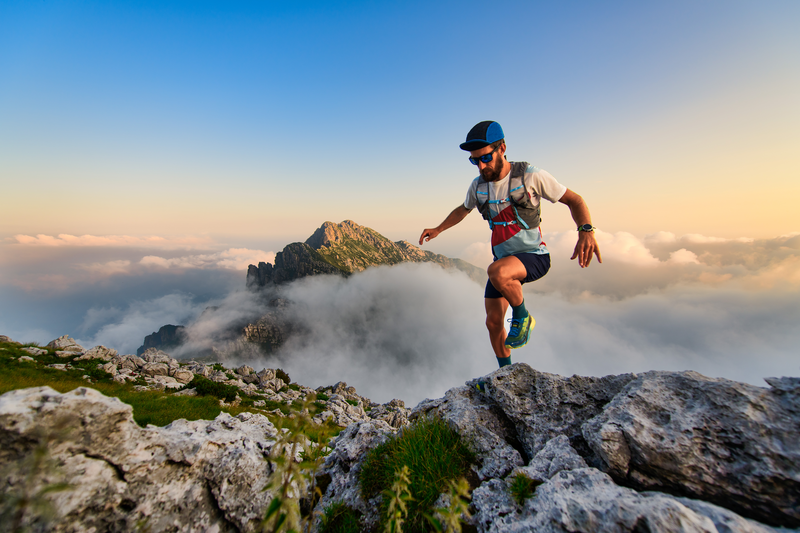
94% of researchers rate our articles as excellent or good
Learn more about the work of our research integrity team to safeguard the quality of each article we publish.
Find out more
ORIGINAL RESEARCH article
Front. Oral. Health , 16 June 2022
Sec. Oral Infections and Microbes
Volume 3 - 2022 | https://doi.org/10.3389/froh.2022.923663
This article is part of the Research Topic The Impact of Inflammation on the Oral Microbiome View all 6 articles
Porphyromonas gingivalis expresses a broad array of virulence factors that enable it to play a central role in the etiopathogenesis of periodontitis. The objective of the present study was to assess the effects of a berry polyphenolic fraction (Orophenol®) composed of extracts from cranberry, wild blueberry, and strawberry on the main pathogenic determinants of P. gingivalis. Orophenol® attenuated the growth of P. gingivalis and decreased its hemolytic activity, its adherence to a basement membrane matrix model, and its proteinase activities. The berry polyphenolic fraction also impaired the production of reactive oxygen species (ROS) by oral keratinocytes stimulated with P. gingivalis. Lastly, using an in vitro model of oral keratinocyte barrier, the fraction exerted a protective effect against the damages mediated by P. gingivalis. In conclusion, the berry polyphenolic fraction investigated in the present study attenuated several pathogenic properties of P. gingivalis. Although future clinical investigations are required, our study provided evidence that the polyphenols contained in this fraction may represent bioactive molecules of high interest for the prevention and/or treatment of periodontal disease.
The Gram-negative strictly anaerobic bacterium Porphyromonas gingivalis is a member of the human oral microbiome and can be regarded as an opportunistic pathogen [1]. P. gingivalis can promote a state of dysbiosis resulting in an imbalance between beneficial commensal bacteria and periodontopathogenic bacteria [2]. It is often isolated concomitantly with Tannerella forsythia and Treponema denticola, forming the red complex initially described by Ximenez-Fyvie et al. [3], and is strongly linked with the chronic form of periodontitis. This bacteria-induced inflammatory disease, which affects tooth-supporting tissues, is characterized by the formation of periodontal pocket, along with loss of clinical attachment and resorption of the alveolar bone. Over the past decade, studies have established a link between P. gingivalis and several systemic disorders, including atherosclerosis, Alzheimer's disease, and rheumatoid arthritis [4, 5]. The ability of this periodontal pathogen to invade the ulcerated oral epithelium and translocate from the oral cavity to distant tissues or organs might explain P. gingivalis-associated systemic disorders [4, 5].
P. gingivalis expresses a broad array of potential virulence factors that are involved in subgingival colonization, immune defense evasion, connective tissue destruction, and exaggerated inflammatory responses [6, 7]. The pathogenic factors produced by P. gingivalis include lipopolysaccharides (LPS), adhesins, hemolysins, and proteinases [6, 7]. Gingipains, which are either secreted or anchored to the outer membrane, are the main proteinases produced by P. gingivalis [8, 9]. Targeting virulence factors is a promising strategy for specifically fighting bacterial pathogens without disturbing the commensal microbiota that often has a beneficial impact [10, 11].
Although a satisfactory therapeutic outcome is commonly achieved with a conventional mechanical treatment, controlling periodontitis in high-risk subjects (smokers, diabetics, elderly populations, etc.) or patients with an aggressive form of the disease often needs an adjunctive treatment with systemic or locally applied antibiotics that may lead to the emergence of bacterial resistance [12, 13]. In recent decades, research has been directed at discovering natural compounds that display beneficial properties with respect to periodontal health as efficient alternatives to conventional antibiotics [14–16]. Polyphenols refer to different families of compounds in edible plants such as fruits and vegetables and with which diverse human health benefits have been linked [17]. These plant secondary metabolites are well-known to possess antibacterial, anti-adhesion, and anti-inflammatory activities that may be relevant with respect to periodontal health [14–18]. Wild blueberries (Vaccinium angustifolium), cranberries (Vaccinium macrocarpon), and strawberries (Fragaria virginiana) contain high amounts of phenolic acids, anthocyanins, and proanthocyanidins [19]. Previous reports have brought clear evidence that proanthocyanidins from berry fruits exhibit anti-biofilm and anti-adhesion properties against important oral pathogens [16, 20, 21]. The objective of the present study was to assess a berry polyphenolic preparation (Orophenol®) composed of extracts from cranberry, wild blueberry, and strawberry for its effect on the pathogenic properties of the periodontal pathogen P. gingivalis.
The berry polyphenolic fraction, commercialized as Orophenol®, was kindly provided by the manufacturer Diana Food Canada Inc. (Champlain, QC, Canada). This fraction contains polyphenol-rich soluble extracts prepared from three berry fruits (cranberry, wild blueberry, strawberry). A stock solution was prepared in distilled water at a concentration of 10 mg/ml, was filter-sterilized (0.2-μm pore size membrane filter), and was kept at 4°C under darkness for up to 1 month. The phenolic content of the preparation was analyzed by chromatographic and mass spectrometry techniques and has been detailed in a previous study [22]. In brief, phenolic acids, flavonoids (flavonols, anthocyanins, flavan-3-ols), and procyanidins made up 10.71, 19.76, and 5.29% (w/w) of the berry polyphenolic preparation, respectively.
Porphyromonas gingivalis ATCC 33277 was grown in Todd-Hewitt broth (THB; Becton Dickinson and Company, Sparks, MD, United States) containing 0.001% (w/v) hemin and 0.0001% (w/v) vitamin K and was incubated at 37°C in an anaerobic atmosphere (80% N2, 10% CO2, and 10% H2).
The effect of the berry polyphenolic fraction on the growth of P. gingivalis was assessed with a broth microdilution method. Briefly, a 24-h culture of P. gingivalis was diluted in fresh medium to an optical density at 660 nm (OD660) of 0.1, which corresponds to a concentration of 1 × 108 colony forming units (CFU)/mL. Equal volumes (100 μl) of the bacterial culture and two-fold serial dilutions of the berry polyphenolic fraction (2,000–7.81 μg/ml) in fresh medium were mixed in the wells of a 96-well tissue culture treated, flat-bottom, microplate (Sarstedt, Newton, NC, United States). Wells without bacteria or fraction were used as controls. The microplate was incubated for 24 h under anaerobic conditions prior to assessing bacterial growth by recording the OD660 using a Synergy 2 microplate reader (BioTek Instruments, Winooski, VT, United States).
Sheep blood (Nutri-Bact, Terrebonne, QC, Canada) was centrifuged (600 × g for 5 min) to harvest erythrocytes, which were washed three times in 50 mM phosphate-buffered saline (pH 7.2, PBS) and suspended [2% (v/v)] in PBS. Equal volumes (1 ml) of the red blood cell suspension, the P. gingivalis suspension (OD660 = 2.0 in PBS; corresponding to to a concentration of 2 × 109 CFU/ml), and the berry polyphenolic fraction (final concentrations of 0, 1.95, 3.9, 7.81, 15.625, and 31.25 μg/ml) were mixed and were incubated at 37°C for 16 h and then at 4°C for 1 h. The assay mixtures were centrifuged (10,000 × g for 5 min), and the absorbance of the supernatants was monitored at 540 nm (A540). Hemolysis induced by P. gingivalis in the absence of the berry polyphenolic fraction was given a value of 100%.
The effect of the berry polyphenolic fraction on the ability of P. gingivalis to adhere to Matrigel® (BD Biosciences, Franklin Lakes, NJ, United States), an in vitro basement membrane matrix model, was investigated. First, bacterial cells were labeled with fluorescein isothiocyanate (FITC) as previously described [23]. P. gingivalis was incubated (2 h) with the Matrigel®, contained into wells of a 96-well microplate, in the presence or absence of the berry polyphenolic fraction (1,000 to 15.625 μg/ml). The wells were then washed three times with PBS and bound bacterial cells were estimated by recording the relative fluorescence units (RUF; excitation wavelength 495 nm; emission wavelength 525 nm) using a Synergy 2 microplate reader (BioTek Instruments). A 100% value was assigned to bacterial adherence determined in the absence of the berry polyphenolic fraction.
The effect of the berry polyphenolic fraction on the P. gingivalis collagenase activity was investigated by mixing a 48-h bacterial culture supernatant with fluorescent collagen DQ (Molecular Probes, Eugene, OR, United States) (100 μg/ml) in the presence of the fraction (final concentrations in the assay: 31.25, 62.5, 125, 250 μg/ml) in the wells of a microplate with a clear bottom and black walls (Greiner Bio-One North America, Monroe, NC, United States). After a 60-min incubation at 37°C, the fluorescence (Ex: 495 nm/Em: 535 nm) resulting from collagen degradation was monitored using a Synergy 2 microplate reader (BioTek Instruments). The berry polyphenolic fraction or the fluorescent substrate alone served as controls. Leupeptin (100 μg/ml) was included as a known inhibitor of P. gingivalis collagenase. Collagenase activity monitored in the absence of the fraction was given a value of 100%.
To investigate the effect of the berry polyphenolic fraction on the activities of P. gingivalis Arg- and Lys-gingipains, a bacterial cell suspension (OD660 of 0.4 in PBS; corresponding to to a concentration of 4 × 108 CFU/ml) was incubated at 37°C in the wells of a 96-well microplate together with 10 mM dithiothreitol and 5 mM of either N-α-benzoyl-DL-arginine-p-nitroanilide (Arg-gingipain substrate) or N-p-tosyl-glycine-proline-lysine-p-nitroanilide (Lys-gingipain substrate) and in the presence or absence of the fraction (final concentrations in the assay: 1.95, 3.91, 7.81, 15.625, 31.25, 62.5, 125, 250 μg/ml). The incubation (37°C) was carried out for 60 min (Arg-gingipain) or 18 h (Lys-gingipain). Cleavage of the gingipain substrates was quantified by monitoring the absorbance at 405 nm (A405) of the cell-free supernatants. Tosyl-L-lysine chloromethyl ketone hydrochloride (TLCK; 4 mM) was included as a positive gingipain inhibitor. Gingipain activity determined in the absence of the berry polyphenolic fraction was assigned a value of 100%.
The effect of the berry polyphenolic fraction (31.25, 62.5, 125, 250 μg/ml) on the catalytic activity of matrix metalloproteinase (MMP)-9 was investigated using a commercial MMP-9 Inhibitor Screening Fluorometric Assay Kit (Abcam Inc., Toronto, ON, Canada). MMP-9 activity was determined after a 20-min incubation at 37°C by monitoring fluorescence (Ex: 328 nm/Em: 420 nm) using a Synergy 2 microplate reader (BioTek Instruments). N-isobutyl-N-(4-methoxyphenylsulfonyl) glycyl hydroxamic acid (NNGH; 1 mM) served as a positive inhibitor control of MMP-9. A 100% value was assigned to the activity obtained in the absence of the berry polyphenolic fraction.
The human oral keratinocyte cell line B11 [24] was generously provided by S. Groeger and J. Meyle (Justus-Lieig-University Giessen, Germany). The cells were cultivated in keratinocyte serum-free medium (K-SFM; Life Technologies Inc., Burlington, ON, Canada) enriched with bovine pituitary extract (50 μg/ml), recombinant epidermal growth factor (5 ng/ml), and penicillin G-streptomycin (100 μg/ml). The ability of the berry polyphenolic fraction to impair the capacity of P. gingivalis to induce reactive oxygen species (ROS) production in oral keratinocytes was investigated using a fluorometric method that monitors the oxidation of 2', 7'-dichlorofluorescein-diacetate (DCF-DA (40 mM in DMSO); Sigma-Aldrich Canada Co., Oakville, ON, Canada) into a fluorescent compound. B11 oral keratinocytes were seeded in the wells (105 cells/well) of a 96-well microplate with black walls and a clear flat bottom (Greiner Bio-One North America) and were incubated overnight at 37°C in a 5% CO2 atmosphere. The cells were washed with Hank's balanced salt solution (HBSS; HyClone Laboratories, Logan, UT, United States) prior to adding 100 μM DCF-DA in HBSS. After 30 min, the keratinocytes were washed twice with HBSS prior to treating with P. gingivalis (in HBSS) at a multiplicity of infection (MOI) of 103 in the absence or presence of the berry polyphenolic fraction (500–7.81 μg/ml). The fluorescence emission at 528 nm, following excitation at 485 nm, corresponding to ROS production was monitored after a 6-h incubation at 37°C using a Synergy 2 microplate reader (BioTek Instruments). A 100% value was assigned to ROS production induced by P. gingivalis in the absence of the fraction.
The protective effect of the berry polyphenolic fraction (500–7.81 μg/ml) against P. gingivalis (MOI of 103)-mediated deleterious effects on oral keratinocyte barrier function was investigated using the B11 cell line according to the procedure described in a previous study [25]. The barrier integrity of the keratinocyte model was assessed by recording the transepithelial electrical resistance (TEER) after a 24-h incubation (37°C/5% CO2) using an ohmmeter (EVOM2, World Precision Instruments, Sarasota, FL, United States). A relative value of 100% was assigned to the TEER recorded in the absence of P. gingivalis and the fraction.
Unless indicated otherwise, all assays were carried out in triplicate in three independent experiments, and the means ± standard deviations (SD) were calculated. Data were analyzed for signifiance using a one-way ANOVA analysis of variance with a post hoc Bonferroni multiple comparison test (GraphPad Software Inc., San Diego, CA, United States). Results were considered statistically significant at P < 0.01.
The effect of the berry polyphenolic fraction on the growth of P. gingivalis ATCC 33277 was examined using a broth microdilution method. Figure 1 shows that the fraction reduced the growth of P. gingivalis in a dose-dependent manner. Significant inhibitions were obtained with concentrations of the berry polyphenolic fraction ≥500 μg/ml. At the highest concentration tested (2,000 μg/ml), the fraction lowered the bacterial growth by 86.7%.
Figure 1. Effect of the berry polyphenolic fraction on the growth of P. gingivalis. A relative value of 100% was assigned to growth in the absence of the berry polyphenolic fraction. Results are expressed as the means ± SD of triplicate assays from three independent experiments. *Significant inhibition (p < 0.01) compared to the control.
The effect of the berry polyphenolic fraction on the hemolytic activity of P. gingivalis was then investigated. As shown in Figure 2, the fraction dose-dependently inhibited hemolysis of sheep erythrocytes. Complete inhibition was obtained with the berry polyphenolic fraction at a concentration of 31.25 μg/ml.
Figure 2. Effect of the berry polyphenolic fraction on the hemolysis of sheep red blood cells by P. gingivalis. A relative value of 100% was assigned to the hemolysis of sheep red blood cells in the absence of the berry polyphenolic fraction. Results are expressed as the means ± SD of triplicate assays from three independent experiments. *Significant inhibition (p < 0.01) compared to the control.
The ability of the fraction to impair the adhesion of FITC-labeled P. gingivalis to a Matrigel® layer was examined. The fraction inhibited the adhesion of P. gingivalis to the basement membrane matrix model in a dose-dependent manner (Figure 3). The inhibition was significant at concentrations ≥250 μg/ml.
Figure 3. Effect of the berry polyphenolic fraction on the adhesion of P. gingivalis to the Matrigel® basement membrane model. A relative value of 100% was assigned to the adherence in the absence of the berry polyphenolic fraction. Results are expressed as the means ± SD of triplicate assays from three independent experiments. *Significant inhibition (p < 0.01) compared to the control.
The effect of the berry polyphenolic fraction on type I collagen degradation by proteinases of P. gingivalis was evaluated. A significant dose-dependent inhibition occurred at concentrations ≥31.25 μg/ml, which decreased type I collagen degradation by 35.8% (Figure 4). We also tested the effect of the berry polyphenolic fraction on the activity of membrane-bound Arg- and Lys-gingipains of P. gingivalis. The fraction reduced in a dose-dependent manner the activity of Arg-gingipain (Figure 5A) and, to a lower degree, Lys-gingipain (Figure 5B). At the highest tested concentration (250 μg/ml), the fraction decreased the activity of Arg-gingipain by 84.2% and Lys-gingipain by 67.6%.
Figure 4. Effect of the berry polyphenolic fraction on the collagenase activity of P. gingivalis. Leupeptin was used as a control inhibitor. A relative value of 100% was assigned to the degradation of type I collagen in the absence of the berry polyphenolic fraction. Results are expressed as the means ± SD of triplicate assays from three independent experiments. *Significant inhibition (p < 0.01) compared to the control.
Figure 5. Effect of the berry polyphenolic fraction on the Arg-gingipain activity (A) and Lys-gingipain activity (B) of P. gingivalis. Tosyl-L-lysine chloromethyl ketone hydrochloride was used as a control inhibitor. A relative value of 100% was assigned to the activity in the absence of the berry polyphenolic fraction. Results are expressed as the means ± SD of triplicate assays from three independent experiments. *Significant inhibition (p < 0.01) compared to the control.
Given that the berry polyphenolic fraction was found to be effective in inhibiting bacterial proteolytic enzymes, we hypothesized that it may also reduce the activity of host-derived MMPs that contribute to destruction of the periodontal tissues. The catalytic activity of MMP-9 used as a model was found to be dose-dependently inhibited by the berry polyphenolic fraction (Figure 6). At concentrations of 62.5, 125, and 250 μg/ml, the fraction decreased MMP-9 activity by 59.4, 72.9, and 88.8%, respectively.
Figure 6. Effect of the berry polyphenolic fraction on MMP-9 activity. N-isobutyl-N-[4-methoxyphenylsulfonyl]glycyl hydroxamic acid was used as a control inhibitor. A relative value of 100% was assigned to the activity in the absence of the berry polyphenolic fraction. Results are expressed as the means ± SD of triplicate assays from three independent experiments. *Significant inhibition (p < 0.01) compared to the control.
In a previous study [22], we showed that at concentrations up to 500 μg/ml, the berry polyphenolic fraction did not significantly decrease the oral keratinocyte (B11 cell line) viability in a colorimetric MTT test. Treating the oral keratinocytes with P. gingivalis (MOI of 103) up-regulated ROS production by 2.3-fold (Figure 7). However, in the presence of the berry polyphenolic fraction (7.81–500 μg/ml), the P. gingivalis-mediated ROS production was significantly attenuated (Figure 7).
Figure 7. Effect of the berry polyphenolic fraction on P. gingivalis-induced ROS production by oral keratinocytes. The + symbol indicates the presence of P. gingivalis at MOI of 103. A relative value of 100% was assigned to ROS production in the absence of the berry polyphenolic fraction. φSignificant increase compared to the control (no P. gingivalis stimulation). Results are expressed as the means ± SD of triplicate assays from three independent experiments. φSignificant increase compared to the control (no P. gingivalis stimulation). *Significant inhibition (p < 0.01) compared to the control (no fraction + P. gingivalis stimulation).
Since P. gingivalis has been shown to induce breakdown of keratinocyte barrier integrity (23, 25, 26), we investigated whether the berry polyphenolic fraction protects oral keratinocytes from the deleterious effect. A treatment (24 h) of keratinocytes with P. gingivalis (MOI of 103) significantly reduced the TEER value by 89.25% (Figure 8). However, the berry polyphenolic fraction provided significant protection against the P. gingivalis-modulated reduction in TEER.
Figure 8. Effect of the berry polyphenolic fraction on P. gingivalis-mediated loss of oral keratinocyte barrier function. The + symbol indicates the presence of P. gingivalis at MOI of 103. A relative value of 100% was assigned to the TEER recorded in the absence of P. gingivalis and the fraction. Results are expressed as the means ± SD of triplicate assays from three independent experiments. φSignificant decrease (p < 0.001) compared to unstimulated control cells. *Significant increase (p < 0.01) compared to P. gingivalis-stimulated cells not treated with the fraction.
P. gingivalis produces a wide range of pathogenic determinants (adhesins, proteases, hemolysin, etc.) that contribute to periodontal tissue colonization and destruction as well as to immune defense evasion [6, 7]. Moreover, in infected periodontal pockets, P. gingivalis LPS up-regulates the expression of pro-inflammatory mediators and MMPs by host mucosal and immune cells thus promoting the continuous inflammation that modulates bone resorption [26]. Since P. gingivalis is a critical player in the pathogenesis of periodontitis, it represents a key bacterial target in view of effective preventive and therapeutic strategies directed against this disease.
Targeting virulence factors to disarm bacterial pathogens has been proposed as a promising anti-infective strategy [10, 11]. Such an approach offers advantages over traditional antibiotics by protecting the commensal microbiota from elimination and applying less selective pressure that leads to the development of resistance. In this respect, the aim of the present study was to investigate the ability of a berry fruit (cranberry, wild blueberry, strawberry) polyphenolic preparation, commercialized as Orophenol®, to attenuate the pathogenic properties of P. gingivalis. The fraction contains various classes of polyphenolic compounds, including phenolic acids, flavonols, flavan-3-ols, anthocyanins and procyanidins [22], which may act synergistically [27], likely bolstering the beneficial properties.
The berry polyphenolic fraction significantly reduced the growth P. gingivalis, although no complete inhibition was observed even at the highest tested concentration (2000 μg/ml). While the exact mechanism of antibacterial action of polyphenols is still unclear, evidence have been brought that most polyphenols interact with the bacterial surface, leading to disruption of membrane integrity and the release of essential intracellular constituents [28–30].
The capacity of P. gingivalis to lyse erythrocytes in infected and inflamed periodontal pockets allows the bacterium to acquire heme to survive and multiply in vivo [31]. Interestingly, the berry polyphenolic fraction completely inhibited the hemolytic activity of P. gingivalis and as such, may impede its ability to proliferate. Although the mechanism involved in the inhibition of the P. gingivalis hemolytic activity by the berry polyphenolic fraction has not been investigated, it may be related to structural changes in erythrocyte membranes caused by the polyphenols thus limiting the binding of the hemolysin, as previously reported in Staphylococcus aureus [32].
The adhesion of P. gingivalis to the oral mucosa by binding to extracellular matrix proteins is a prerequisite for the subsequent invasion of periodontal tissues [1, 7, 33]. We showed that the berry polyphenolic fraction decreases the adhesion of P. gingivalis to a basement membrane matrix model (Matrigel®), which may impair its ability to colonize subgingival areas. This anti-adherence property is in agreement with the results reported by Souissi et al. [22], who showed that the berry polyphenolic fraction inhibits the adherence of the cariogenic bacterium Streptococcus mutans to saliva-coated hydroxyapatite and nickel-chrome alloy.
Porphyromonas gingivalis proteinases may play central roles for allowing the bacterium to colonize gingival tissues, acquire essential nutrients, evade the host immune defenses, and destroy periodontal tissues [8, 9]. Given this, bioactive molecules with the capacity to inhibit P. gingivalis proteinases may represent promising anti-virulence agents. We investigated the ability of the berry polyphenolic fraction to reduce or inhibit the proteolytic activities of P. gingivalis. We showed that the fraction is effective in preventing the degradation of collagen by P. gingivalis. It also inhibited the membrane-bound Arg- and Lys-gingipains.
Given this anti-proteinase activity of the berry polyphenolic fraction and since MMPs are also actively involved in destruction of the connective tissue of the periodontium [34–36], we also assessed the effect of the fraction on MMP activity. Since MMP-9 activity increases in inflamed periodontitis sites and correlates with the severity of the disease [34–36], it was used as a model of MMP. We showed that the fraction significantly inhibits the catalytic activity of MMP-9.
During chronic periodontal inflammation, excessive ROS production by mucosal and immune cells may cause damages to DNA, proteins, and lipids, thus inducing cell death [37, 38]. We used an oral keratinocyte model to demonstrate that P. gingivalis induces the generation of intracellular ROS and that the berry polyphenolic fraction significantly inhibits ROS production by P. gingivalis-stimulated keratinocytes.
The oral epithelium is a physical barrier that protects the underlying gingival connective tissues against invasion by periodontal pathogens [39, 40]. Previous studies have shown that P. gingivalis can damage cell-to-cell junctions and consequently cause disruption of epithelial barrier function [25, 41]. This may contribute to the ability of periodontal pathogens to reach the connective tissues, enter the bloodstream, and induce several systemic disorders, including atherosclerosis, Alzheimer's disease, and rheumatoid arthritis [4, 5, 42]. We showed that the berry polyphenolic fraction can preserve the integrity and function of the oral keratinocyte barrier by limiting the decrease in TEER induced by a P. gingivalis challenge. Part of the protective effect is likely dependent on the ability of the fraction to inhibit the gingipains of P. gingivalis, which have been found to cleave epithelial junction transmembrane proteins, including occludin, E-cadherin, and β1-integrin [43].
In conclusion, the berry polyphenolic fraction (Orophenol®) investigated in the present study attenuated several pathogenic properties of P. gingivalis. Polyphenols in this wild blueberry, cranberry, and strawberry fraction may thus be promising bioactive molecules, which likely act in synergy, to prevent and/or treat periodontal disease. Future studies aimed at investigating the benefits yielded by using oral hygiene products or slow releasing devices (inserted in diseased periodontal pockets) containing the fraction need to be undertaken.
The original contributions presented in the study are included in the article/supplementary material, further inquiries can be directed to the corresponding author/s.
Ethical review and approval was not required for this study in accordance with the local legislation and institutional requirements.
DG: conceptualization, formal analysis, funding acquisition, project administration, supervision, and writing. ABL and KV: investigation and methodology. All authors contributed to the article and approved the submitted version.
This study was financially supported by the Laboratoire de Contrôle Microbiologique of Université Laval.
The authors declare that the research was conducted in the absence of any commercial or financial relationships that could be construed as a potential conflict of interest.
All claims expressed in this article are solely those of the authors and do not necessarily represent those of their affiliated organizations, or those of the publisher, the editors and the reviewers. Any product that may be evaluated in this article, or claim that may be made by its manufacturer, is not guaranteed or endorsed by the publisher.
We are grateful to S. Groeger and J. Meyle (Justus-Liebig-University Giessen, Germany) for kindly providing the B11 keratinocyte cell line.
1. How KY, Song KP, Chan KG. Porphyromonas gingivalis: an overview of periodontopathogenic pathogen below the gum line. Front Microbiol. (2016) 7:53. doi: 10.3389/fmicb.2016.00053
2. Hajishengallis G, Lamont RJ. Breaking bad: manipulation of the host response by Porphyromonas gingivalis. Eur J Immunol. (2014) 44:328–38. doi: 10.1002/eji.201344202
3. Ximenez-Fyvie LA, Haffajee AD, Socransky SS. Microbial composition of supra- and subgingival plaque in subjects with adult periodontitis. J Clin Periodontol. (2000) 27:722–32. doi: 10.1034/j.1600-051x.2000.027010722.x
4. Mei F, Xie M, Huang X, Long Y, Lu X, Wang X, et al. Porphyromonas gingivalis and its systemic impact: current status. Pathogens. (2020) 9:944. doi: 10.3390/pathogens9110944
5. Mulhall H, Huck O, Amar S. Porphyromonas gingivalis, a long-range pathogen: systemic impact and therapeutic implications. Microorganisms. (2020) 8:869. doi: 10.3390/microorganisms8060869
6. Lunar Silva I, Cascales E. Molecular strategies underlying Porphyromonas gingivalis virulence. J Mol Biol. (2021) 433:166836. doi: 10.1016/j.jmb.2021.166836
7. Dahlen G, Basic A, Bylund J. Importance of virulence factors for the persistence of oral bacteria in the inflamed gingival crevice and in the pathogenesis of periodontal disease. J Clin Med. (2019) 8:1339. doi: 10.3390/jcm8091339
8. Fitzpatrick RE, Wijeyewickrema LC, Pike RN. The gingipains: scissors and glue of the periodontal pathogen, Porphyromonas gingivalis. Future Microbiol. (2009) 4:471–87. doi: 10.2217/fmb.09.18
9. Grenier D, La VD. Proteases of Porphyromonas gingivalis as virulence factors in periodontal diseases and potential targets for plant-derived compounds: a review article. Curr Drug Targets. (2011) 12:322–31. doi: 10.2174/138945011794815310
10. Heras B, Scanlon MJ, Martin JL. Targeting virulence not viability in the search for future antibacterials. Br J Clin Pharmacol. (2014) 79:208–15. doi: 10.1111/bcp.12356
11. Fleitas Martinez O, Cardoso MH, Ribeiro SM, Franco OL. Recent advances in anti-virulence therapeutic strategies with a focus on dismantling bacterial membrane microdomains, toxin neutralization, quorum-sensing interference and biofilm formation. Front Cell Infect Microbiol. (2019) 9:74. doi: 10.3389/fcimb.2019.00074
12. Kwon T, Lamster IB, Levin L. Current concepts in the management of periodontitis. Int Dent J. (2021) 71:462–76. doi: 10.1111/idj.12630
13. Graziani F, Karapetsa D, Alonso B, Herrera D. Nonsurgical and surgical treatment of periodontitis: how many options for one disease? Periodontol 2000. (2017) 75:152–88. doi: 10.1111/prd.12201
14. Chinsembu KC. Plants and natural products used in the management of oral infections and improvement of oral health. Acta Trop. (2016) 154:6–18. doi: 10.1016/j.actatropica.2015.10.019
15. Bunte K, Hensel A, Beikler T. 2019. Polyphenols in the prevention and treatment of periodontal disease: a systematic review of in vivo, ex vivo and in vitro studies. Fitoterapia. (2019) 132:30–9. doi: 10.1016/j.fitote.2018.11.012
16. Feghali K, Feldman M, La VD, Santos J, Grenier D. Cranberry proanthocyanidins: natural weapons against periodontal diseases. J Agric Food Chem. (2012) 60:5728–35. doi: 10.1021/jf203304v
17. Fraga CG, Croft KD, Kennedy DO, Tomás-Barberán FA. The effects of polyphenols and other bioactives on human health. Food Funct. (2019) 10:514–28. doi: 10.1039/C8FO01997E
18. Chen Y, Cheng S, Dai J, Wang L, Xu Y, Peng X, et al. Molecular mechanisms and applications of tea polyphenols: a narrative review. J Food Biochem. (2021) 45:e13910. doi: 10.1111/jfbc.13910
19. USDA. USDA Database for the Flavonoid Content of Selected Foods (Release 3.0). Beltsville, MD: U.S. Department of Agriculture, Agricultural Research Service Press (2011).
20. Nawrot-Hadzik I, Matkowski A, Hadzik J, Dobrowolska-Czopor B, Olchowy C, Dominiak M, et al. Proanthocyanidins and flavan-3-ols in the prevention and treatment of periodontitis—antibacterial effects. Nutrients. (2021) 13:165. doi: 10.3390/nu13010165
21. Slobodnikova L, Fialova S, Rendekova K, Kovac J, Mucaji P. Antibiofilm activity of plant polyphenols. Molecules. (2016) 21:1717. doi: 10.3390/molecules21121717
22. Souissi M, Ben Lagha A, Chaieb K, Grenier D. Effect of a berry polyphenolic fraction on biofilm formation, adherence properties and gene expression of Streptococcus mutans and its biocompatibility with oral epithelial cells. Antibiotics. (2021) 10:46. doi: 10.3390/antibiotics10010046
23. Ben Lagha A, Grenier D. Black tea theaflavins attenuate Porphyromonas gingivalis virulence properties, modulate gingival keratinocyte tight junction integrity and exert anti-inflammatory activity. J Periodont Res. (2017) 52:458–70. doi: 10.1111/jre.12411
24. Gröeger S, Michel J, Meyle J. Establishment and characterization of immortalized human gingival keratinocyte cell lines. J Periodontal Res. (2008) 43:604–14. doi: 10.1111/j.1600-0765.2007.01019.x
25. Vaillancourt K, Ben Lagha A, Grenier D. 2022. A phenolic-rich extract of cocoa (Theobroma cacao L) beans impairs the pathogenic properties of Porphyromonas gingivalis and attenuates the activation of nuclear factor kappa B in a monocyte model. Front Oral Health. (2022) 3:867793. doi: 10.3389/froh.2022.867793
26. O'Brien NM, Veith PD, Dashper SG, Reynolds EC. Antigens of bacteria associated with periodontitis. Periodontol 2000. (2004) 35:101–34. doi: 10.1111/j.0906-6713.2004.003559.x
27. Mitra S, Tareq AM, Das R, Emran TB, Nainu F, Chakraborty AJ, et al. Polyphenols: a first evidence in the synergism and bioactivities. Food Rev Int. (2022) 2022:1–23. doi: 10.1080/87559129.2022.2026376
28. Bouarab-Chibane L, Degraeve P, Ferhout H, Bouajila J, Oulahal N. Plant antimicrobial polyphenols as potential natural food preservatives. J Sci Food Agric. (2019) 99:1457–74. doi: 10.1002/jsfa.9357
29. Efenberger-Szmechtyk M, Nowak A, Czyzowska A. Plant extracts rich in polyphenols: antibacterial agents and natural preservatives for meat and meat products. Crit Rev Food Sci Nutr. (2021) 61:149–78. doi: 10.1080/10408398.2020.1722060
30. Bouarab-Chibane L, Forquet V, Lantéri P, Clément Y, Léonard-Akkari L, Oulahal N, et al. Antibacterial properties of polyphenols: characterization and QSAR (qualitative structure-activity relationship) models. Front Microbiol. (2019) 10:829. doi: 10.3389/fmicb.2019.00829
31. Smalley JW, Olczak T. Heme acquisition mechanisms of Porphyromonas gingivalis—strategies used in a polymicrobial community in a heme-limited host environment. Mol Oral Microbiol. (2017) 32:1–23. doi: 10.1111/omi.12149
32. Olchowik-Grabarek E, Sekowski S, Bitiucki M, Dobrzynska I, Shlyonsky V, Ionov M, et al. Inhibition of interaction between Staphylococcus aureus α-hemolysin and erythrocytes membrane by hydrolysable tannins: structure-related activity study. Sci Rep. (2020) 10:11168. doi: 10.1038/s41598-020-68030-1
33. Lamont RJ, Jenkinson HF. Subgingival colonization by Porphyromonas gingivalis. Oral Microbiol Immunol. (2000) 15:341–9. doi: 10.1034/j.1399-302x.2000.150601.x
34. Checchi V, Maravic T, Bellini P, Generali L, Consolo U, Breschi L, et al. The role of matrix metalloproteinases in periodontal disease. Int J Environ Res Public Health. (2020) 17:4923. doi: 10.3390/ijerph17144923
35. Cavalla F, Hernandez-Rios P, Sorsa T, Biguetti C, Hernandez M. Matrix metalloproteinases as regulators of periodontal inflammation. Int J Mol Sci. (2017) 18:440. doi: 10.3390/ijms18020440
36. Kinney JS, Morelli T, Oh M, Braun TM, Ramseier CA, Sugai JV, et al. Crevicular fluid biomarkers and periodontal disease progression. J Clin Periodontol. (2014) 41:113–20. doi: 10.1111/jcpe.12194
37. Wang Y, Andrukhov O, Rausch-Fan X. Oxidative stress and antioxidant system in periodontitis. Front Physiol. (2017) 8:910. doi: 10.3389/fphys.2017.00910
38. Chapple IL, Matthews JB. The role of reactive oxygen and antioxidant species in periodontal tissue destruction. Periodontol 2000. (2007) 43:160–232. doi: 10.1111/j.1600-0757.2006.00178.x
39. Groeger SE, Meyle J. Epithelial barrier and oral bacterial infection. Periodontol 2000. (2015) 69:46–67. doi: 10.1111/prd.12094
40. Senel S. An overview of physical, microbiological and immune barriers of oral mucosa. Int J Mol Sci. (2021) 22:7821. doi: 10.3390/ijms22157821
41. Groeger S, Doman E, Chakraborty T, Meyle J. Effects of Porphyromonas gingivalis infection on human gingival epithelial barrier function in vitro. Eur J Oral Sci. (2010) 118:582–89. doi: 10.1111/j.1600-0722.2010.00782.x
42. Meurman JH, Bascones-Martinez A. Oral infections and systemic health–more than just links to cardiovascular diseases. Oral Health Prev Dent. (2021) 19:441–8. doi: 10.3290/j.ohpd.b1993965
Keywords: P. gingivalis, periodontal disease, gingipain, polyphenol, keratinocyte
Citation: Vaillancourt K, Ben Lagha A and Grenier D (2022) Effects of a Berry Polyphenolic Fraction on the Pathogenic Properties of Porphyromonas gingivalis. Front. Oral. Health 3:923663. doi: 10.3389/froh.2022.923663
Received: 19 April 2022; Accepted: 25 May 2022;
Published: 16 June 2022.
Edited by:
Fabian Cieplik, University Medical Center Regensburg, GermanyReviewed by:
Ruijie Huang, Sichuan University, ChinaCopyright © 2022 Vaillancourt, Ben Lagha and Grenier. This is an open-access article distributed under the terms of the Creative Commons Attribution License (CC BY). The use, distribution or reproduction in other forums is permitted, provided the original author(s) and the copyright owner(s) are credited and that the original publication in this journal is cited, in accordance with accepted academic practice. No use, distribution or reproduction is permitted which does not comply with these terms.
*Correspondence: Daniel Grenier, ZGFuaWVsLmdyZW5pZXJAZ3JlYi51bGF2YWwuY2E=
Disclaimer: All claims expressed in this article are solely those of the authors and do not necessarily represent those of their affiliated organizations, or those of the publisher, the editors and the reviewers. Any product that may be evaluated in this article or claim that may be made by its manufacturer is not guaranteed or endorsed by the publisher.
Research integrity at Frontiers
Learn more about the work of our research integrity team to safeguard the quality of each article we publish.