- 1Department of Periodontics, RAK College of Dental Sciences, RAK Medical & Health Sciences University, Ras al-Khaimah, United Arab Emirates
- 2Department of Physiology, RAK College of Medical Sciences, RAK Medical & Health Sciences University, Ras-al-Khaimah, United Arab Emirates
- 3Department of Oral Radiology, RAK College of Dental Sciences, RAK Medical & Health Sciences University, Ras al-Khaimah, United Arab Emirates
- 4Department of Oral Surgery, RAK College of Dental Sciences, RAK Medical & Health Sciences University, Ras al-Khaimah, United Arab Emirates
- 5Department of Orthodontics, RAK College of Dental Sciences, RAK Medical & Health Sciences University, Ras al-Khaimah, United Arab Emirates
- 6Department of Oral Rehabilitation, Faculty of Dentistry, University of Khartoum, Khartoum, Sudan
Periodontal diseases are chronic inflammatory conditions influenced by bacterial biofilm formation and host immune responses, affecting millions worldwide. Traditional treatments like mechanical debridement and systemic antibiotics often face limitations, including biofilm resilience and antibiotic resistance. Gum Arabic (GA), a natural exudate from Acacia trees, presents a promising alternative with its anti-biofilm and anti-inflammatory properties. This review highlights the role of GA in periodontal therapy, particularly its ability to interfere with quorum sensing (QS) pathways, specifically the AI-2 signaling system used by key periodontal pathogens such as Porphyromonas gingivalis, Aggregatibacter actinomycetemcomitans, and Fusobacterium nucleatum. By disrupting QS, GA inhibits biofilm formation, reduces bacterial virulence, and promotes a balanced oral microbiome. GA's prebiotic properties also encourage the growth of beneficial bacteria, enhancing the host's immune response while preserving the systemic microbiome. Clinical studies demonstrate GA's effectiveness as an adjunct in periodontal therapy, with significant reductions in plaque accumulation, gingival inflammation, and bleeding. This highlights GA's potential as a natural therapeutic agent, offering an effective, antibiotic-sparing option in managing periodontal disease. However, further research is warranted to fully establish GA's role in comprehensive periodontal care and its long-term benefits.
Introduction
Periodontal diseases are complex, chronic inflammatory conditions influenced by biofilm formation and the host immune response (1).
They are among the most prevalent diseases worldwide, affecting up to 50% of the adult population, with severe periodontitis being the 11th most common disease globally (2).
Periodontitis can lead to tooth loss, negatively impacting aesthetics, nutrition, and overall quality of life. Moreover, it imposes significant economic burdens on healthcare systems due to the costs associated with treatment and management (3–6).
Periodontitis is a chronic inflammatory condition that results from an imbalance between the host immune response and bacterial pathogens such as Porphyromonas gingivalis, Treponema denticola, Tannerella forsythia, and Aggregatibacter actinomycetemcomitans (7). These pathogens produce virulence factors, including collagenases, sulfides, and endotoxins, which contribute to tissue damage. Various factors, such as dry mouth, biofilm retention, smoking, metabolic conditions, poor nutrition, certain medications, systemic disorders, and stress, can exacerbate this dysbiotic microbial environment.
Dysbiotic microbiome may trigger an immune response, leading to the production of pro-inflammatory cytokines such as IL-1β, IL-6, IL-1α, TNF-α, and GM-CSF. If this trigger is persistent it may result in a chronic inflammatory state that leads to the irreversible destruction of connective tissue and alveolar bone, driving the initiation and progression of periodontitis (8, 9) (Figure 1). The importance of treating periodontal disease extends beyond oral health. Periodontal infections have been linked to systemic conditions such as cardiovascular disease, diabetes, respiratory diseases, and adverse pregnancy outcomes (10–12).
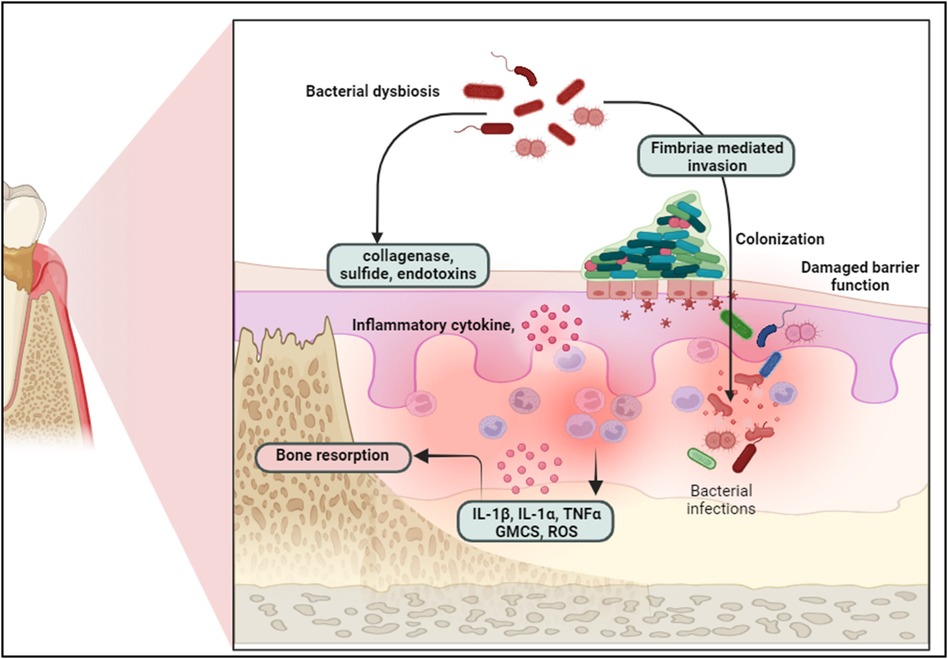
Figure 1. Bacterial dysbiosis triggers epithelial barrier disruption, bacterial colonization, and immune response, leading to inflammatory cytokine release and bone resorption in periodontal disease progression. Created with BioRender.com.
The systemic inflammation induced by periodontal pathogens and their toxins can exacerbate these conditions, highlighting the need for effective periodontal therapy.
Traditional treatments for periodontal disease often include mechanical debridement (scaling and root planing) and the use of systemic or local antibiotics (13). However, these approaches face limitations due to the resilience of biofilms and the growing concern of antibiotic resistance. Biofilms protect bacteria from external threats, making them difficult to eradicate with standard treatments (14, 15). This has spurred interest in alternative therapeutic strategies that can effectively disrupt biofilms and modulate the host immune response without contributing to antibiotic resistance.
Gum Arabic (GA), a natural exudate from Acacia trees, presents a novel approach with the potential for anti-biofilm and anti-inflammatory properties (16). Composed primarily of polysaccharides and proteoglycans, GA has been traditionally used for its medicinal properties, including its ability to soothe inflammation and promote wound healing (17).
Recent research suggests that GA may also have specific actions against biofilm formation and quorum sensing (QS) in periodontal pathogens, offering a promising adjunct to conventional periodontal therapy (18).
Formation of biofilms via quorum sensing
Biofilms are intricate microbial communities embedded in a self-produced extracellular matrix (19, 20). They play a pivotal role in periodontal diseases by enabling bacterial persistence and resistance to treatment (19).
The bacteria within biofilms communicate via quorum sensing (QS), a cell-to-cell signaling mechanism that coordinates collective behaviors beneficial to the bacterial community (21).
Quorum sensing enables bacteria in biofilms to chemically communicate and coordinate their activity as if they were multicellular organisms in response to environmental changes. Bacteria produce and export signaling molecules known as autoinducers (AIs), which are discharged into the environment and built up at a concentration corresponding to cell density, which is the basis of the classical QS signaling system (22).
The autoinducer can attach to and activate a corresponding receptor protein, which in turn triggers changes in gene expression, when a certain concentration of this signal is obtained and, consequently, a “quorum” of cells is present in a specific environment (23, 24) (Figure 2).
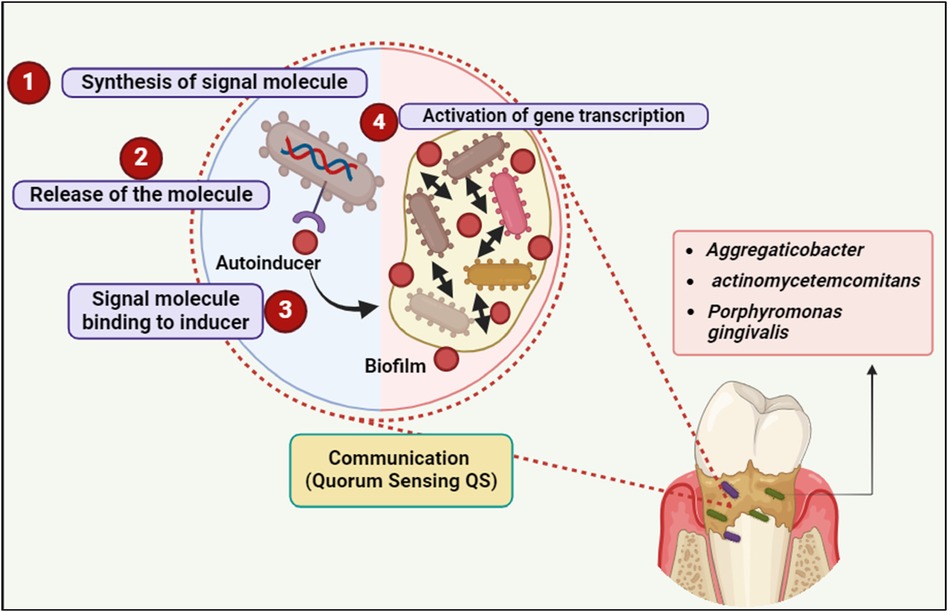
Figure 2. Quorum sensing (QS) communication in biofilm formation involves four key steps: signal molecule synthesis, release, binding to the inducer, and activation of gene transcription, facilitating bacterial communication and biofilm development by species such as Aggregatibacter actinomycetemcomitans and Porphyromonas gingivalis. Created with BioRender.com.
Bacteria use a variety of autoinducers; for example, Gram-negative bacteria frequently emit N-acyl L-homoserine lactone (AHL) derivatives, Gram-positive bacteria release peptide signals, and many species in both categories detect the interspecies signal AI-2. QS pathways regulate a diverse spectrum of gene products and phenotypes, including bioluminescence in marine bacteria and the generation of toxins and virulence factors by specific microbes (25).
When a threshold concentration of autoinducers is reached, these molecules trigger changes in gene expression, leading to coordinated activities like biofilm formation and virulence factor production (23, 24).
The formation of biofilms begins with the initial adhesion of planktonic (free-floating) bacteria to a surface (26, 27) (Figure 3). This process is facilitated by bacterial appendages like fimbriae and pili, which allow bacteria to adhere to surfaces in the oral cavity, such as teeth and epithelial cells. Once attached, the bacteria begin to multiply and form microcolonies (28). During this stage, the bacteria produce extracellular polymeric substances (EPS), a matrix composed of polysaccharides, proteins, and nucleic acids. This matrix protects the bacteria from environmental stressors and facilitates further adhesion of other microbial species (29).
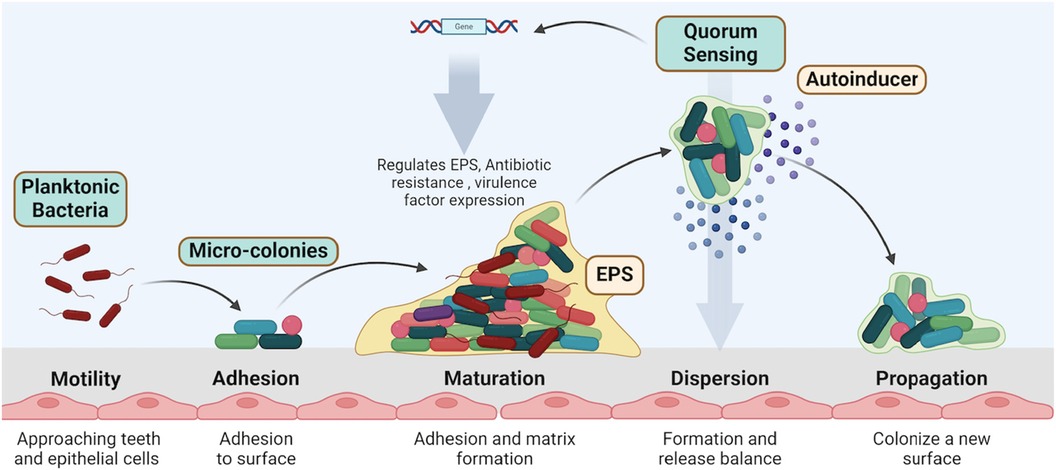
Figure 3. Biofilm formation involves key stages: motility and adhesion of planktonic bacteria, followed by micro-colony maturation, matrix formation, and quorum sensing regulation. Dispersed bacteria propagate to new surfaces, continuing the biofilm lifecycle. Created with BioRender.com.
As the biofilm matures, the microcolonies expand and merge, forming a complex, three-dimensional structure. This structure is highly organized, with channels that allow for nutrient and waste exchange (28, 30) (Figure 3). Quorum sensing plays a critical role at this stage by regulating the production of EPS and other factors necessary for biofilm integrity (31).
Bacteria within the biofilm produce and release signaling molecules known as autoinducers. The concentration of these molecules increases as the bacterial population grows. When the concentration of autoinducers reaches a threshold, bacteria detect these signals through specific receptors (32). This detection triggers a coordinated response, altering gene expression to promote biofilm maturation and maintenance (32).
Quorum sensing regulates various genes involved in biofilm formation, including those responsible for EPS production, antibiotic resistance, and virulence factor expression. For example, Gram-negative bacteria commonly use N-acyl homoserine lactones (AHLs) as autoinducers, while Gram-positive bacteria use oligopeptides (33).
In the final stage of the biofilm life cycle, some bacteria may disperse from the biofilm to colonize new surfaces. This dispersal can be triggered by changes in environmental conditions or nutrient availability. Dispersal is also regulated by quorum sensing, ensuring that bacteria can respond adaptively to their environment (34, 35) (Figure 3).
Effect of quorum sensing on the virulence of the periodontal pathogens
Quorum sensing (QS) not only regulates biofilm formation but also significantly influences the virulence of periodontal pathogens (36). The ability of bacteria to communicate and coordinate their activities enhances their pathogenicity and adaptability within the host environment (36).
QS systems control the expression of genes encoding virulence factors, such as proteases, toxins, and adhesins. In periodontal pathogens like Porphyromonas gingivalis and Aggregatibacter actinomycetemcomitans, QS regulates the production of these factors, contributing to tissue destruction and disease progression (37, 38).
By coordinating the production of virulence factors, QS enhances the ability of bacteria to evade host immune responses (36). For example, QS-regulated proteases can degrade host immune molecules, while other factors can modulate the host inflammatory response to create a more favorable environment for bacterial survival. QS is crucial for maintaining the stability and integrity of the biofilm matrix (39). The production of extracellular polymeric substances (EPS) and other matrix components is tightly regulated by QS, ensuring the biofilm's resilience against physical and chemical challenges, including host immune attacks and antimicrobial treatments (40).
QS also facilitates communication not only within a single bacterial species but also between different species within the biofilm (41). This interspecies communication can enhance the overall virulence of the microbial community (42). For instance, Fusobacterium nucleatum acts as a bridge organism, linking early colonizers with late colonizers like P. gingivalis, thereby promoting a pathogenic biofilm community (43). Furthermore, QS enables bacteria to rapidly adapt to changes in their environment, such as nutrient availability or the presence of antimicrobial agents. This adaptability is critical for the persistence of periodontal pathogens in the dynamic environment of the oral cavity (44).
A key factor in the development of periodontal disease is quorum sensing. Most quorum-sensing research on periodontopathic biofilms is based on Fusobacterium nucleatum and the red complex pathogens (45). Periodontal diseases have a huge worldwide burden and have been linked to several conditions. It is therefore not surprising that research has looked for alternate antibacterial strategies in recent years (3–5).
Dental plaque is a key contributing factor to periodontal disease, hence scaling and root planing are used as a mechanical means of removing plaque (46). Therapeutic plaque control medications are frequently regarded as adjuvant therapies when this is not adequate. Nevertheless, these treatments frequently have unfavorable side effects including tooth discoloration and unpleasant tastes (47, 48). Maintaining the normal oral flora while carefully adjusting the host immune response are the goals of new treatments for periodontal diseases (49).
New findings in quorum quenching, or quorum sensing suppression, point to a possible antibacterial strategy to reduce the toxicity of oral biofilms by blocking bacterial communication networks. Quorum quenchers can be manufactured synthetically or extracted naturally from bacteria, plants, or human saliva (45, 50).
Quorum quenching: a novel approach
It would appear that naturally existing quorum-quenching mechanisms play important roles in the interactions between microbe-microbe and pathogen-host interactions. The discovery of enzymes that degrade quorum-sensing signals in mammalian species represents a significant step forward in the field of research on quorum sensing and quenching (51).
One potential strategy for interfering with the formation of the plaque biofilm is to regulate the quorum-sensing mechanism utilized by various bacterial species. Quorum quenching, often known as QQ, is a term widely used to refer to the interference of quorum sensing (52).
Quorum quenching (QQ) involves disrupting QS pathways, thereby inhibiting biofilm formation and bacterial virulence. This strategy offers a promising alternative to conventional antibiotics, which often lead to resistance (53).
Natural and synthetic compounds can serve as quorum quenchers, providing an innovative approach to managing biofilm-associated infections like periodontal disease (54). Many of these chemicals are not antibiotics, and bacteria do not quickly develop resistance to them. After that, the antibiofilm impact is typically long-lasting. Numerous uses in the food, pharmaceutical, and other sectors are made possible because these substances are often harmless to eukaryotic organisms. Sometimes the ultimate impact of these substances is understood, while in other instances their precise mechanism of action in preventing the formation of biofilms is known (54).
Efficacy of Gum Arabic in oral health
Medicinal plants and their derivatives have long been regarded as safe medicinal agents for use in humans and as a key component of the pharmaceutical industry (55).
Gum secretions are released by leguminous and acacia trees, which are found around the world. These secretions have long been utilized as an anti-inflammatory and in the treatment of many ailments. These plant secretions can function as antibiotics against a wide range of bacterial acids that cause infections in humans (56).
Gum Arabic (GA), also known as acacia gum, is an exudate produced by the Acacia Senegal and Acacia Seyal trees, which are members of the Leguminosae family (57).
GA has the structure of an arabinogalactan-protein complex. This complex consists of Arabic acid salts in magnesium, calcium, and potassium. The GA structure consists of 1-3-linked β-D-galactopyranosyl units, with branches of two to five β-D-galactopyranosyl residues linked together by 1,3-ether linkages and connected to the core β-D-galactopyranosyl chain by 1,6-linkages (58).
GA has Anti-Biofilm Activity by interfering with QS, particularly the AI-2 signaling system used by many periodontal pathogens. This disruption can prevent biofilm formation and reduce bacterial virulence (59).
GA also exhibits broad-spectrum antibacterial effects, targeting both planktonic bacteria and those within biofilms (60).
The prebiotic effects of GA promote the growth of beneficial gut bacteria, leading to the production of short-chain fatty acids like butyrate, which have anti-inflammatory properties (61).
GA has shown potential in reducing antibiotic resistance by acting as a natural adjunct to traditional antimicrobial therapies (60). Its prebiotic properties promote the growth of beneficial bacteria in the gut and oral microbiome, which can outcompete pathogenic strains that may develop resistance (61). Additionally, GA enhances the body's immune response, helping to control infections more efficiently and reducing the need for systemic antibiotics (60). By supporting a balanced microbiome and targeting localized infections, GA limits the overuse of antibiotics, which is a key driver of resistance development. In addition, GA offers significant benefits by preserving the systemic microbiome, particularly the gut microbiome, which is often disrupted by systemic antibiotic use (61).
Antibiotics delivered systemically can lead to gut dysbiosis, antibiotic-associated diarrhea, and the overgrowth of harmful bacteria, such as Clostridium difficile (62). By limiting the treatment to the periodontal pockets, GA can prohibit these complications and maintains the body's microbial balance. Furthermore, the highly localized delivery of GA allows for more concentrated and effective treatment at the site of infection without systemic side effects, improving both efficacy and patient comfort (63). With regard to periodontal therapy, GA compounds have a great deal of promise to support microbiome homeostasis, especially when considering precision medicine. GA may be customized to a person's microbiome and immune response as opposed to using a one-size-fits-all strategy. Its prebiotic qualities promote the development of advantageous microorganisms while preventing the overabundance of harmful bacteria (64).
This targeted modulation is consistent with precision medicine, which focuses on individualized therapy approaches that take into account the distinct features of the patient's microbiome, immune system, and genetic profiles (65). By supporting the host's immune response and encouraging a balanced microbial environment, GA contributes to long-term periodontal health. Its ability to work synergistically with the host's immune system could be tailored to address specific microbiome imbalances in different patients, making it a valuable tool in personalized periodontal therapies. In this way, GA compounds may not adhere to a one-size-fits-all approach but could instead be a versatile component in individualized treatment regimens, enhancing the host-microbiome interaction for optimal outcomes (66, 67).
Molecular mechanisms involved in GA protective action in oral health
• Interference with Autoinducer Production: GA contains components like D-galactose, which can interfere with the production and function of autoinducers. D-galactose has been shown to inhibit the activity of AI-2, a common autoinducer in periodontal pathogens. This inhibition can disrupt the QS signaling necessary for biofilm formation and virulence factor production (60) (Figure 4).
• Blocking Autoinducer Receptors: GA can block the receptors that detect autoinducers, preventing the QS signals from being received and processed by the bacterial cells. This blockade hinders the bacteria's ability to coordinate their activities, thereby reducing biofilm formation and virulence (60).
• Degradation of Signaling Molecules: Some components of GA can degrade signaling molecules used in QS. By breaking down these molecules, GA disrupts the communication network within the biofilm, making it less stable and more susceptible to disruption (68).
• Anti-Inflammatory Effects: GA's anti-inflammatory properties are attributed to its ability to modulate the host immune response. Studies have demonstrated that GA can reduce the levels of pro-inflammatory cytokines, such as IL-6, and inhibit the activation of NFκB, a key regulator of inflammation (69). The prebiotic effects of GA also contribute to its anti-inflammatory properties by promoting the growth of beneficial gut bacteria that produce anti-inflammatory short-chain fatty acids like butyrate (70) (Figure 4).
• Enhancing Host Defense Mechanisms: By modulating the immune response and promoting the growth of beneficial bacteria, GA helps enhance the host’s natural defense mechanisms against periodontal pathogens. This dual action not only disrupts biofilm formation but also supports the resolution of inflammation and healing of periodontal tissues (71).
• Induction of LL-37 Production: Gum Arabic has been shown to influence the production of LL-37, primarily through the generation of butyrate, a short-chain fatty acid produced by the fermentation of dietary fibers by colonic bacteria (72). When GA, is fermented by gut microbiota, it produces butyrate. This compound has been demonstrated to upregulate the expression of LL-37 in human cells, thereby enhancing the innate immune response in the oral cavity. Butyrate induces the expression of the cathelicidin antimicrobial peptide (CAMP) gene, which encodes for LL-37. This process enhances the antimicrobial defense mechanisms of oral tissues (60, 72).
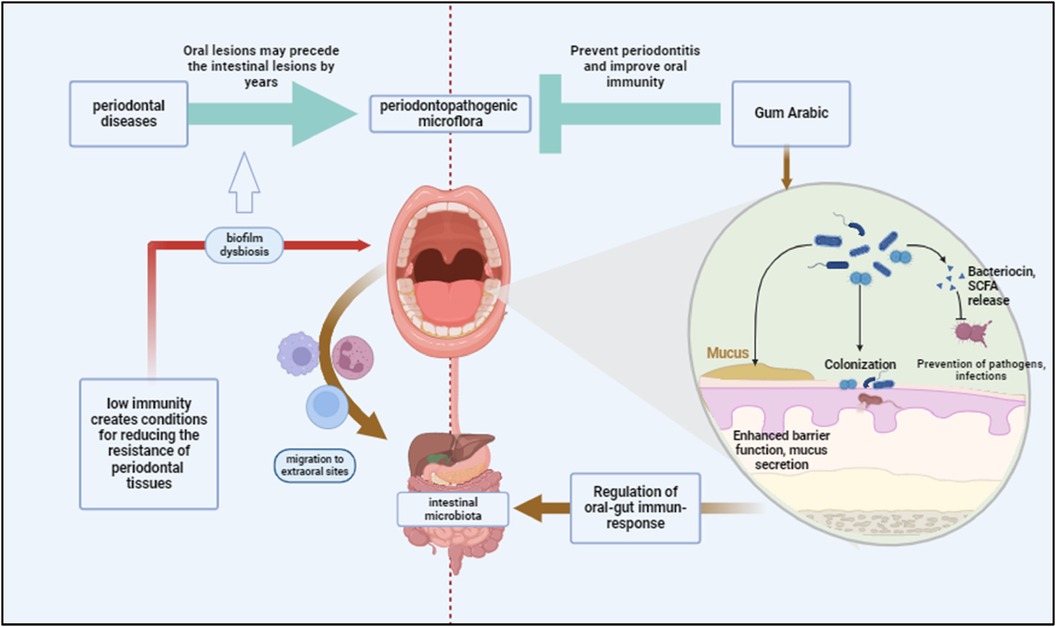
Figure 4. Periodontal diseases contribute to systemic dysbiosis by facilitating the migration of periodontopathogenic bacteria from the oral cavity to the intestines, potentially compromising immunity. Gum Arabic may prevent periodontitis by enhancing gut and oral immunity, improving barrier function, and promoting colonization by beneficial bacteria. Created with BioRender.com.
Bioactive components of Gum Arabic and their potential role in quorum sensing and biofilm
Arabinogalactan may hinder the synthesis of autoinducers such as N-acyl homoserine lactones (AHLs) in Gram-negative bacteria and autoinducer-2 (AI-2) in both Gram-negative and Gram-positive bacteria. This disruption occurs through the suppression of gene activity responsible for synthesizing these signaling molecules, as well as the degradation of QS signal molecules via lactonases and acylases, thus preventing the accumulation of autoinducers necessary for effective QS (73, 74) (Figure 5).
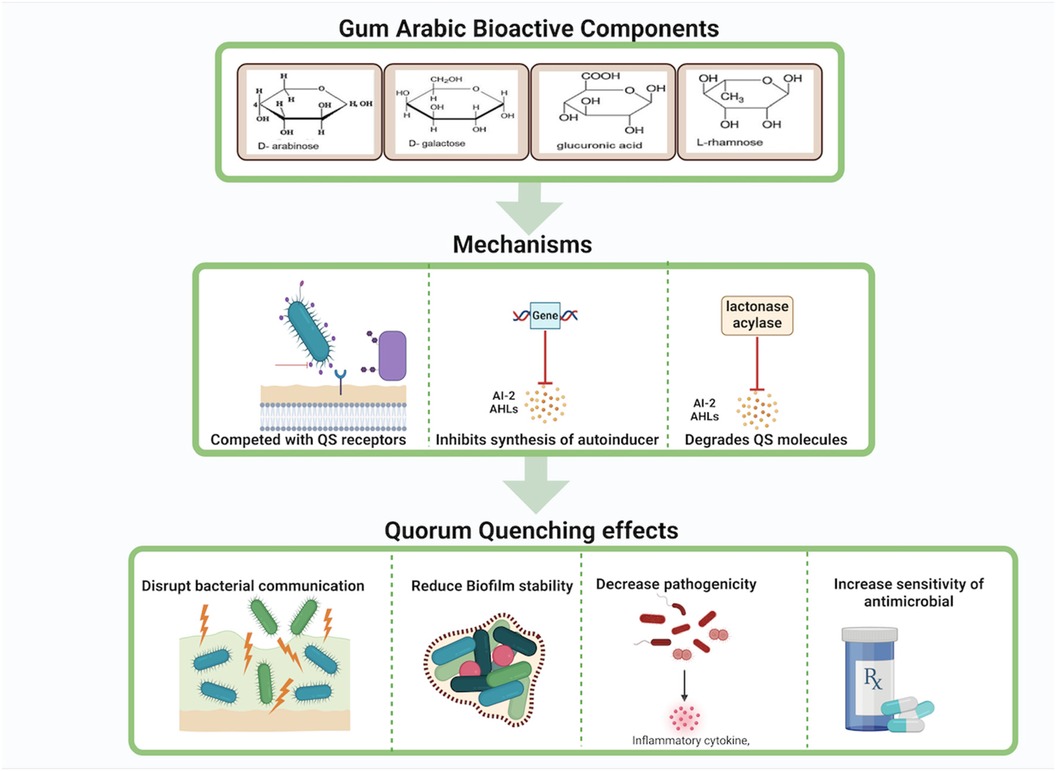
Figure 5. Gum Arabic's bioactive components exhibit quorum quenching effects by competing with quorum sensing receptors, inhibiting the synthesis of autoinducers, and degrading quorum sensing molecules. These mechanisms disrupt bacterial communication, reduce biofilm stability, decrease pathogenicity, and increase bacterial sensitivity to antimicrobials.Created with BioRender.com.
Galactose, another component of gum Arabic, can modulate pathways involved in the synthesis of QS signal molecules. It may downregulate the expression of genes involved in AHL biosynthesis, leading to reduced production of AHLs and impaired bacterial communication (75) Additionally, galactose can facilitate the production or activation of enzymes that degrade AHLs, further disrupting QS signals and reducing biofilm formation and virulence (76) (Figure 5).
Glycoproteins in gum Arabic, composed of protein backbones bound to carbohydrate chains, can also interfere with QS pathways by inhibiting the expression of genes involved in autoinducer biosynthesis (77). These glycoproteins may either have enzymatic activity or support the synthesis of enzymes that hydrolyze AHLs, preventing the buildup of functional QS signals (78). Moreover, glycoproteins might compete for QS receptors, blocking autoinducers from binding and activating these receptors, thereby suppressing QS-regulated gene expression and reducing biofilm stability and pathogenicity (79, 80) (Figures 5, 6).
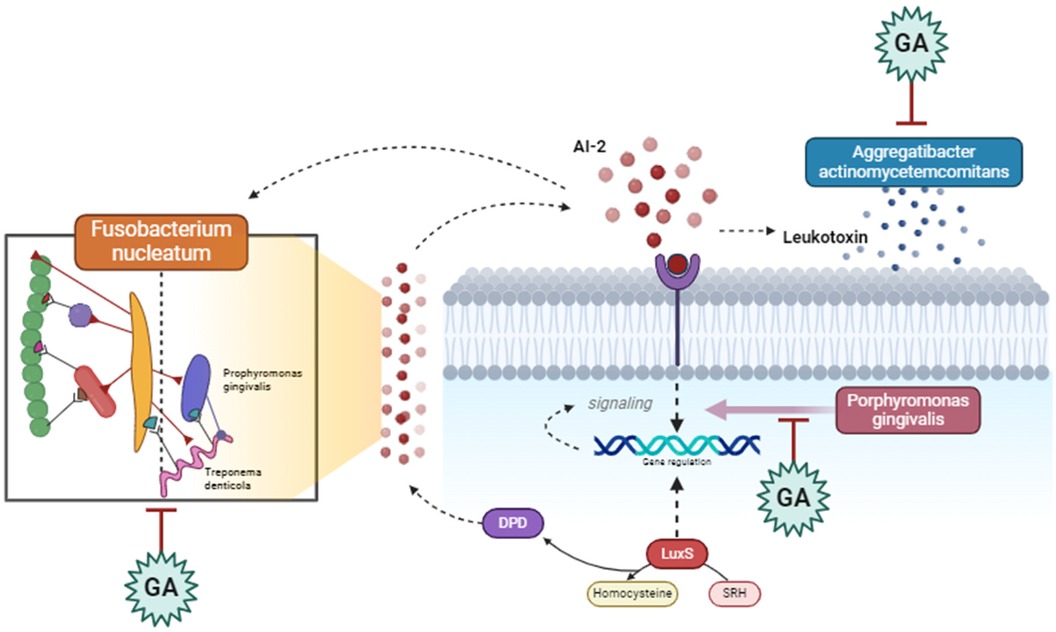
Figure 6. Gum Arabic interferes with the communication between periodontopathogenic bacteria, such as Fusobacterium nucleatum and Porphyromonas gingivalis, by inhibiting quorum sensing signals and leukotoxin production. It also modulates the activity of Aggregatibacter actinomycetemcomitans, reducing virulence factors and downregulating genes involved in pathogenicity, ultimately disrupting bacterial interactions and reducing pathogenic effects in periodontal disease. Created with BioRender.com.
Plausible effects of Gum Arabic on P. gingivalis, A. actinomycetemcomitans, and T. forsythia
Gum Arabic has plausible effects on key periodontal pathogens such as Porphyromonas gingivalis (P. gingivalis), Aggregatibacter actinomycetemcomitans (A. actinomycetemcomitans), and Tannerella forsythia (T. forsythia) (81, 82). These bacteria are significant contributors to periodontal diseases, such as gingivitis and periodontitis, due to their ability to form biofilms and utilize quorum sensing (QS) to coordinate virulence factors. GA's properties suggest it could be a valuable adjunct in managing periodontal diseases by targeting these pathogens (83). According to the research, (GA) has various positive effects on periodontal infections. GA suppresses Porphyromonas gingivalis’ generation of gingipains, which are proteolytic enzymes that contribute to tissue damage and immune evasion. It also interferes with P. gingivalis’ initial adherence to surfaces and disturbs existing biofilms, making them more susceptible to antimicrobial treatments (84). Furthermore, GA's anti-inflammatory qualities aid in minimizing inflammation produced by P. gingivalis infections, minimizing tissue damage, and promoting healing. GA components like D-galactose impede AI-2-mediated quorum sensing, limiting the pathogen's capacity to build biofilms and create virulence factors (81). GA also has direct antibacterial action against A. actinomycetemcomitans, reducing its viability and colonization capacity and disrupting the biofilm extracellular matrix, hence increasing the efficiency of mechanical debridement and antimicrobial treatments (84). Furthermore, GA's anti-inflammatory and antibacterial activities assist in diminishing the overall pathogenicity of T. forsythia, providing a better oral environment. F. nucleatum plays a central role in the formation and maturation of dental biofilms, acting as a bridge organism that facilitates the integration of early and late colonizers in the biofilm community. GA's properties suggest it could be a valuable adjunct in managing periodontal diseases by targeting F. nucleatum. GA can disrupt quorum sensing (QS), the communication mechanism used by F. nucleatum to regulate gene expression related to virulence and biofilm formation (63).
Components of GA, such as D-galactose, interfere with the production of autoinducers like AI-2. This disruption prevents F. nucleatum from reaching the critical concentration needed to trigger QS-regulated activities (81). (Figure 7). Considering this GA can also block the receptors that detect these autoinducers, effectively silencing the QS signals and preventing the coordination necessary for biofilm maturation and virulence factor production. Furthermore, GA claims to reduce the ability of F. nucleatum to adhere to tooth surfaces and other bacteria within the biofilm. This is a crucial step in preventing biofilm formation and maturation (28).
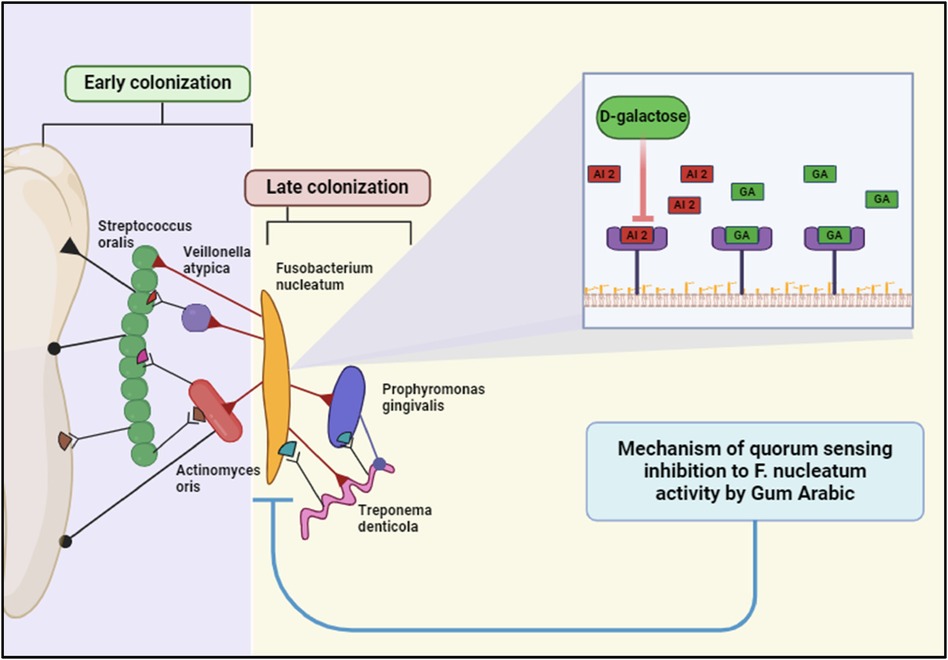
Figure 7. Gum Arabic inhibits Fusobacterium nucleatum quorum sensing via its D-galactose component, disrupting bacterial communication and biofilm maturation. This interference impairs the colonization and pathogenicity of periodontal bacteria, including late colonizers like Porphyromonas gingivalis. Created with BioRender.com.
Evidence supporting Gum Arabic’s effects on periodontal health
GA has demonstrated promising effects on periodontal health, as evidenced by multiple clinical studies. 2-blind crossover trials evaluated the antiplaque properties of GA-containing chewing gum, finding that participants using GA gum exhibited a significant reduction in plaque accumulation compared to the control group (85). Additionally, a randomized controlled trial assessed the efficacy of GA as an adjunct to scaling and root planing (SRP) in treating chronic periodontitis. This study revealed notable improvements in clinical parameters such as reduced gingival inflammation and bleeding in the GA group compared to SRP alone (86). Similarly, another randomized clinical trial investigated the clinical and microbiological benefits of commercially available GA gel and powder in managing gingivitis. Participants using GA products showed significant reductions in gingival index scores and overall inflammation compared to the placebo group (17). These findings underscore GA’s potential as a valuable adjunctive therapy in periodontal treatment, offering a natural and effective means to enhance oral health outcomes.
Therapeutic applications of Gum Arabic
• Preventive and Adjunctive Therapy: GA can be integrated into dental care products, such as mouthwashes, gels, or slow-release formulations, to enhance plaque control and reduce gingival inflammation. Combining GA with conventional periodontal therapies may offer a synergistic approach to managing periodontal diseases (18, 87).
• Nanotechnology and Gum Arabic: Recent studies have explored the use of Gum Arabic (GA) in nanotechnology, particularly in the development of GA-ZnO (Zinc Oxide) nanoparticles, which exhibit enhanced antibacterial properties (88). This integration of GA into nanotechnology has shown promising results in the context of periodontal health. GA-ZnO nanoparticles have demonstrated significant antibacterial activity against a range of periodontal pathogens, including Porphyromonas gingivalis, Aggregatibacter actinomycetemcomitans, and Tannerella forsythia. The zinc oxide component disrupts bacterial cell membranes, leading to cell lysis and death. The combination of GA and ZnO enhances the overall antibacterial effect. GA acts as a stabilizer and dispersing agent, increasing the effectiveness of ZnO nanoparticles. GA-ZnO nanoparticles can also prevent the initial adhesion of bacteria to surfaces, a critical step in biofilm formation. This property is particularly beneficial in maintaining oral hygiene and preventing periodontal diseases. These nanoparticles can penetrate existing biofilms, disrupting their structure and making the bacteria more susceptible to antimicrobial agents and mechanical removal. GA-ZnO nanoparticles can reduce the levels of pro-inflammatory cytokines such as IL-6 and TNF-α (89, 90) (Figure 8). This helps to mitigate the inflammatory response associated with periodontal diseases. GA-ZnO nanoparticles create a less conducive environment for periodontal pathogens by modulating the host immune response, thereby reducing their virulence (91, 92).
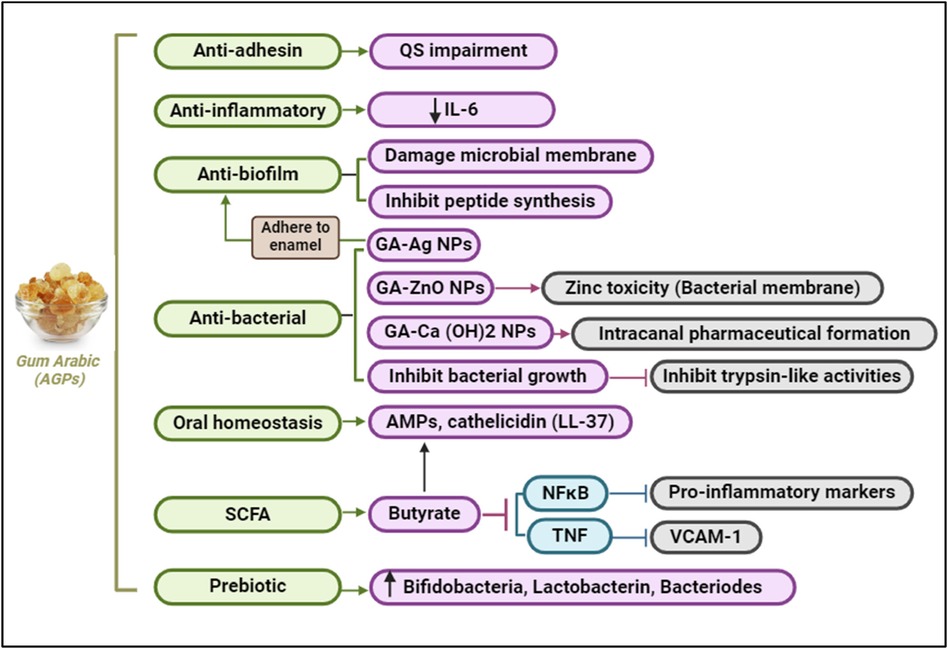
Figure 8. Gum Arabic supports oral health by impairing quorum sensing, inhibiting biofilm formation, reducing inflammation, and exerting antibacterial effects. It also promotes oral homeostasis and prebiotic activity, fostering beneficial bacteria and reducing pro-inflammatory markers. Created with BioRender.com.
Limitations & future directions
Studies have demonstrated that GA can reduce plaque accumulation and improve gingival inflammation indexes. For example, GA has been found to inhibit the adhesion of bacteria to tooth surfaces, thereby reducing plaque formation (86–88). Additionally, GA exhibits anti-inflammatory properties, leading to improved gingival health and reduced bleeding on probing (87).
However, the evidence supporting GA's effectiveness in treating periodontitis remains limited Most studies have focused on its role in plaque control and gingivitis, with fewer (86) investigations exploring its impact on the more severe forms of periodontal disease.
Recent studies have provided empirical evidence supporting the QS inhibitory effects of polysaccharides and polyphenols. For instance, a study by Singh et al. demonstrated that polysaccharides from other natural sources could bind to and neutralize AHLs, effectively disrupting QS and reducing biofilm formation in Escherichia coli (93).
Another study by Mazzantini et al. highlighted the anti-QS activity of polyphenols in Staphylococcus aureus, leading to decreased biofilm formation and virulence (94).
To better substantiate the influence of Gum Arabic in modulating quorum sensing and its potential as a therapeutic agent for periodontal disease, future research ought to focus on the following areas:
○ in vitro studies Using periodontal pathogens to evaluate GA's effects on biofilms’ development and the generation of quorum-sensing molecules.
○ Animal models to assess the therapeutic effectiveness of Gum Arabic in treating periodontal disease.
○ Examining the combined impact of GA in conjunction with other periodontal treatments to improve overall treatment outcomes.
Conclusion
While preliminary findings are promising, the role of GA in the treatment of periodontal disease, including periodontitis, requires more robust evidence. Combining GA with conventional periodontal therapies may offer a synergistic approach, but further research is crucial to confirm its effectiveness and define its place in periodontal care.
What is known about this topic
• Gum Arabic has been investigated as a natural bioactive compound in the process of quorum quenching, which might potentially provide a novel method for controlling periodontal diseases by interrupting bacterial communication. This disturbance may impede the ability of harmful oral biofilms to cause disease without developing antibiotic resistance.
• Quorum quenching may use natural substances such as phytochemicals to inhibit the communication process among bacteria, thereby reducing the likelihood of illness reoccurrence and improving the effectiveness of mechanical therapy.
• The investigation of quorum sensing and its suppression indicates potential uses in dentistry, particularly given the significant worldwide prevalence of oral conditions such as periodontitis.
What this study adds
• Gum Arabic, has the potential to provide a new approach to treating periodontal disorders by inhibiting quorum sensing.
• Gum Arabic can interfere with the bacterial communication mechanisms that cause the formation and maintenance of harmful biofilms in the mouth.
• This strategy prioritizes the inhibition of virulence factor production in bacterial populations as a crucial aspect of periodontal disease management while avoiding any contribution to the escalating issue of antimicrobial resistance.
Author contributions
NH: Conceptualization, Funding acquisition, Validation, Writing – original draft. RB: Conceptualization, Funding acquisition, Validation, Writing – review & editing. MR: Supervision, Validation, Writing – review & editing. NC: Conceptualization, Validation, Writing – review & editing. RM: Conceptualization, Data curation, Validation, Writing – review & editing. SD: Conceptualization, Validation, Writing – review & editing. BG: Conceptualization, Validation, Writing – review & editing.
Funding
The author(s) declare financial support was received for the research, authorship, and/or publication of this article. Financial support for the publication of the article was received from RAK College of Dental Sciences/ RAK Medical & Health Sciences University.
Conflict of interest
The authors declare that the research was conducted in the absence of any commercial or financial relationships that could be construed as a potential conflict of interest.
Publisher's note
All claims expressed in this article are solely those of the authors and do not necessarily represent those of their affiliated organizations, or those of the publisher, the editors and the reviewers. Any product that may be evaluated in this article, or claim that may be made by its manufacturer, is not guaranteed or endorsed by the publisher.
References
1. Hajishengallis G. Immunomicrobial pathogenesis of periodontitis: keystones, pathobionts, and host response. Trends Immunol. (2014) 35:3–11. doi: 10.1016/j.it.2013.09.001
2. GBD 2017 Disease and Injury Incidence and Prevalence Collaborators. Global, regional, and national incidence, prevalence, and years lived with disability for 328 diseases and injuries for 195 countries, 1990–2016: a systematic analysis for the global burden of disease study 2016. Lancet. (2017) 390(10100):1211–59. doi: 10.1016/S0140-6736(17)32154-2
3. Kassebaum N, Bernabć E, Dahiya M, Bhandari B, Murray C, Marcenes W. Global burden of untreated caries: a systematic review and meta-regression. J Dent Res. (2015) 94:650–8. doi: 10.1177/0022034515573272
4. Das M, Upadhyaya V, Ramachandra SS, Jithendra KD. Periodontal treatment needs in diabetic and non-diabetic individuals: a case-control study. Indian J Dent Res. (2011) 22:291–4. doi: 10.4103/0970-9290.84307
5. Wu L, Zhang SQ, Zhao L, Ren ZH, Hu CY. Global, regional, and national burden of periodontitis from 1990 to 2019: Results from the Global Burden of Disease study 2019. J Periodontol. (2022) 93:1445–54. doi: 10.1002/JPER.21-0469
6. Botelho J, Machado V, Leira Y, Proença L, Chambrone L, Mendes JJ. Economic burden of periodontitis in the United States and Europe: an updated estimation. J Periodontol. (2022) 93(3):373–9. doi: 10.1002/JPER.21-0111
7. Abdulkareem AA, Al-Taweel FB, Al-Sharqi AJB, Gul SS, Sha A, Chapple ILC. Current concepts in the pathogenesis of periodontitis: from symbiosis to dysbiosis. J Oral Microbiol. (2023) 15(1):2197779. doi: 10.1080/20002297.2023.2197779
8. Offenbacher S. Periodontal diseases: pathogenesis. Ann Periodontol. (1996) 1:821–78. doi: 10.1902/annals.1996.1.1.821
9. Hasturk H, Kantarci A. Activation and resolution of periodontal inflammation and its systemic impact. Periodontol 2000. (2015) 69(1):255–73. doi: 10.1111/prd.12105
10. Ohki T, Itabashi Y, Kohno T, Yoshizawa A, Nishikubo S, Watanabe S, et al. Detection of periodontal bacteria in thrombi of patients with acute myocardial infarction by polymerase chain reaction. Am Heart J. (2012) 163:164–7. doi: 10.1016/j.ahj.2011.10.012
11. Linden GJ, McClean K, Young I, Evans A, Kee F. Persistently raised C-reactive protein levels are associated with advanced periodontal disease. J Clin Periodontol. (2008) 35(9):741–7. doi: 10.1111/j.1600-051X.2008.01288.x
12. Paraskevas S, Huizinga JD, Loos BG. A systematic review and meta-analyses on C-reactive protein in relation to periodontitis. J Clin Periodontol. (2008) 35:277–90. doi: 10.1111/j.1600-051X.2007.01173.x
13. Socransky SS, Haffajee AD. Dental biofilms: difficult therapeutic targets. Periodontol 2000. (2002) 28:12–55. doi: 10.1034/j.1600-0757.2002.280102.x
14. Khan J, Tarar SM, Gul I, Nawaz U, Arshad M. Challenges of antibiotic resistance biofilms and potential combating strategies: a review. 3 Biotech. (2021) 11(4):169. doi: 10.1007/s13205-021-02707-w
15. Stewart Philip S, William Costerton J. Antibiotic resistance of bacteria in biofilms. Lancet. (2001) 358(9276):135–8. doi: 10.1016/S0140-6736(01)05321-1
16. Shirwaikar A, Shirwaikar A, Prabu SL, Kumar GA. Herbal excipients in novel drug delivery systems. Indian J Pharm Sci. (2008) 70:415–22. doi: 10.4103/0250-474X.44587
17. Calame W, Weseler AR, Viebke C, Flynn C, Siemensma AD. Gum Arabic establishes prebiotic functionality in healthy human volunteers in a dose-dependent manner. Br J Nutr. (2008) 100:1269–75. doi: 10.1017/S0007114508981447
18. Pradeep AR, Agarwal E, Bajaj P, Naik SB, Shanbhag N, Uma SR. Clinical and microbiologic effects of commercially available gel and powder containing Acacia Arabica on gingivitis. Aust Dent J. (2012) 57:312–8. doi: 10.1111/j.1834-7819.2012.01714.x
19. Aparna MS, Yadav S. Biofilms: microbes and disease. Braz J Infect Dis. (2008) 12:526–30. doi: 10.1590/S1413-86702008000600016
20. Sateriale D, Imperatore R, Colicchio R, Pagliuca C, Varricchio E, Volpe MG, et al. Phytocompounds vs. dental plaque bacteria: in vitro effects of myrtle and pomegranate polyphenolic extracts against single-species and multispecies oral biofilms. Front Microbiol. (2020) 11:592265. doi: 10.3389/fmicb.2020.592265
21. Grandclément C, Tannières M, Moréra S, Dessaux Y, Faure D. Quorum quenching: role in nature and applied developments. FEMS Microbiol Rev. (2016) 40:86–116. doi: 10.1093/femsre/fuv038
22. Rutherford ST, Bassler BL. Bacterial quorum sensing: its role in virulence and possibilities for its control. Cold Spring Harb Perspect Med. (2012) 2:a012427. doi: 10.1101/cshperspect.a012427
23. Bzdrenga J, Daudé D, Rémy B, Jacquet P, Plener L, Elias M, et al. Biotechnological applications of quorum quenching enzymes. Chem Biol Interact. (2017) 267:104–15. doi: 10.1016/j.cbi.2016.05.028
24. Hense BA, Schuster M. Core principles of bacterial autoinducer systems. Microbiol Mol Biol R. (2015) 79:153–69. doi: 10.1128/MMBR.00024-14
25. Ng W-L, Bassler BL. Bacterial quorum-sensing network architectures. Annu Rev Genet. (2009) 43:197–222. doi: 10.1146/annurev-genet-102108-134304
26. Jakubovics NS, Goodman SD, Mashburn-Warren L, Stafford GP, Cieplik F. The dental plaque biofilm matrix. Periodontol 2000. (2021) 86:32–56. doi: 10.1111/prd.12361
27. An YH, Dickinson RB, Doyle RJ. In: An YH, Friedman RJ, editors. Mechanisms of Bacterial Adhesion and Pathogenesis of Implant and Tissue Infections. Totowa, NJ, USA: Humana Press (2000).
28. Sharma S, Mohler J, Mahajan SD, Schwartz SA, Bruggemann L, Aalinkeel R. Microbial biofilm: a review on formation, infection, antibiotic resistance, control measures, and innovative treatment. Microorganisms. (2023) 11:1614. doi: 10.3390/microorganisms11061614
29. Irie Y, Borlee BR, O'Connor JR, Hill PJ, Harwood CS, Wozniak DJ, et al. Self-produced exopolysaccharide is a signal that stimulates biofilm formation in Pseudomonas aeruginosa. Proc Natl Acad Sci USA. (2012) 109:20632–6. doi: 10.1073/pnas.1217993109
30. Sauer K, Camper AK, Ehrlich GD, Costerton JW, Davies DG. Pseudomonas aeruginosa displays multiple phenotypes during development as a biofilm. J. Bacteriol. (2002) 184:1140–54. doi: 10.1128/jb.184.4.1140-1154.2002
31. Gupta P, Sarkar S, Das B, Bhattacharjee S, Tribedi P. Biofilm, pathogenesis and prevention–a journey to break the wall: a review. Arch Microbiol. (2016) 198:1–15. doi: 10.1007/s00203-015-1148-6
32. Gu H, Hou S, Yongyat C, De Tore S, Ren D. Patterned biofilm formation reveals a mechanism for structural heterogeneity in bacterial biofilms. Langmuir. (2013) 29:11145–53. doi: 10.1021/la402608z
33. Preda VG, Săndulescu O. Communication is the key: biofilms, quorum sensing, formation and prevention. Discoveries (Craiova). (2019) 7(3):e100. doi: 10.15190/d.2019.13
34. Chao Y, Marks LR, Pettigrew MM, Hakansson AP. Streptococcus pneumoniae biofilm formation and dispersion during colonization and disease. Front Cell Infect Microbiol. (2014) 4:194. doi: 10.3389/fcimb.2014.00194
35. Oppenheimer-Shaanan Y, Steinberg N, Kolodkin-Gal I. Small molecules are natural triggers for the disassembly of biofilms. Trends Microbiol. (2013) 21:594–601. doi: 10.1016/j.tim.2013.08.005
36. Plančak D, Musić L, Puhar I. Quorum sensing of periodontal pathogens. Acta Stomatol Croat. (2015) 49:234–41. doi: 10.15644/asc49/3/6
37. Fong KP, Gao L, Demuth DR. Luxs and arcB control aerobix growth of Actinobacillus actinomycetemcomitans under iron limitation. Infect Immun. (2003) 71(1):298–308. doi: 10.1128/IAI.71.1.298-308.2003
38. James CE, Hasegawa Y, Park Y, Yeung V, Tribble GD, Kuboniwa M, et al. Luxs involvement in the regulation of genes coding for hemin and iron acquisition systems in Porphyromonas gingivalis. Infect Immun. (2006) 74(7):3834–44. doi: 10.1128/IAI.01768-05
39. Turkina MV, Vikström E. Bacteria-host crosstalk: sensing of the quorum in the context of Pseudomonas aeruginosa infections. J Innate Immun. (2019) 11(3):263–79. doi: 10.1159/000494069
40. Ma R, Hu X, Zhang X, Wang W, Sun J, Su Z, et al. Strategies to prevent, curb and eliminate biofilm formation based on the characteristics of various periods in one biofilm life cycle. Front Cell Infect Microbiol. (2022) 12:1003033. doi: 10.3389/fcimb.2022.1003033
41. Whiteley M, Diggle SP, Greenberg EP. Progress in and promise of bacterial quorum sensing research. Nature. (2017) 551:313–20. doi: 10.1038/nature24624
42. Papenfort K, Bassler BL. Quorum sensing signal-response systems in gram-negative bacteria. Nat Rev Microbiol. (2016) 14:576–88. doi: 10.1038/nrmicro.2016.89
43. Pignatelli P, Nuccio F, Piattelli A, Curia MC. The role of Fusobacterium nucleatum in oral and colorectal carcinogenesis. Microorganisms. (2023) 11(9):2358. doi: 10.3390/microorganisms11092358
44. Zhao X, Yu Z, Ding T. Quorum-sensing regulation of antimicrobial resistance in bacteria. Microorganisms. (2020) 8:425. doi: 10.3390/microorganisms8030425
45. Wright PP, Ramachandra SS. Quorum sensing and quorum quenching with a focus on cariogenic and periodontopathic oral biofilms. Microorganisms. (2022) 10:1783. doi: 10.3390/microorganisms10091783
46. Slot D, Valkenburg C, van der Weijden F. Mechanical plaque removal of periodontal maintenance patients: a systematic review and network meta, Äêanalysis. J Clin Periodontol. (2020) 47:107–24. doi: 10.1111/jcpe.13275
47. Gupta D, Nayan S, Tippanawar HK, Patil GI, Jain A, Momin RK, et al. Are herbal mouthwash efficacious over chlorhexidine on the dental plaque? Pharmacognosy Res. (2015) 7:277–81. doi: 10.4103/0974-8490.155874
48. Jafer M, Patil S, Hosmani J, Bhandi S, Chalisserry E, Anil S. Chemical plaque control strategies in the prevention of biofilm-associated oral diseases. J Contemp Dent Pract. (2026) 17:337–43. doi: 10.5005/jp-journals-10024-1851
49. Kwon T, Lamster IB, Levin L. Current concepts in the management of periodontitis. Int Dent J. (2021) 71:462–76. doi: 10.1111/idj.12630
50. Paluch E, Rewak-Soroczyńska J, Jędrusik I, Mazurkiewicz E, Jermakow K. Prevention of biofilm formation by quorum quenching. Appl Microbiol Biotechnol. (2020) 104(5):1871–81. doi: 10.1007/s00253-020-10349-w
51. Dong YH, Wang LH, Zhang LH. Quorum quenching microbial infections: mechanisms and implications. Philos Trans R Soc Lond Biol Sci. (2007) 362:1201–11. doi: 10.1098/rstb.2007.2045
52. Babitha S, Srikanth G, Sachin BM, Sunaina S, Priyanka T, Supriya M. Quorum sensing and quorum quenching: facebook of microbial world. Int J Sci Res. (2014) 3:423–6.
53. Gonzales M, Kergaravat B, Jacquet P, Billot R, Grizard D, Chabrière É, et al. Disrupting quorum sensing as a strategy to inhibit bacterial virulence in human, animal, and plant pathogens. Pathog Dis. (2024) 82:ftae009. doi: 10.1093/femspd/ftae009
54. Zhu X, Chen WJ, Bhatt K, Zhou Z, Huang Y, Zhang LH, et al. Innovative microbial disease biocontrol strategies mediated by quorum quenching and their multifaceted applications: a review. Front Plant Sci. (2023) 13:1063393. doi: 10.3389/fpls.2022.1063393
55. Nasim N, Sandeep IS, Mohanty S. Plant-derived natural products for drug discovery: current approaches and prospects. Nucleus (Calcutta). (2022) 65:399–411. doi: 10.1007/s13237-022-00405-3
56. Gamaleldin AM, Mostafa AM, Al-Shabanah OA, Al-Bekairi AM, Nagi MN. Protective effect of Arabic gum against Acetaminophen-induced hepatotoxicity in mice. Pharmacol. Res. (2003) 48:631–5. doi: 10.1016/S1043-6618(03)00226-3
57. Putro JN, Soetaredjo FE, Lunardi VB, Irawaty W, Yuliana M, Santoso SP, et al. Polysaccharides gums in drug delivery systems: a review. Int J Biol Macromol. (2023) 253:127020. doi: 10.1016/j.ijbiomac.2023.127020
58. Yadav MP, Manuel Igartuburu J, Yan Y, Nothnagel EA. Chemical investigation of the structural basis of the emulsifying activity of Gum Arabic. Food Hydrocolloids. (2007) 21:297–308. doi: 10.1016/j.foodhyd.2006.05.001
59. Liu L, Wu R, Zhang J, Shang N, Li P. D-Ribose interferes with quorum sensing to inhibit biofilm formation of Lactobacillus paraplantarum L-ZS9. Front Microbiol. (2017) 8:1860. doi: 10.3389/fmicb.2017.01860
60. Baien SH, Seele J, Henneck T, Freibrodt C, Szura G, Moubasher H, et al. Antimicrobial and immunomodulatory effect of Gum Arabic on human and bovine granulocytes against Staphylococcus aureus and Escherichia coli. Front Immunol. (2020) 10:3119. doi: 10.3389/fimmu.2019.03119
61. Elnour AAM, Abdurahman NH, Musa KH, Rasheed Z. Prebiotic potential of gum arabic for gut health. Int J Health Sci (Qassim). (2023) 17:4–5.37929233
62. Patangia DV, Ryan CA, Dempsey E, Ross RP, Stanton C. Impact of antibiotics on the human microbiome and consequences for host health. Microbiologyopen. (2022) 11(1). doi: 10.1002/mbo3.1260
63. Gafar AM, Ramadan AM, ElSaid NA, Nurelhuda NM. Effect of Gum Arabic on plaque-induced gingivitis: a randomized controlled trial. Saudi Dent J. (2022) 34:494–502. doi: 10.1016/j.sdentj.2022.06.002
64. Kuraji R, Shiba T, Dong TS, Numabe Y, Kapila YL. Periodontal treatment and microbiome-targeted therapy in management of periodontitis-related nonalcoholic fatty liver disease with oral and gut dysbiosis. World J Gastroenterol. (2023) 29(6):967–96. doi: 10.3748/wjg.v29.i6.967
65. Hashim NT, Babiker R, Rahman MM, Mohamed R, Priya SP, Chaitanya NC, et al. Natural bioactive compounds in the management of periodontal diseases: a comprehensive review. Molecules. (2024) 29(13):3044. doi: 10.3390/molecules29133044
66. Cugini C, Ramasubbu N, Tsiagbe VK, Fine DH. Dysbiosis from a microbial and host perspective relative to oral health and disease. Front Microbiol. (2021) 12:617485. doi: 10.3389/fmicb.2021.617485
67. Campbell C, Kandalgaonkar MR, Golonka RM, Yeoh BS, Vijay-Kumar M, Saha P. Crosstalk between gut microbiota and host immunity: impact on inflammation and immunotherapy. Biomedicines. (2023) 11(2):294. doi: 10.3390/biomedicines11020294
68. Sasaki Y, Komeno M, Ishiwata A, Horigome A, Odamaki T, Xiao JZ, et al. Mechanism of cooperative degradation of gum arabic arabinogalactan protein by Bifidobacterium longum surface enzymes. Appl Environ Microbiol. (2022) 88. doi: 10.1128/aem.02187-21
69. Kaddam LA, Kaddam AS. Effect of gum arabic (Acacia Senegal) on C-reactive protein level among sickle cell anemia patients. BMC Res Notes. (2020) 13:162. doi: 10.1186/s13104-020-05016-2
70. Babiker R, Kaddam L, Mariod A. The role of Gum Arabic as an anti-inflammatory, antioxidant, and immune modulator in COVID-19: a review. Functional Food Sci. (2022) 2:242–57. doi: 10.31989/ffs.v2i10.1019
71. Al-Jubori Y, Ahmed NTB, Albusaidi R, Madden J, Das S, Sirasanagandla SR. The efficacy of Gum Arabic in managing diseases: a systematic review of evidence-based clinical trials. Biomolecules. (2023) 13:138. doi: 10.3390/biom13010138
72. Siednamohammeddeen N, Badi R, Mohammeddeen T, Enan K, Amal S. The effect of Gum Arabic supplementation on cathelicidin expression in monocyte-derived macrophages in mice. BMC Complement Med Ther. (2022) 22:149. doi: 10.1186/s12906-022-03627-9
73. Huang J, Shi Y, Zeng G, Gu Y, Chen G, Shi L, et al. Acyl-homoserine lactone-based quorum sensing and quorum quenching hold promise to determine the performance of biological wastewater treatments: an overview. Chemosphere. (2016) 157:137–51. doi: 10.1016/j.chemosphere.2016.05.032
74. Sionov RV, Steinberg D. Targeting the holy triangle of quorum sensing, biofilm formation, and antibiotic resistance in pathogenic bacteria. Microorganisms. (2022) 10(6):1239. doi: 10.3390/microorganisms10061239
75. Kumar L, Patel SKS, Kharga K, Kumar R, Kumar P, Pandohee J, et al. Molecular mechanisms and applications of N-acyl homoserine lactone-mediated quorum sensing in bacteria. Molecules. (2022) 27(21):7584. doi: 10.3390/molecules27217584
76. Sikdar R, Elias M. Quorum quenching enzymes and their effects on virulence, biofilm, and microbiomes: a review of recent advances. Expert Rev Anti Infect Ther. (2020) 18(12):1221–33. doi: 10.1080/14787210.2020.1794815
77. Verbeke F, De Craemer S, Debunne N, Janssens Y, Wynendaele E, Van de Wiele C, et al. Peptides as quorum sensing molecules: measurement techniques and obtained levels in vitro and in vivo. Front Neurosci. (2017) 11:183. doi: 10.3389/fnins.2017.00183
78. Chen F, Gao Y, Chen X, Yu Z, Li X. Quorum quenching enzymes and their application in degrading signal molecules to block quorum sensing-dependent infection. Int J Mol Sci. (2013) 14:17477–500. doi: 10.3390/ijms140917477
79. Zhang Y, Sass A, Van Acker H, Wille J, Verhasselt B, Van Nieuwerburgh F, et al. Coumarin reduces virulence and biofilm formation in Pseudomonas aeruginosa by affecting quorum sensing, type III secretion, and c-di-GMP levels. Front Microbiol. (2018) 9:1952. doi: 10.3389/fmicb.2018.01952
80. Bjarnsholt T, Givskov M. Quorum-sensing blockade as a strategy for enhancing host defenses against bacterial pathogens. Philos Trans R Soc Lond B Biol Sci. (2007) 362:1213–22. doi: 10.1098/rstb.2007.2046
81. Ryu EJ, Sim J, Sim J, Lee J, Choi BK. D-galactose as an autoinducer 2 inhibitor to control the biofilm formation of periodontopathogens. J Microbiol. (2016) 54:632–7. doi: 10.1007/s12275-016-6345-8
82. Verma K, Dhruvakumar D, Pande M. A clinical and microbiological study to assess the efficacy of Acmella oleracea and Acacia catechu herbs as local drug delivery in treatment of chronic generalized periodontitis patients. J Indian Soc Periodontol. (2022) 26:254–61. doi: 10.4103/jisp.jisp_264_21
83. Clark DT, Gazi MI, Cox SW, Eley BM, Tinsley GF. The effects of Acacia Arabica Gum on the in vitro growth and protease activities of periodontopathic bacteria. J Clin Periodontol. (1993) 20:238–43. doi: 10.1111/j.1600-051X.1993.tb00351.x
84. Kolenbrander PE, Parrish KD, Andersen RN, Greenberg EP. Intergeneric coaggregation of oral Treponema spp. with Fusobacterium spp. and intrageneric coaggregation among Fusobacterium spp. Infect Immun. (1995) 63:4584–8. doi: 10.1128/iai.63.12.4584-4588.1995
85. Gazi MI. The finding of antiplaque features in Acacia arabica type of chewing gum. J Clin Periodontol. (1991) 18:75–7. doi: 10.1111/j.1600-051X.1991.tb01123.x
86. Singhal R, Agarwal V, Rastogi P, Khanna R, Tripathi S. Efficacy of Acacia Arabica Gum as an adjunct to scaling and root planing in the treatment of chronic periodontitis: a randomized controlled clinical trial. Saudi Dent J. (2018) 30:53–62. doi: 10.1016/j.sdentj.2017.10.006
87. Tangade PS, Mathur A, Tirth A, Kabasi S. Anti-gingivitis effects of Acacia Arabica-containing toothpaste. Chin J Dent Res. (2012) 15:49–53. doi: 10.33472/AFJBS.6.2.2024.612-633
88. Ahmed O, Sibuyi N, Fadaka O, Madiehe A, Maboza E, Olivier A, et al. Antimicrobial effects of Gum Arabic-silver nanoparticles against oral pathogens. Bioinorg Chem Appl. (2022) 2022:1–11. doi: 10.1155/2022/9602325
89. Cameron SJ, Sheng J, Hosseinian F, Willmore WG. Nanoparticle effects on stress response pathways and nanoparticle-protein interactions. Int J Mol Sci. (2022) 23(14):7962. doi: 10.3390/ijms23147962
90. Amer NA, Halloull NM, FaragAllah EM, Osman NMS, Refaay NE. Potential mitigating effect of Arabic Gum on zinc oxide-induced cardiotoxicity in adult male albino rats. Afr J Bio Sci. (2024) 6:613–22.
91. Griauzdyte V, Jagelaviciene E. Antimicrobial activity of zinc against periodontal pathogens: a systematic review of in vitro studies. Medicina (Kaunas). (2023) 59:2088. doi: 10.3390/medicina59122088
92. Wang J, Du L, Fu Y, Jiang P, Wang X. Zno nanoparticles inhibit the activity of Porphyromonas gingivalis and Actinomyces naeslundii and promote the mineralization of the cementum. BMC Oral Health. (2019) 19:84. doi: 10.1186/s12903-019-0780-y
93. Singh S, Datta S, Narayanan KB, Rajnish KN. Bacterial exo-polysaccharides in biofilms: role in antimicrobial resistance and treatments. J Genet Eng Biotechnol. (2021) 19:140. doi: 10.1186/s43141-021-00242-y
Keywords: Gum Arabic (GA), periodontal disease, biofilm, quorum sensing, quorum quenching, antibacterial, anti-biofilm, anti-inflammatory
Citation: Hashim NT, Babiker R, Rahman MM, Chaitanya NCSK, Mohammed R, Dasnadi SP and Gismalla BG (2024) Gum Arabic as a potential candidate in quorum quenching and treatment of periodontal diseases. Front. Oral. Health 5:1459254. doi: 10.3389/froh.2024.1459254
Received: 3 July 2024; Accepted: 17 September 2024;
Published: 8 October 2024.
Edited by:
Hatice Hasturk, The Forsyth Institute, United StatesReviewed by:
Srinivasa Rao Sirasanagandla, Sultan Qaboos University, OmanTomaz Alves Da Silva Neto, University of North Carolina at Chapel Hill, United States
Copyright: © 2024 Hashim, Babiker, Rahman, Chaitanya, Mohammed, Dasnadi and Gismalla. This is an open-access article distributed under the terms of the Creative Commons Attribution License (CC BY). The use, distribution or reproduction in other forums is permitted, provided the original author(s) and the copyright owner(s) are credited and that the original publication in this journal is cited, in accordance with accepted academic practice. No use, distribution or reproduction is permitted which does not comply with these terms.
*Correspondence: Nada Tawfig Hashim, bmFkYS50YXdmaWdAcmFrbWhzdS5hYy5hZQ==