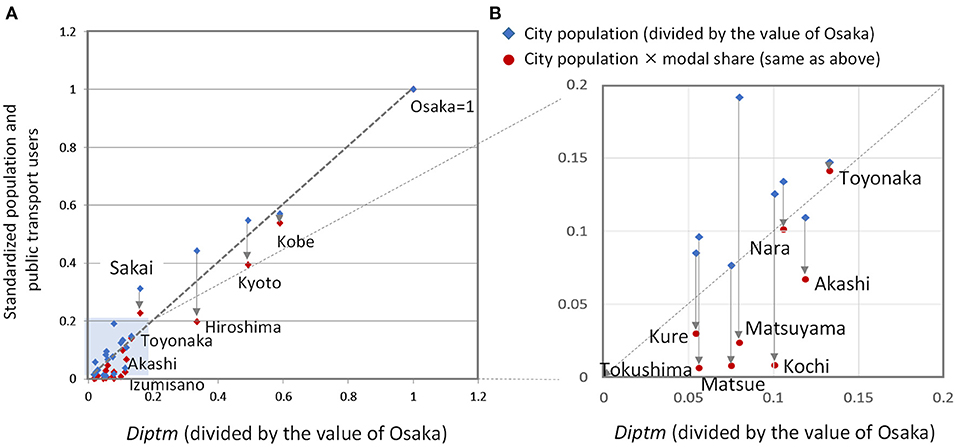
Figure 6. (A) Relationship between Diptm, city population, and public transport usage. (B) Cities shown near the origin (the blue area in Figure 6A).
Evaluating Transport Diversity From the Viewpoint of Metropolitan Areas
Next, the analysis was conducted from the viewpoint of the UEA. For the central cities located in the Japan national axis zone, a significant positive relationship (r = 0.954) was observed between the modal share and average diversity per person (diversity value divided by population size) (Figure 7).
In addition, the suburban cities in the UEA, formed by a central city with high diversity, tended to have better public transport usage. To make it easier to understand, cities are listed in descending order of the modal share in Table 1. Cities located in metropolitan areas, such as Toyonaka, Nara, and Uji, had a better modal share of public transport, despite that the estimated diversity was just average. Based on these results, we considered the effect of transport diversity in central cities on their associated outlying municipalities. The diversity data of a suburban city were replaced with the average of the city's diversity and its central city's diversity. A significant positive relationship (r = 0.922) was observed (Figure 8). The results revealed that public transport usage in a suburban city was affected by the transport diversity of the major city in the area.
Cluster Analysis to Capture Features of Metropolises and Weak Demand Areas
Cluster analysis was performed to identify present issues and discuss implications from the analytical results by allowing us to better understand the unique combination of characteristics in city clusters. According to the results obtained in the last section, a major city impacts their suburban cities on public transport usage. Cluster analysis was thus conducted twice depending on whether the role of a central city in UEA was considered.
Initially, the analysis was applied to identify homogeneous city clusters based on the population and diversity estimated in each city. The 399 cities in western Japan were divided into five clusters (Figure 9). Clusters 4 and 5 were clusters with a relatively high level in both population and diversity, whereas Clusters 1 and 2 had a lower level in population size and diversity. Next, the role of a central city in UEA was taken into account. In cluster analysis, we considered variables including the city's population and diversity, as well as the population and diversity of UEA to which the city belongs. A sample of 48 central cities was classified into six clusters. Ecl_1 (4 cities), Ecl_2 (3 cities), and Ecl_3 (7 cities) were considered as clusters with a level of diversity corresponding to their population size, whereas Ecl_4 (14 cities), Ecl_5 (13 cities), and Ecl_6 (7 cities) were clusters with limited diversity relative to their population size. These results are discussed later in the next section.
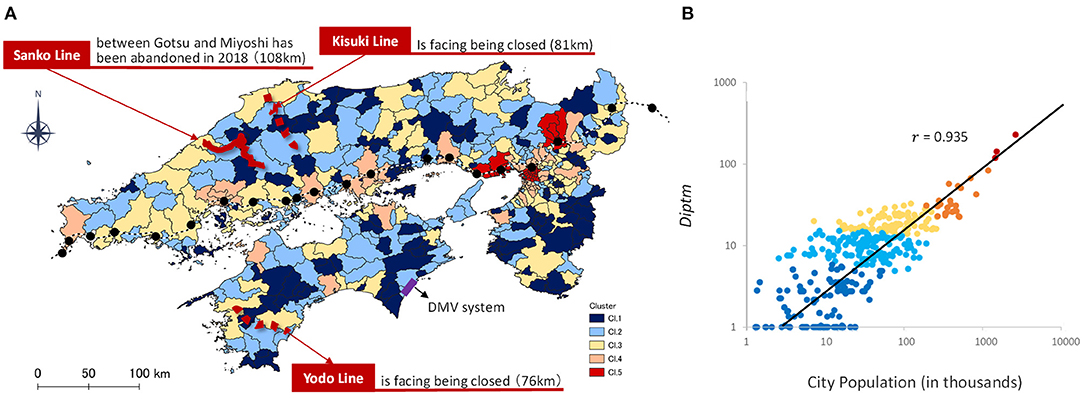
Figure 9. (A) Spatial distribution of city clusters identifying areas with challenges maintaining railway services in terms of transport diversity. (B) Scatter graph to visualize city clusters.
Analysis on Diversity Dependence of Metropolitan Area on Central City
To measure the degree of dependence of a UEA's transport diversity on its central city, this study defined the diversity dependence on central city as:
UEA's diversity dependence on central city
The numerator is a ratio calculated by dividing the diversity in the central city by the diversity in the UEA. To prevent a bias toward cities with higher transport diversity, the ratio is divided by the dependence of the UEA population on their central city.
Figure 10 illustrates the relationship between the diversity in the central city and the diversity dependence of the UEA on the central city. A distinct difference was observed between the clusters derived in the previous section. First, for Ecl_1, Ecl_2, and Ecl_3, which have the level of diversity corresponding to their population, a decrease in diversity results from the population decline (as shown by the light blue circle in Figure 10). Second, the light-blue vector can be divided into two components. The downward arrow indicates that when a central city maintains its diversity, an increase in diversity dependence on the central city will result in a decrease in diversity in suburban cities. Finally, the leftward arrow indicates a decreasing diversity in central cities and points to clusters Ecl_4, Ecl_5, and Ecl_6 (as shown in the orange circle). As these clusters have a limited diversity relative to their population size, it can be interpreted that the decline in diversity of public transport in these cities is more serious than the decline in population.
Discussions and Conclusions
This study attempts to empirically quantify the concept of transport diversity in current cities. The findings of this study substantiate previous studies suggesting that population and public transport modal share are positively related to transport diversity. This study's contribution lies more with a concrete and well-defined metric to concretize the concept of a hierarchy of transport diversity. We interpreted the evenness in transport diversity as the spatial and temporal extent of public transport services corresponding to the number of individuals in biodiversity. With a clear definition of the transport hierarchical structure, a biodiversity index was adapted to evaluate the diversity in public transport systems. The measures developed in this study can be deployed to other regions and countries. Compared to a city-based analysis performed in the previous study (Pareekh et al., 2017), this research further considered the influence of a central city on the metropolitan area related to public transport usage and diversity. The findings of this paper are identified and discussed to provide implications for sustainable transport planning.
First, the results suggest that the diversity of public transport is linked to the city population. Owing to the shrinking population in Japan, it has become difficult to fund and maintain public transport links, especially in areas with weak transport demand. To sustain public transport services, it is essential to provide innovative and effective policies that consider the diverse characteristics of cities. Through cluster analysis of cities, the features of areas with weak transport demand were captured. Clusters 4 and 5 have a relatively high level in both population and diversity and are considered able to sustain public transport services in the future. Conversely, the cities in Clusters 1 and 2 may have challenges in maintaining effective transport services. To illustrate this point, a number of recent cases are presented in Figure 9A. Some railway lines extended to cities in Cluster 1 or Cluster 2 have been abandoned or are facing closure. Therefore, it is especially important to provide innovative transport solutions in areas with weak transport demand. For example, a dual-mode vehicle (DMV) system, which can operate on both conventional road surfaces and a railway track, has been introduced in some rural areas in Tokushima Prefecture. The DMV system is expected to encourage tourism and ensure the travel demands of senior citizens. Cluster 3 is considered a critical cluster because sustainable public transport can be realized due to appropriate strategies implemented in these cities.
Second, cities located in the high-speed railway corridor tended to have higher diversity. The high-speed railway could not only strengthen the hierarchy of the public transport network but also improve the overall performance of urban transport services. However, among the cities situated on the Shinkansen corridor, Aioi (Cluster 1), Onomichi (Cluster 2), and Higashi-Hiroshima (Cluster 3) have a relatively lower level of diversity. In addition, Yamaguchi (Cluster 3) is considered underperforming possibly because it is a prefectural capital as well as a central city of UEA with Shinkansen station stopped by Nozomi (the super-express bullet train that only served at the largest stations). The common feature in these cities mentioned is that the Shinkansen stations are located far from the city's main station. Moreover, the lack of connections between the urban transport network and high-speed railway makes it difficult for the HSR service to function effectively. Consequently, to achieve connectivity between transport infrastructure, a multimodal transport planning is considered essential. This finding is supported strongly by Litman (2017). For the purpose mentioned above, a regional city—Yamaguchi has conducted a demonstration experiment to promote its public transport usage by enhancing the transport diversity in the city (see Appendix A for case study).
Third, this study revealed that public transport usage in a suburban city was significantly influenced by a major city nearby. The suburban cities in the UEA, formed by a central city with high diversity, tended to have better public transport usage. As a result, evaluating transport diversity from the viewpoint of metropolitan areas is critical. A transport network with high diversity conducted in central cities can enhance public transport usage in suburbs. These findings could be confirmed by the current situation of a growing need to travel between cities in the UEA, such as commuting and shopping. As mentioned earlier, there was no significant relationship between public transport usage and city diversity. However, a strong relationship emerged when considering diversity in their central cities. Improving the overall transport diversity in a metropolitan area is considered effective to increase public transport usage. Compared to the qualitative methods suggested in previous studies, this study provides quantitative evidence that a multimodal transport system supports attractive public transport.
Fourth and lastly, the reasons behind the decline in public transport are identified and three types of public transport decline are derived from the research findings demonstrated in Figure 10. First of all, a decrease in diversity results from the population decline. The loss of population density in the mid-sized cities, the regional core cities in Japan, leads to challenges in maintaining public transport diversity. Moreover, a decrease in diversity in suburban cities results from an increase in diversity dependence on the central city. This may occur in the new towns in the suburb of metropolitan areas. Last, for regional cities with small populations, the decline in public transport diversity in these cities is more serious than the decline in population. To develop appropriate strategies and ensure sustainable public transport services, it is necessary to consider the types of decline in public transport in cities.
For circumstances of a shrinking population and super-aging, the compact city has been regarded as a desired urban form in Japan. Compact city planning focuses on relatively high residential density and promotes public transport usage through the intensification of public transport and optimal access to cities (Bibri et al., 2020). Despite a reduction of infrastructure cost in a concentrated transport network, the compact city planning in Japan has not focused on the variety of transport modes, especially in the weak demand areas. Transport diversity is considered an important factor for infrastructure resilience (Rahimi-Golkhandan et al., 2019), which provides the ability of a transport network to withstand, operate, and to recover nature hazards. In terms of sustainable transport, it is suggested that transport diversity should be maintained in metropolises while more transport options are needed in weak demand areas.
With the aim to achieve sustainable public transport in both metropolises and weak transport demand areas, this research focused on measuring mobility choices. Future research could explore the role of highway infrastructure in transport diversity. This research has identified the reasons behind the decline in public transport, future works could explore appropriate strategies for each type of transport decline. Owing to the recent impact of COVID-19, users' needs for transport services are expected to become more diverse. There is a potential to improve the diversity index by considering comfort and safety, such as infection control, for future research.
Data Availability Statement
The raw data supporting the conclusions of this article will be made available by the authors, without undue reservation.
Author Contributions
RN conceived of the presented idea, was in charge of the data curation and analysis, and drafted the manuscript. C-CC helped in strengthening the theoretical thinking of the study, compiled the literature review and case study, and wrote the final manuscript. YA conceived the idea and participated in the design of the study. KY and KD did proofreading and corrections and were in charge of overall supervision. All authors discussed the results and contributed to the final approval of the version to be submitted.
Conflict of Interest
The authors declare that the research was conducted in the absence of any commercial or financial relationships that could be construed as a potential conflict of interest.
Acknowledgments
The authors are grateful to the office of Yamaguchi Prefecture who supported this research by providing their feedback and helpful advice.
References
Amoaning-Yankson, S., and Amekudzi-Kennedy, A. (2017). Transportation system resilience: opportunities to expand from principally technical to sociotechnical approaches. Trans. Res. Rec. 2604, 28–36. doi: 10.3141/2604-04
Aratani, T., and Todoroki, T. (2010). Time series analysis of intercity mobility by public transportation service in Japan. Infrastruct. Plann. Rev. 27, 643–652. doi: 10.2208/journalip.27.643
Bibri, S. E., Krogstie, J., and Kärrholm, M. (2020). Compact city planning and development: emerging practices and strategies for achieving the goals of sustainability. Dev. Built Environ. 4:100021. doi: 10.1016/j.dibe.2020.100021
Clarke, K. R., and Warwick, R. M. (1998). A taxonomic distinctness index and its statistical properties. J. Appl. Ecol. 35, 523–531. doi: 10.1046/j.1365-2664.1998.3540523.x
Doi, K., and Kii, M. (2012). Looking at sustainable urban mobility through a cross-assessment model within the framework of land-use and transport integration. IATSS Res. 35, 62–70. doi: 10.1016/j.iatssr.2012.02.004
Feng, C. M., and Hsieh, C. H. (2009). Implications of transport diversity for quality of life. J. Urban Plann. Dev. 135, 13–18. doi: 10.1061/(ASCE)0733-9488(2009)135:1(13)
Hatoko, M. (2019). A basic study on measuring diversity index of inter-regional access and its relationship with regional structure. JSCIP 75, I201–I212. doi: 10.2208/jscejipm.75.I_201
Kanemoto, Y., and Tokuoka, K. (2001). Metropolitan Area Definitions in Japan. CSIS Discussion Paper 37, 1–81.
Litman, T. (2017). Evaluating Transportation Diversity: Multimodal Planning for Efficient and Equitable Communities. Victoria, BC: Victoria Transport Policy Institute.
Ministry of Land, Infrastructure, Transport and Tourism. (2016). Nationwide Person Trip Survey. Available online at: http://www.mlit.go.jp/toshi/tosiko/toshi_tosiko_tk_000033.html (accessed January 20, 2020).
Ministry of Land, Infrastructure, Transport and Tourism. (2017). National Land Numerical Information: Bus Route Data. National land numerical information download. Available online at: http://nlftp.mlit.go.jp/ksj/gml/datalist/KsjTmplt-N07.html
Ministry of Land, Infrastructure, Transport and Tourism. (2019a). List of Community Buses. Available online at: https://www.mlit.go.jp/common/001193366.pdf (accessed December 16, 2019).
Ministry of Land, Infrastructure, Transport and Tourism. (2019b). National Land Numerical Information: Railroad Data. National land numerical information download. Available online at: http://nlftp.mlit.go.jp/ksj/gml/datalist/KsjTmplt-N02-v2_3.html
Ministry of Land, Infrastructure, Transport and Tourism. (2020a). To Realize Regional Sustainable Public Transport. Available online at: https://wwwtb.mlit.go.jp/hokkaido/bunyabetsu/tiikikoukyoukoutsuu/78jinzaiikusei/koutuukikakuka.pdf (accessed February 4, 2021).
Ministry of Land, Infrastructure, Transport and Tourism. (2020b). Act on the Revitalization and Rehabilitation of Local Public Transportation Systems. Available online at: https://www.mlit.go.jp/sogoseisaku/transport/sosei_transport_tk_000055.html (accessed January 20, 2020).
Ministry of Land, Infrastructure, Transport and Tourism. (2020c). Abandoned Railroads in Recent Years. Available online at: https://www.mlit.go.jp/common/001344605.pdf (accessed January 12, 2021).
Nakagawa, D., Hatoko, M., and Kato, Y. (1994). A Study on the changes of possibility of mutual access by improvement of inter city transportation. Doboku Gakkai Ronbunshu 1994, 47–56. doi: 10.2208/jscej.1994.47
Nezu, Y., Kanda, Y., Koike, A., Shiromizu, Y., and Fujii, S. (2013). Study of prediction of the total effect by building resilient infrastructure in west Japan. J. Jpn. Soc. Civ. Eng. Ser. F4 69, I57–I68. doi: 10.2208/jscejcm.69.I_57
Official Statistics of Japan. (2019). Boundary Data by Municipality. E-Stat. Available online at: https://www.e-stat.go.jp/gis
Ohgaki, S. (2008). “Distinctness and similarity: new indicators for taxonomic,” in Argonauta 15, 10–22. Available online at: http://www.mus-nh.city.osaka.jp/iso/argo/nl15/nl15-10-22.pdf
Pareekh, P., Mitra, S., and Majumdar, B. B. (2017). Quantifying dimensions of transportation diversity: a City-based comparative approach. Trans. Res. Procedia 25, 3174–3187. doi: 10.1016/j.trpro.2017.05.359
Rahimi-Golkhandan, A., Garvin, M. J., and Brown, B. L. (2019). Characterizing and measuring transportation infrastructure diversity through linkages with ecological stability theory. Trans. Res. A Policy Pract. 128, 114–130. doi: 10.1016/j.tra.2019.07.013
Statistics Bureau of Japan. (2016). 2015 Population Census. Available online at: https://www.stat.go.jp/data/kokusei/2015/kekka.html
Tinsley, H. E., and Brown, S. D., (Eds.). (2000). Handbook of Applied Multivariate Statistics and Mathematical Modeling. Academic Press.
Transportation Research Board (1997). “Committee for a study of transportation, and sustainable environment,” in Toward a Sustainable Future: Addressing the Long-Term Effects of Motor Vehicle Transportation on Climate and Ecology, Vol. 251 (Washington, DC: National Academy Press), 254.
United Nations. (2007). Third High-Level Meeting on Transport, Environment and Health (2008). Available online at: https://unece.org/fileadmin/DAM/env/cep/14%20CEP/ECE_AC21_SC_2007_3_e.pdf
Warwick, R. M., and Clarke, K. R. (1995). New ‘biodiversity’ measures reveal a decrease in taxonomic distinctness with increasing stress. Mar. Ecol. Prog. Ser. 129, 301–305. doi: 10.3354/meps129301
Weikard, H. P. (1996). Sustainable Freedom of Choice: A New Concept (No. 11). Finanzwissenschaftliche Diskussionsbeiträge.
Appendix A
Multimodal Transport Solution Introduced in a Regional City of Japan
Yamaguchi City is a mid-sized city, known as the regional core city of the nation, with a population of ~200 thousand people. With a Shinkansen station located a 20-min commuting distance away from the main station in the city center, the conventional railway and bus systems serve as major modes for the city's public transport. Similar to other regional cities in Japan, Yamaguchi City has to meet the challenge of maintaining transport services under a shrinking population and weak demand within the context of growing sustainability concerns. For the following purposes, multimodal transport services have been introduced to Yamaguchi City: (1) to link local tourist destinations with existing transport infrastructure, thereby supporting regional business and development and (2) to improve the connection between the intracity transport network and high-speed rail service, thereby enhancing the availability of public transport. With a variety of transport services integrated on a MaaS platform, a demonstration experiment was started in December 2020 (Figure A1).
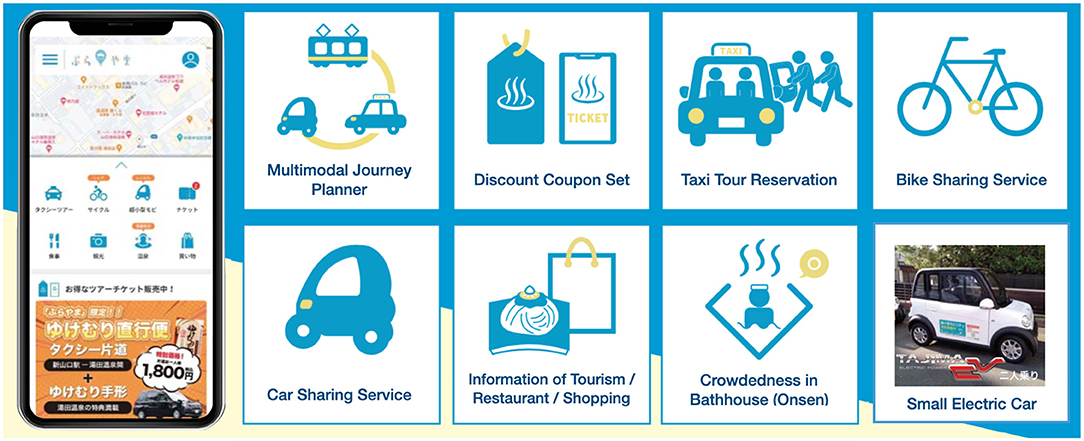
Figure A1. Integration of various forms of transport services into a MaaS platform. Access from: https://maas-y.com/.
To implement innovative urban transport solutions in a local context, Yamaguchi City encourages diverse transport options. The new mobility services introduced in the demonstration experiment include a more flexible tour, free small electric car sharing service, bike sharing service, and discount coupon set combined transport tickets and coupons for local restaurants. The various services are integrated into a single platform (application or web portal) (Table A1), enabling users to access convenient transport options as well as to shape users' experiences and preferences. With greater awareness of the “sharing economy” as well as undertaking ICT solutions, diverse transport options in sustainable transport systems are possible to be achieved without intensive capital investment.
Keywords: public transport, sustainability, diversity index, hierarchical structure, high-speed railway
Citation: Nakase R, Chou C-C, Aoki Y, Yoh K and Doi K (2021) Evaluating Hierarchical Diversity and Sustainability of Public Transport: From Metropolis to a Weak Transport Demand Area in Western Japan. Front. Sustain. Cities 3:667711. doi: 10.3389/frsc.2021.667711
Received: 14 February 2021; Accepted: 03 May 2021;
Published: 31 May 2021.
Edited by:
Kh Md Nahiduzzaman, University of British Columbia Okanagan, CanadaReviewed by:
Luis A. Guzman, University of Los Andes, ColombiaUneb Gazder, University of Bahrain, Bahrain
Copyright © 2021 Nakase, Chou, Aoki, Yoh and Doi. This is an open-access article distributed under the terms of the Creative Commons Attribution License (CC BY). The use, distribution or reproduction in other forums is permitted, provided the original author(s) and the copyright owner(s) are credited and that the original publication in this journal is cited, in accordance with accepted academic practice. No use, distribution or reproduction is permitted which does not comply with these terms.
*Correspondence: Kenji Doi, doi@civil.eng.osaka-u.ac.jp