- Department of Plant and Microbial Biology, Koshland Hall, University of California, Berkeley, Berkeley, CA, United States
Increasingly, architects are embracing “biomorphic urbanism,” a design ideology that takes inspiration from nature to develop more sustainable cities that reduce the environmental impact of urban life. At the moment, plants are incorporated into biomorphic urban designs for conservation or aesthetic reasons. Here, I argue the role of plants in building more sustainable cities can be augmented by integrating plant sciences, ecology, and urban design. I propose that we can develop synthetic Function-Specific Plant Systems (FSPSs) which harness the genetic and metabolic diversity of plants to perform specific services that benefit society and the environment as a whole. FSPSs can contribute to three broad categories of urban life: Urban Landscape and Infrastructure; Biodiversity and the Environment; and Human Health. Across the three categories, FSPSs can be designed to provide nine key services: flood control, soil stabilization, fire control, climate control, water treatment, habitat for endangered flora and fauna, pest control, air purification, and modulation of human immune systems. The plants included in each FSPS are based on several considerations, including (1) functional traits, (2) biogeography, and (3) cultural concerns. In the future, synthetic biology could improve, expand and diversify these services. This approach harnesses plant biodiversity to transform urban spaces while meeting key UN Sustainable Development Goals.
Greening Future Cities
For millennia, we have imagined what future societies will look like, from Thomas More's Utopia and Aldous Huxley's Brave New World to the artists of the Italian futurismo movement and popular films like Ridley Scott's Blade Runner. Such ideas have also entered the architectural world, most notably with National Geographic's The Cities issue (National Geographic, 2019a). This issue depicts what our cities could look like: sustainable, resilient, and beautifully designed. Central to future cities thinking is the concept of biomorphic urbanism: the idea that the natural world can inspire how we design the spaces we inhabit and how our cities function (Beatley and Newman, 2013; Kindel, 2019; Pedersen Zari, 2019).
This push toward sustainable urbanism is sorely needed. Urban populations are set to expand from 3 billion people as of 2000 to 6.4 billion by 2050; areas of greatest growth include sub-Saharan Africa and South and Central Asia (Figure 1; Angel et al., 2011). Such influxes of humans into urban spaces will create new stresses on transportation infrastructure, housing, water, and use of other natural and non-renewable resources (McPhearson et al., 2016). This shift in human population dynamics will also impact the natural world. For example, wildlife migration patterns, behavior, and biological processes (like mating and reproduction) will shift with loss of habitats and perturbation of food webs (Hilty et al., 2006; Su et al., 2011). Urban landcover in biodiversity hotspots is projected to expand by 200% by 2030, threatening the continuity of and ecosystem services provided by major global biodiversity hotspots, such as the Eastern Afromontane and Guinean Forests of Western Africa (Seto et al., 2012).
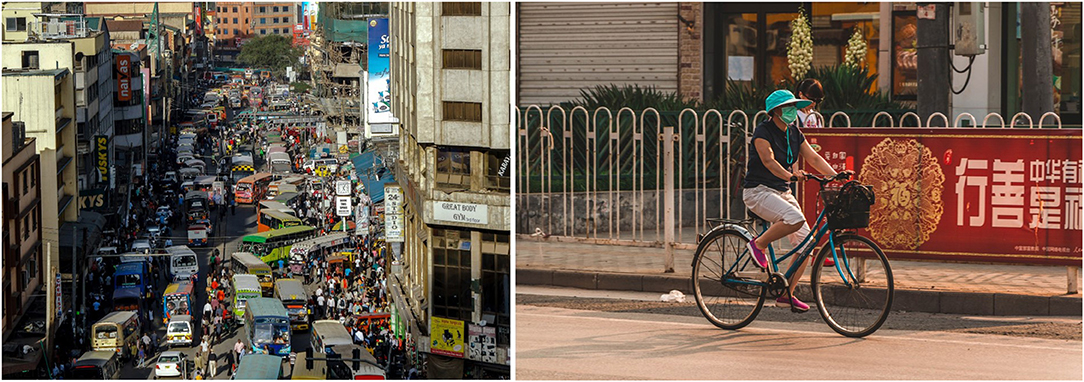
Figure 1. Urban populations are projected to double by 2030. How can we make current and future cities more sustainable while also maintaining and preserving biological diversity and ecosystem services?
Integrating biomorphism into the design of new cities or improvement of old ones could mitigate the effects of anthropogenic urbanism while also providing new prototypes of how humans and nature can coexist in a more balanced and fair manner. Biomorphic urbanism could also help mitigate the effects of climate change on urban centers, including flooding, storms, sea-level rise, forest fires and drought (Leichenko, 2011; Riffat et al., 2016). Examples of realized future cities include Seagull Island (China), Singapore, Silk City (Kuwait), and the Kitakyusho EcoTown project (Japan) (McDonnell and MacGregor-Fors, 2016; Riffat et al., 2016; National Geographic, 2019b). More idealized, futuristic urban spaces include Ocean Spirals (Shimizu Corporation), Lilypad City (Vincent Callebaut), and the Venus Project (Jacque Fresco) (McDonnell and MacGregor-Fors, 2016).
Biomorphic urban designers have developed well-thought out and creative architectural designs and urban plans. However, I believe the application of biomorphism to urban planning could be expanded to include the role of plants in terms of ecosystem service provisioning and human well-being. To date, sustainability architects think about vegetation in two main ways: (1) as a resource to cordon off or restore and (2) as an aesthetic medium. Here, I argue a third possibility: that we create entirely new buffer landscapes designed to perform specific functions that improve the sustainability of urban living, contribute to biodiversity conservation, and use resources more sustainably. I contend that we can use plants in a very directed manner to perform specific urban functions on different timescales (temporary vs. long-term). These specific urban functions relate to how cities are designed, how humans inhabit them, and how the natural environment and human activities interact. I argue these designed landscapes, termed loosely Function-Specific Plant Systems (FSPSs), can provide nine key services which could improve the sustainability of future cities. Moreover, I argue synthetic biology could expand these roles in the future.
Putting Plants to Work
Designed Function-Specific Plant Systems (FSPSs) provide services that fall into three categories: Urban Landscape and Infrastructure; Biodiversity and the Environment; and Human Health. Table 1 lists the services under each category, the biological/ecological rational behind each service, examples of projects that provide data on the benefits of each service, and references which support the science behind each service. Many of these services provided by FSPSs align with the UN 2030 Sustainable Development Goals, namely SDG 6 Clean Water & Sanitation, SDG 11 Sustainable Cities and Communities, SDG 14 Life Below Water, and SDG 15 Life on Land (United Nations, 2018). Under the category of Urban Landscape and Infrastructure, FSPSs could be used to reduce flooding in coastal urban areas, stabilize shorelines from erosion, and reduce the impact of wildfires. The latter may become particularly important in areas of the Western US and Australia, where wild fire events are increasing annually. Under the category of Biodiversity and the Environment, FSPSs could be used to remove harmful industrial chemicals from waterways (e.g., rivers, lakes, storm run-off) while also providing habitats for native species. Finally, under the category of Human Health, FSPSs could be used to control pests like mosquitos, remove harmful pollutants (e.g., benzene) from urban environments, and potentially, alter the volatilomes of urban landscapes to promote human health and well-being.
The selection process for which plants to include in each FSPS should be guided by three factors: (1) functional traits, (2) biogeography, and (3) cultural concerns. Functional traits are measurable, documented physiological (e.g., root length/architecture) or metabolic (e.g., enzymes) features of a given plant that contribute to the performance of a given service (e.g., water purification). Biogeography, defined here as including climate, elevation, terrain, and biodiversity, should also be taken into account to ensure the plants selected and used in FSPSs are local (where possible) and can survive under the environmental conditions where the FSPS is located. Finally, cultural concerns may also guide plant selection. For example, plants considered invasive should not be used. Where possible, plants with specific biocultural value (e.g., rare, indigenous, or medicinal plants) could also be used as a way to integrate these synthetic landscapes into the wider fabric of society. Ultimately, this means that each FSPS will be highly specialized, rooted to local geography, climate, biodiversity, and community.
The FSPSs described above could be incorporated into pre-existing and new urban designs in four ways. First, certain FSPSs could be used to develop “buffer zones” that surround certain urban features. For example, stands of cypress (Cupressus sempervirens) could be established around fire-prone areas (Della Rocca et al., 2014) while eucalyptus (Tyagi, 2016) could be planted around residential areas in malaria-prone regions. Second, plants and trees with specific functional traits could be incorporated into urban landscaping. For example, urban landscapers could integrate plane trees, ivy and ferns along urban walkways and around transit hubs to reduce the amount of small particulate matter and volatile organic compounds from automobiles (Claudio, 2011). Similarly, when designing green walls, landscape architects could select plants known for sequestering and/or degrading predominant air pollutants, such as BTEX, solvents, pesticides, adhesives, coatings and cleaning agents (McDonald et al., 2018). Third, FSPSs could be incorporated into green architecture. Architects routinely incorporate plants into building designs for aesthetic reasons, yet being more conscious about what plants and trees are used could increase the impact of these features on human health and the environment. Research on volatilomes is in its infancy (Giannoukos et al., 2019), but several studies suggest that the compounds produced by plants and bacteria can impact human health and well-being (Maffei et al., 2011; Ljunggren et al., 2019; Liddicoat et al., 2020). These VOCs (such as terpenoids) are highly lipophilic and can pass the blood-brain barrier, causing neurophysiological and behavioral changes in mammals (such as reduced anxiety and improved memory), while also reducing risk/duration of infections and other illnesses (Maffei et al., 2011). Potentially, selection of plants in urban residential units can be guided by these principles. Finally, FSPSs could be integrated into urban art installations (Sachdev, 2019). These installations could play with form and function, culture and identity, conservation and education, thereby pioneering new ways of integrating plants into urban landscapes.
Advances in plant synthetic biology could also expand these roles (French, 2019; Küken and Nikoloski, 2019; Mortimer, 2019). Examples include augmenting the native abilities of plants to perform specific functions [such as biotransformation of industrial toxins (Doty et al., 2000) or production of insect-repellant volatiles (Tyagi, 2016)] by over-expressing key enzymes or by altering plant metabolomes; developing new raw materials on-site that meet the needs of urban construction and consumption (Sakamoto et al., 2018); locally producing natural plant-derived colorants for urban textile and food industries to replace toxic chemicals (Appelhagen et al., 2018); and genetically engineering plants to use urban resources (water, nitrogen etc.) more sustainably to produce drugs/food for urban populations (López-Arredondo et al., 2015).
Challenges to Implementation
Implementing FSPSs face three key challenges: sustainable design, cost effectiveness, and environmental impact.
The design of FSPSs is key to extracting the greatest benefits from the plant systems while minimizing their carbon footprint. Design includes everything from sourcing raw materials to intended use and end of life. Whether new objects, materials, or buildings, design accounts for an estimated 80% of their environmental impact (Childers et al., 2015). The materials used in their construction should be organic where possible. A key charge against living walls and green roofs is that the materials used in their construction are derived from fossil-fuels (Bianchini and Hewage, 2012), minimizing the carbon they offset (but not other services, such as reduced energy use, air purification, and removing pollutants from storm water drainage) (Pulselli et al., 2014). One possibility would be to use bioplastics, cellulose made from bacteria, or mycelium-based materials from fungi (Munoz and Riley, 2008; Florea et al., 2016; Sagnelli et al., 2016; Abhijith et al., 2018).
Another critical aspect of design is which plants to use. On the one hand, native plants are ideal because they are well-suited to local environments. However, non-native plants have a key advantage, namely increased capacity to perform a given service (e.g., air purification). Whether native or non-native plants are used, central to the design of FSPSs is the focus on principles instead of specific plants. For example, plants sown onto coastlines to control soil erosion may change over the years based on changing local environmental conditions (e.g., salinity, temperature, etc.). GIS could play a key role in the design of FSPSs in the future. Using GIS, we can geospatially map climatological, biological, chemical, and plant functional trait data to model and predict how FSPSs might respond in multiple circumstances (Grêt-Regamey et al., 2013; Jackson et al., 2013; Nemec and Raudsepp-Hearne, 2013; Masson et al., 2014). Employing key design concepts from ecological engineering—such as self-design and systems theory—could also be used to design more complex, multi-species FSPSs that are self-sustaining, resilient, generate zero waste, recycle nutrients, and require minimal management (Bergen et al., 2001; Costanza, 2012; Mitsch, 2012).
The intended end-user of a FSPS should also be taken into account. Key variables to consider include lifespan and location. For example, a green roof might be designed to last 10 years while a water purification system would be in use on a much longer time-frame (e.g., 20–50 years). Location and end-user (e.g., domestic, civic, industrial) will also determine the size, composition, and design of FSPSs. For example, plant-based water purification systems for single-family households will take a much different form from those used to purify water from industrial sites.
Management of FSPSs must also be cost effective. Two methods currently used to green cities-living walls and green roofs-provide multiple amenities (e.g., reduced energy use from heating/cooling; improved air quality; storm drainage) yet some would argue that the design, cost and maintenance of these structures outweighs their benefits. Both features rely on the use of non-degradable polymers for construction. The construction and annual maintenance of these structures can also be up to four times more expensive than alternatives (such as using attic floor insulation or simply planting more trees) (Yok Tan and Sia, 2005; Michael et al., 2010). At all times, Life Cycle Assessments (LCA) can be used to determine the economic cost and environmental benefit of each FSPS, with the design modified accordingly for maximum benefit and least cost (Guinée et al., 2011). One challenge with using LCAs as a benchmark, however, is our current inability to put a monetary value on the services many plants provide (e.g., air purification, modulation of human immune system).
The short- and long-term effects of synthetic ecosystems FSPSs on existing ecosystems must also be monitored and mitigated where needed. For example, would planting cypress fire buffers in Bay-area fire-prone cities lead to a decline in native bird species? Small scale field trials, long term data collection, and monitoring (Tilman, 1989) can be used to quantify changes in ecosystem services/provisioning and could be used to determine the risks and benefits of a FSPS in a given geographic location.
Integrating Plants into Urban Design
We need to think about how plants will fit into future cities models. Greater collaboration between plant scientists, ecologists, architects and engineers is needed to understand how we can translate knowledge of ecological ideas/processes into products/services for future urban societies. This collaboration is also needed to ensure plants and the functions they perform can be scaled up to city-level and that their impact (benefit) outweighs their cost. As such, field trials will become increasingly important to test whether principles of ecological engineering hold up under real conditions before expanding to entire urban landscapes. How these units are designed will be critical to how they function and how they are experienced. Working with artists and designers will also allow a re-imagining of urban landscapes which can push the boundaries of how form, function and aesthetics can go together.
Former industrial areas are good places to test out some of these designs, as these landscapes are currently under re-design and could benefit from some of the services (e.g., water purification) listed above. The FSPSs described above could also be incorporated into urban development in rapidly expanding low-economic income countries, where city re-design is underway and funding is readily available. Countries with the greatest area of urban land cover (5% as of 2000)—including Bahrain, Belgium, Netherlands, the UK, Italy and Germany (Angel et al., 2011)—could also stand to benefit from supporting research on the development and local application of FSPSs. To integrate FSPSs into mega cities (where urban landscapes and infrastructure are already largely “mapped out”) however will require a two-fold effort. First, there must be local support from citizens inhabiting these spaces (e.g., activists, businesses). Second, there must be support (legal, policy and financial) from urban governors, city planners, and civil engineers to enact these goals. Perhaps the easiest way to incorporate FSPSs into mega cities will be in terms of new developments and/or renovations of old buildings and city spaces.
While not a silver bullet, a more nuanced incorporation of plants into urban design will bring us one step closer to mitigating the effects of urbanism on the natural environment and human health in the near future.
Data Availability Statement
The original contributions presented in the study are included in the article/supplementary material, further inquiries can be directed to the corresponding author/s.
Author Contributions
KF conceived and wrote the perspective piece.
Conflict of Interest
The author declares that the research was conducted in the absence of any commercial or financial relationships that could be construed as a potential conflict of interest.
References
Abhijith, R., Ashok, A., and Rejeesh, C. R. (2018). Sustainable packaging applications from mycelium to substitute polystyrene: a review. Mater. Today Proc. 5, 2139–2145. doi: 10.1016/j.matpr.2017.09.211
Angel, S., Parent, J., Civco, D. L., Blei, A., and Potere, D. (2011). The dimensions of global urban expansion: estimates and projections for all countries, 2000–2050. Prog. Plan. 75, 53–107. doi: 10.1016/j.progress.2011.04.001
Appelhagen, I., Wulff-Vester, A.K., Wendell, M., Hvoslef-Eide, A.-K., Russell, J., Oertel, A., et al. (2018). Colour bio-factories: towards scale-up production of anthocyanins in plant cell cultures. Metab. Eng. 48, 218–232. doi: 10.1016/j.ymben.2018.06.004
Beatley, T., and Newman, P. (2013). Biophilic cities are sustainable, resilient cities. Sustainability 5, 3328–3345. doi: 10.3390/su5083328
Bergen, S. D., Bolton, S. M. L., and Fridley, J. (2001). Design principles for ecological engineering. Ecol. Eng. 18, 201–210. doi: 10.1016/S0925-8574(01)00078-7
Bianchini, F., and Hewage, K. (2012). How “green” are the green roofs? Lifecycle analysis of green roof materials Build Environ. 48, 57–65. doi: 10.1016/j.buildenv.2011.08.019
Bowers, W. S., Sener, B., Evans, P. H., Bingol, F., and Erdogan, I. (1995). Activity of Turkish Medicinal Plants Against Mosquitoes Aedes aegypti and Anopheles gambiae. Int. J. Trop. Insect Sci. 16, 339–342. doi: 10.1017/S1742758400017379
Brydon, J., Roa, M. C., Brown, S. J., and Schreier, H. (2006). “Integrating wetlands into watershed management: effectiveness of constructed wetlands to reduce impacts from urban stormwater,” in Environmental Role of Wetlands in Headwaters, J. Krecek, and M. Haigh, eds. (Dordrecht: Springer Netherlands), 143–154. doi: 10.1007/1-4020-4228-0_12
Carbó-Ramírez, P., and Zuria, I. (2011). The value of small urban greenspaces for birds in a Mexican city. Landsc. Urban Plan. 100, 213–222. doi: 10.1016/j.landurbplan.2010.12.008
Chaithong, U., Choochote, W., Kamsuk, K., Jitpakdi, A., Tippawangkosol, P., Chaiyasit, D., et al. (2006). Larvicidal effect of pepper plants on Aedes aegypti (L.) (Diptera: Culicidae). J. Vector Ecol. 31, 138–144. doi: 10.3376/1081-1710(2006)31[138:LEOPPO]2.0.CO;2
Childers, D. L., Cadenasso, M. L., Grove, J. M., Marshall, V., McGrath, B., and Pickett, S. T. A. (2015). An ecology for cities: a transformational nexus of design and ecology to advance climate change resilience and urban sustainability. Sustainability 7, 3774–3791. doi: 10.3390/su7043774
Claudio, L. (2011). Planting healthier indoor air. Environ. Health Perspect. 119, a426–a427. doi: 10.1289/ehp.119-a426
Coleman, J., Hench, K., Garbutt, K., Sexstone, A., Bissonnette, G., and Skousen, J. (2001). Treatment of domestic wastewater by three plant species in constructed wetlands. Water. Air. Soil Pollut. 128, 283–295. doi: 10.1023/A:1010336703606
Costanza, R. (2012). Ecosystem health and ecological engineering. Ecol. Eng. 45, 24–29. doi: 10.1016/j.ecoleng.2012.03.023
Della Rocca, G., Hernando, C., Madrigal, J., Danti, R., Moya, J., Guijarro, M., et al. (2015). Possible land management uses of common cypress to reduce wildfire initiation risk: a laboratory study. J. Environ. Manage. 159, 68–77. doi: 10.1016/j.jenvman.2015.05.020
Della Rocca, G., Danti, R., Raddi, P., Moya, B., and Moya, J. (2014). Implementation of the “cypress system” as a green firewall. For. Méditerr 3, 275–280.
Dhang, P. (2014). Urban Insect Pests: Sustainable Management Strategies (Makati: CABI). doi: 10.1079/9781780642758.0000
Doty, S.L., Shang, T.Q., Wilson, A.M., Tangen, J., Westergreen, A.D., Newman, L.A., et al. (2000). Enhanced metabolism of halogenated hydrocarbons in transgenic plants containing mammalian cytochrome P450 2E1. Proc. Natl. Acad. Sci. U.S.A. 97, 6287–6291. doi: 10.1073/pnas.97.12.6287
Dryess, C. T. (1975). Grass-legume mixtures for erosion control along forest roads in western Oregon. J. Soil Water Conserv. 30, 169–173.
Florea, M., Hagemann, H., Santosa, G., Abbott, J., Micklem, C.N., Spencer-Milnes, X., et al. (2016). Engineering control of bacterial cellulose production using a genetic toolkit and a new cellulose-producing strain. Proc. Natl. Acad. Sci. U.S.A. 113, E3431–E3440. doi: 10.1073/pnas.1522985113
French, K. E. (2019). Harnessing synthetic biology for sustainable development. Nat. Sustain. 2, 250–252. doi: 10.1038/s41893-019-0270-x
Giannoukos, S., Agapiou, A., Brki,ć, B., and Taylor, S. (2019). Volatolomics: A broad area of experimentation. J. Chromatogr. B 1105, 136–147. doi: 10.1016/j.jchromb.2018.12.015
Grêt-Regamey, A., Celio, E., Klein, T. M., and Wissen Hayek, U. (2013). Understanding ecosystem services trade-offs with interactive procedural modeling for sustainable urban planning. Landsc. Urban Plan. 109, 107–116. doi: 10.1016/j.landurbplan.2012.10.011
Guinée, J.B., Heijungs, R., Huppes, G., Zamagni, A., Masoni, P., Buonamici, R., et al. (2011). Life cycle assessment: past, present, and future. Environ. Sci. Technol. 45, 90–96. doi: 10.1021/es101316v
Hilty, J. A., Brooks, C., Heaton, E., and Merenlender, A. M. (2006). Forecasting the effect of land-use change on native and non-native mammalian predator distributions. Biodivers. Conserv. 15:2853. doi: 10.1007/s10531-005-1534-5
Huskinson, D. (2018). Scientists Monitor New Wetland Designed for Flood Control and Improved Stream Habitat.
Idilfitri, S., and Mohamad, N. H. N. (2012). Role of ornamental vegetation for birds' habitats in urban parks: case study FRIM, Malaysia. Procedia - Soc. Behav. Sci. 68, 894–909. doi: 10.1016/j.sbspro.2012.12.275
Ikin, K., Knight, E., Lindenmayer, D. B., Fischer, J., and Manning, A. D. (2013). The influence of native versus exotic streetscape vegetation on the spatial distribution of birds in suburbs and reserves. Divers. Distrib. 19, 294–306. doi: 10.1111/j.1472-4642.2012.00937.x
Jackson, B., Pagella, T., Sinclair, F., Orellana, B., Henshaw, A., Reynolds, B., et al. (2013). Polyscape: A GIS mapping framework providing efficient and spatially explicit landscape-scale valuation of multiple ecosystem services. Landsc. Urban Plan. 112, 74–88. doi: 10.1016/j.landurbplan.2012.12.014
Jaenson, T. G. T., Pålsson, K., and Borg-Karlson, A.-K. (2006). Evaluation of extracts and oils of mosquito (Diptera: Culicidae) repellent plants from Sweden and Guinea-Bissau. J. Med. Entomol. 43, 113–119. doi: 10.1093/jmedent/43.1.113
Jim, C. Y., and Chen, W. Y. (2008). Assessing the ecosystem service of air pollutant removal by urban trees in Guangzhou (China). J. Environ. Manage. 88, 665–676. doi: 10.1016/j.jenvman.2007.03.035
Jo, H.-K., and McPherson, G. E. (1995). Carbon storage and flux in urban residential greenspace. J. Environ. Manage. 45, 109–133. doi: 10.1006/jema.1995.0062
Kivaisi, A. K. (2001). The potential for constructed wetlands for wastewater treatment and reuse in developing countries: a review. Ecol. Eng. 16, 545–560. doi: 10.1016/S0925-8574(00)00113-0
Küken, A., and Nikoloski, Z. (2019). Computational Approaches to Design and Test Plant Synthetic Metabolic Pathways. Plant Physiol. 179, 894–906. doi: 10.1104/pp.18.01273
Leichenko, R. (2011). Climate change and urban resilience. Curr. Opin. Environ. Sustain. 3, 164–168. doi: 10.1016/j.cosust.2010.12.014
Levy, S. (2015). The ecology of artificial wetlands. BioScience 65, 346–352. doi: 10.1093/biosci/biv022
Li, Q., Kobayashi, M., Wakayama, Y., Inagaki, H., Katsumata, M., Hirata, Y., et al. (2009). Effect of phytoncide from trees on human natural killer cell function. Int. J. Immunopathol. Pharmacol. 22, 951–959. doi: 10.1177/039463200902200410
Licata, M., Gennaro, M. C., Tuttolomondo, T., Leto, C., and Bella, S. L. (2019). Research focusing on plant performance in constructed wetlands and agronomic application of treated wastewater – a set of experimental studies in Sicily (Italy). PLoS ONE 14:e0219445. doi: 10.1371/journal.pone.0219445
Liddicoat, C., Sydnor, H., Cando-Dumancela, C., Dresken, R., Liu, J., Gellie, N.J.C., et al. (2020). Naturally-diverse airborne environmental microbial exposures modulate the gut microbiome and may provide anxiolytic benefits in mice. Sci. Total Environ. 701:134684. doi: 10.1016/j.scitotenv.2019.134684
Lin, B.-S., and Lin, Y.-J. (2010). Cooling effect of shade trees with different characteristics in a subtropical urban park. HortScience 45, 83–86. doi: 10.21273/HORTSCI.45.1.83
Ljunggren, J., Borrero-Echeverry, F., Chakraborty, A., Lindblom, T.U.T., Hedenström, E., Karlsson, M., et al. (2019). Yeast volatomes differentially affect larval feeding in an insect herbivore. Appl. Environ. Microbiol. 85. doi: 10.1128/AEM.01761-19
López-Arredondo, D., González-Morales, S. I., Bello-Bello, E., Alejo-Jacuinde, G., and Herrera, L. (2015). Engineering food crops to grow in harsh environments. F1000Research 4. doi: 10.12688/f1000research.6538.1
Lubke, R. A., and Hertling, U. M. (2001). The role of European marram grass in dune stabilization and succession near Cape Agulhas, South Africa. J. Coast. Conserv. 7, 171–182. doi: 10.1007/BF02742479
Lukwa, N., Nyazema, N. Z., Curtis, C. G., Mwaiko, G. L., and Chandiwana, S. K. (1999). People's perceptions about malaria transmission and control using mosquito repellent plants in a locality in Zimbabwe. Cent. Afr. J. Med. 45. doi: 10.4314/cajm.v45i3.8456
Maffei, E. M., Gertsch, J., and Appendino, G. (2011). Plant volatiles: production, function and pharmacology. Nat. Prod. Rep. 28, 1359–1380. doi: 10.1039/c1np00021g
Masson, V., Marchadier, C., Adolphe, L., Aguejdad, R., Avner, P., Bonhomme, M., et al. (2014). Adapting cities to climate change: a systemic modelling approach. Urban Clim. 10, 407–429. doi: 10.1016/j.uclim.2014.03.004
Matsuba, M., Nishijima, S., and Katoh, K. (2016). Effectiveness of corridor vegetation depends on urbanization tolerance of forest birds in central Tokyo, Japan. Urban For. Urban Green. 18, 173–181. doi: 10.1016/j.ufug.2016.05.011
McDonald, B.C., Gouw, J.A., de Gilman, J.B., Jathar, S.H., Akherati, A., Cappa, C.D., Jimenez, J.L., et al. (2018). Volatile chemical products emerging as largest petrochemical source of urban organic emissions. Science 359, 760–764. doi: 10.1126/science.aaq0524
McDonnell, M. J., and MacGregor-Fors, I. (2016). The ecological future of cities. Science 352, 936–938. doi: 10.1126/science.aaf3630
McPhearson, T., Pickett, S.T.A., Grimm, N.B., Niemelä, J., Alberti, M., Elmqvist, T., et al. (2016). Advancing urban ecology toward a science of cities. BioScience 66, 198–212. doi: 10.1093/biosci/biw002
Michael, B., Chris, H., and Scott, M. H. (2010). Cost-Effectiveness of Green Roofs. J. Archit. Eng. 16, 136–143. doi: 10.1061/(ASCE)AE.1943-5568.0000022
Mitsch, W. J. (2012). What is ecological engineering? Ecol. Eng. 45, 5–12. doi: 10.1016/j.ecoleng.2012.04.013
Mng'ong'o, F.C., Sambali, J.J., Sabas, E., Rubanga, J., Magoma, J., Ntamatungiro, A.J., et al. (2011). Repellent plants provide affordable natural screening to prevent mosquito house entry in tropical rural settings—results from a pilot efficacy study. PLoS ONE 6:e25927. doi: 10.1371/journal.pone.0025927
Mortimer, J. C. (2019). Plant synthetic biology could drive a revolution in biofuels and medicine. Exp. Biol. Med. 244, 323–331. doi: 10.1177/1535370218793890
Munoz, L. E. A., and Riley, M. R. (2008). Utilization of cellulosic waste from tequila bagasse and production of polyhydroxyalkanoate (PHA) bioplastics by Saccharophagus degradans. Biotechnol. Bioeng. 100, 882–888. doi: 10.1002/bit.21854
Narayan, S., Beck, M.W., Wilson, P., Thomas, C.J., Guerrero, A., Shepard, C.C., et al. (2017). The value of coastal wetlands for flood damage reduction in the Northeastern USA. Sci. Rep. 7, 1–12. doi: 10.1038/s41598-017-09269-z
Nemec, K. T., and Raudsepp-Hearne, C. (2013). The use of geographic information systems to map and assess ecosystem services. Biodivers. Conserv. 22, 1–15. doi: 10.1007/s10531-012-0406-z
Nowak, D. J., Crane, D. E., and Stevens, J. C. (2006). Air pollution removal by urban trees and shrubs in the United States. Urban For. Urban Green. 4, 115–123. doi: 10.1016/j.ufug.2006.01.007
Nowak, D. J., Greenfield, E. J., Hoehn, R. E., and Lapoint, E. (2013). Carbon storage and sequestration by trees in urban and community areas of the United States. Environ. Pollut. 178, 229–236. doi: 10.1016/j.envpol.2013.03.019
Omolo, M. O., Okinyo, D., Ndiege, I. O., Lwande, W., and Hassanali, A. (2004). Repellency of essential oils of some Kenyan plants against Anopheles gambiae. Phytochemistry 65, 2797–2802. doi: 10.1016/j.phytochem.2004.08.035
Pålsson, K., and Jaenson, T. G. T. (1999). Plant products used as mosquito repellents in Guinea Bissau, West Africa. Acta Trop. 72, 39–52. doi: 10.1016/S0001-706X(98)00083-7
Pattison-Williams, J. K., Pomeroy, J. W., Badiou, P., and Gabor, S. (2018). Wetlands, flood control and ecosystem services in the smith creek drainage basin: a case study in Saskatchewan, Canada. Ecol. Econ. 147, 36–47. doi: 10.1016/j.ecolecon.2017.12.026
Pedersen Zari, M. (2019). Understanding and designing nature experiences in cities: a framework for biophilic urbanism. Cities Health. doi: 10.1080/23748834.2019.1695511. [Epub ahead of print].
Pulselli, R. M., Pulselli, F. M., Mazzali, U., Peron, F., and Bastianoni, S. (2014). Emergy based evaluation of environmental performances of Living Wall and Grass Wall systems. Energy Build. 73, 200–211. doi: 10.1016/j.enbuild.2014.01.034
Ragheb, A. A., El-Darwish, I. I., and Ahmed, S. (2016). Microclimate and human comfort considerations in planning a historic urban quarter. Int. J. Sustain. Built Environ. 5, 156–167. doi: 10.1016/j.ijsbe.2016.03.003
Riffat, S., Powell, R., and Aydin, D. (2016). Future cities and environmental sustainability. Future Cities Environ. 2:1. doi: 10.1186/s40984-016-0014-2
Rousseau, D. P. L., Lesage, E., Story, A., Vanrolleghem, P. A., and De Pauw, N. (2008). Constructed wetlands for water reclamation. Desalination 218, 181–189. doi: 10.1016/j.desal.2006.09.034
Sachdev, G. (2019). Engaging with plants in an urban environment through street art and design. Plants People Planet 1, 271–289. doi: 10.1002/ppp3.10055
Sagnelli, D., Hebelstrup, K.H., Leroy, E., Rolland-Sabaté, A., Guilois, S., Kirkensgaard, J.J.K., et al. (2016). Plant-crafted starches for bioplastics production. Carbohydr. Polym. 152, 398–408. doi: 10.1016/j.carbpol.2016.07.039
Sakamoto, S., Somssich, M., Nakata, M.T., Unda, F., Atsuzawa, K., Kaneko, Y., et al. (2018). Complete substitution of a secondary cell wall with a primary cell wall in Arabidopsis. Nat. Plants 4, 777–783. doi: 10.1038/s41477-018-0260-4
Seto, K. C., Güneralp, B., and Hutyra, L. R. (2012). Global forecasts of urban expansion to 2030 and direct impacts on biodiversity and carbon pools. Proc. Natl. Acad. Sci. U.S.A. 109, 16083–16088. doi: 10.1073/pnas.1211658109
Su, Z., Zhang, R., and Qiu, J. (2011). Decline in the diversity of willow trunk-dwelling weevils (Coleoptera: Curculionoidea) as a result of urban expansion in Beijing, China. J. Insect Conserv. 15, 367–377. doi: 10.1007/s10841-010-9310-6
Sukumar, K., Perich, M. J., and Boobar, L. R. (1991). Botanical derivatives in mosquito control: a review. J. Am. Mosq. Control Assoc. 7, 210–237.
Tang, Y., Chen, A., and Zhao, S. (2016). Carbon storage and sequestration of urban street trees in Beijing, China. Front. Ecol. Evol. 4. doi: 10.3389/fevo.2016.00053
Tilman, D. (1989). “Ecological experimentation: strengths and conceptual problems,” in Long-Term Studies in Ecology: Approaches and Alternatives, G.E. Likens, ed. (New York, NY: Springer New York), 136–157. doi: 10.1007/978-1-4615-7358-6_6
Tyagi, B. K. (2016). “Advances in vector mosquito control technologies, with particular reference to herbal products,” in Herbal Insecticides, Repellents and Biomedicines: Effectiveness and Commercialization, V. Veer, and R. Gopalakrishnan, eds. (New Delhi: Springer India), 1–9. doi: 10.1007/978-81-322-2704-5_1
Vrhovšek, D., Kukanja, V., and Bulc, T. (1996). Constructed wetland (CW) for industrial waste water treatment. Water Res. 30, 2287–2292. doi: 10.1016/0043-1354(96)00114-5
Yahia, M. W., Johansson, E., Thorsson, S., Lindberg, F., and Rasmussen, M. I. (2018). Effect of urban design on microclimate and thermal comfort outdoors in warm-humid Dar es Salaam, Tanzania. Int. J. Biometeorol. 62, 373–385. doi: 10.1007/s00484-017-1380-7
Yang, J., Yu, Q., and Gong, P. (2008). Quantifying air pollution removal by green roofs in Chicago. Atmos. Environ. 42, 7266–7273. doi: 10.1016/j.atmosenv.2008.07.003
Yin, S., Shen, Z., Zhou, P., Zou, X., Che, S., and Wang, W. (2011). Quantifying air pollution attenuation within urban parks: An experimental approach in Shanghai, China. Environ. Pollut. 159, 2155–2163. doi: 10.1016/j.envpol.2011.03.009
Keywords: sustainable development, climate change, synthetic biology, biomorphic urbanism, ecology
Citation: French KE (2021) Designing Function-Specific Plant Systems for Sustainable Urban Development. Front. Sustain. Cities 3:581764. doi: 10.3389/frsc.2021.581764
Received: 09 July 2020; Accepted: 15 April 2021;
Published: 01 June 2021.
Edited by:
Marian Stuiver, Wageningen University and Research, NetherlandsReviewed by:
Mekuria Argaw Denboba, Addis Ababa University, EthiopiaCicely Marshall, University of Cambridge, United Kingdom
Copyright © 2021 French. This is an open-access article distributed under the terms of the Creative Commons Attribution License (CC BY). The use, distribution or reproduction in other forums is permitted, provided the original author(s) and the copyright owner(s) are credited and that the original publication in this journal is cited, in accordance with accepted academic practice. No use, distribution or reproduction is permitted which does not comply with these terms.
*Correspondence: Katherine E. French, RS5rYXRoZXJpbmUuZnJlbmNoQGxibC5nb3Y=