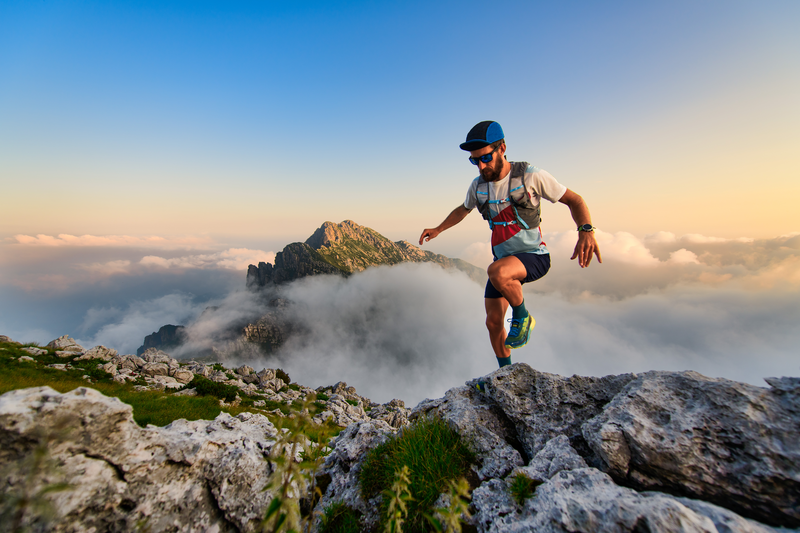
94% of researchers rate our articles as excellent or good
Learn more about the work of our research integrity team to safeguard the quality of each article we publish.
Find out more
REVIEW article
Front. Oral. Health , 17 April 2023
Sec. Oral Cancers
Volume 4 - 2023 | https://doi.org/10.3389/froh.2023.942604
Pleomorphic adenoma (PA) is the most common salivary gland tumor, accounting for 50%–60% of these neoplasms. If untreated, 6.2% of PA may undergo malignant transformation to carcinoma ex-pleomorphic adenoma (CXPA). CXPA is a rare and aggressive malignant tumor, whose prevalence represents approximately 3%–6% of all salivary gland tumors. Although the pathogenesis of the PA-CXPA transition remains unclear, CXPA development requires the participation of cellular components and the tumor microenvironment for its progression. The extracellular matrix (ECM) comprises a heterogeneous and versatile network of macromolecules synthesized and secreted by embryonic cells. In the PA-CXPA sequence, ECM is formed by a variety of components including collagen, elastin, fibronectin, laminins, glycosaminoglycans, proteoglycans, and other glycoproteins, mainly secreted by epithelial cells, myoepithelial cells, cancer-associated fibroblasts, immune cells, and endothelial cells. Like in other tumors including breast cancer, ECM changes play an important role in the PA-CXPA sequence. This review summarizes what is currently known about the role of ECM during CXPA development.
Pleomorphic adenoma (PA) is the most common salivary gland tumor (SGT), accounting for 50%–60% of these neoplasms (1). If untreated, it is estimated that up to 6.2% of PAs undergo malignant transformation to carcinoma ex pleomorphic adenoma (CXPA) (2). Multiple recurrences, male sex, advanced age, previous radiation therapy, and tumor size are the most frequently reported risk factors for the malignancy process (1, 3, 4). CXPA can be characterized as a rare and aggressive malignant tumor (5), that represents approximately 3%–6% of all SGTs and presents a 5-year survival of around 63% (6, 7). Most of them affect the parotid glands, usually manifesting in adult women between 50 and 70 years old (5, 7). Recent studies showed that genomic instability (8, 9), metabolic shifts (10), and changes in the tumor microenvironment (TME), mainly governed by the myoepithelial cell (11), are the most important molecular events oin the CXPA carcinogenesis.
TME is an active component that is in constant remodeling (12–14). Neoplastic cells can regulate the microenvironment to promote cell survival (15, 16). The role of the TME is now becoming appreciated as an important component in cancer development, which is driven by interactions between tumor cells and their microenvironment. Many reports have stated that is possible to reprogram genotypically malignant cells into phenotypically normal cells by manipulating the TME and inhibiting signaling pathways (17, 18). The extracellular matrix (ECM) is the non-cellular component of the TME composed of assembled macromolecules such as laminins, proteoglycan (PG) complex, collagen, integrins, and cadherins. Several studies have focused on the ECM etiology and genesis of neoplasms to provide a targeted therapeutic basis (16, 17, 19–21). The highly organized structure of the ECM is also home to important biomolecules and growth factors, as well as biomechanical forces that can modulate cancer cells to promote the metastatic cascade (22). PA and CXPA are tumors that present a dense and abundant ECM with high amounts of PGs and collagens (Figure 1). In the PA-CXPA sequence, ECM is especially interesting as its structure and components change throughout the PA malignant transformation, showing a potential promoting role in the CXPA progression and invasion.
Figure 1. Schematic illustration of extracellular matrix (ECM) components in the tumor microenvironment of PA and CXPA. ECM is rich in macromolecules such as laminins, proteoglycan (PG) complex, collagen, and fibronectin. The interaction between cells and ECM is mainly mediated by cell receptors of the matrix components, such as integrins. Metalloproteinases (MMPs) promote the degradation and remodeling of ECM, favoring cell proliferation.
In this review, we provided an overview of the key components of the ECM and their degradation enzymes aiming at improving our knowledge of the mechanisms contributing to the progression of the PA-CXPA sequence and CXPA dissemination. We also discussed ECM-based diagnosis as well as the potential new approaches for direct and complementary therapeutic targets for this tumor.
Proteoglycans (PGs) are macromolecules formed by a central protein in which one or more chains are covalently linked (23). PGs are important in modulating the mechanical properties of ECM, including its rigidity (24). The remodeling of the ECM represents an important factor in tumor development as significant modification in the synthesis of PGs occurs (25–27). ECMs enriched with PGs are associated with malignant transformation, inflammatory infiltration in the TME, and tumor aggressiveness (24, 28). PGs are implicated in cell migration/invasion and alteration of TME in different tumors such as colorectal carcinoma, esophageal carcinoma, hepatocellular carcinoma, and breast carcinoma (29–32). In all these carcinomas, targeting PGs may provide new therapeutic approaches in the future.
Regarding PA, recently a systematic literature review highlighted the importance of PGs in tumor development (33). Their findings showed that while normal salivary gland myoepithelial cells cannot secrete PGs, neoplastic myoepithelial cells of PA produce numerous PGs reflecting tumor development and its biological behavior (33). Xylosyltransferase II (XT-II), an isoform of xylosyltransferase I (XT-I) is involved in the initial step of PGs biosynthesis. Silencing of XT-I and XT-II genes via RNAi blocked PGs biosynthesis in neoplastic myoepithelial cells from the primary culture of salivary gland PA, leading to inhibition of invasion, migration, and tumor implantation into the fibroblast framework (34, 35). These findings provide evidence for the crucial role of PGs in the formation of the PA tumor stroma (Table 1). Indeed, over time, several PGs have been identified and correlated with PA ECM including lumican, chondromodulin-I, chondroitin 4S, chondroitin 6S, dermatan sulfate, heparan sulfate, and keratan sulfate (36–40). In this regard, it is worth citing syndecan-1 (CD138), a member of the cell surface heparan sulfate PGs family. CD138 has been reported in several types of tumors, including breast, urinary bladder, gallbladder, pancreatic, ovarian, endometrial, and prostate cancer, and normal tissues (41). In PA, however, CD138 expression did not present any correlation regarding the tumor behavior (42). Further studies, however, are encouraged to understand the role of CD138 in the malignant transformation of PA.
Extremely common, the presence of myxoid stroma in PA has already been correlated with recurrence (43). In this regard, among many other structural components, the myxoid stroma of the PA is rich in perlecan (37). This PG acts in the signaling of such growth factors as bFGF (44). In PA, the presence of perlecan in the myxoid stroma was critical for capsular invasion, and vascular involvement of the neoplasm (45). We believe that the study of perlecan in the matrix in the PA-CXPA sequence may provide important evidence for the tumor invasion of CXPA and, consequently, distant metastasis of this neoplasm.
Hyaluronan (HA) and its binding molecules, cartilage binding protein (LP), and PGs (as aggrecan and versican) are structural components of ECM (46, 47). HA-LP-aggrecan complexes are present in the chondromyxoid matrix of the PA, but not in malignant SGTs. In histopathological analysis, this finding is crucial and may assist in distinguishing de novo carcinomas from those originating in a PA (48). Indeed, aggrecan has long been reported as an important character of the epithelial-mesenchymal transition (EMT) and tissue heterogeneity in PA (49, 50). While versican is specific for malignant neoplasms (51), in PA it may be present in mesenchymal fibrous areas and some myoepithelial cells (48). This finding may indicate an area susceptible to PA malignant transformation.
Homing cell adhesion molecule (CD44) is a transmembrane PG related to cell-cell adhesion and cell-ECM adhesion. It interacts with several ligands, including hyaluronic acid, collagen, FN, and laminin (52). CD44 has been reported as an important character for PA tumor initiation (53). Curiously, while the CD44 gene is overexpressed in PA (54), the CD44 protein is downregulated (55–57). CD44 protein expression is increased as malignant transformation takes place (58, 59). Different results, however, can be found in the literature. The use of different isoforms and heterogeneity in analysis among different studies may justify the reduced expression of CD44 in CXPA in other studies (56, 60). CD44 has been recognized as one of the markers of multipotency of neoplastic cells in SGTs (61) and this sheds light on its potential as a therapeutic target for the treatment of CXPA.
In several cancers, including breast and colon carcinomas, the tenascin family can be considered an important marker of tumor progression (62). The expression of tenascin in PA is related to tumor expansion (63). TN is observed in the stromal compartment, being more pronounced in the denser stromal and chondroid areas than in the myxoid and hyaline areas (64, 65). The expression of tenascin in chondroid areas could be explained by the fact that tenascin binds to chondroitin sulfate proteoglycans of the matrix (62). Chondromyxoid differentiation in the PA may be induced by tenascin (66). It is important to note that tenascin in the PA is usually present among the epithelial tumor cells that form the ductiform structures and are therefore not in direct communication with the supporting stroma (67). Other groups had already suggested that tenascin is produced by epithelial cells of the SGTs (68).
Interestingly, during PA malignant transformation, this pattern is altered. Tenascin expression is absent in the malignant ductal epithelial cells of the CXPA while a strong and diffuse positivity is present in the cytoplasm of the CXPA malignant myoepithelial cells (69). These findings indicate the importance of tenascin as a contributor to the myoepithelial cell-promoting potential in the CXPA (11). Indeed, a key promoting role of tenascin in the CXPA progression was evidenced in the study by Félix and collaborators (2004) where all cases of CXPA presenting positivity for tenascin showed metastases at some point during the follow-up period (70) (Table 1). Furthermore, tenascin seems to be important for CXPA tumor invasion and progression. In the invasive areas, tenascin showed strong expression on the tumor front of intracapsular and minimally invasive CXPA, while its expression was focal within the tumor. Interestingly, expression was low or negative in frankly invasive CXPA (70).
Collagen represents about 30% of the total proteins in humans and is the most abundant fibrous protein in the interstitial ECMs (16, 23). The collagen superfamily is composed of twenty-eight different types of collagens that differ in structure and properties (71). In cancer, collagen is modified to provide modulation of the TME favoring malignant cellular proliferation (72). Several studies have shown that collagen is an important prognostic factor correlated with cancer invasion, lymph node metastasis, clinical stage, and treatment resistance of various types of cancers, such as esophageal carcinoma, pancreatic carcinoma, colorectal carcinoma, and ovarian carcinoma (73).
In the very first report on the diversity of collagen expression in PA, the authors showed evidence of the relationship between collagen and PA cell proliferation (74) (Table 1). Other studies, however, showed that this tumor presents a heterogeneous pattern of collagen expression. Type I and II collagens compose extracellular collagen crystalloids, structures that can be found in the tumor parenchyma (75). Type II collagen is also present in the PA chondroid areas (49, 76) while type IV collagen is more common in PA with hyaline, fibrous, and chondroid stroma (37). Type VII collagen seems to have a more heterogeneous and diffuse expression in different PA ECMs types (77). When malignant transformation occurs, the interstitial matrix becomes increasingly desmoplastic to allow invasion of cancer cells. Tumor desmoplasia is a feature associated with increased activity of cancer-associated fibroblasts (CAFs) (78). The role of CAFs in CXPA seems to have been explored only recently. A single study available in the literature using immunolabeling of vimentin and α-SMA showed that CAFs are abundantly present in carcinomatous areas of CXPA (79). Several clinical trials targeting CAFs are currently active in different tumors, such as head and neck cancer, gastrointestinal carcinoma, hepatocellular carcinoma, breast carcinoma, melanoma, and other solid tumors (80). This highlights the need for further exploration of the role of CAFs in CXPA and other salivary carcinomas.
What is known so far, is that of all existing collagen types, type I and IV collagens seem to be the most important in the PA malignant transformation. Araújo and colleagues (2009), analyzed epithelial components of CXPA at different stages of invasion. The frankly invasive CXPA showed type I collagen expression among the small nests of tumor cells that embraced the invasive front. In these areas, direct contact of tumor cells with type I collagen could be associated with reduced expression of adhesion molecules E-cadherin and β-catenin and with invasive behavior of ductal epithelial cells (81). On the other hand, in the earliest stages, as in intraductal carcinomas, type I collagen present an amorph and disorganized morphology (81). In this initial stage, type I collagen would act by impairing the function of myoepithelial cells as tumor suppressors, by inducing the increase of fibroblast growth factor 2 (FGF-2.) Indeed, type I collagen has the ability to affect tumor cell behavior (78). In mammary gland cancer, for example, type I collagen is deregulated and is implicated with more invasive behavior and metastasis of tumor cells (82, 83). Type IV collagen appears to be even more important and has already been related to CXPA metastatic behavior, being significantly more expressed in CXPA than in developed metastases (84). Indeed, type IV collagen is involved in tumor invasion and metastasis in other similar models of tumorigenesis, such as colorectal cancer (85) and breast cancer (86) (Table 1).
Integrins are transmembrane heterodimers that bind to ECM components providing support for cell motility and invasion (87). There are twenty-four different integrin dimers, each with different tissue and matrix binding specificity (88, 89). Due to their complex action on cellular mechanisms, an alteration in integrin-mediated adhesion and signaling may participate in cancer initiation and progression (87, 90). Crosstalk between integrin and ECM is crucial for maintaining tumor, metastasis, and drug resistance (91). Studies also pointed to a relationship between the activation of these proteins and the maintenance of tumor stem cells (90).
In the normal salivary gland, the expression of integrins and their subunits usually occurs in myoepithelial cells, basal cells, and ductal cells (92, 93). Similar characteristics were found in PA with basically all tumor cells positive for VLA-integrin. Interestingly, this similarity in pattern could suggest a more important pathogenic action of ductal basal cells (92). In a similar fashion to the normal salivary gland, the expression of integrin and all its subunits in PA can be noted in luminal cells and intercellular contacts of myoepithelial cells (94). The role of this protein in PA-CXPA progression has not yet been explored. An increased expression of integrin in malignant salivary tumors, in addition to its relationship with invasion capacity, has already been reported (62). However, more aggressive salivary gland carcinomas (SGCs) seem to present a lower expression of the protein (94) probably due to its deterioration in face of cell adhesion loss (95) (Table 1).
During the evolution of carcinoma in situ to an invasive CXPA, the malignant luminal cells normally surrounded by benign myoepithelial cells invade the stromal area, while myoepithelial cells undergo a process of differentiation becoming autophagic and senescent (96). The myoepithelial cell in the autophagy/senescence process may produce metabolites that would be used as energy by the epithelial cells. The epithelial cells may break the basement membrane and invade the adjacent tissues (96, 11).
Recent studies have shown that the loss of integrin-mediated cell adhesion induces autophagy, and it may contribute to the autophagy of myoepithelial cells. As the role of integrin in the PA-CXPA sequence is still unclear, more studies correlating these hypotheses are needed. On the other hand, clinical trials have failed to investigate the efficacy of therapies targeting integrins in prostate cancer, colorectal cancer, melanoma, glioma, and other solid tumors (97). Several promising possibilities of integrins as therapeutic targets await clinical trials, this way studies focused on the heterogeneous expression of integrin in PA-CXPA should be encouraged.
Laminins are extracellular glycoprotein constituents of the basement membrane responsible for the stratification of the epithelial cells and connective tissue (98, 99). About 16 laminin trimers are reported in vivo. The distribution of laminin isoforms depends on tissue type, and they are important for embryogenesis, organogenesis, angiogenesis, and tissue repair (100–102). In tumorigenesis, once activated, these proteins promote cell proliferation, migration, differentiation, and metastasis (98, 99). Indeed, laminin facilitates cell migration and invasion in invasive ductal breast cancer (103), gastric cancer (104), and bladder cancer (105). In addition, laminin may be correlated with the immune response in ovarian cancer (104).
In the normal salivary gland, laminin is expressed in the basement membrane, around the acini and ducts, associated with the presence of the myoepithelial cells (93). In SGCs, laminin expression is reduced, and its discontinuation is frequently observed due to the destruction of the basement membrane in the face of malignancy (93). Similarly, to the normal salivary gland and other SGCs, in PA and CXPA laminin is expressed in the ECM component (67). Studies demonstrated a pattern of intense immunohistochemical labeling around neoplastic cell clusters (37, 84, 106). A similar pattern has also been noted in other SGCs such as adenoid cystic carcinoma and SGCs (67, 106). While CXPA laminin expression is prominent in benign myoepithelial cells surrounding malignant areas, PA laminin expression in myoepithelial cells was shown to be reduced (93, 107). This pattern suggests a change in the myoepithelial cell phenotype during CXPA development (109). With regards to the expression of laminin in PA matrices, this protein is more frequently observed in hyaline matrices when compared to myxoid and chondroid matrices (37, 106). When malignant transformation occurs, laminin expression was shown to be present in both benign and malignant areas of the CXPA (84) (Table 1).
Fibronectins are composed of two subunits covalently connected with disulfide bonds at their C-termini (108). Several stimuli trigger the production of fibronectin matrix fibers, and continuous production is required to maintain the presence of the prior fibronectin matrix (108, 109). In addition to being involved in the stages of morphogenesis, remodeling, and repair (109), the fibronectin matrix plays a favorable role in tumor progression and is dramatically upregulated around the tumor vascular network (16, 23). In head and neck cancer, fibronectin may promote proliferation, migration, and invasion of tumor cells and induces macrophage M2 polarization in vitro (110). In breast cancer, fibronectin expression in tumor cells promotes metastasis (111). Pharmacological inhibition of fibronectin in breast cancer slowed cancer progression in vitro and in vivo, and this highlights its role as adjuvant therapy in these tumors (112).
In PA, fibronectin exhibits strong reactivity in the fibrous stroma (65), especially in the chondromyxoid matrix (66, 67). In PA malignant transformation, in areas of in situ carcinoma, fibronectin expression is shown to be increased when compared to areas of residual PA. At this stage, fibronectin may assist in inhibiting myoepithelial cell suppressor function (70) and, therefore, contribute to tumor growth. Fibronectin showed a different expression pattern in different types of CXPA invasion. While in intracapsular CXPA the pattern of the fibronectin expression was found to be around ductal structures, in minimally invasive and frankly invasive tumors the fibers positive for fibronectin showed to be present among the malignant cell nests. This may point to a dynamic performance of the ECM in the progression of the CXPA (89). However, some areas of the tumor edge of intracapsular and minimally invasive CXPA do not express fibronectin. This may highlight the conflicting roles of fibronectin throughout tumor progression (113) (Table 1) and further studies are encouraged to define its role in disease progression, especially given its recent role in cancer therapy (114).
The cadherin superfamily is composed of over 100 members there are expressed in the highest levels in distinct tissue types during development (115). In malignant tumors, the cadherins family is downregulated causing a reduction in cell-cell adhesiveness (116). In breast cancer, tumor types of analogs to SGCs, E-cadherin has been associated with invasion and metastasis (117–119).
In the normal salivary gland, cadherins were found in the membrane around acinar and ductal cells, but with absent expression in myoepithelial cells (120, 121). In the case of PA and CXPA, the pattern of expression is similar, with loss of expression in carcinomatous regions associated with loss of cell cohesion and invasion (81, 120, 121). In CXPA methylation of the E-cadherin promoter was related to luminal differentiation, high tumor grade, tumor size, and high TNM stage (122). In contrast, in PA, overexpression of the cadherin-11 subunit has been related to tumor recurrence (123). E-cadherin has already been indicated as a mediator of adenoma-carcinoma progression in pancreatic tumors (124). Few studies have evaluated the relationship of this protein with the PA malignant transformation. Araújo et al. (2009) showed that type I collagen can reduce the membrane expression of E-cadherin in frankly invasive CXPA, and maybe a factor that contributes to tumor invasion (81). Genelhu and collaborators (2007) related the expression of β-catenin—participant of the adhesion complex E-cadherin/catenins—with molecular events in the phenotype transition and initiation of PA-CXPA progression (125) (Table 1).
MMPs are a group of zinc-dependent endopeptidases related to the degradation and remodeling of the ECM. To date, 23 MMPs have been identified and many are implicated in cancer, especially MMP-1, MMP-2, MMP-3, and MMP-9 (126, 127). MMPs can degrade the protein components of the ECM and basement membrane, facilitating tumor invasion and progression (126, 128). MMP-2 and MMP-9 are perhaps the most studied. MMP-2 is known to cleave tenascin. MMP-9 can degrade laminin, collagen IV, and FN (129). The action of MMPs is regulated by the interaction of tissue inhibitors: TIMP-1, TIMP-2, TIMP-3, and TIMP-4 (130). Alterations in TIMPs are present in all human cancers and play an important role not only in the TME but also in cellular interaction with cytokines and growth factors (130, 131).
A recent meta-analysis evaluated the relationships of variants in MMP-2, MMP-7, and MMP-9 for cancer risk (132). The results of this work showed that MMP-2 rs243865 is most correlated with esophageal cancer and lung cancer, MMP-7 rs11568818 with bladder and cervical cancer, and MMP-9 rs3918242 with breast cancer. In breast cancer, the pattern of expression of MMPs can assist in tumor prognostic classification. It has been reported that neoplasms with high expression of TIMPs were correlated with a more indolent clinical course and good prognosis, while tumors with high expression of MMPs were correlated with a more aggressive clinical course and increased risk of recurrences (131).
In SGTs, the expression of MMPs have been shown similarities across benign tumors (133) while in carcinomas, the imbalance between MMPs/TIMPs is associated with tumor invasion and metastasis. Overexpression of MMP-2 was associated with more aggressive behavior (134), while MMP-7, MMP-9, and MMP-13 are related to a poor prognosis (135–137). The positive expression of the MMPs inducer (EMMPRIN) was considered an angiogenic factor in these tumors, reinforcing the promoting role of MMPs in the induction, maintenance, and progression of SGTs (138).
Regarding PA, MMP-2, MMP-9, TIMP-1, and TIMP-2 seem to control local invasiveness in vitro (139). A study using ELISA compared MMPs and TIMPs in the salivary fluid of patients with PA and salivary SGCs. Their results showed that MMP-8, TIMP-1, and TIMP-2 are important biomarkers for the diagnosis of PA (140). Although there is a high expression of MMP-2 and MMP-9 in the myoepithelial cells of the PA, the expression of MMP-2 is significantly higher and more stable than MMP-9 (141). Regarding MMP-2, in vitro assays showed that its action leads to the degradation of the basement membrane and contact of myoepithelial cells with the tumoral stroma. This mechanism may be dependent on EGF interaction with tumor cells that act to modify the expression of the E-cadherin/β-catenin complex (142, 143).
Given the MMPs changes found in PA and cancers, we could hypothesize that MMPs may play a promoting role in the PA malignant transformation, with the myoepithelial cells as a key component (70). Corroborating with this hypothesis, a recent study by Martinez et al. showed an increase in MMP-2 and MMP-9 and decreased TIMP-2 mRNA and protein expression when myoepithelial cells were exposed to epithelial cells exosomes in vitro (144) (Table 1).
With regards to TIMPs in PA and other SGCs, different studies have shown that the increase in MMPs expression is followed by an increase in TIMP expression (especially TIMP-1 and TIMP-2) and this may represent a regulatory mechanism to maintain the balance of the MMPs/TIMPs ratio (141, 145).
In summary, in this work, we reviewed the complexity of the ECM in the CXPA development and progression. The studies summarized here have revealed how the heterogeneity and versatility of the ECM in the PA and during its malignant transformation may affect the biology and behavior of these tumors. Important changes in the components of the ECM in this class of tumors including the presence of perlecan in PA and its association with tumor recurrence as well as the higher expression of fibronectin, collagen type I, and IV, and lower expression of e-cadherin in the CXPA development. As in other cancer types, this review reinforced the perceptions and addressed PA-CXPA not only as a disease of cell transformation and uncontrolled proliferation but also as changes in TME that undergo dynamic remodeling during all stages of the PA-CXPA sequence. Further knowledge of the role of ECM in this entity may provide tools for promising therapeutic targets hampering cancer cells' ability to metastasize and effectively limit the spread of CXPA malignant cells.
JFS and FVM contributed to the conception, design, data acquisition, and interpretation drafted and critically revised the manuscript. RALS, LL, MCFRA, and IAD contributed to data acquisition and interpretation and critically revised the manuscript. AA and author ESAE contributed to the conception, design, and critically revised manuscript. JNS and IOB critically revised the manuscript. All authors contributed to the article and approved the submitted version.
The São Paulo State Research Foundation (FAPESP, São Paulo, Brazil, grant numbers 15/07304-0; 19/06809-2; 19/09419-0; 2021/12567-1) and the Coordination of Training of Higher Education Graduate Foundation (CAPES, Brasilia, Brazil, finance code 001).
Figure 1 was created with BioRender.com.
The authors declare that the research was conducted in the absence of any commercial or financial relationships that could be construed as a potential conflict of interest.
All claims expressed in this article are solely those of the authors and do not necessarily represent those of their affiliated organizations, or those of the publisher, the editors and the reviewers. Any product that may be evaluated in this article, or claim that may be made by its manufacturer, is not guaranteed or endorsed by the publisher.
1. Bell D, Bullerdiek J, Gnepp DR, Schwartz MR, Stenman G, Triantafyllou A. Pleomorphic adenoma. In: El-Naggar A, Chan JKC, Grandis JR, Takata T, Slootweg P, editors. World health organization classification of tumours. Lyon: IARC Press (2017). p. 185–6.
2. Gnepp DR. Malignant mixed tumors of the salivary glands: a review. Pathol Annu. (1993) 28(Pt 1):279–328. Available at: http://www.ncbi.nlm.nih.gov/pubmed/8380049.8380049
3. Singh K, Agarwal C, Pujani M, Verma P, Chauhan V. Carcinoma ex pleomorphic adenoma: A diagnostic challenge on cytology. Diagn Cytopathol. (2017) 45:651-54. doi: 10.1002/dc.23704
4. Egal ES, Mariano FV, Altemani AM, Metze K. Age and adenoma size are independent risk factors for the development of carcinoma ex pleomorphic adenoma. Oral Oncol. (2018) 84:106–7. doi: 10.1016/j.oraloncology.2018.07.003
5. Mariano FV, Noronha ALF, Gondak RO, Altemani AdA, de Almeida OP, Kowalski LP. Carcinoma ex pleomorphic adenoma in a Brazilian population: clinico-pathological analysis of 38 cases. Int J Oral Maxillofac Surg. (2013) 42:685–92. doi: 10.1016/j.ijom.2013.02.012
6. Ye P, Gao Y, Mao C, Guo C-B, Yu G-Y, Peng X. Carcinoma ex pleomorphic adenoma: is it a high-grade malignancy? J Oral Maxillofac Surg. (2016) 74:2093–104. doi: 10.1016/j.joms.2016.03.037
7. Williams M, Ihrler S, Seethala R. Carcinoma ex pleomorphic adenoma. In: El-Naggar A, Chan JKC, Grandis JR, Takata T, Slootweg P, editors. World health organization classification of head and neck tumours. Lyon: IARC Press (2017). p. 176–7.
8. Antony J, Gopalan V, Smith RA, Lam AKY. Carcinoma ex pleomorphic adenoma: a comprehensive review of clinical, pathological and molecular data. Head Neck Pathol. (2012) 6:1–9. doi: 10.1007/s12105-011-0281-z
9. El-Naggar AK, Callender D, Coombes MM, Hurr K, Luna MA, Batsakis JG. Molecular genetic alterations in carcinoma ex-pleomorphic adenoma: a putative progression model? Genes, Chromosom Cancer. (2000) 27:162–8. doi: 10.1002/(SICI)1098-2264(200002)27:2%3C162::AID-GCC7%3E3.0.CO;2-9
10. Scarini JF, Rosa LF, Souza RADL, Egal ESA, Tincani AJ, Martins AS, et al. Gene and immunohistochemical expression of HIF-1α, GLUT-1, FASN, and adipophilin in carcinoma ex pleomorphic adenoma development. Oral Diseases. (2020) 26(6):1190–99. doi: doi: 10.1111/odi.13332
11. Scarini JF, Egal ESA, de Lima-Souza RA, Crescencio LR, Emerick C, Kowalski LP, et al. Two sides of the same coin: insights into the myoepithelial cells in carcinoma ex pleomorphic adenoma development. Crit Rev Oncol Hematol. (2021) 157:103195. doi: 10.1016/j.critrevonc.2020.103195
12. Yu H, Mouw JK, Weaver VM. Forcing form and function: biomechanical regulation of tumor evolution. Trends Cell Biol. (2011) 21:47–56. doi: 10.1016/j.tcb.2010.08.015
13. Lu P, Weaver VM, Werb Z. The extracellular matrix: a dynamic niche in cancer progression. J Cell Biol. (2012) 196:395–406. doi: 10.1083/jcb.201102147
14. Gerarduzzi C, Hartmann U, Leask A, Drobetsky E. The matrix revolution: matricellular proteins and restructuring of the cancer microenvironment. Cancer Res. (2020) 80:2705–17. doi: 10.1158/0008-5472.CAN-18-2098
15. Malik R, Lelkes PI, Cukierman E. Biomechanical and biochemical remodeling of stromal extracellular matrix in cancer. Trends Biotechnol. (2015) 33:230–6. doi: 10.1016/j.tibtech.2015.01.004
16. Bonnans C, Chou J, Werb Z. Remodelling the extracellular matrix in development and disease. Nat Rev Mol Cell Biol. (2014) 15:786–801. doi: 10.1038/nrm3904
17. Cox TR, Erler JT. Remodeling and homeostasis of the extracellular matrix: implications for fibrotic diseases and cancer. Dis Model Mech. (2011) 4:165–78. doi: 10.1242/dmm.004077
18. Bissell MJ, Radisky D. Putting tumours in context. Nat Rev Cancer. (2001) 1:46–54. doi: 10.1038/35094059
19. Druso JE, Fischbach C. Biophysical properties of extracellular matrix: linking obesity and cancer. Trends Cancer. (2018) 4:271–3. doi: 10.1016/j.trecan.2018.02.001
20. Erdogan B, Webb DJ. Cancer-associated fibroblasts modulate growth factor signaling and extracellular matrix remodeling to regulate tumor metastasis. Biochem Soc Trans. (2017) 45:229–36. doi: 10.1042/BST20160387
21. Denys H, Braems G, Lambein K, Pauwels P, Hendrix A, De Boeck A, et al. The extracellular matrix regulates cancer progression and therapy response: implications for prognosis and treatment. Curr Pharm Des. (2009) 15:1373–84. doi: 10.2174/138161209787846711
22. Kai F, Drain AP, Weaver VM. The extracellular matrix modulates the metastatic journey. Dev Cell. (2019) 49:332–46. doi: 10.1016/j.devcel.2019.03.026
23. Theocharis AD, Skandalis SS, Gialeli C, Karamanos NK. Extracellular matrix structure. Adv Drug Deliv Rev. (2016) 97:4–27. doi: 10.1016/j.addr.2015.11.001
24. Barkovskaya A, Buffone A Jr, Žídek M, Weaver VM. Proteoglycans as Mediators of Cancer Tissue Mechanics. Front Cell Dev Biol. (2020) 8:569377. doi: 10.3389/fcell.2020.569377
25. Theocharis AD, Gialeli C, Bouris P, Giannopoulou E, Skandalis SS, Aletras AJ, et al. Cell-matrix interactions: focus on proteoglycan-proteinase interplay and pharmacological targeting in cancer. FEBS J. (2014) 281:5023–42. doi: 10.1111/febs.12927
26. Iozzo RV, Sanderson RD. Proteoglycans in cancer biology, tumour microenvironment and angiogenesis. J Cell Mol Med. (2011) 15:1013–31. doi: 10.1111/j.1582-4934.2010.01236.x
27. Iozzo RV, Schaefer L. Proteoglycan form and function: a comprehensive nomenclature of proteoglycans. Matrix Biol. (2015) 42:11–55. doi: 10.1016/j.matbio.2015.02.003
28. Gray AL, Pun N, Ridley AJL, Dyer DP. Role of extracellular matrix proteoglycans in immune cell recruitment. Int J Exp Pathol. (2022) 103:34–43. doi: 10.1111/iep.12428
29. Dituri F, Gigante G, Scialpi R, Mancarella S, Fabregat I, Giannelli G. Proteoglycans in cancer: friends or enemies? A special focus on hepatocellular carcinoma. Cancers (Basel). (2022) 14:1902. doi: 10.3390/cancers14081902
30. Fernández-Ponce C, Geribaldi-Doldán N, Sánchez-Gomar I, Navarro Quiroz R, Atencio Ibarra L, Gomez Escorcia L, et al. The role of glycosyltransferases in colorectal cancer. Int J Mol Sci. (2021) 22:5822. doi: 10.3390/ijms22115822
31. Lepucki A, Orlińska K, Mielczarek-Palacz A, Kabut J, Olczyk P, Komosińska-Vassev K. The role of extracellular matrix proteins in breast cancer. J Clin Med. (2022) 11:1250. doi: 10.3390/jcm11051250
32. Zhu Y, Cheung ALM. Proteoglycans and their functions in esophageal squamous cell carcinoma. World J Clin Oncol. (2021) 12:507–21. doi: 10.5306/wjco.v12.i7.507
33. Wang J, Zhang YN. Roles of proteoglycans in the tumourigenesis and development of adenoid cystic carcinoma and pleomorphic adenoma of the salivary gland: a systematic review. Chinese J Dent Res Off J Sci Sect Chinese Stomatol Assoc. (2020) 23:11–25. doi: 10.3290/j.cjdr.a44332
34. Liu H, Wang J, Ren G, Zhang Y, Dong F. The relationship between proteoglycan inhibition via xylosyltransferase II silencing and the implantation of salivary pleomorphic adenoma. J Oral Pathol Med. (2017) 46:504–12. doi: 10.1111/jop.12510
35. Ren G, Zhang Y, Wang J, Liu H, Dong F. Effect of xylosyltransferase-I silencing on implanting growth of salivary pleomorphic adenoma. Med Sci Monit. (2018) 24:3772–81. doi: 10.12659/MSM.911014
36. Kusafuka K, Ishiwata T, Sugisaki Y, Takemura T, Kusafuka M, Hisha H, et al. Lumican expression is associated with the formation of mesenchyme-like elements in salivary pleomorphic adenomas. J Pathol. (2004) 203:953–60. doi: 10.1002/path.1599
37. Saku T, Cheng J, Okabe H, Koyama Z. Immunolocalization of basement membrane molecules in the stroma of salivary gland pleomorphic adenoma. J Oral Pathol Med. (1990) 19:208–14. doi: 10.1111/j.1600-0714.1990.tb00827.x
38. Shibutani T, Iwayama Y, Tsubone M, Ando C, Yamada K, Mori M. Immunohistochemical localization of glycosaminoglycans with the use of monoclonal antibodies in salivary pleomorphic adenomas. Anticancer Res (1990) 10:1533–42. Available at: http://www.ncbi.nlm.nih.gov/pubmed/22852262285226
39. Nara Y, Takeuchi J, Yoshida K, Fukatsu T, Nagasaka T, Kawaguchi T, et al. Immunohistochemical characterisation of extracellular matrix components of salivary gland tumours. Br J Cancer. (1991) 64:307–14. doi: 10.1038/bjc.1991.297
40. Kusafuka K, Hiraki Y, Shukunami C, Yamaguchi A, Kayano T, Takemura T. Cartilage-Specific matrix protein chondromodulin-I is associated with chondroid formation in salivary pleomorphic adenomas. Am J Pathol. (2001) 158:1465–72. doi: 10.1016/S0002-9440(10)64097-7
41. Kind S, Merenkow C, Büscheck F, Möller K, Dum D, Chirico V, et al. Prevalence of syndecan-1 (CD138) expression in different kinds of human tumors and normal tissues. Dis Markers. (2019) 2019:1–11. doi: 10.1155/2019/4928315
42. Alaeddini M, Yazdani F, Etemad-Moghadam S. Stromal and epithelial syndecan-1 expression in benign and malignant salivary gland tumors: which is more reflective of behavior? Braz J Otorhinolaryngol. (2021) 87:171–7. doi: 10.1016/j.bjorl.2019.07.006
43. Stennert E, Guntinas-Lichius O, Klussmann JP, Arnold G. Histopathology of pleomorphic adenoma in the parotid gland: a prospective unselected series of 100 cases. Laryngoscope. (2001) 111:2195-2200. doi: 10.1097/00005537-200112000-00024
44. Whitelock JM, Murdoch AD, Iozzo RV, Underwood PA. The degradation of human endothelial cell-derived perlecan and release of bound basic fibroblast growth factor by stromelysin, collagenase, plasmin, and heparanases. J Biol Chem. (1996) 271:10079–86. doi: 10.1074/jbc.271.17.10079
45. Maruyama S, Cheng J, Yamazaki M, Liu A, Saku T. Keratinocyte growth factor colocalized with perlecan at the site of capsular invasion and vascular involvement in salivary pleomorphic adenomas. J Oral Pathol Med. (2009) 38:377–85. doi: 10.1111/j.1600-0714.2008.00742.x
46. Watanabe H, Cheung SC, Itano N, Kimata K, Yamada Y. Identification of hyaluronan-binding domains of aggrecan. J Biol Chem. (1997) 272:28057–65. doi: 10.1074/jbc.272.44.28057
47. Wight TN. Versican: a versatile extracellular matrix proteoglycan in cell biology. Curr Opin Cell Biol. (2002) 14:617–23. doi: 10.1016/S0955-0674(02)00375-7
48. Kuwabara H, Nishikado A, Hayasaki H, Isogai Z, Yoneda M, Kawata R, et al. Characteristic formation of hyaluronan-cartilage link protein-proteoglycan complex in salivary gland tumors. Appl Immunohistochem Mol Morphol. (2016) 24:373–81. doi: 10.1097/PAI.0000000000000198
49. Zhao M, Takata T, Ogawa I, Takekoshi T, Nikai H. Immunohistochemical demonstration of bone morphogenetic protein-2 and type II collagen in pleomorphic adenoma of salivary glands. J Oral Pathol Med. (2007) 27:293–6. doi: 10.1111/j.1600-0714.1998.tb01959.x
50. Muramatsu K, Kusafuka K, Watanabe H, Mochizuki T, Nakajima T. Ultrastructural immunolocalization of a cartilage-specific proteoglycan, aggrecan, in salivary pleomorphic adenomas. Med Mol Morphol. (2009) 42:47–54. doi: 10.1007/s00795-008-0429-4
51. Ricciardelli C, Sakko AJ, Ween MP, Russell DL, Horsfall DJ. The biological role and regulation of versican levels in cancer. Cancer Metastasis Rev. (2009) 28:233–45. doi: 10.1007/s10555-009-9182-y
52. Isacke CM, Yarwood H. The hyaluronan receptor, CD44. Int J Biochem Cell Biol. (2002) 34:718–21. doi: 10.1016/S1357-2725(01)00166-2
53. Shen S, Lu H, Liu L, Wang Y, Zhang C, Yang W, et al. Role of CD44 in tumor-initiating cells of salivary gland pleomorphic adenoma: more than a surface biomarker. Oral Dis. (2020) 26:547–57. doi: 10.1111/odi.13279
54. Ianez RCF, Coutinho-Camillo CM, Buim ME, Pinto CAL, Soares FA, Lourenco SV. CD24 And CD44 in salivary gland pleomorphic adenoma and in human salivary gland morphogenesis: differential markers of glandular structure or stem cell indicators? Histopathology. (2013) 62:1075–82. doi: 10.1111/his.12109
55. Fok TC, Lapointe H, Tuck AB, Chambers AF, Jackson-Boeters L, Daley TD, et al. Expression and localization of osteopontin, homing cell adhesion molecule/CD44, and integrin αvβ3 in pleomorphic adenoma, polymorphous low-grade adenocarcinoma, and adenoid cystic carcinoma. Oral Surg Oral Med Oral Pathol Oral Radiol. (2013) 116:743–51. doi: 10.1016/j.oooo.2013.09.004
56. Franchi A, Moroni M, Paglierani M, Santucci M. Expression of CD44 standard and variant isoforms in parotid gland and parotid gland tumours. J Oral Pathol Med. (2001) 30:564–8. doi: 10.1034/j.1600-0714.2001.300910.x
57. Xing R, Regezi J, Stern M, Shuster S, Stern R. Hyaluronan and CD44 expression in minor salivary gland tumors. Oral Dis. (2008) 4:241–7. doi: 10.1111/j.1601-0825.1998.tb00287.x
58. Soave DF, Oliveira da Costa JP, da Silveira GG, Ianez RCF, de Oliveira LR, Lourenço SV, et al. CD44/CD24 Immunophenotypes on clinicopathologic features of salivary glands malignant neoplasms. Diagn Pathol. (2013) 8:29. doi: 10.1186/1746-1596-8-29
59. Shamloo N, Taghavi N, Yazdani F, Shalpoush S, Ahmadi S. CD44 Expression in pleomorphic adenoma, carcinoma ex pleomorphic adenoma and their adjacent normal salivary glands. Dent Res J (Isfahan) (2018) 15:361–6.30233657
60. Yang S, Wang H-P, Wang X-Y, Guo L-J, Tang X-F, Gao Q-H, et al. Expression of CD44V6 in parotid pleomorphic adenoma and carcinoma ex pleomorphic adenoma. Expert Opin Investig Drugs. (2010) 19:S101–8. doi: 10.1517/13543781003718866
61. Moura JDO, Gonzaga AKG, Queiroz SIML, Martins MD, Pinto LP, Souza LD. Immunohistochemical expression of OCT4 and CD44 in major and minor salivary gland neoplasms. Braz Oral Res. (2021) 35. doi: 10.1590/1807-3107bor-2021.vol35.0073
62. Chiquet-Ehrismann R, Tucker RP. Tenascins and the importance of adhesion modulation. Cold Spring Harb Perspect Biol. (2011) 3:a004960. doi: 10.1101/cshperspect.a004960
63. Westernoff TH, Jordan RCK, Regezi JA, Ramos DM, Schmidt BL. β-6 integrin, tenascin-C, and MMP-1 expression in salivary gland neoplasms. Oral Oncol. (2005) 41:170–4. doi: 10.1016/j.oraloncology.2004.08.002
64. Soini Y, Pääkkö P, Virtanen I, Lehto V-P. Tenascin in salivary gland tumours. Virchows Arch A Pathol Anat Histopathol. (1992) 421:217–22. doi: 10.1007/BF01611178
65. Bento PM, Freitas RdA, Pinto LP, Souza Ld. Tenascin and fibronectin in pleomorphic adenoma of the salivary gland. J Appl Oral Sci. (2006) 14:198–202. doi: 10.1590/S1678-77572006000300010
66. Sunardhi-Widyaputra S, Van Damme B. Immunohistochemical expression of tenascin in normal human salivary glands and in pleomorphic adenomas. Pathol Res Pract. (1993) 189:138–43. doi: 10.1016/S0344-0338(11)80083-X
67. Raitz R, Martins MD, Araújo VC. A study of the extracellular matrix in salivary gland tumors. J Oral Pathol Med. (2003) 32:290–6. doi: 10.1034/j.1600-0714.2003.00019.x
68. Shrestha P, Sumitomo S, Ogata K, Yamada K, Takai Y, Yang L, et al. Immunoreactive tenascin in tumours of salivary glands: evidence for enhanced expression in tumour stroma and production by tumour cells. Eur J Cancer B Oral Oncol. (1994) 30B:393–9. doi: 10.1016/0964-1955(94)90018-3
69. Araujo VD, Furuse C, Cury PR, Altemani A, Alves VAF, de Araujo NS. Tenascin and fibronectin expression in carcinoma ex pleomorphic adenoma. Appl Immunohistochem Mol Morphol AIMM. (2008) 16:48–53. doi: 10.1097/PAI.0b013e31802eff1c
70. Felix A, Rosa JC, Fonseca I, Cidadao A, Soares J. Pleomorphic adenoma and carcinoma ex pleomorphic adenoma: immunohistochemical demonstration of the association between tenascin expression and malignancy. Histopathology. (2004) 45:187–92. doi: 10.1111/j.1365-2559.2004.01924.x
71. Heino J. The collagen family members as cell adhesion proteins. Bioessays. (2007) 29:1001–10. doi: 10.1002/bies.20636
72. Zhang J, Liu J, Zhang H, Wang J, Hua H, Jiang Y. The role of network-forming collagens in cancer progression. Int J Cancer. (2022) 151:833-42. doi: 10.1002/ijc.34004
73. Xu S, Xu H, Wang W, Li S, Li H, Li T, et al. The role of collagen in cancer: from bench to bedside. J Transl Med. (2019) 17:309. doi: 10.1186/s12967-019-2058-1
74. Line SRP, Torloni H, Junqueira LCU. Diversity of collagen expression in the pleomorphic adenoma of the parotid gland. Virchows Arch A Pathol Anat Histopathol. (1989) 414:477–83. doi: 10.1007/BF00781704
75. Skalova A, Leivo I, Michal M, Saksela E. Analysis of collagen isotypes in crystalloid structures of salivary gland tumors. Hum Pathol. (1992) 23:748–54. doi: 10.1016/0046-8177(92)90343-2
76. Landini G. Immunohistochemical demonstration of type II collagen in the chondroid tissue of pleomorphic adenomas of the salivary glands. Pathol Int. (2008) 41:270–6. doi: 10.1111/j.1440-1827.1991.tb03355.x
77. Wetzels RH, Velden Lvd, Schaafsma HE, Manni JJ, Leigh IM, Vooijs GP, et al. Immunohistochemical localization of basement membrane type VII collagen and laminin in neoplasms of the head and neck. Histopathology. (1992) 21:459–64. doi: 10.1111/j.1365-2559.1992.tb00431.x
78. Nissen NI, Karsdal M, Willumsen N. Collagens and cancer associated fibroblasts in the reactive stroma and its relation to cancer biology. J Exp Clin Cancer Res. (2019) 38:115. doi: 10.1186/s13046-019-1110-6
79. Kinoshita I, Jin D, Higashino M, Terada T, Kurisu Y, Takai S, et al. Increase in chymase-positive mast cells in recurrent pleomorphic adenoma and carcinoma ex pleomorphic adenoma of the parotid gland. Int J Mol Sci. (2021) 22:12613. doi: 10.3390/ijms222312613
80. Feng B, Wu J, Shen B, Jiang F, Feng J. Cancer-associated fibroblasts and resistance to anticancer therapies: status, mechanisms, and countermeasures. Cancer Cell Int. (2022) 22:166. doi: 10.1186/s12935-022-02599-7
81. Araújo Vd, Demasi APD, Furuse C, Altemani A, Alves VA, Freitas LL, et al. Collagen type I may influence the expression of E-cadherin and Beta-catenin in carcinoma ex-pleomorphic adenoma. Appl Immunohistochem Mol Morphol. (2009) 17:312–8. doi: 10.1097/PAI.0b013e3181946ea6
82. Badaoui M, Mimsy-Julienne C, Saby C, Van Gulick L, Peretti M, Jeannesson P, et al. Collagen type 1 promotes survival of human breast cancer cells by overexpressing Kv10.1 potassium and Orai1 calcium channels through DDR1-dependent pathway. Oncotarget. (2018) 9:24653–71. doi: 10.18632/oncotarget.19065
83. Barcus CE, O’Leary KA, Brockman JL, Rugowski DE, Liu Y, Garcia N, et al. Elevated collagen-I augments tumor progressive signals, intravasation and metastasis of prolactin-induced estrogen receptor alpha positive mammary tumor cells. Breast Cancer Res. (2017) 19:9. doi: 10.1186/s13058-017-0801-1
84. Félix A, Costa Rosa J, Fonseca I, Cidadão A, Soares J. Laminin and collagen IV in pleomorphic adenoma and carcinoma ex-pleomorphic adenoma: an immunohistochemical study. Hum Pathol. (1999) 30:964–9. doi: 10.1016/S0046-8177(99)90251-4
85. Rolff HC, Christensen IJ, Vainer B, Svendsen LB, Eefsen RL, Wilhelmsen M, et al. The prognostic and predictive value of soluble type IV collagen in colorectal cancer: a retrospective multicenter study. Clin Cancer Res. (2016) 22:2427–34. doi: 10.1158/1078-0432.CCR-15-2342
86. Lindgren M, Jansson M, Tavelin B, Dirix L, Vermeulen P, Nyström H. Type IV collagen as a potential biomarker of metastatic breast cancer. Clin Exp Metastasis. (2021) 38:175–85. doi: 10.1007/s10585-021-10082-2
87. Hamidi H, Ivaska J. Every step of the way: integrins in cancer progression and metastasis. Nat Rev Cancer. (2018) 18:533–48. doi: 10.1038/s41568-018-0038-z
88. Schumacher S, Dedden D, Nunez RV, Matoba K, Takagi J, Biertümpfel C, et al.. Structural insights into integrin α5β1 opening by fibronectin ligand. Sci Adv. (2021) 7:eabe9716. doi: doi: 10.1126/sciadv.abe9716
89. Hynes RO. Integrins: bidirectional, allosteric signaling machines. Cell. (2002) 110:673–87. doi: 10.1016/s0092-8674(02)00971-6
90. Desgrosellier JS, Cheresh DA. Integrins in cancer: biological implications and therapeutic opportunities. Nat Rev Cancer. (2010) 10:9–22. doi: 10.1038/nrc2748
91. Su CY, Li JQ, Zhang LL, Wang H, Wang F, Tao Y, et al.. The Biological Functions and Clinical Applications of Integrins in Cancers. Front Pharmacol. (2020) 11:579068. doi: 10.3389/fphar.2020.579068
92. Sunardhi-Widyaputra S, Van Damme B. Distribution of the VLA family of integrins in normal salivary gland and in pleomorphic adenoma. Pathol Res Pract. (1994) 190:600–8. doi: 10.1016/S0344-0338(11)80398-5
93. Caselitz J, Schmitt P, Seifert G, Wustrow J, Schuppan D. Basal membrane associated substances in human salivary glands and salivary gland tumours. Pathol Res Pract. (1988) 183:386–94. doi: 10.1016/S0344-0338(88)80084-0
94. Lourenco SV, Kapas S, Williams DM, Leite K, Araujo VC. Expression patterns of integrins on pleomorphic adenoma and adenoid cystic carcinoma: study on specimens and in vitro investigation of the effects of extracellular matrix on the expression of these adhesion molecules. J Oral Pathol Med. (2004) 33:574–80. doi: 10.1111/j.1600-0714.2004.00239.x
95. Frenette PS, Wagner DD. Adhesion molecules. N Engl J Med. (1996) 334:1526–9. doi: 10.1056/NEJM199606063342308
96. Silva CAB, Martinez EF, Demasi APD, Altemani A, da Silveira Bossonaro JP, Araújo NS, et al. Cellular senescence and autophagy of myoepithelial cells are involved in the progression of in situ areas of carcinoma ex-pleomorphic adenoma to invasive carcinoma. An in vitro model. J Cell Commun Signal. (2015) 9:255–65. doi: 10.1007/s12079-015-0291-9
97. Bergonzini C, Kroese K, Zweemer AJM, Danen EHJ. Targeting Integrins for Cancer Therapy - Disappointments and Opportunities. Front Cell Dev Biol. (2022) 10:863850. doi: doi: 10.3389/fcell.2022.863850
98. Rousselle P, Scoazec JY. Laminin 332 in cancer: when the extracellular matrix turns signals from cell anchorage to cell movement. Semin Cancer Biol. (2020) 62:149–65. doi: 10.1016/j.semcancer.2019.09.026
99. Kleinman HK, Weeks BS, Schnaper HW, Kibbey MC, Yamamura K, Grant DS. The laminins: a family of basement membrane glycoproteins important in cell differentiation and tumor metastases. Vitam Horm. (1993) 47:161-86. doi: 10.1016/s0083-6729(08)60446-x
100. Iorio V, Troughton LD, Hamill KJ. Laminins: roles and utility in wound repair. Adv Wound Care. (2015) 4:250–63. doi: 10.1089/wound.2014.0533
101. Miner JH, Yurchenco PD. Laminin functions in tissue morphogenesis. Annu Rev Cell Dev Biol. (2004) 20:255–84. doi: 10.1146/annurev.cellbio.20.010403.094555
103. Troughton LD, O’Loughlin DA, Zech T, Hamill KJ. Laminin N-terminus α31 is upregulated in invasive ductal breast cancer and changes the mode of tumour invasion. PLoS One. (2022) 17:e0264430. doi: 10.1371/journal.pone.0264430
104. Iwamuro M, Shiraha H, Kobashi M, Horiguchi S, Okada H. Laminin 511-E8 fragment offers superior adhesion properties for gastric cancer cells compared with full-length laminin 511. Curr Issues Mol Biol. (2022) 44:1539–51. doi: 10.3390/cimb44040105
105. Hao N, Yang D, Liu T, Liu S, Lu X, Chen L. Laminin-integrin a6b4 interaction activates notch signaling to facilitate bladder cancer development. BMC Cancer. (2022) 22:558. doi: 10.1186/s12885-022-09645-7
106. Toida M, Takeuchi J, Hara K, Sobue M, Tsukidate K, Goto K, et al. Histochemical studies of intercellular components of salivary gland tumors with special reference to glycosaminoglycan, laminin and vascular elements. Virchows Arch A Pathol Anat Histopathol. (1984) 403:15–26. doi: 10.1007/BF00689335
107. de Araújo VC, Altemani A, Furuse C, Martins MT, de Araújo NS. Immunoprofile of reactive salivary myoepithelial cells in intraductal areas of carcinoma ex-pleomorphic adenoma. Oral Oncol. (2006) 42:1011–6. doi: 10.1016/j.oraloncology.2005.12.021
108. Pankov R, Yamada KM. Fibronectin at a glance. J Cell Sci. (2002) 115:3861–3. doi: 10.1242/jcs.00059
109. Larsen M, Wei C, Yamada KM. Cell and fibronectin dynamics during branching morphogenesis. J Cell Sci. (2006) 119:3376–84. doi: 10.1242/jcs.03079
110. Zhou W-H, Du W-D, Li Y-F, Al-Aroomi MA, Yan C, Wang Y, et al. The overexpression of fibronectin 1 promotes cancer progression and associated with M2 macrophages polarization in head and neck squamous cell carcinoma patients. Int J Gen Med. (2022) 15:5027–42. doi: 10.2147/IJGM.S364708
111. Jun BH, Guo T, Libring S, Chanda MK, Paez JS, Shinde A, et al. Fibronectin-expressing mesenchymal tumor cells promote breast cancer metastasis. Cancers (Basel). (2020) 12:2553. doi: 10.3390/cancers12092553
112. Ghura H, Keimer M, von Au A, Hackl N, Klemis V, Nakchbandi IA. Inhibition of fibronectin accumulation suppresses tumor growth. Neoplasia. (2021) 23:837–50. doi: 10.1016/j.neo.2021.06.012
113. Lin T-C, Yang C-H, Cheng L-H, Chang W-T, Lin Y-R, Cheng H-C. Fibronectin in cancer: friend or foe. Cells. (2019) 9:27. doi: 10.3390/cells9010027
114. Han Z, Lu Z-R. Targeting fibronectin for cancer imaging and therapy. J Mater Chem B. (2017) 5:639–54. doi: 10.1039/C6TB02008A
115. Halbleib JM, Nelson WJ. Cadherins in development: cell adhesion, sorting, and tissue morphogenesis. Genes Dev. (2006) 20:3199–214. doi: 10.1101/gad.1486806
116. Wijnhoven BPL, Dinjens WNM, Pignatelli M. E-cadherin—catenin cell—cell adhesion complex and human cancer. Br J Surg. (2002) 87:992–1005. doi: 10.1046/j.1365-2168.2000.01513.x
117. Karsten N, Kolben T, Mahner S, Beyer S, Meister S, Kuhn C, et al. The role of E-cadherin expression in primary site of breast cancer. Arch Gynecol Obstet. (2022) 305:913–20. doi: 10.1007/s00404-021-06198-1
118. Rätze MAK, Koorman T, Sijnesael T, Bassey-Archibong B, van de Ven R, Enserink L, et al. Loss of E-cadherin leads to Id2-dependent inhibition of cell cycle progression in metastatic lobular breast cancer. Oncogene. (2022) 41:2932–44. doi: 10.1038/s41388-022-02314-w
119. Na T-Y, Schecterson L, Mendonsa AM, Gumbiner BM. The functional activity of E-cadherin controls tumor cell metastasis at multiple steps. Proc Natl Acad Sci. (2020) 117:5931–7. doi: 10.1073/pnas.1918167117
120. Prabhu S, Kaveri H, Rekha K. Benign ; malignant salivary gland tumors: comparison of immunohistochemical expression of e-cadherin. Oral Oncol. (2009) 45:594–9. doi: 10.1016/j.oraloncology.2008.08.004
121. Economopoulou P, Hanby A, Odell EW. Expression of E-cadherin, cellular differentiation and polarity in epithelial salivary neoplasms. Oral Oncol. (2000) 36:515–8. doi: 10.1016/S1368-8375(00)00043-9
122. Xia L, Hu Y, Gu T, Wang L, Tian Z. Promoter hypermethylation may contribute to E-cadherin repression in the human salivary carcinoma ex pleomorphic adenoma. Int J Oncol. (2018) 52:496-04. doi: 10.3892/ijo.2017.4210
123. Brieger J, Duesterhoeft A, Brochhausen C, Gosepath JAN, Kirkpatrick CJ, Mann WJ. Recurrence of pleomorphic adenoma of the parotid gland-predictive value of cadherin-11 and fascin. APMIS. (2008) 116:1050–7. doi: 10.1111/j.1600-0463.2008.01088.x
124. Perl A-K, Wilgenbus P, Dahl U, Semb H, Christofori G. A causal role for E-cadherin in the transition from adenoma to carcinoma. Nature. (1998) 392:190–3. doi: 10.1038/32433
125. Genelhu MCLS, Gobbi H, Arantes DCB, Cardoso S V, Cassali GD. Immunolocalization of β-catenin in pleomorphic adenomas and carcinomas ex-pleomorphic adenomas of salivary glands. Appl Immunohistochem Mol Morphol. (2007) 15:273–8. doi: 10.1097/01.pai.0000213123.04215.95
126. Conlon GA, Murray GI. Recent advances in understanding the roles of matrix metalloproteinases in tumour invasion and metastasis. J Pathol. (2019) 247:629–40. doi: 10.1002/path.5225
127. Danø K, Rømer J, Nielsen BS, Bjørn S, Pyke C, Rygaard J, et al. Cancer invasion and tissue remodeling-cooperation of protease systems and cell types. APMIS. (1999) 107:120–7. doi: 10.1111/j.1699-0463.1999.tb01534.x
128. Borkham-Kamphorst E, Alexi P, Tihaa L, Haas U, Weiskirchen R. Platelet-derived growth factor-D modulates extracellular matrix homeostasis and remodeling through TIMP-1 induction and attenuation of MMP-2 and MMP-9 gelatinase activities. Biochem Biophys Res Commun. (2015) 457:307–13. doi: 10.1016/j.bbrc.2014.12.106
129. Verma RP, Hansch C. Matrix metalloproteinases (MMPs): chemical–biological functions and (Q)SARs. Bioorg Med Chem. (2007) 15:2223–68. doi: 10.1016/j.bmc.2007.01.011
130. Jackson HW, Defamie V, Waterhouse P, Khokha R. TIMPs: versatile extracellular regulators in cancer. Nat Rev Cancer. (2017) 17:38–53. doi: 10.1038/nrc.2016.115
131. Bergamaschi A, Tagliabue E, Sørlie T, Naume B, Triulzi T, Orlandi R, et al. Extracellular matrix signature identifies breast cancer subgroups with different clinical outcome. J Pathol. (2008) 214:357–67. doi: 10.1002/path.2278
132. Huang C, Xu S, Luo Z, Li D, Wang R, Wang T. Epidemiological Evidence Between Variants in Matrix Metalloproteinases-2, -7, and -9 and Cancer Risk. Front Oncol. (2022)12:856831. doi: 10.3389/fonc.2022.856831
133. Lipari L, Mauro A, Gallina S, Tortorici S, Buscemi M, Tetè S, et al. Expression of gelatinases (MMP-2, MMP-9) and cyclooxygenases (COX-1, COX-2) in some benign salivary gland tumors. Int J Immunopathol Pharmacol. (2012) 25:107–15. doi: 10.1177/039463201202500113
134. Nagel H, Laskawi R, Wahlers A, Hemmerlein B. Expression of matrix metalloproteinases MMP-2, MMP-9 and their tissue inhibitors TIMP-1, -2, and -3 in benign and malignant tumours of the salivary gland. Histopathology. (2004) 44:222–31. doi: 10.1111/j.0309-0167.2004.01814.x
135. de Vicente JC, Lequerica-Fernández P, López-Arranz JS, Esteban I, Fresno MF, Astudillo A. Expression of matrix metalloproteinase-9 in high-grade salivary gland carcinomas is associated with their metastatic potential. Laryngoscope. (2008) 118:247–51. doi: 10.1097/MLG.0b013e318158f754
136. Luukkaa H, Klemi P, Leivo I, Makitie AA, Irish J, Gilbert R, et al. Expression of matrix metalloproteinase-1, -7, -9, -13, ki-67, and HER-2 in epithelial-myoepithelial salivary gland cancer. Head Neck. (2010) 32:1019–27. doi: 10.1002/hed.21277
137. Luukkaa H, Klemi P, Hirsimäki P, Vahlberg T, Kivisaari A, Kähäri V-M, et al. Matrix metalloproteinase (MMP)-1, -9 and -13 as prognostic factors in salivary gland cancer. Acta Otolaryngol. (2008) 128:482–90. doi: 10.1080/00016480801922895
138. Huang Z-Q, Chen W, Li H-G, Li J-S, Xu Z-Y, Lin Z-Y. Extracellular matrix metalloproteinase inducer expression in salivary gland tumors. J Craniofac Surg. (2010) 21:1855–60. doi: 10.1097/SCS.0b013e3181f43f7e
139. Falcao ASC, Kataoka MdS, Ribeiro NAB, Diniz JAPJ, Alves SMJ, Ribeiro ALR, et al. A novel cell line derived from pleomorphic adenoma expresses MMP2, MMP9, TIMP1, TIMP2, and shows numeric chromosomal anomalies. PLoS One. (2014) 9:e105231. doi: 10.1371/journal.pone.0105231
140. Kochurova EV. Comparative role of matrixins in diagnostics of parotid gland tumors. Bull Exp Biol Med. (2019) 166:383–5. doi: 10.1007/s10517-019-04355-w
141. Zhang X, Wang Y, Yamamoto G, Tachikawa T. Expression of matrix metalloproteinases MMP-2, MMP-9 and their tissue inhibitors TIMP-1 and TIMP-2 in the epithelium and stroma of salivary gland pleomorphic adenomas. Histopathology. (2009) 55:250–60. doi: 10.1111/j.1365-2559.2009.03355.x
142. Navarini NF, Araujo Vd, Brown AL, Passador-Santos F, Souza Id, Napimoga MH, et al. The EGF signaling pathway influences cell migration and the secretion of metalloproteinases by myoepithelial cells in pleomorphic adenoma. Tumour Biol. (2015) 36:205–11. doi: 10.1007/s13277-014-2624-3
143. Navarini NF, De Araújo VC, Sperandio M, Napimoga MH, Teixeira LN, De Araújo NS, et al. Effect of epithelial growth factor on matrix metalloproteinase-2 and E-cadherin/β-catenin expression in an in situ model of tumorigenesis. Oncol Lett. (2017) 14:3136–40. doi: 10.3892/ol.2017.6513
144. Martinez EF, de Araújo VC, Navarini NF, de Souza IF, Rena GB, Demasi APD, et al. Microvesicles derived from squamous cell carcinoma induce cell death, autophagy, and invasion of benign myoepithelial cells. J Oral Pathol Med. (2020) 49:761–70. doi: 10.1111/jop.13037
145. Souza Freitas V, Dos Santos JN, de Andrade Santos PP, Nonaka CFW, Pereira Pinto L, de Souza LB. Expression of matrix metalloproteinases (MMPs-2, -7, -9, and -26) and tissue inhibitors of metalloproteinases (TIMPs-1 and -2) in pleomorphic adenomas and adenoid cystic carcinomas. Eur Arch Otorhinolaryngol. (2018) 275:3075–82. doi: 10.1007/s00405-018-5176-0
Keywords: extracellular matrix (ECM), pleomorphic adenoma (PA), carcinogenesis, carcinoma ex pleomorphic adenoma (CXPA), review
Citation: Scarini JF, de Lima-Souza RA, Lavareze L, Ribeiro de Assis MCF, Damas II, Altemani A, Egal ESA, dos Santos JN, Bello IO and Mariano FV (2023) Heterogeneity and versatility of the extracellular matrix during the transition from pleomorphic adenoma to carcinoma ex pleomorphic adenoma: cumulative findings from basic research and new insights. Front. Oral. Health 4:942604. doi: 10.3389/froh.2023.942604
Received: 12 May 2022; Accepted: 17 March 2023;
Published: 17 April 2023.
Edited by:
Ali-Farid Safi, Craniologicum—Center for Craniomaxillofacial Surgery, SwitzerlandReviewed by:
Martin Kauke-Navarro, Yale New Haven Health System, United States© 2023 Scarini, de Lima-Souza, Lavareze, Ribeiro de Assis, Damas, Altemani, Egal, dos Santos, Bello and Mariano. This is an open-access article distributed under the terms of the Creative Commons Attribution License (CC BY). The use, distribution or reproduction in other forums is permitted, provided the original author(s) and the copyright owner(s) are credited and that the original publication in this journal is cited, in accordance with accepted academic practice. No use, distribution or reproduction is permitted which does not comply with these terms.
*Correspondence: Fernanda Viviane Mariano Zm1hcmlhbm9AdW5pY2FtcC5icg==
Specialty Section: This article was submitted to Oral Cancers, a section of the journal Frontiers in Oral Health
Disclaimer: All claims expressed in this article are solely those of the authors and do not necessarily represent those of their affiliated organizations, or those of the publisher, the editors and the reviewers. Any product that may be evaluated in this article or claim that may be made by its manufacturer is not guaranteed or endorsed by the publisher.
Research integrity at Frontiers
Learn more about the work of our research integrity team to safeguard the quality of each article we publish.