- Dental Physical Sciences Unit, Centre for Oral Bioengineering, Institute of Dentistry, Faculty of Medicine and Dentistry, Queen Mary University of London, London, United Kingdom
Introduction: Silver Diammine Fluoride (SDF) is a clinically used topical agent to arrest dental caries. However, the kinetics of its chemical interactions with hydroxyapatite (HA), the principal inorganic component of dental enamel, are not known. The aim was to characterize the step-wise chemical interactions between SDF and HA powder during the clinically important process of remineralization.
Methods: Two grams of HA powder were immersed in 10 ml acetic acid pH = 4.0 for 2 h to mimic carious demineralization. The powder was then washed and dried for 24 h and mixed with 1.5 ml SDF (Riva Star) for 1 min. The treated powder was then air-dried for 3 min, and 0.2 g was removed and stored in individual tubes each containing 10 ml remineralizing solution. Powder was taken from each tube at various times of exposure to remineralization solution (0 min, 10 min, 2 h, 4 h, 8 h, 24 h, and 10 days), and characterized using Magic Angle Spinning-Nuclear Magnetic Resonance (MAS-NMR) spectroscopy.
Results and discussion: 19F MAS-NMR spectra showed that calcium fluoride (CaF2) started to form almost immediately after HA was in contact with SDF. After 24 h, the peak shifted to −104.5 ppm suggesting that fluoride substituted hydroxyapatite (FSHA) was formed with time at the expense of CaF2. The 31P MAS-NMR spectra showed a single peak at 2.7 ppm at all time points showing that the only phosphate species present was crystalline apatite. The 35Cl MAS-NMR spectra showed formation of silver chloride (AgCl) at 24 h. It was observed that after the scan, the whitish HA powder changed to black color. In conclusion, this time sequence study showed that under remineralization conditions, SDF initially reacted with HA to form CaF2 which is then transformed to FSHA over time. In the presence of chloride, AgCl is formed which is subsequently photo-reduced to black metallic silver.
Introduction
Dental caries is a multifactorial process leading to a net mineral loss of dental hard tissues. It is a dynamic process which depends on the interaction of protective and pathologic factors in saliva and plaque biofilm (1). As reported in the global survey (2), 2.3 billion people suffer from dental caries of permanent teeth and more than 530 million children suffer from caries of primary teeth. In the UK, dental caries is the most common preventable disease and despite the prevention procedures provided by dentists in UK, the prevalence of experience of dental decay in 5-year-old children in England (d3mft) was 23.4%.
In the oral environment, the caries process is an alternating cycle of demineralization, the loss of tooth mineral tissue (principally calcium hydroxyapatite) via reactions with organic acids at lower pHs, and remineralization the redeposition of mineral from local calcium and phosphate ions at higher pHs (3) leading to net loss of mineral from the tooth, resulting in cavitation (1). If demineralization exceeds remineralization, then tissue loss occurs, whereas, if remineralization exceeds demineralization, then tissue replacement occurs, which is the aim of non-surgical clinical intervention such as SDF. Saliva is a unique biologic fluid with a complex composition. Saliva acts as a buffering agent, and plays an important role in the demineralization and remineralization in the oral cavity. Salivary calcium, phosphorous and hydroxyl ions are at a dynamic equilibrium with apatite mineral in enamel (4). During remineralization, the calcium and phosphate ions combine with the fluoride ions to rebuild a new surface layer on the subsurface demineralized lesion (1).
Non-restorative caries control (NRCC) treatment with silver diammine fluoride (SDF) is becoming a popular management strategy (5, 6). In the UK (for example), during the COVID pandemic, SDF was used as an intervention to arrest/remineralize cavitated carious lesions in primary teeth for pre-cooperative children due to the long general anesthetic waiting list (7). Clinical trials showed that SDF is an effective cariostatic agent, and safe to be used in children (6–14). However, SDF has the disadvantages of staining teeth black, unpleasant taste, gingival burn, and tattooing, which deters dentists to use it routinely due to low parental acceptance (15).
Solid state Nuclear Magnetic Resonance (NMR) spectroscopy is used to characterize compounds formed in chemical interactions and has been used in inorganic mineralized tissue dental research to identify various components (16, 17). For example, 19F Magic angle spinning (MAS)-NMR can identify all existing fluorine compounds in crystalline, amorphous, or adsorbed forms, within enamel mineral (18–20).
It is known that fluoride (F) interacts with the hydroxyapatite (HA) in enamel or dentine to form fluorapatite (FA) which provides cariostatic protection (e.g., 19, 21–23). Further, other studies have investigated the compounds formed when high concentration F products such as SDF (44,800 ppm F) interact with dental hard tissues (19, 24, 25). However, these previous studies did not investigate the intermediate phases in a time sequential manner, or used the detailed capability and sensitivity of 19F MAS-NMR. Hence, the aim of this current study was to investigate the chemical interactions between SDF and HA powder, and characterize the products, under standard in vitro remineralizing conditions at a sequence of time points within 24 h (known to be the time period over which the calcium is used up) and finally at 10 days using 19F, 31P and 35Cl MAS-NMR spectroscopies in order to understand the complex chemistry during the remineralization processes.
Materials and methods
To mimic exposure of dental hard tissue mineral to cariogenic acidic conditions, 2 g of HA powder (4.14 µm particle size, P3R SD, Captal HA, Plasma Biotal, UK) were immersed in 10 ml of demineralizing solution (0.1 mol/L acetic acid buffered to pH = 4.0 using potassium hydroxide) in a centrifuge tube and placed in a shaking-incubator at 37˚C for 2 h (26, 27). After centrifugation for 3 min, the powder was collected, washed, and dried on filter paper for 24 h in an incubator at 37°C. The demineralized HA powder was then mixed with 1.5 ml of 38% SDF (Riva Star, SDI, Australia, LOT 1213678) solution for 1 min, using cement spatula and made into a paste and then air-dried for 3 min, following the British Society of Paediatric Dentists (BSPD) clinical protocol for SDF application. The SDF treated demineralized HA powder was divided equally into 10 samples of 0.2 g each and stored in darkened centrifuge tubes to prevent light interaction with SDF. In 7 of the tubes, 10 ml of remineralization solution [2.0 mmol/L CaCl2,1.2 mmol/L KH2PO4, 150 mmol/L NaCl and buffered to pH = 7.0 using potassium hydroxide; as described by Siddiqui et al. (28)] were added. These tubes were placed in a shaking incubator at 37°C for different time intervals (t = 0 min, 10 min, 2 h, 4 h, 8 h, 24 h, and 10 days). At the end of each time point, the powder was collected from one of the tubes, washed, dried and analyzed using MAS-NMR spectroscopies.
MAS-NMR spectroscopy
19F, 31P and 35Cl MAS-NMR spectra were collected using a 600 MHz, 14.1 T, Avance NEO spectrometer (Bruker, Germany) using the parameters listed in Table 1. The 35Cl MAS-NMR spectra were referenced to 0 ppm of the signal in solid NaCl purchased commercially (29, 30). The spectra were processed and analyzed using the TopSpin software package (Bruker, version 4.0.8).
Results
Figure 1 shows the time series of 19F MAS-NMR spectra of HA powder treated with SDF and immersed in remineralization solution. The initial (t = 0 min) spectrum shows a dominant sharp peak at −115.8 ppm which is demonstrative of loosely bound fluoride adsorbed on the surface (31). This sharp signal was also present in the t = 10 min sample, though with the center shifted to −116.9 ppm. Also, the broad minor peak centered at −108.1 ppm shows instantaneous reactionary products. Similarly, at t = 10 min, a very small and broad peak was visible at −107.8 ppm indicating the formation of calcium fluoride (CaF2) (18). At t = 2 h, the sharp peak around −116 ppm was replaced by a broad peak at −107 ppm confirming formation of CaF2 (18). At t = 4 h, CaF2 formation continued as indicated by the broader peak at −108 ppm. At t = 24 h this peak position shifted to −105.2 ppm, indicating the formation of fluoride substituted hydroxyapatite (FSHA) (18), which is a mineral in which some (but not all) of the hydroxyl (-OH) groups in HA are substituted by F. At t = 10 days, the broad peak remained but shifted to −104.4 ppm, confirming the formation of FSHA. In addition to this signal, the spectra at t = 24 h and t = 10 day also showed a peak at −108 ppm.
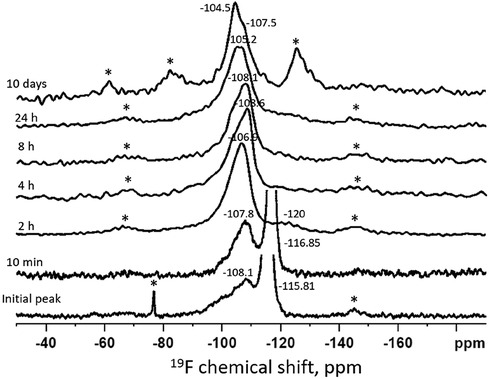
Figure 1. Time series of 19F MAS-NMR spectra of HA powder treated with SDF and immersed in remineralization solution. The duration of immersion is indicated next to each spectrum. The asterisks show the spinning side bands. The initial spectrum shows a peak position at −116 ppm indicating presence of free fluoride and the broad peak at −108 ppm suggesting the presence of a mixture of CaF2 and FSHA. With passage of time, the peak shifted from −108 ppm to −104.5 ppm indicating that more FSHA were formed at the expense of CaF2.
Figure 2 shows the time series of 31P MAS-NMR spectra of the demineralized HA powder treated with SDF, collected after immersion in remineralization solution. From t = 0 to t = 24 h, there was only one single sharp peak around 2.7 ppm, suggesting the crystalline structure of the HA did not change during their exposure to remineralization solution. No other phosphate phases were detected.
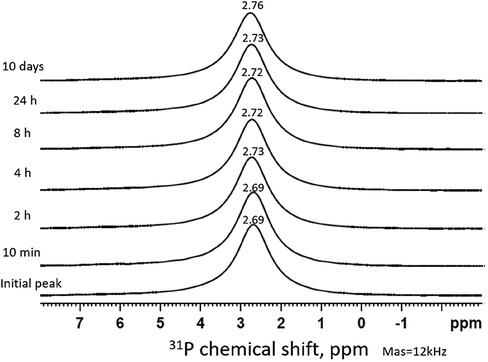
Figure 2. Time series of 31P MAS-NMR spectra of HA powder treated with SDF and immersed in remineralization solution. The duration of immersion is indicated next to each spectrum. The peak position (2.7 ppm) represents the HA pattern which remains the same throughout the time sequence.
Figure 3 shows a 35Cl MAS-NMR spectrum of the demineralized HA powder treated with SDF after immersion in remineralization solution for 24 h. The spectrum showed the reference peak at 0 ppm for NaCl. The sharp peak at 36.5 ppm shows presence of silver chloride (AgCl).
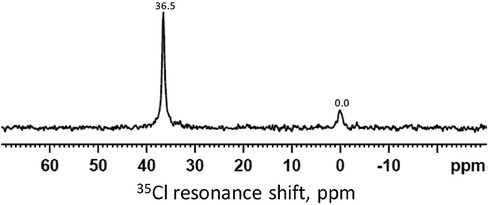
Figure 3. 35Cl MAS-NMR spectrum of the demineralized HA powder treated with SDF and immersed in the remineralization solution for 24 h. The peak at 0 ppm is the reference peak for NaCl. The peak at 36.5 ppm indicates the presence of AgCl.
After the NMR scan, when the powder was retrieved, the whitish color changed to black as shown in Figure 4.
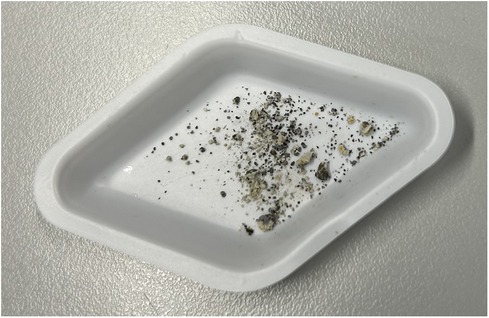
Figure 4. Powder retrieved after NMR scan and exposed to light. The white powder turned to black indicating metallic silver was formed.
Discussion
The 19F MAS-NMR results (Figure 1) shows CaF2 was dominantly formed at an early stage (t < 2 h). This could be due to the very high F concentration (44,800 ppm) in SDF. When SDF dissolved in the remineralizing solution, the free F− ions could react rapidly with the Ca2+ ions in the solution to form insoluble CaF2 (20, 32, 33). However, as the signal at −108 ppm was broad and covered a wide range of values with down to −100 ppm, contemporaneous formation (albeit a small amount) of FSHA (between −102 and −107 ppm) at this initial state cannot be excluded. Furthermore, the asymmetrical peaks at −106 to −104 ppm at later time points indicated the overlap of FSHA and CaF2. However, no fully fluoride substituted fluorapatite (FA) peak was observed. Investigating the chemical shift of the current spectra, the maximum substitution was up to 20% (18). From the trend of the chemical shift, FSHA was formed over time at the expense of CaF2. In the oral environment, the SDF may interact saliva with high calcium rapidly to form insoluble CaF2, which acts as a reservoir for FSHA formation, providing protection against acidic attack, though not as effective as fully substituted FA (34–36).
The 31P spectra (Figure 2) show the presence of HA in all time points, mainly from the HA powder. It is surprising that no other phosphate products such as silver phosphate (Ag3PO4) was detected, as reported in previous literature (26, 37). This is due to the presence of NaCl in the remineralizing solution, causing the formation of AgCl instead (Figure 3). As the content of Ag+ was small compared to the NaCl concentration, all the Ag ions were used up before they could combine with the phosphate ions. In previous studies, the demineralizing solutions did not contain NaCl, hence, Ag3PO4 was formed (26).
In the present experiment, the powders removed from the tubes were white as they were kept away from light. The black color (Figure 4) after NMR scan was likely due to the photo-reduction of AgCl to metallic silver. Clinically, SDF is topically applied using an applicator brush onto carious tooth surfaces which turn black in minutes, mainly on dentine and less so on enamel. As oral environment saliva contains chloride ions, it is likely AgCl particles are formed, which is a whitish insoluble powder. If the AgCl particles are deposited on the smooth enamel surface, they will be washed away. However, if they are deposited and accumulate in rough exposed dentinal tubules, they cannot be washed away quickly. The AgCl is then photo-reduced to black metallic silver which causes the discoloration in dentine. As these insoluble Ag particles block the dentine tubules, they may act as pulpal barrier, thus reducing dental pain and have anti-bacterial effect to reduce caries progression (38–40).
Conclusions
This study characterized the products formed as a reaction between HA and SDF under remineralizing conditions. It was found that initially CaF2 was formed, which subsequently changed to FSHA over a 24 h period. AgCl was formed rapidly which could be photo-reduced to metallic silver.
Data availability statement
The raw data supporting the conclusions of this article will be made available by the authors, without undue reservation.
Author contributions
MK: Conceptualization, Data curation, Formal Analysis, Investigation, Methodology, Writing – original draft. SS: Supervision, Writing – review & editing, Conceptualization. NK: Formal Analysis, Investigation, Methodology, Supervision, Writing – review & editing. PA: Conceptualization, Methodology, Supervision, Writing – review & editing. FW: Conceptualization, Methodology, Supervision, Writing – review & editing, Writing – original draft.
Funding
The author(s) declare financial support was received for the research, authorship, and/or publication of this article.
This research did not receive any specific grant from funding agencies in the public, commercial, or not-for-profit sectors, but was financially supported by the Institute of Dentistry.
Acknowledgments
We wish to thank Dr Harold Toms and Dr Nasima Kanwal, both the facility managers of cross faculty NMR Centre at Queen Mary University of London, London for their contribution to this project.
Conflict of interest
The authors declare that the research was conducted in the absence of any commercial or financial relationships that could be construed as a potential conflict of interest.
Publisher's note
All claims expressed in this article are solely those of the authors and do not necessarily represent those of their affiliated organizations, or those of the publisher, the editors and the reviewers. Any product that may be evaluated in this article, or claim that may be made by its manufacturer, is not guaranteed or endorsed by the publisher.
References
1. Featherstone JDB. Dental caries: a dynamic disease process. Aust Dent J. (2008) 53(3):286–91. doi: 10.1111/j.1834-7819.2008.00064.x
2. Kassebaum NJ, Smith AGC, Bernabé E, Fleming TD, Reynolds AE, Vos T, et al. Global, regional, and national prevalence, incidence, and disability-adjusted life years for oral conditions for 195 countries, 1990–2015: a systematic analysis for the global burden of diseases, injuries, and risk factors. J Dent Res. (2017) 96(4):380–7. doi: 10.1177/0022034517693566
3. Anderson P, Hector MP, Rampersad M. Critical pH in resting and stimulated whole saliva in groups of children and adults. Int J Paediatr Dent. (2001) 11(4):266–73. doi: 10.1046/j.1365-263X.2001.00293.x
4. Dodds M, Roland S, Edgar M, Thornhill M. Saliva A review of its role in maintaining oral health and preventing dental disease. BDJ Team. (2015) 2:15123. doi: 10.1038/bdjteam.2015.123
5. Bani Hani A, Santamaría RM, Hu S, Maden M, Albadri S. Minimal intervention dentistry for managing carious lesions into dentine in primary teeth: an umbrella review. Eur Arch Paediatr Dent. (2021) 23(5):667–93. doi: 10.1007/s40368-021-00675-6
6. Urquhart O, Tampi MP, Pilcher L, Slayton RL, Araujo MWB, Fontana M, et al. Nonrestorative treatments for caries: systematic review and network meta-analysis. J Dent Res. (2018) 98(1):14–26. doi: 10.1177/0022034518800014
7. Recommendations for Paediatric Dentistry during the recovery phase of the COVID-19 pandemic (2020).
8. Chu CH, Lo ECM, Lin H. Effectiveness of silver diamine fluoride and sodium fluoride varnish in arresting dentin caries in Chinese pre-school children. J Dent Res. (2002) 81(11):767–70. doi: 10.1177/0810767
9. Chu CH, Lo ECM. Promoting caries arrest in children with silver diamine fluoride: a review. Oral Health Prev Dent. (2008) 6(4):315–21. doi: 10.3290/j.ohpd.a14177
10. Fung MHT, Duangthip D, Wong MCM, Lo ECM, Chu CH. Randomized clinical trial of 12% and 38% silver diamine fluoride treatment. J Dent Res. (2018) 97(2):171–8. doi: 10.1177/0022034517728496
11. Seifo N, Robertson M, MacLean J, Blain K, Grosse S, Milne R, et al. The use of silver diamine fluoride (SDF) in dental practice. Br Dent J. (2020) 228(2):75–81. doi: 10.1038/s41415-020-1203-9
12. Yassin R, Amer H, Tantawi ME. Effectiveness of silver diamine fluoride versus sodium fluoride varnish combined with mother’s motivational interviewing for arresting early childhood caries: a randomized clinical trial. BMC Oral Health. (2023) 23(1):710. doi: 10.1186/s12903-023-03456-3
13. Zheng FM, Yan IG, Duangthip D, Lo ECM, Gao SS, Chu CH. Caries prevention using silver diamine fluoride: a 12-month clinical trial. Int Dent J. (2023) 73(5):667–73. doi: 10.1016/j.identj.2022.12.005
14. Gao SS, Chen KJ, Duangthip D, Wong MCM, Lo ECM, Chu CH. Arresting early childhood caries using silver and fluoride products—a randomised trial. J Dent. (2020) 103:103522. doi: 10.1016/j.jdent.2020.103522
15. Crystal YO, Niederman R. Evidence-based dentistry update on silver diamine fluoride. Dent Clin North Am. (2019) 63(1):45–68. doi: 10.1016/j.cden.2018.08.011
16. White DJ, Bowman WD, Faller RV, Mobley MJ, Wolfgang RA, Yesinowski JP. 19F MAS-NMR and solution chemical characterization of the reactions of fluoride with hydroxyapatite and powdered enamel. Acta Odontol Scand. (1988) 46(6):375–89. doi: 10.3109/00016358809004791
17. Yesinowski JP, Mobley MJ. Fluorine-19 MAS-NMR of fluoridated hydroxyapatite surfaces. J Am Chem Soc. (1983) 105:6191–3. doi: 10.1021/ja00357a060
18. Gao Y, Karpukhina N, Law RV. Phase segregation in hydroxyfluorapatite solid solution at high temperatures studied by combined XRD/solid state NMR. RSC Adv. (2016) 6(105):103782–90. doi: 10.1039/c6ra17161c
19. Hiraishi N, Sayed M, Hill R, Shimada Y. Solid-state NMR spectroscopy measurement of fluoride reaction by bovine enamel and dentin treated with silver diammine fluoride. Dent Mater. (2022) 38(5):769–77. doi: 10.1016/j.dental.2022.04.017
20. Mohammed NR, Kent NW, Lynch RJ, Karpukhina N, Hill R, Anderson P. Effects of fluoride on in vitro enamel demineralization analyzed by 19F MAS-NMR. Caries Res. (2013) 47(5):421–8. doi: 10.1159/000350171
21. Buzalaf MAR, Pessan JP, Honório HM, Ten Cate JM. Mechanisms of action of fluoride for caries control. Monogr Oral Sci. (2011) 22:97–114. doi: 10.1159/000325151
22. Rosin-Grget K, Lincir I. Current concept on the anticaries fluoride mechanism of the action. Coll Antropol. (2001) 25(2):703–12. Available online at: https://api.semanticscholar.org/CorpusID:3657996111811302
23. White DJ, Nancollas GH. Physical and chemical considerations of the role of firmly and loosely bound fluoride in caries prevention. J Dent Res. (1990) 69(2_suppl):587–94. doi: 10.1177/00220345900690s116
24. Mei ML, Nudelman F, Marzec B, Walker JM, Lo ECM, Walls AW, et al. Formation of fluorohydroxyapatite with silver diamine fluoride. J Dent Res. (2017) 96(10):1122–8. doi: 10.1177/0022034517709738
25. Yu OY, Mei ML, Zhao IS, Li QL, Lo EC, Chu CH. Remineralisation of enamel with silver diamine fluoride and sodium fluoride. Dent Mater. (2018) 34(12):e344–52. doi: 10.1016/j.dental.2018.10.007
26. Huang WT, Anderson P, Duminis T, Shahid S. Effect of topically applied silver compounds on the demineralisation of hydroxyapatite. Dent Mater. (2022) 38(4):709–14. doi: 10.1016/j.dental.2022.02.013
27. Ferizoli B, Cresswell-Boyes AJ, Anderson P, Lynch RJ, Hill RG. Effects of fluoride on in vitro hydroxyapatite demineralisation analysed by 19F MAS-NMR. Front Dental Med. (2023) 24(4):1171827. doi: 10.3389/fdmed.2023.1171827
28. Siddiqui S, Anderson P, Al-Jawad M. Recovery of crystallographic texture in remineralized dental enamel. PLoS One. (2014) 9(10):e108879. doi: 10.1371/journal.pone.0108879
29. Bryce DL, Sward GD. Solid-state NMR spectroscopy of the quadrupolar halogens: chlorine-35/37, bromine-79/81, and iodine-127. Magn Reson Chem. (2006) 44(4):409–50. doi: 10.1002/mrc.1741
30. Holmes ST, Vojvodin CS, Veinberg N, Iacobelli EM, Hirsh DA, Schurko RW. Hydrates of active pharmaceutical ingredients: a 35Cl and 2H solid-state NMR and DFT study. Solid State Nucl Magn Reson. (2022) 122:101837. doi: 10.1016/j.ssnmr.2022.101837
31. Duke CV, Miller JM, Clark JH, Kybett AP. 19F mas NMR and FTIR analysis of the adsorption of alkali metal fluorides onto alumina. J Mol Catal. (1990) 62(2):233–42. doi: 10.1016/0304-5102(90)85216-5
32. Cate JMT. Review on fluoride, with special emphasis on calcium fluoride mechanisms in caries prevention. Eur J Oral Sci. (1997) 105(5):461–5. doi: 10.1111/j.1600-0722.1997.tb00231.x
33. Øgaard B. The cariostatic mechanism of fluoride. Compend Contin Educ Dent. (1999) 20(1 Suppl):10–7. quiz 34.
34. García-Godoy F, Hicks MJ. Maintaining the integrity of the enamel surface. J Am Dent Assoc. (2008) 139:25S–34S. doi: 10.14219/jada.archive.2008.0352
35. Hicks J, García-Godoy F, Flaitz CM. Biological factors in dental caries enamel structure and the caries process in the dynamic process of demineralization and remineralization (part 2). J Clin Pediatr Dent. (2005) 28(2):119–24. doi: 10.17796/jcpd.28.2.617404w302446411
36. Robinson C. Fluoride and the caries lesion: interactions and mechanism of action. Eur Arch Paediatr Dent. (2009) 10(3):136–40. doi: 10.1007/bf03262674
37. Yamaga R, Nishino M, Yoshida S, Yokomizo I. Diammine silver fluoride and its clinical application. J Osaka Univ Dent Sch. (1972) 12:1–20. PMID: 4514730.4514730
38. Arends J, Ruben JL, Christoffersen J, Jongebloed WL, Zuidgeest TG. Remineralization of human dentine in vitro. Caries Res. (1990) 24(6):432–5. doi: 10.1159/000261304
39. Seto J, Horst JA, Parkinson DY, Frachella JC, DeRisi JL. Enhanced tooth structure via silver microwires following treatment with 38 percent silver diamine fluoride. Pediatr Dent. (2020) 42(3):226–31. Available at: https://pubmed.ncbi.nlm.nih.gov/3252232832522328
Keywords: remineralization, MAS-NMR, cariostatic, SDF, silver chloride, fluoride-substituted hydroxyapatite
Citation: Kaur M, Shahid S, Karpukhina N, Anderson P and Wong FSL (2024) Characterization of chemical reactions of silver diammine fluoride and hydroxyapatite under remineralization conditions. Front. Oral. Health 5:1332298. doi: 10.3389/froh.2024.1332298
Received: 2 November 2023; Accepted: 24 January 2024;
Published: 1 March 2024.
Edited by:
Raman Bedi, King’s College London, United KingdomReviewed by:
Sandrine Bittencourt Berger, North University of Paraná, BrazilDavid John Manton, University Medical Center Groningen, Netherlands
© 2024 Kaur, Shahid, Karpukhina, Anderson and Wong. This is an open-access article distributed under the terms of the Creative Commons Attribution License (CC BY). The use, distribution or reproduction in other forums is permitted, provided the original author(s) and the copyright owner(s) are credited and that the original publication in this journal is cited, in accordance with accepted academic practice. No use, distribution or reproduction is permitted which does not comply with these terms.
*Correspondence: F. S. L. Wong Zi5zLmwud29uZ0BxbXVsLmFjLnVr