- 1Doctorado en Geografía, Instituto de Geografía, Pontificia Universidad Católica de Chile, Santiago, Chile
- 2Núcleo de Estudios Sistémicos Transdisciplinarios, Universidad de Chile, Santiago, Chile
- 3Red de Pobreza Energética, Universidad de Chile, Santiago, Chile
- 4Doctorado Territorio, Espacio y Sociedad, Universidad de Chile, Santiago, Chile
- 5Centro de Ciencia del Clima y la Resiliencia (CR)2, Universidad de Chile, Santiago, Chile
- 6Escuela de Gobierno, Universidad Adolfo Ibañez, Santiago, Chile
- 7Facultad de Ciencias Sociales, Universidad de Chile, Santiago, Chile
Energy poverty is a crucial concept in current global energy policy, both for the importance of securing equitable access to high-quality energy services to all human populations and to advance toward a just energy transition to a decarbonized economy. Nonetheless, one of the limitations of this concept due to its focus at the household scale, it has tended to omit relevant energy conditions at a territorial scale, which can also be a dimension of significant deprivation (e.g., transportation, schools, hospitals, public services, industrial uses among others.). On the other hand, energy services are highly dependent on context: on the geographic, ecological, technical, economic, and sociocultural conditions. This context-dependency determines the range of energy and technological alternatives available in a territory. Hence, a conceptual framework is required to better understand the starting point to a just energy transition, capable of integrating the complexity of socio-techno-ecological systems. To fill this gap, we present a framework based on the concept of Territorial Energy Vulnerability (TEV), defined as the propensity of a territory to not guarantee equitable access—in quantity and quality—to resilient energy services that allow the sustainable human and economic development of its population. That is a greater probability of inequity in access to energy services or a significant impacts derived from socio-natural risks that make it incapable of guaranteeing a sustainable and resilient provision of these services. Built on state-of-the-art conceptualizations of risk, we develop an indicator-based framework on vulnerability understood as the combination of sensitivity and resilience characteristics of socio-techno-ecological systems. Sensitivity relates to economic, demographic, infrastructure, technology, culture, and knowledge characteristics of socio-techno-ecological components. Meanwhile, resilience is presented in a three-dimensional framework based on flexibility, register, and self-transformation capacity of socio-techno-ecological systems. An application of this framework is developed using three case studies: Arica, Los Andes and Coyhaique, all Chilean cities with diverse ecological, technical, economic, and sociocultural conditions that shape territorial vulnerability. Using this framework as a diagnostic tool, the development of a just energy transition could adapt existing concepts of energy poverty and decarbonization pathways into context-specific guidelines and policies.
Introduction
Ensuring access to affordable, reliable, and sustainable energy for all is a crucial pillar of sustainable development (United Nations, 2015), supporting as much economic development as the satisfaction of a large variety of human needs (Bhatia and Angelou, 2015; Thomson et al., 2017; Nadimi and Tokimatsu, 2018; Robić and Ančić, 2018). At the same time, however, generation, distribution, and consumption of energy account for more than 70% of global GHG emissions (Climate Watch, 2019), a result of approximately 81% of global energy demand being met by fossil fuels including oil, coal, and gas (International Energy Agency, 2019). Urgent efforts toward the decarbonization of energy systems are needed to maintain global warming below 1.5°C. over pre-industrial levels (Falk et al., 2019; IPCC, 2019a).
The need to simultaneously ensure a secure energy provision, advance toward a more environmentally benign energy matrix, and reduce energy poverty, is known as the energy trilemma, a key challenge for advancing toward sustainable energy development (International Energy Agency, 2018; World Energy Council, 2019). Facing this trilemma requires devising strategies for a just energy transition (McCauley and Heffron, 2018), which complies with climate goals while ensuring energy justice.
Cities play a key role in tackling this trilemma: they are hotspots in the global carbon cycle, accounting for the majority of the CO2 emissions from global final energy use (Nangini et al., 2019), while they also influence and mediate the effects of climate change on society (McCarthy et al., 2010). Conversely, cities can act as frontrunners and sites of innovative climate action and governance (van der Heijden, 2019). This fact urges the development of an integrated approach to design climate-sensitive urban governance, i.e., able to enact mitigative, adaptive, and transformative efforts in the face of a changing planet (Brondizio et al., 2016; Bai, 2018), while also fostering justice and equality. However, up to this point, urban governance remains a strongly fragmented field targeted by a variety of epistemic communities encompassing urban planning, disaster management, and climate vulnerability, to quote just a few (Wolfram et al., 2017).
At the same time, a significant interlinkage exists between urban and energy planning and priorities, so that a stronger understanding is needed of the spatial, political, and ethical dimensions of urban energy transitions, including how these may either transform or reproduce existing forms of injustice and segregation (Rutherford and Coutard, 2014; Debizet et al., 2016; Huang and Broto, 2018). Along these lines, an emerging literature on Positive Energy Districts (PEDs) and Energy Positive Neighborhoods (EPN) has been arguing for a form of urban planning seeking to synergically reduce GHG emissions and alleviate energy inequalities by means of a higher energy efficiency and renewable energy generation (Ala-Juusela et al., 2016; Monti et al., 2016; Walker et al., 2017).
Fostering a just transition based, for example, on PEDs or EPNs, requires paying deep attention to the context in which the transition occurs: territorial characteristics and dynamics, in fact, influence not only the particular energy needs and acceptability thresholds of energy services but also the chances of success of energy transitions efforts and the emergence of potential unintended consequences of said transitions upon the inequality and vulnerability of the target communities (Bouzarovski and Tirado Herrero, 2017a; Frantál and Nováková, 2019; Urquiza et al., 2019; O'Sullivan et al., 2020). Moreover, it must be noted that while often there is a tendency on the part of scholarship and public policy to grant absolute preference to “modern” energy services (mostly based on electricity), place-based evidence discards the possibility to conceive a unique hierarchy among energy sources: not only households tend to mix different sources in their daily routine (combining different kinds of technologies), but the relative desirability of each of these tend to vary according to the specific context, including socioecological, sociotechnical conditions, and sociocultural practices (Scarpellini et al., 2015; García-Ochoa and Graizbord, 2016; Herington et al., 2017; Billi et al., 2018).
On the other hand, methodologies and indicators used to gauge energy poverty and deprivation have tended to pay limited attention to territorial contexts. Even pioneering studies struggling to incorporate these variables have mostly focused their analysis on the household domain. In contrast, little attention has been paid to: (a) non-household manifestations of EP (e.g., related to transportation, workplace, education and health institutions, public lighting, among others), or (b) extra-domestic determinants of household energy deprivation. Moreover, there is a lack of comprehensive approaches clarifying how energy deprivation may be affected by context dynamics, including technological, socio-political, and climatic trends.
To fill this gap, this paper aims to outline an analytical framework to examine what we call “territorial energy vulnerability” (TEV in the following), understood as the propensity of a territory to see its ability to ensure high-quality energy services affected by hazards or dangers such as climate change or socio-natural disasters. TEV is not meant to be a substitute, but rather as a complement, for household EP measures, struggling to provide context and depth to the analysis of energy deprivation and just energy transitions. Territorial energy vulnerability is animated by a holistic understanding of energy security, understanding the latter as the capability of a territory to ensure equitable access—in terms of both quality and quantity—to resilient and sustainable energy services furthering the human and economic development of its population, respecting both planetary and local ecosystem limits (Urquiza and Billi, 2020).
To inform such a framework, we combine notions and insights from energy poverty and sociotechnical transitions literature with those deriving from a socio-anthropological understanding of territorial dynamics, plus the latest advancement of the climate change community on the conceptualization of risk and vulnerability. Also, the integrated view of urban planning proposed in PEDs literature, where multiple energy uses are considered simultaneously (e.g., households, public luminaires, commercial, etc.), and the importance of context-specific trajectories to PEDs is at the core of our territorial framework (Ala-Juusela et al., 2016; Lindholm et al., 2021; Moreno et al., 2021). An analysis based on TEV framework can facilitate the implementation of a just energy transition based on context-specific guidelines and policies coherent with existing concepts of energy poverty and decarbonization pathways (such as PEDs).
To illustrate our framework, we analyze three case studies of major cities in Chile: Arica, Los Andes, and Coyhaique. Chile is a perfect testbed for our approach both because of its strongly varying climatic, economic, and sociocultural characteristics. Because of its condition of a “middle-development” country, it represents a category that has traditionally been under-attended by energy poverty literature (Urquiza et al., 2019). Noticeably, while in this paper we develop the framework with an explicit focus on urban spaces, in the future it could be extended to rural or peri-urban contexts.
The rest of the paper is structured as follows: section Literature review provides a literature review on existing attempts to include a territorial perspective in energy poverty research, discussing the lack of complex and multiscalar understandings of territorial dynamics. Section Theoretical framework illustrates our analytical framework. section Description of case studies introduces our case studies. Section Results presents the data and methods used in the cases studied. Section Results and section Discussion respectively present and discuss our results to broaden our understanding of energy poverty and just energy transition in cities. Finally, the article is closed in section Conclusion with the main conclusions.
Literature Review
Energy poverty has been traditionally measured through either of two pathways: on the one hand, economic indicators, such as the well-known “Ten Percent Rule” (Boardman, 1991), the “Low Income-High Cost” (Hills, 2012), the “Minimum Income Standard” (Moore, 2012) among others, that had mainly focused on the affordability of energy expenditure in high-development countries (Urquiza et al., 2019). On the other hand, energy access indicators such as the Multidimensional Energy Poverty Index (Nussbaumer et al., 2012) have concentrated on geographical or technological barriers to energy, observed in access to technology. This aspect has tended to be especially relevant in low-development countries (Urquiza et al., 2019).
However, both affordability and access-oriented energy poverty indicators must assume quality standards as evaluation criteria (Urquiza et al., 2019). Quality can thus be visualized as a third “hidden” dimension of energy poverty, in two relevant senses: as underlying the need to adopt quality standards suitable to different particular socioeconomic, cultural, climate, and geographical conditions as a precondition to evaluate both energy affordability and access; and as an object of evaluation in its own sake—e.g., in terms of reliability or safeness of energy provision—. As argued in Urquiza et al. (2019), this hidden dimension is significant when observing energy poverty in territorially heterogenous, middle-development countries like Chile.
The quality dimension, in turn, drive us to think about patterns and determinants of energy availability—or lack thereof—in a territory and how this impacts household energy poverty. In this context, the link between the energy trilemma (International Energy Agency, 2018; World Energy Council, 2019) is fundamental: to tackle energy poverty, we need to improve reliable and high-quality energy provision, that is to say, adequate, reliable, safe, and non-polluting energy, to reach an environmentally benign energy matrix. However, energy provision depends on territorial structural conditions (such as infrastructure, politics, markets, among others) that contribute to reproduce spatial inequalities related to energy. Bouzarovski et al. (2015) have emphasized the importance of understanding the sociotechnical legacies of countries' development trajectories to understand the political and economic landscape that frame energy poverty expressions; these conditions can be understood as driving forces of energy poverty.
The effect of household characteristics on the probabilities of becoming energy poor is well known (Imbert et al., 2016; Belaïd, 2018; Primc et al., 2019), but recent literature has expressed the importance of a geographical analysis where energy poverty conditions can relate to phenomena occurring at a larger scale (Bouzarovski and Simcock, 2017; Robinson et al., 2019). Scientific literature offers at least two different ways to understand the spatial dimension of energy poverty.
First, some authors have emphasized the importance of spatializing energy poverty, e.g., understanding it through a spatial lens as an energy injustice problem (Bouzarovski and Simcock, 2017; Bouzarovski et al., 2017; Robinson et al., 2019). They have identified how spatial variables can explain energy poverty expressions more precisely than other variables like household socioeconomic conditions (Jimenez, 2017; Besagni and Borgarello, 2019; Mattioli et al., 2019). This has been studied especially in the case of Central and Eastern European countries (Bouzarovski et al., 2015; Bouzarovski and Tirado Herrero, 2017a; Sokołowski et al., 2020) but increasingly in other European countries (Besagni and Borgarello, 2019; Mattioli et al., 2019; O'Sullivan et al., 2020), highlighting geographical disparities and central-periphery relations in the EU community (Bouzarovski and Tirado Herrero, 2017b; Golubchikov and O'Sullivan, 2020). In the same context, energy poverty incidence has also been related to neighborhoods' urban and architectural conditions, emphasizing the importance of understanding the historical context and development of housing complexes in urban and rural areas (Besagni and Borgarello, 2019).
The second perspective of territorial effects on energy poverty has paid attention to climatic and geographical conditions that generate differentiated energy poverty expressions, such as in the case of islands (Wolf et al., 2016; Surroop et al., 2018; Ioannidis et al., 2019; Lozano et al., 2019). An emphasis has been made regarding how altitude (Katsoulakos and Kaliampakos, 2016; Papada and Kaliampakos, 2016), temperature (Puzzolo et al., 2016; Kerimray et al., 2018; Besagni and Borgarello, 2019), and climatic variability (Ioannidis et al., 2019) relate to different energy needs that households can have. Similarly, literature has stressed the importance of daily (Puzzolo et al., 2016) and seasonal variability (Puzzolo et al., 2016; Pollard et al., 2018) of these conditions. This group of the energy poverty literature usually emphasizes how remote (Dugoua et al., 2017; Pollard et al., 2018), mountainous (Katsoulakos and Kaliampakos, 2016; Papada and Kaliampakos, 2016), or isolated areas face specific conditions that create barriers for improved access to cleaner, more efficient fuels, posing a challenge to energy transitions.
Both perspectives, the spatial expression of energy poverty and geophysical conditions as a cause of energy poverty, have been observed across different scales, for example, climate macrozones that can produce a different heat demand (Besagni and Borgarello, 2019); geographical conditions like isolation (Dugoua et al., 2017) that can condition energy access; the spatial distribution within a city (Mattioli et al., 2019) or between different regions (Bouzarovski and Tirado Herrero, 2017a); among others. Other studies have focused on how economic conditions at a regional scale can increase the risk and prevalence of energy poverty conditions creating an regional risk measure or structural energy poverty vulnerability, such as energy prices, income, unemployment rate, labor market conditions, energy market characteristics, among others (Dodson and Sipe, 2006, 2008; Velte et al., 2013; Recalde et al., 2019).
Furthermore, some authors (Bouzarovski and Thomson, 2018) identify differences within urban contexts, producing different landscapes and typologies of energy vulnerability, forming particular socio-spatial forms of inequity. This literature also concludes that expenditure indicators are insufficient to describe the complex ways in which socio-spatial vulnerabilities interplay toward an intensification of energy poverty conditions, related to equally complex geographical and demographic contexts (Robinson et al., 2018).
The relationship between these two perspectives—that is, between the literature on the spatial expressions of energy poverty and the literature on climate and geography as drivers of energy poverty—is complex, sometimes overlapping (Bouzarovski and Simcock, 2017). Despite these considerations, this literature still fails to integrate both perspectives better and describe territorial determinants of energy poverty.
Regarding this issue, there have been some notable contributions in this sense from Robinson et al. (2019) and Mattioli et al. (2019). These authors have made efforts to assess the occurrence of energy poverty at a smaller, neighborhood-like scale. Similarly, Besagni and Borgarello (2019) and Pollard et al. (2018) have made efforts to produce a detailed sub-national disparities outlook. All these proposals, however, support what Bouzarovski and Simcock (2017) have noted, namely that “aggregating and averaging figures over units of political and material space both reveals and hides differences; justice (…) defined at one scale does not necessarily mean justice is achieved elsewhere” (p. 642). The recognition of the complex spatial embeddedness (Bouzarovski and Tirado Herrero, 2017a) of energy poverty is, however, still tied to the availability of sufficiently detailed, spatially disaggregated data.
Another aspect not commonly covered by energy poverty literature is that energy poverty conditions, i.e., unequal access to high-quality energy services, can also occur outside the household, especially in crucial social services such as education and health institutions, but also in diverse workplaces like offices, outdoor work, commerce, among others. Some articles have done this by focusing on relevant energy uses that are often not considered in the broader context of energy poverty, such as transport (Mattioli et al., 2019). The recognition of the relevance of educational, health-related and other social and productive uses of energy has been present mostly in the energy transition literature (Wolf et al., 2016; Surroop et al., 2018; Lozano et al., 2019).
Finally, and most important for this paper, there remains a limited understanding of how spatial and territorial determinants could mediate the vulnerability of the energy system to potential climatic, economic, or socio-political threats, thus potentially generating or increasing future energy deprivation. Hence, recent literature suggests the importance of developing a dynamic perspective of energy poverty that may address this aspect, where not only the analysis of the current energy access gaps is necessary, but also a reference to short and long-term impacts on high-quality, equitable energy access, that is, the risk of the energy system (Urquiza and Billi, 2020).
Several studies (Dugoua et al., 2017; Baptista, 2018; Besagni and Borgarello, 2019; Munro and Bartlett, 2019) emphasize the importance of considering the historical context of specific technical and sociocultural trajectories, and how energy poverty changes not only through space but also through time. However, usually, there is a lack of attention to the “risk” component of energy security, especially considering the impacts of the new globally changing scenarios. Relevant scholarship on energy vulnerabilities (Middlemiss and Gillard, 2015; Petrova, 2018; Ioannidis et al., 2019; Mattioli et al., 2019; Robinson et al., 2019) has focused on household sensitivity toward energy poverty while neglecting a broader systemic perspective that integrates environmental hazards (e.g., climate change) and sociotechnical complexities (e.g., energy transitions as a source of uncertainty to some energy system actors). Some authors have emphasized that energy transitions can increase social vulnerability—rather than decreasing it—highlighting the need to consider the links between energy vulnerability and energy transitions from a geographical perspective (Bouzarovski et al., 2017).
Even though there is a growing literature that considers, on the one hand, energy vulnerability (Middlemiss and Gillard, 2015; Petrova, 2018) and on the other the multiscalar nature of the problem, there is a need for a comprehensive framework able to embrace the complex territorial determinants of energy vulnerability and poverty, considering not only sociotechnical conditions but also socioecological and sociocultural domains. A narrowed approach can cause energy transition policies to rely on energy poverty and security measures that underestimate the complexities of sociotechnical processes existing in-between households and with national energy systems.
Improving the attention to the “risk” component through a TEV lens can provide a tighter link with sustainable development and energy transition literature, providing more tools to analyze temporal dynamics within energy poverty.
Theoretical Framework
Vulnerability in Risk Literature
Following the conceptualization of the Intergovernmental Panel on Climate Change (IPCC) on its fifth assessment report, risk is a multidimensional concept understood as the probability that something of value to society would be in danger with an uncertain outcome, as a result of the interaction between three components: hazard, exposure, and vulnerability of the system [IPCC, 2012, 2019b; Centro de Ciencia del Clima y la Resiliencia (CR)2, 2018]. In this proposal, vulnerability is understood as a system's propensity to suffer negative impacts derived from a hazard [IPCC, 2012, 2019b; Centro de Ciencia del Clima y la Resiliencia (CR)2, 2018].
In turn, Vulnerability can be decomposed into two dimensions: sensitivity, understood as the systemic characteristics that increase the probability of the exposed components to suffer negative impacts, and response and adaptation capacity, which relates to the systemic capacity of facing and overcoming adverse conditions presented by hazards, exposure, and sensitivity.
Following previous efforts [Centro de Ciencia del Clima y la Resiliencia (CR)2, 2018], we propose to relate the concepts of response and adaptation capacity with the thriving literature on resilience, which would allow for a more systemic and holistic account on the matter. Although there are different definitions of resilience, one of the most known conceptualizations comes from the ecological systems literature and is understood as the capacity of a complex system to reduce the impact of disturbances by adapting and/or preserving its structural relationships (Folke et al., 2005; Cumming, 2011). In this conceptualization, the system maintains its identity and essential characteristics (function and organization), but multiple balance points are considered as viable, avoiding a rigid conceptualization of resilience (Gunderson and Holling, 2002; Folke, 2006; Cumming, 2011).
The sensitivity varies depending on the specific threats the system faces. Conversely, the response and adaptation capacities—in addition to having a specific expression to a particular hazard—can also be understood from a generic perspective. This means that resilience can be visualized as emergent characteristics of the system, allowing it to maintain its functions in the face of multiple threats, in some cases unknown. For example, a response protocol can be designed for socionatural disasters, but it can later be adapted to other types of hazards and increase the system's response capacity in general. On the other hand, an adaptation strategy whose focus is on scientific research and public awareness of risks could identify new sources of risks not priorly acknowledged. This article aims to describe energy systems' vulnerability; hence, a specific point of view will be developed centered on energy sociotechnical systems.
Moreover, resilience could be analyzed from two perspectives. First, an expressive perspective focused on the effects of disturbances and how the system could reduce the impacts and maintain its services after the event occurred. Second, from a predictive perspective focused on the characteristics of structures and components of a system that could estimate a system's resilience to a future threat (Folke, 2006; Urquiza and Billi, 2018). Although both perspectives are relevant, this work's interest is to account for a predisposition toward energy vulnerability, therefore, it focuses on a predictive perspective of resilience.
As previously argued [Centro de Ciencia del Clima y la Resiliencia (CR)2, 2018], there are three attributes associated with a resilient system: flexibility, memory, and self-transformation [Biggs et al., 2012; Centro de Ciencia del Clima y la Resiliencia (CR)2, 2018; Urquiza and Billi, 2018]. A resilient system is one that can react adequately and re-establish the provision of its services after a threat (flexibility); generate information and learning about its relationship with the environment, considering the reaction of its structures to hazards (memory); and adapt and/or transform its structures and components to maintain its services over time (self-transformation) (see Table 1). This transformation can occur spontaneously or in a planned way, based on specific objectives. The latter is crucial when talking about complex adaptive systems with cognitive capacities—such as socioecological, sociotechnical or sociocultural systems—since they have the quality of reflexively adapting to their environment (Gunderson and Holling, 2002; Berkes et al., 2003; Luhmann, 2006).
The literature relates at least three characteristics to flexibility: diversity, connectivity, and redundancy of its components and structures (Smith and Stirling, 2010; Molyneaux et al., 2016; Binder et al., 2017). Second, memory relates to the production of information about system functioning and its relationship with the environment (distinguishing itself from it) and with the ability to produce learning from this information (Berkes et al., 2003; Folke et al., 2005; Nykvist, 2014). Finally, self-transformation implies the degree to which the coordination of the system components, the capacity for anticipation and decision-making allow a socioecological or sociotechnical system to adapt to a threat and manage the risk it faces (Desouza and Flanery, 2013; Urquiza Gómez and Cadenas, 2015; Urquiza and Billi, 2018). This quality of the system can be aimed at adapting current structures over time (adaptive governance) or transforming these structures toward new equilibrium (transformative governance) (Biggs et al., 2015; Centro de Ciencia del Clima y la Resiliencia (CR)2, 2018).
The state of this set of characteristics (flexibility, memory, and self-transformation) is historically dependent and in turn conditions the system's response and adaptation capacity. In this way, with greater flexibility, learning, and self-transformation, a socioecological, sociocultural, or sociotechnical system can better face the hazards and manage the risks it experiences (Folke et al., 2005; Desouza and Flanery, 2013; Urquiza and Billi, 2018).
Vulnerability in Energy Sociotechnical Systems
As we are changing the scale of analysis from household to territorial level, the concept of sociotechnical system is interesting to integrate the diversity of vulnerability characteristics present both inside and outside the household. Sociotechnical systems literature acknowledges the importance of considering both the technological (hard) aspects and the structures, agents, and processes (soft) that shape the development of complex systems such as energy, transport, among others (Rip and Kemp, 1988; Smith and Stirling, 2010). Elements such as artifacts, knowledge, economic capital, human resources, cultural meanings, natural resources, regulations, among others, are the central focus of sociotechnical analysis (Geels, 2002, 2004, 2011).
Hence, energy sociotechnical systems' sensitivity and resilience characteristics should be observed considering infrastructure, regulations, institutions, actors, and organizations involved in the production of energy services and technologies (Geels, 2004). At least five components of energy sociotechnical systems should be accounted for in the context of vulnerability analysis (see Figure 1 for a graphical summary).
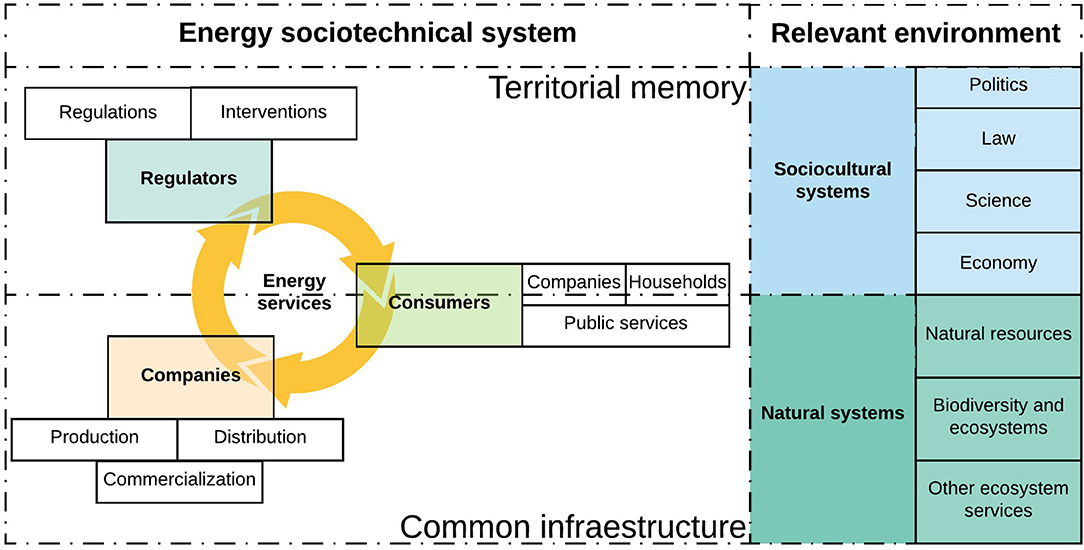
Figure 1. Schematic representation of energy sociotechnical system. Source: adapted from Red de Pobreza Energética (2020).
First, companies that produce, distribute, and commercialize energy and technologies, commonly oriented by economic criteria, even though in some contexts they can be part of the public sector, meaning the existence of mixed criteria in decision making. Their role and behavior in sociotechnical systems depend on characteristics such as economic resources, infrastructure, technologies, organizational culture, and specialized knowledge (Arnold-Cathalifaud, 2008; Rodriguez, 2008).
Second, regulators are the actors in charge of applying the normative ruleset that configure the interactions within an energy sociotechnical system as well as the design and implementation of policies that directly intervene on infrastructure, technologies, markets, and energy uses (Geels, 2004; Urquiza et al., 2018). As an organization, a government's capacities depend on its economic resources, infrastructure, technologies, organizational culture and knowledge, but its decisional logic is based on a complex relationship between political and technical criteria (Arnold-Cathalifaud, 2008; Rodriguez, 2008).
Third, technology and energy consumers who require high quality energy services related to food, hygiene, air conditioning, lighting, electrical devices, and productive uses to meet their needs (Urquiza et al., 2019). Among consumers we find households—the usual focus of energy poverty literature—but also, from a territorial perspective, companies that produce goods and services, and public services, which implies a diversity of both rationalities and energy needs. On the other hand, these consumers can be producers of energy at the same time when they have the technological capabilities or resources required. Examples of this possibility are generation based on solar energy or, in some contexts, the use of firewood from recollection in nearby forests (Schueftan et al., 2016).
These three components interact in an immediate level within the energy sociotechnical system, and they are the ones who, based on their constant interactions, shape the specific structures that Geels defines as sociotechnical regime. Two other components are relevant from a territorial perspective as they represent a specific base upon which companies, regulators, and consumers interact: the territorial memory and the common infrastructure and resources.
Territorial memory refers to a territorial system's capacity to learn about the relationship with its immediate environment, considering its structures and processes (Urquieta et al., 2017; Amigo, 2019). This is expressed in the availability of knowledge, information, decisions, and historical experiences, but also in values and perceptions, which condition the disposition to technologies and energy uses. This notion rests upon a rich field of discussion regarding the complex cultural values, perceptions, assemblages, and flows through which energy systems are shaped at territorial levels (Stephenson et al., 2010; Strauss et al., 2016; Munro and Bartlett, 2019; Kumar, 2020).
The common infrastructure corresponds to the artifacts and material components of shared use necessary for the generation, distribution, and energy use. Given that some aspects of the sociotechnical energy system require high-cost investments (Makki and Mosly, 2020), it is common to encounter policies designed to incentivize the adoption or construction of a particular technology (Geels, 2004; Acosta et al., 2018).
This energy sociotechnical system has constant interactions with its environment, especially with some elements of the sociocultural and ecological systems that are crucial for its operation. Regarding sociocultural systems (Luhmann, 2006), the political system is relevant as its decisions impact public organizations and market characteristics; the legal system generates laws and regulations, which synthesize the formal “rules of the game” for various aspects of sociotechnical systems; the scientific system generates knowledge and applied research on relevant technologies for the production and use of energy; and the economic system conditions the demand and energy supply both at macro and microeconomic scales (Geels, 2004).
In the case of ecological systems, they provide various contributions necessary for the operation of the system, among them the natural resources used for the production, distribution and use of energy (Kadykalo et al., 2019); and the climatic conditions of the territory, which affect the energy demand but also on the energy potential of the territory. Likewise, the energy sociotechnical system impacts biodiversity and ecosystems, primarily due to pollutants' emissions at a global and local scale (Rogelj et al., 2018; IPCC, 2019a).
Territorial Energy Vulnerability
Considering the above, TEV is understood as the propensity of a territory to not guarantee equitable access—in quantity and quality—to resilient energy services that allow the sustainable human and economic development of its population. That is, a greater probability of gaps in access or inequity in quality energy services or significant impacts derived from socio-natural risks that make it incapable of guaranteeing a sustainable and resilient provision of these services.
This propensity is conditioned by the sensitivity and resilience characteristics of the energy sociotechnical system of the territory. The sensitivity characteristics refer to economic resources, sociodemographic, infrastructure, technological, cultural, and knowledge characteristics of the sociotechnical systems' main components (companies, regulators, consumers, common infrastructure, and territorial memory). On the other hand, resilience refers to the capacity for response and adaptation that emerges from the capacity for flexibility, memory, and self-transformation of the energy system structure. In this way, the emergent combination of these conditions (sensitivity and resilience) describes a specific sociotechnical configuration that affects availability, prices, demand, reliability, security, adequacy, and pollution of the sources and technologies used for energy services.
This sociotechnical configuration helps describe a global view of the context where gaps in equitable access to high-quality energy services occur for various users in a territory. Also, given a certain hazard, TEV will make it possible to understand, and in some cases project, how the energy sociotechnical system would react, visualizing the intermediate impacts and the risk that this entails on access to high-quality energy services (see Figure 2).
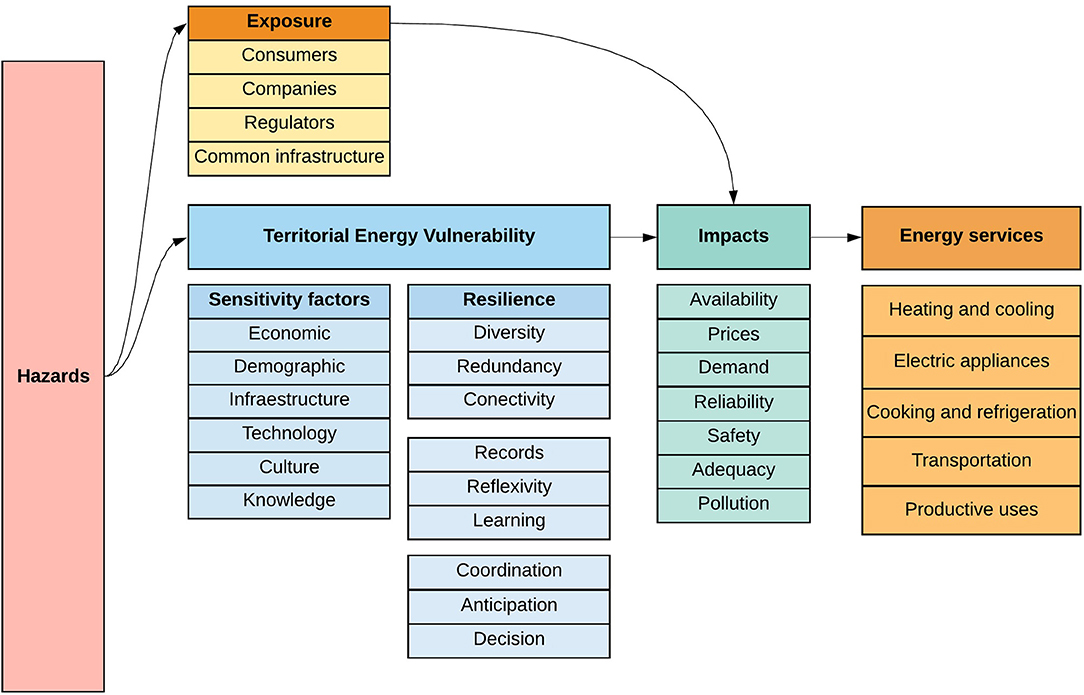
Figure 2. Schematic representation of territorial energy vulnerability. Source: adapted from Red de Pobreza Energética (2020).
Description of Case Studies
To test the heuristic potential of the TEV framework proposed in this paper, we analyze three middle-sized Chilean cities located in different climates: Arica, a city located at the northern coast of Chile with a hot-desert climate; Los Andes, a cold semi-arid inland city located in a valley near Andes mountains; and Coyhaique, a tundra inland city located in the southern extreme of Chile, within near Patagonia (Sarricolea et al., 2017). A brief description of case studies is presented in this section, providing general geophysical, climate and demographic characteristics.
Arica, Arica and Parinacota Region
The city of Arica (18°28′S, 70°18′W) is Chile's northernmost urban settlement, and it is the capital of the Arica and Parinacota Region (see Figure 3). Its location is close to national borders with Perú (within 15 km) while being considerably distant (over 300 km.) from other similarly sized cities in Chile. This port city has 221,364 inhabitants, which is over 90% of the regional population, and doubles (8%) the national average (4%) proportion of the international migrant population (Instituto Nacional de Estadísticas, 2018).
According to data from the (CR)2 (Ministerio del Medio Ambiente, 2020) for the 1981–2010 time period, the annual average temperature in Arica was 17.2°C, with a thermal amplitude of 9.2°C. Likewise, The annual daily maximum temperature for the period 1981–2010 was 27.9°C, while the average daily minimum is around 12.6°C. Even when located within the Atacama Desert, its location on a coastal area explains the fact that on average onlyapproximately 83 days of the year exceed 25°C as a maximum temperature.
Los Andes, Valparaíso Region
The city of Los Andes (32°50′S 70°37′W) is a mid-sized urban settlement with 66,708 inhabitants located on the Aconcagua Valley near the Andes mountains (Instituto Nacional de Estadísticas, 2018). Its main economic activities are agricultural and cooper mining, strongly influencing its surrounding landscape.
Located in a semi-arid climate, Los Andes has an average temperature of 13.3°C, with a thermal amplitude of 14.9°C. The annual daily maximum temperature for the period 1981–2010 is 31.1°C, while the average minimum is just over 5.9°C. Unlike Arica, Los Andes has 107 days of the year reaching over 25°C as a maximum temperature, of which 20 days exceed 30°C. Another aspect to highlight is that the temperature of the coldest day reaches 6.3°C on average.
Coyhaique, Aysén Region
Coyhaique (45°34′S, 72°40′W) is one of the southernmost cities in Chile. Located in western Patagonia, it is the largest urban settlement on the Aysén Region and it's Region's capital city, with 57,818 inhabitants. Settled at the beginning of the twentieth century, it has historically relied on the surrounding livestock, forestry resources, and natural tourist attractions as its main economic activities. Due to massive residential firewood use as a heating and cooking source, high PM2.5 concentrations are common throughoutthrough the coldest months of the year (Huneeus et al., 2020).
Coyhaique's annual average temperature for the 1981–2010 period was 7.6°C, with a thermal amplitude of 10°C. This period's daily maximum temperature corresponds to 28.2°C and has the lowest daily average minimum temperature of the three case studies, reaching 2.6°C in the 1981–2010 period. Also, this city has the fewest days that exceed 25°C, reaching only 6 days of the average year, and where the coldest night temperature goes down to −11.5°C, with 92 days of the year with a minimum temperature below 0°C.
Data and Methods
As stated above, we follow an indicator-based methodological structure inspired by the work of IPCC Assessment Report 5 and the Center for Climate concept of risk, Resilience Research (CR2) Vulnerability Framework and GIZ climate change impact evaluation methodological guidelines [IPCC, 2012; GIZ, 2017, 2018; Centro de Ciencia del Clima y la Resiliencia (CR)2, 2018].
To describe the territory's sensitivity factors related to hazards and gaps in equitable access to high-quality energy services, a set of indicators were estimated for the three case studies (see Table 2). Although the proposed framework in section Theoretical framework comprises six dimensions (economic, demographic, infrastructure, technology, culture, and knowledge), in this article only four of them will be included due to lack of data related to culture and knowledge dimensions.
Finally, to describe the resilience of the sociotechnical system, we use analysis of the set of indicators presented in Table 3 to understand better the flexibility, memory, and self-transformation characteristics of the system.
Results
In this section, we offer a description of sensitivity characteristics of Arica, Los Andes, and Coyhaique that impact present and, probably, future conditions of energy access and could become barriers to just energy transition policies. Also, we describe some characteristics of the energy system and territory that can help to project response and adaptation capacity (resilience) of each case study.
Sensitivity Analysis
Demographic Characteristics
Regarding the sociodemographic characteristics of the populations of each case, all three cities share a relatively similar dependency ratio (ratio between economic active population and children and elderly): 48.9% for Arica, 46.89% in the case of Los Andes, and 47.5% for Coyhaique, above of the national dependency ratio (45.9%) as registered by the last National Census in 2017 (Instituto Nacional de Estadísticas, 2018). The presence of vulnerable age groups shows differences between these cities. While the proportion of the elderly population (above 65 years) in Coyhaique is 7% of its total, Arica and Los Andes proportions are 10 and 10.8%, respectively, reaching the same national average levels (10.6%). On the other hand, the presence of children (under 5 years) is relatively similar in these cities, although Arica (7.2%) and Los Andes (6.1%) exhibit a slightly greater proportion than Coyhaique (5.8%), the latter closer to the national average (5.7%).
As for women-led households, all cities share similar proportions as well, not far from the national average of 42%: 44.8% of Arica's households were headed by women, 41.3% in the case of Los Andes, and 43.4% in Coyhaique (Instituto Nacional de Estadísticas, 2018).
These demographic conditions are useful to begin to understand the energy needs present in each city and to identify factors that increase vulnerability of the territory. For example, the higher presence of children and elderly population in Arica and Los Andes could increase negative impacts of heatwaves in present conditions. Also, the presence of women-led households could increase negative impacts of energy prices shocks given the gender income inequalities present in Chile.
Socioeconomic Characteristics
Los Andes and Arica have a 7 and 8.3%, respectively, of households under conditions of income poverty, different from Coyhaique, which stands out with only 2.7% of households living below the national poverty line. According to a multidimensional poverty index (Ministerio de Desarrollo Social, 2017), a different trend is observed as Coyhaique has 18.1% of poor households, Los Andes 11.89% of households living in poverty, and Arica with 21.1% of households. Given the greater presence of poor households in Arica, even though it is still relevant in Los Andes and Coyhaique, energy transition policies (such as PEDs guidelines) should be careful not to increase vulnerability and include equity principles in its design.
Regarding electricity prices (for an average household consuming 180 kW/h) exhibit similar magnitudes in these cities near the national average cost of US$ 0.17 per kW/h. In Los Andes and Coyhaique, prices are slightly higher (US$ 0.18 per kW/h), and Arica cost is slightly lower with US$ 0.16 per kW/h. It is worth noting that these electricity prices are regulated by the Tariff Equity Law designed to transform highly unequal energy prices in previous decades, this law reduces prices so that no bill is 10% above the national average (calculated for a standard household with a 180 kW/h monthly bill). Official estimates point out that both Arica and Coyhaique are currently benefited by this measure, with a 16 and 52% reduction in electricity bills, respectively (Ministerio de Energía, 2017a).
Infrastructural and Technological Characteristics
Each city's technological and infrastructural characteristics have social and environmental implications that increase the propensity to energy-related deprivations. First, due to the massive use of high humidity firewood, a higher concentration of particulate material 2.5 (PM2.5) is observed in Coyhaique (Red de Pobreza Energética, 2019), reaching an annual average of 39.5 μg/m3 in 2019 and at least 92 days of the same year above 50 μg/m3 daily average according to the data from the National Air Quality Information System (SINCA). On the same topic, Arica's 2019 annual average was 11.8 μg/m3, however, the sources of these particles were related to vehicle emissions and other minor sources. Unfortunately, there are no air quality stations in Los Andes.
In terms of infrastructure, the housing stock built before 2000 was not regulated by nationally thermal enforced from the year 2000, meaning that at least 66% of these buildings suffer from thermal efficiency problems (Schueftan and González, 2015; Red de Pobreza Energética, 2019). This proportion rises to 70% in Arica and Coyhaique, in Los Andes, this proportion is comparatively lower with 50% of the dwellings, but still is a significant number of households. This condition increases the vulnerability to harsh weather conditions (such as extreme cold in Coyhaique, and heatwaves in Los Andes and Arica) as households struggle to cope with unhealthy indoor temperatures.
Coyhaique stands out with 93% of households that use firewood with a higher household consumption (see Table 4). The latter is also true for Liquified Petroleum Gas usage, for which Coyhaique also consumes significantly more than Los Andes and Arica. Both of these results can be attributed to the higher base heat demand in Coyhaique households due to climatic conditions (Schueftan and González, 2015; Sarricolea et al., 2017; Red de Pobreza Energética, 2019).
Finally, the proportion of forest or shrubland cover within the city is interesting because a greater presence of urban vegetation reduces heat waves and heat island impacts (Salmond et al., 2016; Zölch et al., 2016). According to the classification proposed by De Gregorio and Jansen (2005) and applied by (Hernández et al., 2016; Zhao et al., 2016), Coyhaique has the highest proportion of its area covered by forest or shrubland, reaching 52%, while Los Andes comprises 14% and Arica only 1% of its total area.
Resilience
Resilience could be observed in emergent properties of energy sociotechnical systems such as flexibility, memory, and self-transformation capacity. The Chilean National Electrical System provides on-grid connections for 14 of the 16 regions in the country's continental ground. Autonomous from this large system, Mid-sized Electric Systems are not connected to the National System and supply electricity to smaller and distant cities. Coyhaique is supplied by one of these Mid-size systems (Sistema Eléctrico Aysén, SEA); meanwhile, Arica and Los Andes are part of the National System.
One aspect of resilience related to flexibility is the response capacity of the system. In this regard, Coyhaique had an average System Average Interruption Duration Index (SAIDI) between 2015 and 2018 of 22.8 h; meanwhile, Los Andes and Arica had 10.7 and 15.6, respectively, in the same period. The extreme weather conditions of Coyhaique, where snowstorms are common in winter, are a source of electric interruption; nonetheless, the national average SAIDI of 15.7 suggest that quality of Chilean electric service is low compared to international standard and its vulnerability to diverse kind of hazards results in high interruption duration. The Coyhaique electric source's autonomous condition could amplify the risks if the system is not resilient to the local conditions that it faces.
A second interesting energy system feature is its dependency or autonomy of its installed capacity related to domestic demand. This measure is better understood at a regional scale rather than at a city scale, because given the centralized model of energy generation in the Chilean case, cities are dependent of generation units located outside city borders commonly associated with industrial uses. Hence, regional energy systems have a large impact on the city energy supply's resilience. In this dimension, Arica and Parinacota Region (Arica) have a dependency of −67% of its total electricity consumption, meaning that the 60 GWh produced locally are not sufficient to meet the 185 GWh required to its domestic demand. Even though this city is connected to the National Electric System, given the large distance between cities (for example, between Arica and Los Andes there are ~2,000 km by road) the operational cost of electric grids could increase if no local generation is secured, moreover, given the greater temperatures projected under climate change scenarios the efficiency of these transmission lines could be dramatically decreased (Burillo et al., 2019).
Aysén Region (Coyhaique) has an external dependency of its electricity consumption of −59% as its 27 GWh produced locally are not capable of meeting the 67 GWh demanded, hence, being an autonomous system, currently, private off-grid self-generation technology helps to overcome this deficit. Valparaíso Region (Los Andes), on the other hand, produces approximately 3.5 times the domestic demand of 4.026 GWh.
A distributed energy system on urban settlements could improve response capacity of the system if its design is coherent with the territory's renewable potential as improve redundancy of energy supply—in addition to reduction of GHG emissions. In this regard, Arica and Los Andes cities have a high photovoltaic potential as the annual average of Global Horizontal Irradiance is 5.7 and 5.75 KWh/m2/day, respectively. Coyhaique is far behind with 3.45 KWh/m2/day, well below the national average of 4.96 KWh/m2/day (Ministerio de Energía, 2017b). This geophysical condition can explain that the present distributed potential capacity of Arica is 471.4 KW, Los Andes 393 KW, while Coyhaique only sum a total of 25.5 KW (Comisión Nacional de Energía, 2020). This aspect is relevant to PEDs oriented policies in these cities given that northern regions of Chile (e.g., Los Andes, Arica) have high photovoltaic generation potential, even at a global scale.
Another aspect of flexibility relates to the diversity present in the energy matrix of each system (Molyneaux et al., 2012, 2016; Binder et al., 2017). Shannon-Weaver index (Binder et al., 2017) of these three cities describes that Aysén Region (Coyhaique) installed electricity capacity is the less diverse of the three regions compared with 0.37 index (where 0 means no balance and diversity, and 1 the opposite), followed by Arica and Parinacota Region (Arica) with 0.46 and Valparaiso (Los Andes) with a 0.57 Shannon-Weaver balance/diversity index. This is caused by the importance of Diesel generation in Aysén and Arica and Parinacota with 54 and 41% of the total installed capacity, respectively (see Table 5). In both cases, the second most important electricity source is mini-hydro generation with 39 and 33%, respectively. On the other hand, Valparaiso Region has a more diverse electricity generation matrix with Natural Gas, Coal, and Diesel as its main source (46, 24, and 18%, respectively).
The relative importance of renewables in the Chilean energy matrix is increasing faster than expected, hence, the observed 25% of photovoltaic solar generation in the Valparaíso Region and the 5% of wind power generation observed in Arica and Parinacota. Although it is crucial that renewables become the main energy source worldwide to reduce GHG emissions, it is also vital to ensure that its variability does not pose a vulnerability to the energy system but as a source of diversity and sustainability.
Other dimension of resilience relates to the capacity to store, publish, and learn from diverse kinds of data sources about the energy sociotechnical system's functioning. In this regard, official open data platforms such as www.energiaabierta.cl or www.energiaregion.cl display a large quantity of information at national and local scale about installed capacity, generation, energy prices, among other crucial information about electricity and LPG. Nonetheless, there is a large gap on open demand-side information such as residential, commercial, or industrial consumption.
Data surveys about residential use are applied bi-annual at commune scale, in the case of de Socioeconomic Characterization Survey (Ministerio de Desarrollo Social, 2017), but only consider information about energy sources chosen by households and not about quantity, expenses, technological characteristics, among others crucial data to estimate residential consumption and access. Other relevant household surveys include a detailed view of energy consumption, technological and dwelling characteristics, even about informal markets such as firewood consumption, nonetheless, their application occurs sporadically and led by private consultants (Corporación de Desarrollo Tecnológico, 2010, 2015, 2019). Smart meters, capable of producing real-time data, are still reduced to a small percentage of users and policies to installed this kind of technology have faced major public scrutiny (Revista Electricidad, 2019).
Data about other kind of consumers—public, commercial, or productive uses—are less accessible than residential data, even at aggregated level. In this regard is relevant to note that energy models used to evaluate energy policies in Chile, such as climate change mitigation plans, rely heavily on assumptions of energy consumption rather than empirical data (MAPS Chile, 2014; Centro de Energia, 2019).
The third dimension of resilience proposed in this article relates to the capacity to self-transform the energy sociotechnical system's structure and components. This dimension is closely linked to governance and political system, hence on of the aspects to analyze is the presence of policies oriented to regulate and transform the actual energy sociotechnical system. One of these policies in the Chilean context is called Local Energy Strategy, which comprises a detailed participative diagnostic on the city's energy system, consumption, access, and energy potential. This instance could enhance local governance of the energy system in the context of a highly centralized political system. Both Los Andes and Coyhaique have already defined a Local Energy Strategy which highlight some of the aspects already exposed in this article (Municipalidad de Coyhaique, 2014; Municipalidad De Los Andes, 2017).
On this topic is relevant to mention the recent failure of a massive change of electricity meters to smart ones due to public rejection of the project, mainly because public opinion refused to the idea that households would have to pay for its installation costs (Revista Electricidad, 2019). On the contrary, “Energy 2050” was a relatively successful long-range participative policy construction where private, public, and scientific actors engaged together in a forecasting exercise that built a broad image of energy system development in Chile and is still legitimated for most of stakeholders as a guideline (Urquiza et al., 2018).
Discussion
The results presented above show how climatic, demographic, economic, infrastructural, technological, and institutional variables combine into very different profiles of TEV across the three case studies.
Arica is the largest city analyzed where sensitivity characteristics related to the presence of age vulnerable population (both children and elderly), higher proportion of both income and multidimensional poverty and as an older city, most housing stock was constructed without regulation. Resilience capacity in this city seems to be transforming as renewable electricity generation (solar and hydro) covers up to 74% of total installed capacity and is expected to grow over time (Ministerio de Energía, 2015). Nonetheless, its dependency on the national electric system to meet its domestic demand could be a source of risk if climate change impacts or socio-natural disasters compromise its integration. These issues should be considered in its current process to define the Local Energy Strategy to increase the city's response and adaptive capacity and enhance the energy transition process. Distributed photovoltaic potential of this city is higher compared to national average, and the inclusion of PED concept into local policies could increase its autonomy and redundancy.
Los Andes has a similar demographic profile to Arica, but the former's population size and poverty incidence (both income and multidimensional) are smaller. As only 49% of its housing stock was built before the year 2000, Los Andes could be an example of recent developed cities in Chile under new dwelling regulation. There are large and diverse electricity generators near this city. Its service interruptions index is the smaller of these three cities, and its resilience capacity looks well-suited to confront climate change and socio-natural disaster impacts. However, this condition is explained as other mid-size cities, and large metropolises (such as Valparaíso and Santiago) are located near and depend on the same generation, hence its capacity should be planned to integrate all of this growing cities. On the other hand, its dependency on fossil fuel-based electricity generation could endanger this capacity as a major transformation is required to meet NDC Chilean compromises on GHG emissions (Gobierno de Chile, 2020). In this regard, PED could become a transformative guideline to benefit from the photovoltaic potential and increase distributed energy importance.
Coyhaique is the smaller and is the one who faces the coldest weather conditions of these three cities. Although the presence of age vulnerable population (elderly and children) and income poverty is smaller, probably due to the same harsh weather condition, the multidimensional poverty incidence could be symptomatic of a different kind of sensitivity. The large thermal energy demand and low quality of buildings could explain the massive use of firewood as a heating energy source (Red de Pobreza Energética, 2019) and the high PM2.5 concentrations in this city. On the other hand, the autonomous condition of its electricity generation and high service interruption could express the energy system's difficulties in adapting to its harsh environment. This antecedent could endanger economic development and energy transition trajectories as user untrust of the electrical system causes rejection of electric-based heating technologies.
These profiles translate into significantly diverse conditions of sensitivity and resiliency of the three cities to various possible disturbances that may arise in the future and endanger the provision of energy services. Also, a territorial assessment based on TEV concept can better inform PEDs policies in multiples aspects. First, it can help identify crucial sensitivity conditions to avoid policies that may inadvertently increase inequality and causes negative impacts on population wellbeing. For example, in the case of Arica and Los Andes the presence of children and elderly population and its vulnerability to heatwaves. requires specific planning to protect these groups. Second, it may contribute in describing preexisting gaps (technical, economical, informational, etc.) necessary to face before beginning a process of PED implementation, For example, the dependence on private off-grid self-generation in Coyhaique to satisfy the local energy demand need to be addressed in early stages of PED projects. Third, it may offer a baseline assessment of response and adaptive capacity (resilience) of the energy system and possible indicators of the positive impact of PED model, for example, to increase the learning capacity of a territory implementing better data acquisition on consumers, ideally on real-time, to improve decision making and efficiency of energy system management. This territorial scale assessment, hence, could enhance the integrated view of energy system present in PEDs literature by describing the preexisting vulnerability conditions.
Conclusion
Adopting a territorial, system-based outlook on energy poverty and energy transition is a necessary step to advance a more integral understanding of energy security, one which recognizes the contextual conditions that influence both energy uses and the processes of sociotechnical provision, and that acknowledges the potential impacts of diverse hazards in maintaining equitable access to energy for all. A deeper attention to the territorial and spatial conditions that determine the range of energy and technological alternatives available in a territory, and their vulnerability to hazards and stresses, is necessary to advance toward a just, sustainable and resilient energy transition to a decarbonized economy. Within cities, in particular, this may support a more contextualized and spatially-aware approach to the integration between urban planning and energy development in general, and to the deployment of Positive Energy Districs and PENs policies in particular.
To contribute to this challenge, the article proposed a framework based on the concept of TEV, defined as the propensity of a territory to not guarantee equitable access—in quantity and quality—to resilient energy services that allow the sustainable human and economic development of its population. In particular, the paper proposed and illustrated by a case study of three Chilean cities crucial sensitivity and resilience indicators to assess preexisting gaps in energy access and resilience of energy services within urban spaces.
Since most of the factors observed relate to the daily provision of energy services, it can also work as a proxy of present capabilities to satisfy energy needs. As such, it is a very useful complement to traditional energy poverty analysis, based on indicators of energy affordability, access, or quality (Urquiza et al., 2019). While energy poverty focuses on expressing energy deprivation, TEV describes the underlying systemic conditions which may explain or anticipate such deprivation.
In terms of a just transition to sustainable cities based on PED guidelines, the contribution of our proposed approach is two-fold: first, in visualizing structural inequalities relating to energy deprivation, it contributes to making a stronger case for the need for a deep transformation of the energy regime: a transformation motivated not only by the need to decarbonize the energy matrix but also by the imperative of ensuring access to affordable, reliable, and sustainable energy for all, as implied in sustainable development goals (United Nations, 2015). Second, it pushes to reflect both on the possible dangers which may befall the transition effort—potentially hampering its results—and upon the risk that the transition itself can have on energy deprivation: as shown by previous research (Reyes et al., 2015; Bouzarovski and Tirado Herrero, 2017b; Frantál and Nováková, 2019; Urquiza et al., 2019; van der Wiel et al., 2019; O'Sullivan et al., 2020). If not properly planned, transitions may end up worsening—instead of alleviate—existing conditions of inequality, particularly among the most sensitive groups and sectors, and/or in territories showing the lower resiliency to change.
Moreover, because it takes the territory as its fundamental unit of analysis, the framework can provide an outlook of the differential distribution of energy deprivation across different territories. Therefore, it relates both to strands of literature that have attempted to identify climate and geographical drivers of energy poverty (Katsoulakos and Kaliampakos, 2016; Besagni and Borgarello, 2019; Ioannidis et al., 2019) and those striving to spatialize energy deprivation (Bouzarovski and Simcock, 2017; Bouzarovski et al., 2017; Robinson et al., 2019). Hence, it offers a strong analytical platform to deepen our understanding of different manifestations of energy deprivation that may occur outside the household boundary (Wolf et al., 2016; Mattioli et al., 2019). Along these lines, it is our contention that the TEV approach offers a powerful and flexible platform to advance toward a better understanding of forms of energy deprivation occurring outside the household (Mattioli et al., 2019). Future studies may extend the work presented in this paper by complementing the framework with indicators explicitly tackling technological, economical, or socio-cultural barriers to a full and equitable access to high-quality energy services within educational, health-related, and other social and productive uses, as suggested by Urquiza and Billi (2020). Likewise, an interesting future avenue of research may involve combining traditional energy poverty analysis and the TEV framework, exploiting the two approaches' complementarity while contrasting the descriptions of the energy deprivation they provide.
Before concluding, a warning must be raised about information requirements: the TEV approach is way more demanding in terms of data concerning traditional energy poverty indicators. We do not consider this a drawback, however. While in our case studies, we could only apply a fraction of the variables identified in our framework, the results were highly informative concerning differential patterns of sensitivity and resilience across the examined cities. On the other hand, outlining an ambitious yet operable analytical framework may signal the need to develop more data to provide a more complete picture on territorial energy processes and their impacts on energy deprivation, a requirement already pointed out by existing literature on energy poverty spatialization (Bouzarovski et al., 2017).
Data Availability Statement
Publicly available datasets were analyzed in this study. This data can be found here: Ministerio del Medio Ambiente (2020). Proyecto Atlas Riesgos Climáticos (ARClim). https://arclim.mma.gob.cl Ministerio de Desarrollo Social (2015). Encuesta de Caracterización Socioeconómica (CASEN). http://observatorio.ministeriodesarrollosocial.gob.cl/ Ministerio de Desarrollo Social (2017). Encuesta de Caracterización Socioeconómica Nacional (CASEN). http://observatorio.ministeriodesarrollosocial.gob.cl/ Comisión Nacional de Energía (2020). Energía Abierta. http://energiaabierta.cl/ Instituto Nacional de Estadísticas (2018). Censo de Población y Vivienda 2017. https://www.ine.cl/estadisticas/sociales/censos-de-poblacion-y-vivienda.
Author Contributions
RC, CA, MB, and AU: conceptualization, methodology, investigation, writing—original draft, supervision, and funding acquisition. MF: conceptualization, methodology, investigation, and writing—original draft. NA and JN: methodology, investigation, and writing—review and editing. All authors contributed to the article and approved the submitted version.
Funding
This research was funded by the Ministry of Science and Technology of Chile through FONDECYT N°11180824, ANID. RC and CA were funded by the National Ph.D. Scholarship Program 2020 (RC by scholarship #21200800 and CA by #21200803), Research and Development National Agency (ANID), Ministry of Science and Technology. MB, JN, and NA were funded by the Center for Climate and Resilience Research (FONDAP #1511009).
Conflict of Interest
The authors declare that the research was conducted in the absence of any commercial or financial relationships that could be construed as a potential conflict of interest.
Acknowledgments
The authors would like to thank the Ministry of Science and Technology of Chile, Center for Climate and Resilience Research (FONDAP #1511009) and the Energy Poverty Network, leadered by University of Chile, for institutional support to this research.
References
Acosta, C., Ortega, M., Bunsen, T., Koirala, B. P., and Ghorbani, A. (2018). Facilitating energy transition through energy commons: an application of socio-ecological systems framework for integrated community energy systems. Sustainability 10:366. doi: 10.3390/su10020366
Ala-Juusela, M., Crosbie, T., and Hukkalainen, M. (2016). Defining and operationalising the concept of an energy positive neighbourhood. Energy Conver. Manage. 125, 133–140. doi: 10.1016/j.enconman.2016.05.052
Amigo, C. (2019). Cultura y Vulnerabilidad Energética Territorial ulnerProblema de la Contaminación en Coyhaique. Universidad de Chile.
Arnold-Cathalifaud, M. (2008). las organizaciones desde la teoría de los sistemas sociopoiéticos. Cint. Moeb. 32, 90–108. doi: 10.4067/S0717-554X2008000200002
Bai, X. (2018). Six research priorities for cities and climate change. Nat. Clim. Chang. 555, 23–25. doi: 10.1038/d41586-018-02409-z
Baptista, I. (2018). Space and energy transitions in sub-Saharan Africa: understated historical connections. Energy Res. Soc. Sci. 36, 30–35. doi: 10.1016/j.erss.2017.09.029
Belaïd, F. (2018). Exposure and risk to fuel poverty in France: examining the extent of the fuel precariousness and its salient determinants. Energy Pol. 114, 189–200. doi: 10.1016/j.enpol.2017.12.005
Berkes, F., Colding, J., and Folke, C. (2003). Navigating social-ecological systems: building resilience for complexity and change. Biol. Conserv. 119:581. doi: 10.1016/j.biocon.2004.01.010
Besagni, G., and Borgarello, M. (2019). The socio-demographic and geographical dimensions of fuel poverty in Italy. Energy Res. Soc. Sci. 49, 192–203. doi: 10.1016/J.ERSS.2018.11.007
Bhatia, M., and Angelou, N. (2015). Beyond Connections. Energy Access Redefined ESMAP Technical Report.
Biggs, R., Schlüter, M., Biggs, D., Bohensky, E. L., BurnSilver, S., Cundill, G., et al. (2012). Toward principles for enhancing the resilience of ecosystem services. Annu. Rev. Environ. Resour. 37, 421–448. doi: 10.1146/annurev-environ-051211-123836
Biggs, R., Schlüter, M., and Schoon, M. L. (2015). Principles for Building Resilience: Sustaining Ecosystem Services in Social-Ecological Systems. Cambridge: Cambridge University Press. doi: 10.1017/CBO9781316014240
Billi, M., Amigo, C., Calvo, R., and Urquiza, A. (2018). Economía de la pobreza energética ‘por qué y cómo garantizar un acceso universal y equitativo a la energía? Econ. Polít. 5, 35–65. doi: 10.15691/07194714.2018.006
Binder, C. R., Mühlemeier, S., and Wyss, R. (2017). An indicator-based approach for analyzing the resilience of transitions for energy regions. Part I: theoretical and conceptual considerations. Energies 10, 1–18. doi: 10.3390/en10010036
Bouzarovski, S., Herrero, S. T., Petrova, S., Frankowski, J., Matoušek, R., and Maltby, T. (2017). Multiple transformations: theorizing energy vulnerability as a socio-spatial phenomenon. Geograf. Ann. B Hum. Geograp. 99, 20–41. doi: 10.1080/04353684.2016.1276733
Bouzarovski, S., and Simcock, N. (2017). Spatializing energy justice. Energy Policy 107, 640–648. doi: 10.1016/j.enpol.2017.03.064
Bouzarovski, S., and Thomson, H. (2018). Energy vulnerability in the grain of the city: toward neighborhood typologies of material deprivation. Ann. Am. Assoc. Geograph. 108, 695–717. doi: 10.1080/24694452.2017.1373624
Bouzarovski, S., and Tirado Herrero, S. (2017a). Geographies of injustice: the socio-spatial determinants of energy poverty in Poland, the Czech Republic and Hungary. Post Commun. Econ. 29, 27–50. doi: 10.1080/14631377.2016.1242257
Bouzarovski, S., and Tirado Herrero, S. (2017b). The energy divide: integrating energy transitions, regional inequalities and poverty trends in the European Union. Eur. Urban Reg. Stud. 24, 69–86. doi: 10.1177/0969776415596449
Bouzarovski, S., Tirado Herrero, S., Petrova, S., and Ürge-Vorsatz, D. (2015). Unpacking the spaces and politics of energy poverty: path-dependencies, deprivation and fuel switching in post-communist Hungary. Local Environ. 21, 1151–1170. doi: 10.1080/13549839.2015.1075480
Brondizio, E. S., O'brien, K., Bai, X., Biermann, F., Steffen, W., Berkhout, F., et al. (2016). Re-conceptualizing the Anthropocene: a call for collaboration. Glob. Environ. Change 39, 318–327. doi: 10.1016/j.gloenvcha.2016.02.006
Burillo, D., Chester, M. V., Pincetl, S., and Fournier, E. (2019). Electricity infrastructure vulnerabilities due to long-term growth and extreme heat from climate change in Los Angeles County. Energy Policy 128, 943–953. doi: 10.1016/j.enpol.2018.12.053
Centro de Ciencia del Clima y la Resiliencia (CR)2. (2018). Marco de Evaluación de la Vulnerabilidad. Available online at: http://www.cr2.cl/wp-content/uploads/2019/06/MARCO_VULNERABILIDAD_CR2_2018.pdf (accessed May 30, 2021).
Centro de Energia (2019). Estudio de Actualización y Complementación de Herramientas de Prospectivas de Largo Plazo Asociadas a la Demanda Energética.
Climate Watch (2019). Global Historic Emissions. Available online at: https://www.climatewatchdata.org/ghg-emissions?breakBy=sectorandchartType=percentageandend_year=2016andstart_year=1990 (accessed May 30, 2021).
Corporación de Desarrollo Tecnológico (2010). Estudio de Usos Finales y Curva de Oferta de la Conservación de la Energía en el Sector Residencial. 404.
Corporación de Desarrollo Tecnológico (2015). Medición del Consumo Nacional de Leña y Otros Combustibles Sólidos Derivados de la Madera.
Corporación de Desarrollo Tecnológico (2019). Informe Final Uso de la Energía Hogares Chile 2018. In Diciembre 2019.
Cumming, G. S. (2011). Spatial Resilience in Social-Ecological Systems. Dordrecht: Springer Netherlands. doi: 10.1007/978-94-007-0307-0
De Gregorio, A., and Jansen, L. (2005). Land Cover Classification System Classification Concepts and User Manual Software version (2).
Debizet, G., Tabourdeau, A., Gauthier, C., and Menanteau, P. (2016). Spatial processes in urban energy transitions: considering an assemblage of Socio-Energetic Nodes. J. Clean. Prod. 134, 330–341. doi: 10.1016/j.jclepro.2016.02.140
Desouza, K. C., and Flanery, T. H. (2013). Designing, planning, and managing resilient cities: a conceptual framework. Cities 35, 89–99. doi: 10.1016/j.cities.2013.06.003
Dodson, J., and Sipe, N. (2006). “Suburban shocks: assessing locational vulnerability to rising household fuel and mortgage interest costs.” in 29th Australasian Transport Research Forum, ATRF 06 (Gold Coast, QLD: Queensland Transport).
Dodson, J., and Sipe, N. (2008). Unsettling Suburbia : The New Landscape of Oil and Mortgage Vulnerability in Australian Cities. Urban Research Program (GU).
Dugoua, E., Liu, R., and Urpelainen, J. (2017). Geographic and socio-economic barriers to rural electrification: new evidence from Indian villages. Energy Policy 106, 278–287. doi: 10.1016/j.enpol.2017.03.048
Falk, J., Gaffney, O., Falk, J., Gaffney, O., Bhowmik, A. K., Bergmark, P., et al. (2019). Exponential Roadmap 1.5. Future Earth.
Folke, C. (2006). Resilience: the emergence of a perspective for social-ecological systems analyses. Glob. Environ. Change 16, 253-267. doi: 10.1016/j.gloenvcha.2006.04.002
Folke, C., Hahn, T., Olsson, P., and Norberg, J. (2005). Adaptive governance of social-ecological systems. Annu. Rev. Environ. Resour. 30, 441–473. doi: 10.1146/annurev.energy.30.050504.144511
Frantál, B., and Nováková, E. (2019). On the spatial differentiation of energy transitions: exploring determinants of uneven wind energy developments in the Czech Republic. Morav. Geograph. Rep. 27, 79–91. doi: 10.2478/mgr-2019-0007
García-Ochoa, R., and Graizbord, B. (2016). Privation of energy services in Mexican households: an alternative measure of energy poverty. Energy Res. Soc. Sci. 18, 36–49. doi: 10.1016/j.erss.2016.04.014
Geels, F. W. (2002). Technological transitions as evolutionary reconfiguration processes: a multi-level perspective and a case-study. Res. Policy 31, 1257–1274. doi: 10.1016/S0048-7333(02)00062-8
Geels, F. W. (2004). From sectoral systems of innovation to socio-technical systems: insights about dynamics and change from sociology and institutional theory. Res. Policy 33, 897–920. doi: 10.1016/j.respol.2004.01.015
Geels, F. W. (2011). Ontologies, socio-technical transitions (to sustainability), and the multi-level perspective. Res. Policy 39, 495–510. doi: 10.1016/j.respol.2010.01.022
GIZ (2018). Evaluación de Riesgo Climático para la Adaptación basada en Ecosistemas Guía Para Planificadores y Practicantes.
Golubchikov, O., and O'Sullivan, K. (2020). Energy periphery: uneven development and the precarious geographies of low-carbon transition. Energy Build. 211:109818. doi: 10.1016/j.enbuild.2020.109818
Gunderson, L. H., and Holling, C. S. (2002). Panarchy. Understanding Transformations in Human and Natural Systems. Washington, DC: Island Press. doi: 10.4324/9781315715865
Herington, M., Lant, P., Smart, S., Greig, C., and van de Fliert, E. (2017). Defection, recruitment and social change in cooking practices: energy poverty through a social practice lens. Energy Res. Soc. Sci. 34, 272–280. doi: 10.1016/j.erss.2017.09.001
Hernández, H. J., Galleguillos, M., and Estades, C. (2016). Mapa de Cobertura de Suelos de Chile 2014: Descripción del Producto. Laboratorio GEP, Facultad de Ciencias Forestales y de la Conservación de la Naturaleza, Universidad de Chile.
Hills, J. (2012). Getting the Measure of Fuel Poverty: Final Report of the Fuel Poverty Review Report. Available online at: http://eprints.lse.ac.uk/43153 (accessed May 30, 2021).
Huang, P., and Broto, V. C. (2018). Interdependence between urban processes and energy Transitions: the dimensions of urban energy transitions (DUET) framework. Environ. Innov. Soc. Trans. 28, 35–45. doi: 10.1016/j.eist.2018.03.004
Huneeus, N., Urquiza, A., Gayó, E., Osses, M., Arriagada, R., Valdés, M., et al. (2020). El aire que respiramos: pasado, presente y futuro – Contaminación atmosférica por MP2,5 en el centro y sur de Chile. Zenedo 102:4498768. doi: 10.5281/zenodo.4498768
Imbert, I., Nogues, P., and Sevenet, M. (2016). Same but different: on the applicability of fuel poverty indicators across countries - insights from France. Energy Res. Soc. Sci. 15, 75–85. doi: 10.1016/j.erss.2016.03.002
International Energy Agency (2019). World Energy Balance Overview 2019. doi: 10.1017/CBO9781107415324.004
Ioannidis, A., Chalvatzis, K. J., Li, X., Notton, G., and Stephanides, P. (2019). The case for islands' energy vulnerability: electricity supply diversity in 44 global islands. Renew. Energy 143, 440–452. doi: 10.1016/j.renene.2019.04.155
IPCC (2012). Managing the Risks of Extreme Events and Disasters to Advance Climate Change Adaptation. doi: 10.1017/cbo9781139177245
IPCC (2019a). Global Warming of 1.5°C. An IPCC Special Report on the Impacts of Global Warming of 1.5°C Above Pre-Industrial Levels and Related Global Greenhouse Gas Emission Pathways, in the Context of Strengthening the Global Response to the Threat of Climate Change 53, Issue 9).
IPCC (2019b). Summary for policymakers. IPCC Special Report on the Ocean and Cryosphere in a Changing Climate. In IPCC Special Report on the Ocean and Cryosphere in a Changing Climate. http://www.ipcc.ch/publications_and_data/ar4/wg2/en/spm.html
Jimenez, R. (2017). Barriers to electrification in Latin America: income, location, and economic development. Energy Strat. Rev. 15, 9–18. doi: 10.1016/j.esr.2016.11.001
Kadykalo, A. N., López-Rodriguez, M. D., Ainscough, J., Droste, N., Ryu, H., Ávila-Flores, G., et al. (2019). Disentangling ‘ecosystem services' and ‘nature's contributions to people.' Ecosyst. People 15, 269–287. doi: 10.1080/26395916.2019.1669713
Katsoulakos, N. M., and Kaliampakos, D. C. (2016). Mountainous areas and decentralized energy planning: insights from Greece. Energy Policy 91, 174–188. doi: 10.1016/j.enpol.2016.01.007
Kerimray, A., De Miglio, R., Rojas-Solórzano, L. Ó., and Gallachóir, B. P. (2018). Causes of energy poverty in a cold and resource-rich country: evidence from Kazakhstan. Local Environ. 23, 178–197. doi: 10.1080/13549839.2017.1397613
Kumar, M. (2020). Non-universal nature of energy poverty: energy services, assessment of needs and consumption evidences from rural Himachal Pradesh. Energy Policy 138:111235. doi: 10.1016/j.enpol.2019.111235
Lindholm, O., Rehman, H. U., and Reda, F. (2021). Positioning positive energy districts in European cities. Buildings 11, 1–31. doi: 10.3390/buildings11010019
Lozano, L., Querikiol, E. M., Abundo, M. L. S., and Bellotindos, L. M. (2019). Techno-economic analysis of a cost-effective power generation system for off-grid island communities: a case study of Gilutongan Island, Cordova, Cebu, Philippines. Renew. Energy 140, 905–911. doi: 10.1016/j.renene.2019.03.124
Makki, A. A., and Mosly, I. (2020). Factors affecting public willingness to adopt renewable energy technologies: an exploratory analysis. Sustainability 12, 1–11. doi: 10.3390/su12030845
Mattioli, G., Philips, I., Anable, J., and Chatterton, T. (2019). Vulnerability to motor fuel price increases: socio-spatial patterns in England. J. Transport Geogr. 78, 98–114. doi: 10.1016/j.jtrangeo.2019.05.009
McCarthy, M. P., Best, M. J., and Betts, R. A. (2010). Climate change in cities due to global warming and urban effects. Geophys. Res. Lett. 37:L09705. doi: 10.1029/2010GL042845
McCauley, D., and Heffron, R. (2018). Just transition: integrating climate, energy and environmental justice. Energy Policy 119, 1–7. doi: 10.1016/j.enpol.2018.04.014
Middlemiss, L., and Gillard, R. (2015). Fuel poverty from the bottom-up: characterising household energy vulnerability through the lived experience of the fuel poor. Energy Res. Soc. Sci. 6, 146–154. doi: 10.1016/j.erss.2015.02.001
Ministerio de Desarrollo Social (2017). Encuesta de Caracterización Socioeconómica Nacional (CASEN).
Ministerio de Energía (2017a). Comienzan Rebajas de Cuentas de Luz en la Región de Aysén por Implementación de Ley de Equidad Tarifaria Residencial.
Molyneaux, L., Brown, C., Wagner, L., and Foster, J. (2016). Measuring resilience in energy systems: insights from a range of disciplines. Renew. Sustain. Energy Rev. 59, 1068–1079. doi: 10.1016/j.rser.2016.01.063
Molyneaux, L., Wagner, L., Froome, C., and Foster, J. (2012). Resilience and electricity systems: a comparative analysis. Energy Policy 47, 188–201. doi: 10.1016/j.enpol.2012.04.057
Monti, A., Pesch, D., Ellis, K., and Mancarella, P. (2016). Energy Positive Neighborhoods and Smart Energy Districts: Methods, Tools, and Experiences From the Field. London; San Diego, CA; Cambridge, MA; Oxford: Academic Press.
Moore, R. (2012). Definitions of fuel poverty: implications for policy. Energy Policy 49, 19–26. doi: 10.1016/j.enpol.2012.01.057
Moreno, A. G., Vélez, F., Alpagut, B., Hernández, P., and Montalvillo, C. S. (2021). How to achieve positive energy districts for sustainable cities: a proposed calculation methodology. Sustainability 13, 1–19. doi: 10.3390/su13020710
Munro, P. G., and Bartlett, A. (2019). Energy bricolage in Northern Uganda: rethinking energy geographies in Sub-Saharan Africa. Energy Res. Soc. Sci. 55, 71–81. doi: 10.1016/j.erss.2019.04.016
Nadimi, R., and Tokimatsu, K. (2018). Energy use analysis in the presence of quality of life, poverty, health, and carbon dioxide emissions. Energy 153, 671–684. doi: 10.1016/j.energy.2018.03.150
Nangini, C., Peregon, A., Ciais, P., Weddige, U., Vogel, F., Wang, J., et al. (2019). A global dataset of CO 2 emissions and ancillary data related to emissions for 343 cities. Sci. Data 6:180280. doi: 10.1038/sdata.2018.280
Nussbaumer, P., Bazilian, M., and Modi, V. (2012). Measuring energy poverty: focusing on what matters. Renew. Sustain. Energy Rev. 16, 231–243. doi: 10.1016/j.rser.2011.07.150
Nykvist, B. (2014). Does social learning lead to better natural resource management? A case study of the modern farming community of practice in Sweden. Soc. Nat. Resour. 27, 436-450. doi: 10.1080/08941920.2013.861562
O'Sullivan, K., Golubchikov, O., and Mehmood, A. (2020). Uneven energy transitions: understanding continued energy peripheralization in rural communities. Energy Policy 138:111288. doi: 10.1016/j.enpol.2020.111288
Papada, L., and Kaliampakos, D. (2016). Developing the energy profile of mountainous areas. Energy 107, 205–214. doi: 10.1016/j.energy.2016.04.011
Petrova, S. (2018). Encountering energy precarity: geographies of fuel poverty among young adults in the UK. Trans. Inst. Br. Geograph. 43, 17–30. doi: 10.1111/tran.12196
Pollard, S. L., Williams, K. N., O'Brien, C. J., Winiker, A., Puzzolo, E., Kephart, J. L., et al. (2018). An evaluation of the Fondo de Inclusión Social Energético program to promote access to liquefied petroleum gas in Peru. Energy Sustain. De. 46, 82–93. doi: 10.1016/j.esd.2018.06.001
Primc, K., Slabe-Erker, R., and Majcen, B. (2019). Constructing energy poverty profiles for an effective energy policy. Energy Policy 128, 727–734. doi: 10.1016/j.enpol.2019.01.059
Puzzolo, E., Pope, D., Stanistreet, D., Rehfuess, E. A., and Bruce, N. G. (2016). Clean fuels for resource-poor settings: a systematic review of barriers and enablers to adoption and sustained use. Environ. Res. 146, 218–234. doi: 10.1016/j.envres.2016.01.002
Recalde, M., Peralta, A., Oliveras, L., Tirado-Herrero, S., Borrell, C., Palència, L., et al. (2019). Structural energy poverty vulnerability and excess winter mortality in the European Union: exploring the association between structural determinants and health. Energy Policy 133:110869. doi: 10.1016/j.enpol.2019.07.005
Red de Pobreza Energética (2019). Acceso Equitativo a Energía de Calidad en Chile. Hacia un Indicador Territorializado y Tridimensional de Pobreza Energética.
Red de Pobreza Energética (2020). Vulnerabilidad Energética Territorial: Desigualdad Más Allá del Hogar.
Revista Electricidad (2019). Polémica por Medidores Inteligentes Escala y Diputados Ingresan Proyecto Para Que Usuarios No Asuman Costo.
Reyes, R., Nelson, H., Navarro, F., and Retes, C. (2015). The firewood dilemma: human health in a broader context of well-being in Chile. Energy Sustain. Dev. 28, 75–87. doi: 10.1016/j.esd.2015.07.005
Rip, A., and Kemp, R. (1988). “Technological change,” in Human Choices and Climate Change, Vol. 2, eds S. Rayner and E. L. Malone (Battelle: Columbus, OH), 327–399.
Robić, S., and Ančić, B. (2018). Exploring health impacts of living in energy poverty: case study Sisak-Moslavina County, Croatia. Energy Build. 169, 379–387. doi: 10.1016/j.enbuild.2018.03.080
Robinson, C., Bouzarovski, S., and Lindley, S. (2018). ‘Getting the measure of fuel poverty': the geography of fuel poverty indicators in England. Energy Res. Soc. Sci. 36, 79–93. doi: 10.1016/J.ERSS.2017.09.035
Robinson, C., Lindley, S., and Bouzarovski, S. (2019). The spatially varying components of vulnerability to energy poverty. Ann. Am. Assoc. Geograph. 109, 1188–1207. doi: 10.1080/24694452.2018.1562872
Rogelj, J., Shindell, D., Jiang, K., Fifita, S., Forster, P., Ginzburg, V., et al. (2018). “Mitigation Pathways Compatible with 1.5°C in the Context of Sustainable Development,” in Global Warming of 1.5°C. An IPCC Special Report on the Impacts of Global Warming of 1.5°C Above Pre-Industrial Levels and Related Global Greenhouse Gas Emission Pathway. IPCC Special Report Global Warming of 1.5 °C. p. 82.
Rutherford, J., and Coutard, O. (2014). Urban Energy Transitions: Places, Processes and Politics of Socio-Technical Change. : London: Sage Publications.
Salmond, J. A., Tadaki, M., Vardoulakis, S., Arbuthnott, K., Coutts, A., Demuzere, M., et al. (2016). Health and climate related ecosystem services provided by street trees in the urban environment. Environ. Health 15:S36. doi: 10.1186/s12940-016-0103-6
Sarricolea, P., Herrera-Ossandon, M., and Meseguer-Ruiz, Ó. (2017). Climatic regionalisation of continental Chile. J. Maps 13, 66–73. doi: 10.1080/17445647.2016.1259592
Scarpellini, S., Rivera-Torres, P., Suárez-Perales, I., and Aranda-Usón, A. (2015). Analysis of energy poverty intensity from the perspective of the regional administration: empirical evidence from households in southern Europe. Energy Policy 86, 729–738. doi: 10.1016/j.enpol.2015.08.009
Schueftan, A., and González, A. D. (2015). Proposals to enhance thermal efficiency programs and air pollution control in south-central Chile. Energy Policy 79, 48–57. doi: 10.1016/j.enpol.2015.01.008
Schueftan, A., Sommerhoff, J., and González, A. D. (2016). Firewood demand and energy policy in south-central Chile. Energy Sustain. Dev. 33, 26–35. doi: 10.1016/j.esd.2016.04.004
Smith, A., and Stirling, A. (2010). The politics of social-ecological resilience and sustainable socio-technical transitions. Ecol. Soc. 15:11. doi: 10.5751/ES-03218-150111
Sokołowski, J., Lewandowski, P., Kiełczewska, A., and Bouzarovski, S. (2020). A multidimensional index to measure energy poverty: the Polish case. Energy Sour. B Econ. Plann. Policy 15, 92–112. doi: 10.1080/15567249.2020.1742817
Stephenson, J., Barton, B., Carrington, G., Gnoth, D., Lawson, R., and Thorsnes, P. (2010). Energy cultures: a framework for understanding energy behaviours. Energy Policy 38, 6120–6129. doi: 10.1016/j.enpol.2010.05.069
Strauss, S., Rupp, S., and Love, T., (eds.). (2016). Cultures of Energy. Walnut Creek, CA: Left Coast Press.
Surroop, D., Raghoo, P., and Bundhoo, Z. M. A. (2018). Comparison of energy systems in Small Island Developing States. Util. Policy 54, 46–54. doi: 10.1016/j.jup.2018.07.006
Thomson, H., Snell, C., and Bouzarovski, S. (2017). Health, well-being and energy poverty in Europe: a comparative study of 32 European countries. Int. J. Environ. Res. Public Health 14:584. doi: 10.3390/ijerph14060584
Urquieta, M. A., Mariñez, C., and Jorquera, C. (2017). Territorio como medium: discusión sobre rendimientos analíticos para las observaciones de la complejidad socioespacial. Rev. MAD 37, 143–166). doi: 10.5354/0718-0527.2017.47279
Urquiza Gómez, A., and Cadenas, H. (2015). Sistemas socio-ecológicos: elementos teóricos y conceptuales para la discusión en torno a vulnerabilidad hídrica. L'Ordinaire des Amériques 218. doi: 10.4000/orda.1774
Urquiza, A., Amigo, C., Billi, M., Calvo, R., Labraña, J., Oyarzún, T., et al. (2019). Quality as a hidden dimension of energy poverty in middle- development countries. Literature review and case study from Chile. Energy Build. 204:109463. doi: 10.1016/j.enbuild.2019.109463
Urquiza, A., Amigo, C., Billi, M., and Espinosa, P. (2018). Participatory energy transitions as boundary objects: the case of Chile's Energía2050. Front. Energy Res. 6:134. doi: 10.3389/fenrg.2018.00134
Urquiza, A., and Billi, M. (2018). Water markets and social-ecological resilience to water stress in the context of climate change: an analysis of the Limarí basin, Chile [en prensa]. Environ. Dev. Sustain. 22, 1929–1951. doi: 10.1007/s10668-018-0271-3
Urquiza, A., and Billi, M. (2020). Seguridad Hídrica y Energética en América Latina y el Caribe: Hacia una Aproximación Territorial Para el Análisis de Brechas y Riesgos de la Población. Documentos de Proyectos, Estudios e Investigaciones.
van der Heijden, J. (2019). Studying urban climate governance: where to begin, what to look for, and how to make a meaningful contribution to scholarship and practice. Earth Syst. Govern. 1:100005. doi: 10.1016/j.esg.2019.100005
van der Wiel, K., Stoop, L. P., Van Zuijlen, B. R. H., Blackport, R., den Broek, M. A., and Selten, F. M. (2019). Meteorological conditions leading to extreme low variable renewable energy production and extreme high energy shortfall. Renew. Sustain. Energy Rev. 111, 261–275. doi: 10.1016/j.rser.2019.04.065
Walker, S., Labeodan, T., Maassen, W., and Zeiler, W. (2017). A review study of the current research on energy hub for energy positive neighborhoods. Energy Proc. 122, 727–732. doi: 10.1016/j.egypro.2017.07.387
Wolf, F., Surroop, D., Singh, A., and Leal, W. (2016). Energy access and security strategies in Small Island Developing States. Energy Policy 98, 663–673. doi: 10.1016/j.enpol.2016.04.020
Wolfram, M., Frantzeskaki, N., and Maschmeyer, S. (2017). Cities, systems and sustainability: status and perspectives of research on urban transformations. Current Opin. Environ. Sustain. 22, 18–25. doi: 10.1016/j.cosust.2017.01.014
Zhao, Y., Feng, D., Yu, L., Wang, X., Chen, Y., Bai, Y., et al. (2016). Detailed dynamic land cover mapping of Chile: accuracy improvement by integrating multi-temporal data. Remote Sens. Environ. 183, 170–185. doi: 10.1016/j.rse.2016.05.016
Keywords: energy poverty, cities, vulnerability, territorial, resilience, just energy transition
Citation: Calvo R, Amigo C, Billi M, Fleischmann M, Urquiza A, Álamos N and Navea J (2021) Territorial Energy Vulnerability Assessment to Enhance Just Energy Transition of Cities. Front. Sustain. Cities 3:635976. doi: 10.3389/frsc.2021.635976
Received: 30 November 2020; Accepted: 17 June 2021;
Published: 21 July 2021.
Edited by:
Cait Robinson, University of Liverpool, United KingdomReviewed by:
Sujit Sikder, Leibniz Institute for Ecological Urban and Regional Development (IOER), GermanySiddharth Sareen, University of Stavanger, Norway
Copyright © 2021 Calvo, Amigo, Billi, Fleischmann, Urquiza, Álamos and Navea. This is an open-access article distributed under the terms of the Creative Commons Attribution License (CC BY). The use, distribution or reproduction in other forums is permitted, provided the original author(s) and the copyright owner(s) are credited and that the original publication in this journal is cited, in accordance with accepted academic practice. No use, distribution or reproduction is permitted which does not comply with these terms.
*Correspondence: Rubén Calvo, cmNhbHZvQHVnLnVjaGlsZS5jbA==
†These authors have contributed equally to this work and share first authorship