- 1Laboratory of Molecular Oral Physiopathology, Centre de Recherche des Cordeliers, INSERM, Université Paris Cité, Sorbonne Université, Paris, France
- 2AP-HP, Dental Medicine Department, Pitié-Salpétrière Hospital, GHN-Université Paris Cité, Paris, France
- 3Laboratoire de Géologie de Lyon, UM R5276, CNRS, Université Lyon 1, École Normale Supérieure de Lyon 46, Lyon, France
Fluoride (F) is added to many dental care products as well as in drinking water to prevent dental decay. However, recent data associating exposure to F with some developmental defects with consequences in many organs raise concerns about its daily use for dental care. This systematic review aimed to evaluate the contribution of dental care products with regard to overall F intake through drinking water and diet with measurements of F excretion in urine used as a suitable biomarker. According to the Preferred Reporting Items for Systematic Reviews and Meta-Analyses (PRISMA) guidelines using keywords related to chronic exposure to F in the human population with measurements of F levels in body fluids, 1,273 papers published between 1995 and 2021 were screened, and 28 papers were finally included for data extraction concerning daily F intake. The contribution of dental care products, essentially by toothbrushing with kinds of toothpaste containing F, was 38% in the mean regardless of the F concentrations in drinking water. There was no correlation between F intake through toothpaste and age, nor with F levels in water ranging from 0.3 to 1.5 mg/L. There was no correlation between F intake and urinary F excretion levels despite an increase in its content in urine within hours following exposure to dental care products (toothpastes, varnishes, or other dental care products). The consequences of exposure to F on health are discussed in the recent context of its suspected toxicity reported in the literature. The conclusions of the review aim to provide objective messages to patients and dental professionals worried about the use of F-containing materials or products to prevent initial caries or hypomineralized enamel lesions, especially for young children.
Introduction
Fluoride (F) is the lighter halogen element and is largely present in food and drinking water with levels depending on the geological environment of the area. It is also added to dental care products used for oral hygiene and dentistry to prevent dental decay. It is admitted that tooth brushing with fluoridated toothpaste is a fundamental cornerstone for the prevention of early childhood caries [1]. It protects against caries by generating fluoridated apatite more resistant to acids produced by oral bacteria, increasing the remineralization process, and inhibiting bacterial enolase activity [2, 3]. However, limits to the prescription of F have been repeatedly advised, mostly because of the narrow safety range for its use. According to the European Food and Safety Authority (EFSA), the recommended doses to prevent caries have been evaluated approximately 0.05–0.07 mg/kg/day, which is close to the amount that may cause enamel hypomineralization, called dental fluorosis (>0.1 mg/kg/day) [4].
The main sources of F intake are fluoridated drinking water, dietary F, infant formulas, and F-containing dental care products, especially toothpaste. Some foods and beverages contain high levels of F, such as tea [5]. The increased prevalence of dental fluorosis indicates that some young children are exposed to F from sources other than drinking water, essentially the F-containing toothpaste they may swallow. F can substitute hydroxyl of the hydroxyapatite containing matrices, to form fluorapatite, underlying its extracellular effects in enamel, dentin, and bone [6]. F tropism for apatite explains its expected reinforced effects on enamel as well as dental and bone fluorosis when absorbed in excess [7]. Besides biomineralized matrices, many experimental studies report F effects on cell differentiation, proliferation, and apoptosis that may explain its toxic effects on the development and the physiology of many other tissues and organs when ingested at high doses [8–11]. The severity of F effects is related to the dose and duration of exposure as well as to its combination with other environmental factors as suggested by experimental studies on rodents and zebrafish [7, 12, 13]. The severity of F effects also appears to be contingent on the genetic background in rodents and humans and renal function [8, 14–17]. Once absorbed, F travels throughout the body via the blood circulation before being filtered by the kidney and excreted in urine, which thus ensures the majority of F removal from the body. Approximately 60% of ingested F by healthy adults are excreted in the urine, but only 45% for children, with the rest re-circulating into the plasma or deposited into the bone [18]. As a consequence, plasma and urinary excretion reflect a physiologic homeostasis determined by previous F intake, rate of F uptake and removal from bone, and the efficiency with which the kidneys excrete F.
Dental fluorosis and other F side effects on health may occur due to F overload from a combination of various sources, such as drinking water, dental care products used for caries prevention, medication with fluoridated products, and anesthetics, each source being innocent alone but with an unclear dose-response relationship when combined [19]. Due to the general awareness of relations between human environment and health, many patients are currently questioning their physicians and dentists about the safety of the prescribed treatments. Dentists strongly advice to brush teeth at least two times a day with fluorinated toothpastes, preconize fluorinated varnishes to protect children's teeth from caries, and higher fluorinated gels for specific patients and use biomaterials, such as adhesives or ionomer cements, for conservative dentistry and orthodontic treatments that may contain F.
The aim of this study is to provide a qualitative and descriptive analysis of the numerical data to evaluate the contribution of dental care products in the total daily fluoride intake (TDFI) based on urine monitoring and regarding the literature from 1995 to 2021. In the light of these results, dentists will be able to qualify the place that F takes in prevention and treatment programs in the overall systemic exposure of patients.
Methods
This systematic review is conducted according to the Preferred Reporting Items for Systematic Reviews and Meta-Analyses (PRISMA) guidelines (Figure 1).
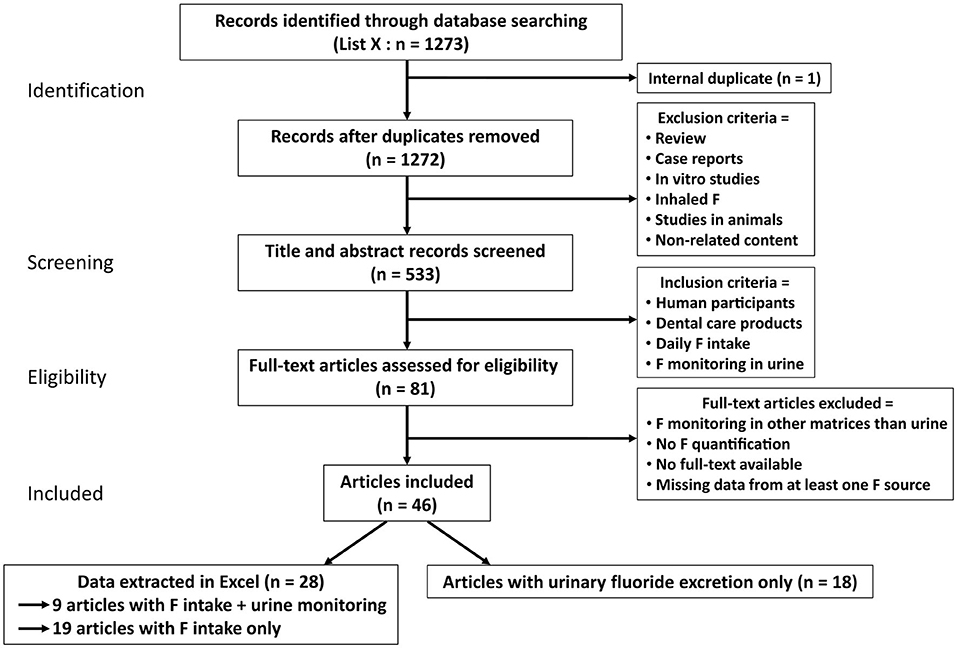
Figure 1. The Preferred Reporting Items for Systematic Reviews and Meta-Analyses (PRISMA) flowchart for the systematic review. From the 1,273 articles found in PubMed included in the search, 46 studies were included and 28 selected in this review for their analyses. Among the 28 articles, 19 only listed the estimated total daily fluoride intake (TDFI) (Table 1). The other nine articles had information regarding both the TDFI and the daily urinary fluoride excretion (DUFE) (Table 2).
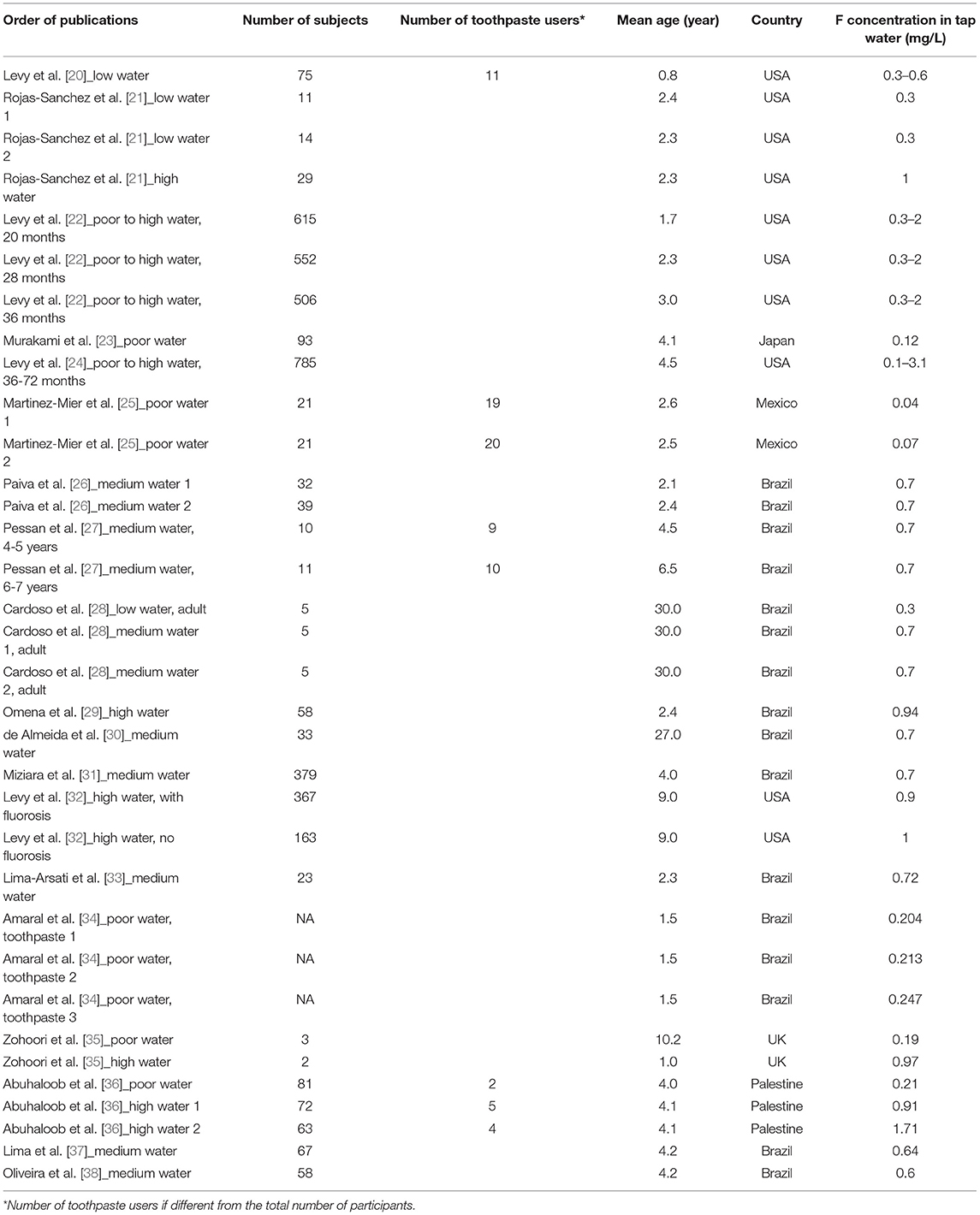
Table 1. Number of participants, their mean age and country of residence, with the associated F concentration in tap water (mg/L) in articles with only estimation of F intake in our Excel database.
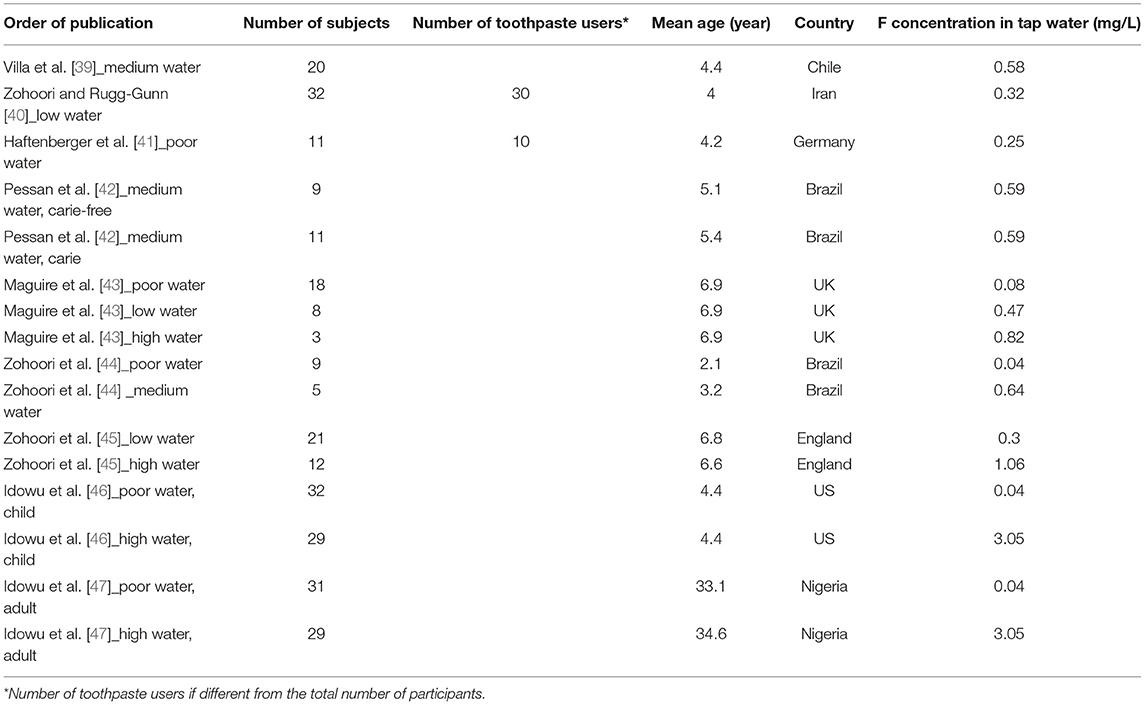
Table 2. Number of participants, their mean age and country of residence, with the associated F concentration in tap water (mg/L) in articles regarding estimated F intake and urine monitoring in our Excel database.
Search Strategy
The following search equation was entered in PubMed/Medline using the Booleans: (((dent*) OR (mouth*) OR (teeth) OR (tooth*) OR (enamel)) OR ((resin?) OR (“glass ionomer*”) OR (“bioactive glass*”) OR (composite?)) AND ((urin* fluori*) OR (plasma* fluori*) OR (“blood fluori*”) OR (“saliva fluori*”) OR (“bone fluori*”) OR (“hair fluori*”) OR (“nail fluori*”)) AND ((1995/1/1:2021/12/31[pdat]) AND (english[Filter] OR french[Filter]))) OR (((dent*) OR (mouth*) OR (teeth) OR (tooth*) OR (enamel)) OR ((resin?) OR (“glass ionomer*”) OR (“bioactive glass*”) OR (composite?)) AND ((“chronic fluoride”) OR (“chronic exposure to fluoride”) OR (“chronic fluoride exposure”) OR (“fluoride intake”) OR (“daily fluoride intake”) OR (“systemic fluoride”)) AND ((1995/1/1:2021/12/31[pdat]) AND (english[Filter] OR french [Filter]))).
Open access articles were retrieved and those with restricted access were retrieved through institutional access. Only two articles were excluded because the full-text was not accessible.
We checked that none of the included studies in this review were retracted due to error or fraud.
Eligibility Criteria
Inclusion Criteria
When establishing the search equation, language was limited to English and French, and articles were restricted from 01/01/1995 to 31/12/2021. The articles were selected taking into account the following inclusion criteria: (1) studies with human participants, (2) studies involving topical use of F-containing dental care products, (3) studies estimating the TDFI from water, beverages, such as juices, milk and infant formulas, meals, and dental care products which are mainly toothpastes in this review, and (4) studies monitoring F exposure through urine as a contemporary biomarker.
Exclusion Criteria
The exclusion process consisted of two steps. The first was applied before the inclusion of articles with the following criteria on title and abstract: (1) studies conducted on animals, (2) studies in vitro, (3) articles focusing on inhaled F, which may be found in some anesthesia, (4) articles with no related content to F exposure, and (5) reviews and case reports. The second step consisted on excluding those that had the following criteria: (1) F monitoring in other matrices than urine (plasma, saliva, nails, and hair), (2) articles with no F quantification or estimation, (3) articles that were not accessible, and (4) studies with missing data from at least one source of F either from water, beverages, solid food, or dental care product.
Process of Study Selection
First, all articles resulting of the search equation were entered in Zotero software. Elimination of duplicates was performed. Then the screening of title and abstract by two independent reviewers (HS et SH) was carried out according to the first step of the exclusion process. The same reviewers proceeded to select the articles by applying the inclusion criteria. The content of the abstract of each study was analyzed and the articles with relevant information regarding the subject of the current review were carefully chosen. Finally, the selected articles were evaluated through full-text analysis to determine which of them would be useful for the elaboration of the systematic review. This second step of the exclusion process was performed independently and in duplicate by each reviewer to compare the recorded information and correct the differences that were found during this step. In the case of disagreement between reviewers, a third reviewer (SB) was involved to resolve it.
Data Charting Process
Among the articles included, those relating the daily F intake of each source and monitoring the F in urine were selected. All data were entered in Excel software and was sorted to identify the authors, year of publication, title of article, country of study, number of participants, age and gender of participants, sources of exposure to fluorides, F concentration in tap water, and F monitoring in urine. Data extraction was performed by HS and RE.
Data Synthesis
Studies' characteristics are as follows:
• Year: from 1995 to 2021. The year 1995 was selected because it was the last recent date mentioned by Fejerskov et al. [48], which compiled all data from the previous years.
• Country: Supplementary Figure 1 represents the Mondial geographical repartition of the included articles in Excel. The percentage was calculated by counting the number of studies conducted in a country and dividing it by the total number of articles in Excel.
• Age: it was notified when it was presented (Tables 1, 2).
• Size of the cohort: the number of subjects was reported for each study when available. Moreover, the number of participants using dental care products was mentioned when it differed from the total number of participants (Tables 1, 2).
• Gender: proportion of men or women in participants was reported when data were available.
• All the articles selected in Excel are listed in Table 1 for those estimating F intake and in Table 2 for those estimating F intake and urine monitoring with the number of subjects, their mean age, countries of residence, and F concentration in tap water.
For outcome measures, we extracted information for the following parameters when they were available:
• The concentration of F in tap water in the area was entered in Excel. From our database, a range of F concentration was determined and quartiles were calculated. We obtained different categories of water depending on F content: poor (<0.3 mg/L), low (0.3–0.51 mg/L), medium (0.52–0.77 mg/L), and high (0.77–1.5 mg/L).
• Estimated intakes of F sources were water, beverages, diet, dental products, and supplements. Depending on the authors, the report of the sources may vary by combination of the listed sources.
• Method of assessment of daily dietary F intake (DDFI): diet diary during 2–3 days, duplicate plate method, diet history, and food frequency questionnaire (FFQ).
• Method of assessment of F intake from dental products: sample collection, toothpaste applied/expectorate collected, toothbrushing questionnaire, and toothpaste/toothbrush weighing before and after brushing.
• Contribution of dental care products to the TDFI: some values were easily found in articles and others needed to be calculated, when possible, by taking into account that not all participants had oral hygiene habits.
• Fluoride excretion: urine, or urine and feces (due to infant participants wearing diapers).
• Kinetic studies: those studies were based on timeline variations of F exposure depending on the dental care uses.
• Method of assessment of F in urine: urinary F concentration, urinary F excretion (by collecting 24-h urine, or spot urine, or time-controlled urine). Daily urinary fluoride excretion (DUFE) or F retention were reported when presented in the article.
• Analytical method: F-ion selective electrode, hexamethyl-di-siloxane diffusion, or not reported.
• Validity of data and methods: F intake, F excretion (urine collection), and F analytical method, or not reported.
• Reporting of the investigation of any relationship between F-containing dental products and F excretion.
Data Reporting
All data were reported and homogenized for intercomparisons in μg/day or μg/kg bw/day for F intake from diet and toothpaste, TDFI and DUFE, and in mg/L for F concentration in tap water.
In some publications, the contribution of diet and toothpaste was not reported. To be able to compare all the selected published data, we calculated the percentage of contribution of diet and toothpaste to the mean TDFI. This was based on the mean F intake extracted from the included articles regardless of the availability of percentage data. Despite not being optimal, this allowed to keep the maximum number of articles (Figure 2).
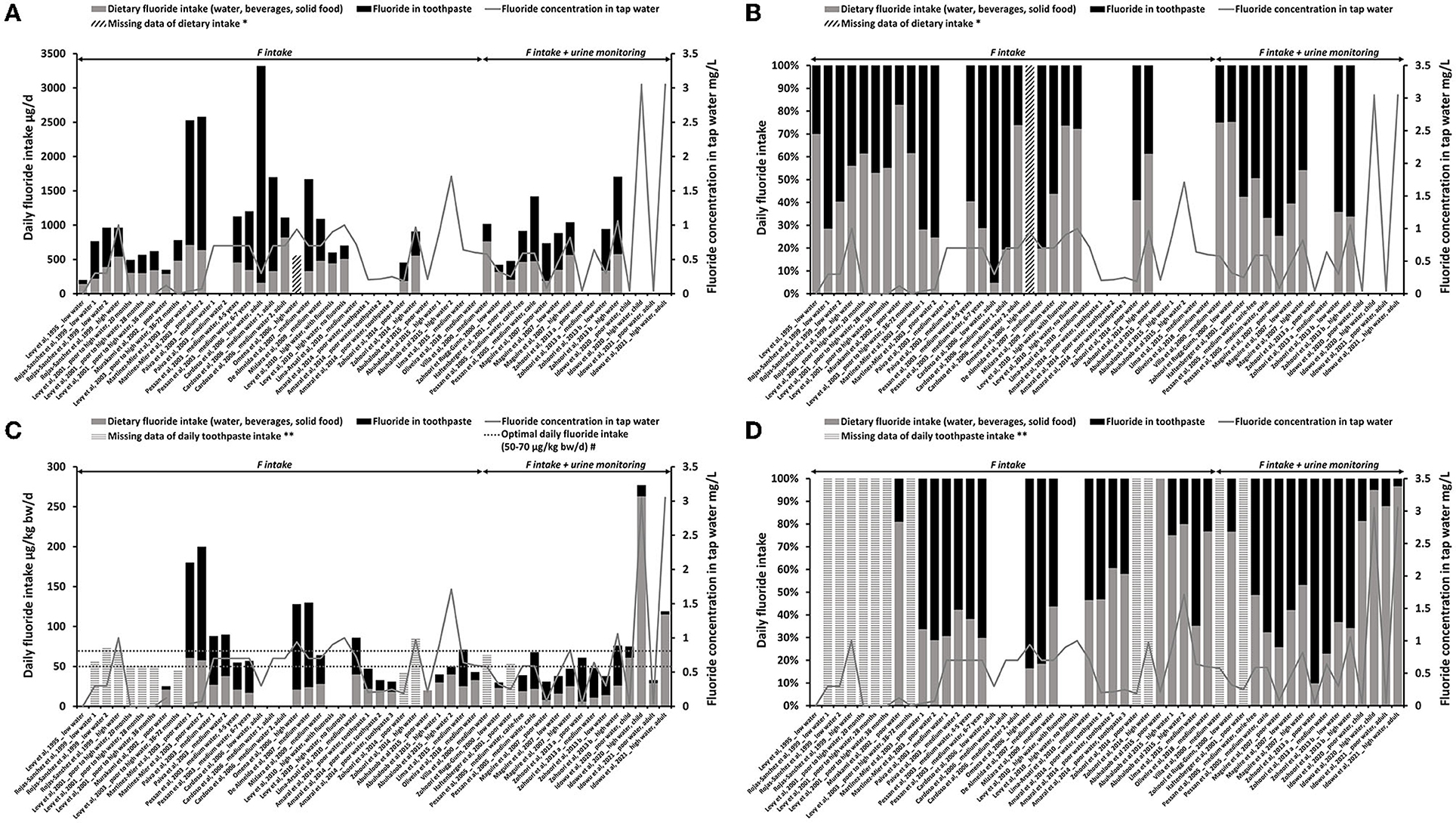
Figure 2. Estimated F intake from diet (water, beverages, and solid foods) (gray bars) and from toothpaste (black bars). F concentration in tap water is represented by the gray line (mg/L). (A) Total daily fluoride intake with dietary and toothpaste inputs (μg/day). (B) The contribution of daily diet (water, beverages, and solid foods) and toothpaste (%) to the estimated F intake in μg/day [based on (A)]. (C) TDFI with dietary and toothpaste inputs (μg/kg bw/day) with reference values of optimal daily F intake [50–70 μg/kg bw/day, the European Food and Safety Authority (EFSA)]. (D) The contribution of daily diet (water, beverages, and solid foods) and toothpaste (%) to the estimated F intake in μg/kg bw/day [based on (C)]. *Diagonal hatched bars represent missing data of daily dietary F intake (DDFI). **Horizontal hatched bars represent missing data of daily F intake from toothpaste. #Optimal range of daily F intake reported in the literature.
Results
From our initial selection of 1,273 articles, 46 met the inclusion criteria and only 28 were included in our systematic review as digital data concerning the estimation of daily F intake was reported by authors (Figure 1). Among these 46 articles, 18 were used only for the discussion and were not included into our database since they reported urine F excretion without any estimation of the TDFI [49–66]. Concerning the 28 selected studies, they were carried out in countries all over the world with almost half of the studies (43%) carried out in Brazil (Supplementary Figure 1) [20–47]. In our database, we reported the estimated F intake and urinary F monitoring. However, 19 publications only reported the estimated TDFI (Table 1) and the other nine reported both (Table 2). The 28 selected articles can be mentioned more than once depending on their categories of fluoridated tap water (explained in methods). Only two studies were performed with adults whose ages ranged from 20 to 35 years [28, 47]. Some studies present a high number of participants, however, in some of these publications, the number of children using dental care products can narrow down to 5% of the initial cohort [36]. In other publications, especially for urinary measures, the number of subjects is about 18 children. To simplify the figures, we compared the contribution of toothpaste only with dietary intake (such as water, beverages, and food sources) without taking into account supplements as only three studies mentioned them [20, 22, 41]. In most studies, the dental care product was toothpaste. Only one study had varnish in association with toothpaste [42]. F intake from the diet varied depending on the F concentration in tap water, meals, and beverages. It should be mentioned that local public water also affects the F content in meals (thus in diet) during the cooking. This additional F input was included into the diet.
In Figure 2, the TDFI (reported in μg/day in Figure 2A and μg/kg bw/day in Figure 2C) and the fraction of diet or toothpaste exposures were represented. Figures 2B,D showed the percentage of toothpaste in the total exposure.
Without acknowledging age or F concentration in water, total F intake was between 340 and 3,320 μg/day. Based on those concentrations, toothpaste F represented 15–95% of the TDFI reported in μg/day (Figures 2A,B); in the published percentages, its variation ranged from 19 to 84% (19 publications). This discrepancy is caused by the fact that the authors did not calculate all the toothpaste contribution percentages.
In Figure 2A, we can notice that the high F concentration in tap water was not associated with a higher input of the diet in the TDFI [21, 25, 27, 30, 42, 43, 45]. In Figures 2C,D, the age of the children was taken into consideration by dividing the body weight of the subjects. The TDFI was driven to the optimal range (50–70 μg/kg bw/day) or above due to the F intake from toothpaste (Figure 2C). The variations of F intake from diet seemed to be less sensitive to the F concentrations in water than the variations due to the concentrations and good practice of the use of dental care products in the area. The F intake from toothpaste represented 3–90% of the TDFI when reported to the body weight (Figure 2D), corresponding to 1–84% in published data, which discrepancy was due to the same reasons as those cited above.
Extremely high F concentrations in the water (>1.5 mg/L) were associated with a lower contribution of toothpaste, <20% of the total F intake. Among the three measurements included in our database, one was measured on an adult population supposed to have a better use of toothpaste (no swallowing) [47]. Therefore, between the two studies carried out in extremely high-fluoridated areas in children (>1.5 mg/L), only one reported an extremely high daily dietary input (Figure 2C) [36, 46].
When all the data were taken into consideration, the mean contribution of dental care products to the total exposure was 38 ± 27%. The F exposure through toothbrushing was thus significant when put into perspective with the TDFI for children: 39–51%, regardless of the F concentration in water (0.3–1.5 mg/L) [Table 3, the values reported by [20, 22, 24] were excluded]. However, in the case of extremely-fluoridated water (>1.5 mg/L), the dental care products contribution was estimated approximately 3% in the two studies carried out in children [36, 46].
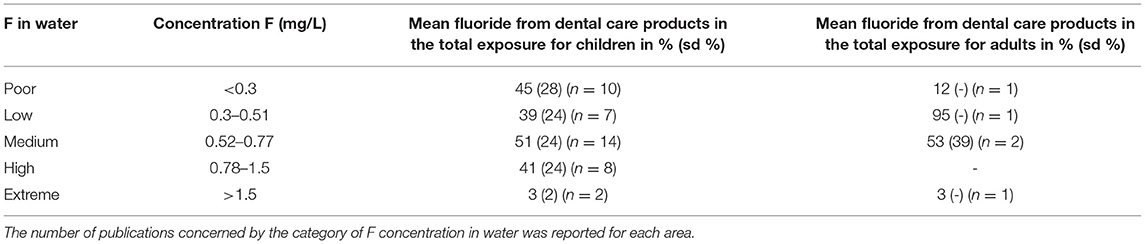
Table 3. Contribution of dental care products in F exposure depending on the F concentration in tap water for children and adults.
In adults, the mean contribution of dental care products to the total exposure was 12% in poor, 95% in low, 53% in medium, and 3% in extremely-fluoridated water (Table 3) [28, 47].
The contribution of toothpaste in different fluoridated areas according to the mean age of participants was displayed in Figure 3. As most of the articles were kinetic studies, only values at peak-level were considered to evaluate the maximum effect of dental care products on the daily intake. Only data recorded 24 h after exposure have been reported for the kinetic studies. Data reported by Levy et al. [20, 22, 24] were not included because the different areas with different F concentrations could not be distinguished. Calculated correlations (R2) were all below 0.14 showing the absence of correlation between daily F intake from toothpaste and the age of children regardless of the tap water F concentrations (Figure 3).
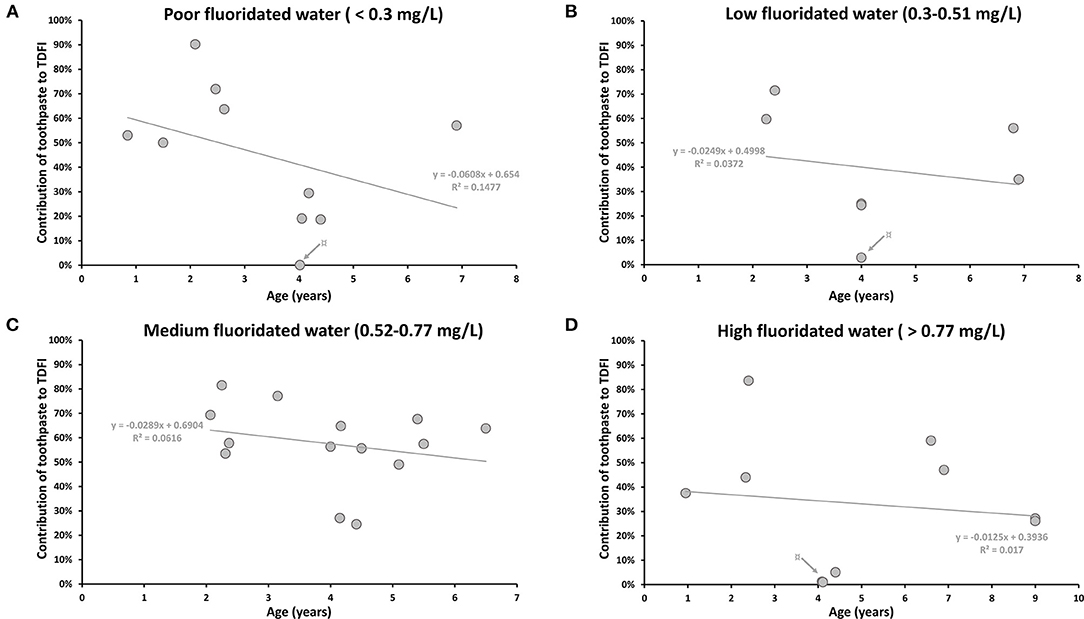
Figure 3. The contribution of daily toothpaste intake according to mean age (years) of participants in areas with different F concentration in drinking water (mg/L). (A) The contribution of toothpaste to the TDFI (%) in poor fluoridated water (<0.3 mg/L). ¤ Gray arrow indicates the lowest value from Abuhaloob et al. [36]: two toothpaste users among 81 participants. (B) The contribution of toothpaste to the TDFI (%) in low fluoridated water (0.3–0.51 mg/L). ¤ Gray arrow indicates the lowest value from Zohoori and Rugg-Gunn [40]: 3 toothpaste users among 28 in Darab (not the same region presented in Figure 2; Table 3). (C) The contribution of toothpaste to the TDFI (%) in medium fluoridated water (0.52–0.77 mg/L). (D) The contribution of toothpaste to the TDFI (%) in high fluoridated water (>0.77 mg/L). ¤ Gray arrow indicates the lowest values from Abuhaloob et al. [36]: nine toothpaste users among 135 participants.
Nevertheless, there was a tendency to present the highest estimation of daily F intake from toothpaste for the youngest children (younger than 4 years old) for all types of water (Figure 3). This observation was even more pronounced in poor-fluoridated areas, where the subjects under 4 years-old and older children were exposed to F from toothpaste between 50–90% and 0.04–57%, respectively (Figure 3A). The possible high contribution of toothpaste may be explained by the swallowing behavior for children under 4 years old [21, 25, 29, 30, 44].
Due to their better practice, adults should not be exposed to F through dental care products (Supplementary Figure 2) [28, 47]. However, Cardoso et al. (2006) reported high percentage values of toothpaste contribution that varied between 26 and 95% (Supplementary Figure 2) [28]. This high contribution for adult subjects was linked to their dental care practices. In this study, the authors actually reported that some subjects brushed their teeth three or four times a day. Another study also showed a non-negligible contribution of toothpaste to the TDFI, with an F ingestion from toothpaste of ~12 and 3% for 31 and 29 adults in poor and high-fluoridated areas, respectively [47].
To follow objectively the TDFI and to understand the capacity to eliminate absorbed F, we searched if there was a relation between the DUFE and the TDFI and the F intake from toothpaste (Figure 4A), as well as between the DUFE and the percentage of daily F intake from toothpaste (Figure 4B). For subjects living in high-fluoridated areas, data from Idowu et al. [46, 47] were removed.
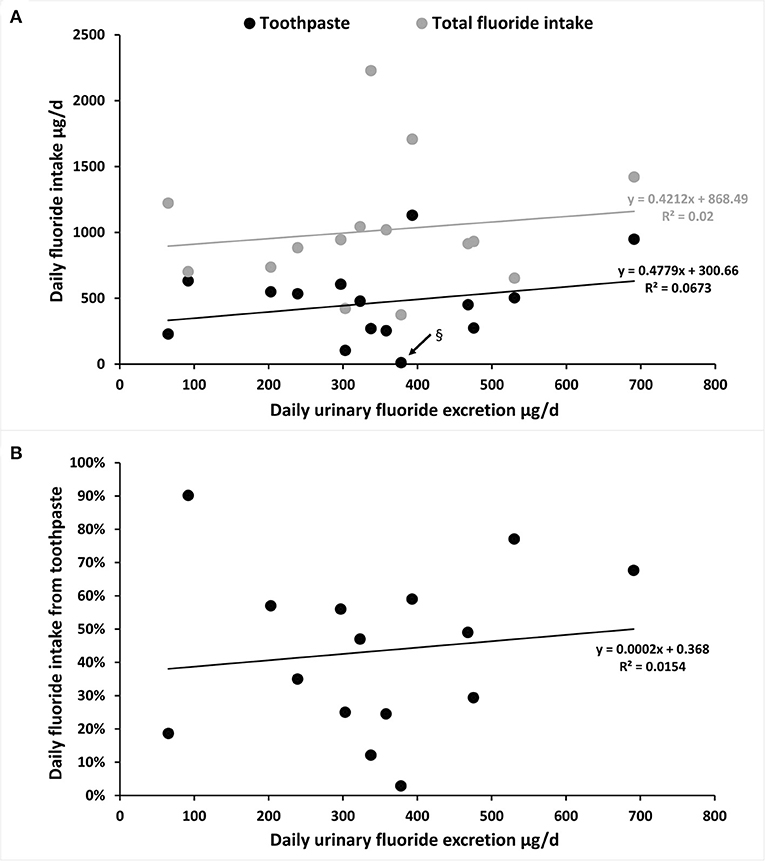
Figure 4. Estimation of the mean DUFE (μg/day) in relation with the mean TDFI (diet and toothpaste) or only daily F intake from toothpaste in participants aged 1–7 years old and 20–35 years old [the highest dot in (A)]. (A) The mean DUFE (μg/day) in relation with TDFI and daily F intake from toothpaste (μg/day). § Black arrow indicates the lowest value of F intake from toothpaste from Zohoori and Rugg-Gunn [40]: three toothpaste users among 28 in Darab (not the same region presented in Figure 2; Table 3). (B) The mean DUFE (μg/day) in relation with daily F intake from toothpaste (%).
There was a similar tendency to increase DUFE with increased TDFI and F intake through toothpaste (Figures 4A,B). However, with an R2 below 0.07, there was no correlation between DUFE and TDFI or between DUFE and daily F intake from toothpaste.
Discussion
The present systematic review showed that the mean contribution of F-containing dental care products, mainly toothpastes, is 38% regardless of the age of children or F concentration in drinking water. These data are slightly lower than those published earlier by Paiva et al. [26], reporting a 65% contribution. The difference may be either due to the method of collecting data or to evolution of the use of less fluoridated toothpastes.
The contribution of F intake was not correlated with the age of children. However, children under 4 years old presented a very high TDFI as well as some adults who did not respect the good practice of dental care uses. Those two cases highlight the importance of: (i) dental products on the exposome, (ii) the types of F, bio-assimilation, and concentrations in the dental care products, and (iii) the importance of dental care products adapted to age, but more importantly, the results show the importance of good dental care habits (no swallowing, rinsing with water after toothbrushing, exposure to F after meals, and use of appropriate amount of toothpaste). The variation of F intake from the diet seemed to be less sensitive to the water concentration than the variation due to the concentration and good use of dental care products in the different areas. Therefore, despite the fact that studies in this field are lacking especially for adults, impact of dental care products on TDFI for adults should not be negligible. This requests to be further investigated in the case of misconducted use as it can increase the risk of excessive F intake.
The increase in F intake, especially due to dental care products did not necessarily correlate with the amount of F excretion. This result can be explained by the fact that we were looking at the reported means, which smoothed the values. The lack of correlation between DUFE and TDFI can also be due to (i) poor estimation of F inputs (additional sources and under or overestimation), and/or (ii) bias of the methodological and/or analytical quantification of urinary excretion (data were reported in means for each publication, choice of collection, and measure of the F into the urine), and/or (iii) variability of excretion capacity for each organism. In addition, these data were based on nine publications which could be a limitation of the study.
Urine is the only biomarker capable of measuring F excretion. However, urine may not be the most pertinent biomarker for the estimation of TDFI especially in children due to F accumulation during bone growth and mineralization. Children can retain more F in their skeleton (~50%) than adults (approximately 36%), with inverse retention in bone with age of the children and with the excess of F excreted in urine [67]. The absence of correlation between DUFE and TDFI suggests that there is a variability but a non-negligible amount of F was not eliminated from the organism. Our data showed a variation of DUFE between 65.2 and 691 μg/day that may have informed on the F bioavailability, its residence time (clearance), and its interactions with different tissues. The majority of body F is bound in hard tissues, such as bones and teeth, and <1% can be found in soft tissues [17].
Further investigation combining measures of F in plasma and urine could be informative on the bioavailability of F and its interactions with different organs. Once absorbed, F reaches peak serum concentrations after 20–60 min, and then returns to the baseline after approximately 15 h suggesting that part of the oral F passes through systemic route [56, 57, 68, 69]. This is probably the reason why a relation has been reported between supplement use or the amount of toothpaste used for brushing and child's fluorosis scores [65]. Most pharmacokinetic analyses showed a transient increase in the urinary F excretion approximately 1–3 h after topical application of fluoridated varnishes in adults and in children, after the use of a fluoridated mouthrinse solution, or after brushing with F-containing toothpastes [42, 56–58, 60, 64, 66]. A return to baseline is reported by all the studies 24–72 h after the end of the exposure, irrespective of the source. The minimal recommended period of urine collection is 24 h to obtain good estimations of the daily amount of F excretion. The DUFE is the variable generally recommended for the estimation of the daily F exposure. The amount of excreted F is obtained by multiplying the 24-h urinary volume by its F concentration [18].
As a consequence, we have proposed an experimental model of cumulative F exposure following the age of the individuals considering three different thresholds of 30, 300, and 1,300 μg F/day (Supplementary Figure 3) and a model of mixed exposures (1,300 μg F/day until 4 years, then 300 μg F/day until 8 years, and 30 μg F/day until 16 years). The thresholds have been defined based on the estimated F retention. Those values were obtained by subtracting the DUFE from the TDFI and were estimated between a few μg and 1,890 μg/day. Thus, this model is a cumulative representation, which includes daily F bone retention to estimate the trapped F into the body over a span of several years. According to this model, early age exposure could drastically affect the total F retention into the organism. Even though the residence time (i.e., half-life) of F into the different organs remains not well known, the exposure to a high absorption of dental care products may print a high F content over the years. In addition, bad habits of dental care products may impact F exposure for the adults. Therefore, these data showing a non-negligible contribution to daily F intake through toothbrushing using F-containing toothpastes may be discussed in the light of the literature as F was reported to pass through the blood-placental barrier and the blood-brain barrier thus subsequently cause learning problems [70]. In fact, most of studies on the safety of toothpastes and dental care products are short-term pharmacokinetics studies that do not consider long-term effects, whereas F accumulated in bone may be released in specific situations associated to skeletal loss, such as lactation [71]. However, we found no study that has explored the contribution of dental care products to total F exposure in pregnant and lactating women nor studies taking into account the gender, especially in young children. This concern is even more important considering that recent studies reported a relation between prenatal F exposure and lower performance intelligence quotient (IQ) in boys, but not in girls [72]. An increase of 0.5 mg/L of F concentration in the water (approximately equal to the difference between fluoridated and non-fluoridated regions) was associated with a 7.9-point lower IQ score in formula-fed infants and 6.3-point lower IQ score in breastfed children in both boys and girls, suggesting that postnatal exposure to F may affect both sexes [73]. Sex-dependent susceptibility to F may be due to multiple biological and behavioral reasons, they have also been reported in several experimental studies in rodents, and more recently in zebrafish [12, 13].
In conclusion, our review highlights the major F contribution from dental care products regardless of the area or F concentration in drinking water. This additional source presents a large variability depending on the concentration, chemical forms, and amount of the dental product used. However, the good usage of these products also seems to be determinant for the contribution to TDFI. Therefore, the contribution of F intake through toothpaste can be easily controlled and adapted to the patient. Consequently, the future studies on F exposure and toxicity need to take into consideration exposure to F-containing dental care products, habits of use, and individual features (gender, age, diet, caries, etc.). Furthermore, considering the contribution of dental care products to the TDFI, the “optimal daily F intake” estimated approximately 50–70 μg/kg bw/day by EFSA could be reevaluated to determinate the optimal DDFI depending on each individual. The contribution of ~39–51% due to dental care products suggests that the optimal daily dietary F may be half of the EFSA values.
Data Availability Statement
The original contributions presented in the study are included in the article/Supplementary Material, further inquiries can be directed to the corresponding author.
Author Contributions
HS and SH selected the papers and reviewed them independently. SB was the third reviewer. HS and RE organized the data. HS, SH, and SB analyzed the data and the discussion. SH and SB drafted the manuscript. All authors contributed to the writing of the article and approved the final version.
Funding
The study was funded by the French National Institute of Health and Medical Research (INSERM), the Université Paris Cité (Idex Project FLUOREMAIL), and the National Agency for Safety of Food and Environment (ANSES) (Grant 2019/1/230).
Conflict of Interest
The authors declare that the research was conducted in the absence of any commercial or financial relationships that could be construed as a potential conflict of interest.
Publisher's Note
All claims expressed in this article are solely those of the authors and do not necessarily represent those of their affiliated organizations, or those of the publisher, the editors and the reviewers. Any product that may be evaluated in this article, or claim that may be made by its manufacturer, is not guaranteed or endorsed by the publisher.
Supplementary Material
The Supplementary Material for this article can be found online at: https://www.frontiersin.org/articles/10.3389/froh.2022.916372/full#supplementary-material
References
1. Kumar S, Tadakamadla J, Johnson NW. Effect of Toothbrushing Frequency on incidence and increment of dental caries: a systematic review and meta-analysis. J Dent Res. (2016) 95:1230–6. doi: 10.1177/0022034516655315
2. Moreno EC, Kresak M, Zahradnik RT. Fluoridated hydroxyapatite solubility and caries formation. Nature. (1974) 247:64–5. doi: 10.1038/247064a0
3. Hamilton IR. Biochemical effects of fluoride on oral bacteria. J Dent Res. (1990) 69:682–3. doi: 10.1177/00220345900690S128
4. Fejerskov O, Larsen MJ, Richards A, Baelum V. Dental tissue effects of fluoride. Adv Dent Res. (1994) 8:15–31. doi: 10.1177/08959374940080010601
5. Satou R, Oka S, Sugihara N. Risk assessment of fluoride daily intake from preference beverage. J Dent Sci. (2021) 16:220–8. doi: 10.1016/j.jds.2020.05.023
6. Aoba T. The effect of fluoride on apatite structure and growth. Crit Rev Oral Biol Med Off Publ Am Assoc Oral Biol. (1997) 8:136–53. doi: 10.1177/10454411970080020301
7. Aoba T, Fejerskov O. Dental fluorosis: chemistry and biology. Crit Rev Oral Biol Med Off Publ Am Assoc Oral Biol. (2002) 13:155–70. doi: 10.1177/154411130201300206
8. Johnston NR, Strobel SA. Principles of fluoride toxicity and the cellular response: a review. Arch Toxicol. (2020) 94:1051–69. doi: 10.1007/s00204-020-02687-5
9. Chachra D, Vieira APGF, Grynpas MD. Fluoride and mineralized tissues. Crit Rev Biomed Eng. (2008) 36:183–223. doi: 10.1615/CritRevBiomedEng.v36.i2-3.40
10. Barbier O, Arreola-Mendoza L, Del Razo LM. Molecular mechanisms of fluoride toxicity. Chem Biol Interact. (2010) 188:319–33. doi: 10.1016/j.cbi.2010.07.011
11. Wei Q, Deng H, Cui H, Fang J, Zuo Z, Deng J, et al. A mini review of fluoride-induced apoptotic pathways. Environ Sci Pollut Res Int. (2018) 25:33926–35. doi: 10.1007/s11356-018-3406-z
12. Mullenix PJ, Denbesten PK, Schunior A, Kernan WJ. Neurotoxicity of sodium fluoride in rats. Neurotoxicol Teratol. (1995) 17:169–77. doi: 10.1016/0892-0362(94)00070-T
13. Wang G, Wang T, Zhang X, Chen J, Feng C, Yun S, et al. Sex-specific effects of fluoride and lead exposures on histology, antioxidant physiology, and immune system in the liver of zebrafish (Danio rerio). Ecotoxicol Lond Engl. (2022) 31:396–414. doi: 10.1007/s10646-022-02519-5
14. Everett ET, McHenry M a. K, Reynolds N, Eggertsson H, Sullivan J, Kantmann C, et al. Dental fluorosis: variability among different inbred mouse strains. J Dent Res. (2002) 81:794–8. doi: 10.1177/0810794
15. Huang H, Ba Y, Cui L, Cheng X, Zhu J, Zhang Y, et al. COL1A2 gene polymorphisms (Pvu II and Rsa I), serum calciotropic hormone levels, and dental fluorosis. Community Dent Oral Epidemiol. (2008) 36:517–22. doi: 10.1111/j.1600-0528.2007.00424.x
16. Zhou GY, Ren LJ, Hou JX, Cui LX, Ding Z, Cheng XM, et al. Endemic fluorosis in Henan province, China: ERα gene polymorphisms and reproductive hormones among women. Asia Pac J Clin Nutr. (2016) 25(4):911–9. doi: 10.6133/apjcn.062016.01
17. Buzalaf MAR, Whitford GM. Fluoride metabolism. Monogr Oral Sci. (2011) 22:20–36. doi: 10.1159/000325107
18. Villa A, Anabalon M, Zohouri V, Maguire A, Franco AM, Rugg-Gunn A. Relationships between fluoride intake, urinary fluoride excretion and fluoride retention in children and adults: an analysis of available data. Caries Res. (2010) 44:60–8. doi: 10.1159/000279325
19. Martins CC, Paiva SM, Lima-Arsati YB, Ramos-Jorge ML, Cury JA. Prospective study of the association between fluoride intake and dental fluorosis in permanent teeth. Caries Res. (2008) 42:125–33. doi: 10.1159/000119520
20. Levy SM, Kohout FJ, Kiritsy MC, Heilman JR, Wefel JS. Infants' fluoride ingestion from water, supplements and dentifrice. J Am Dent Assoc 1939. (1995) 126:1625–32. doi: 10.14219/jada.archive.1995.0110
21. Rojas-Sanchez F, Kelly SA, Drake KM, Eckert GJ, Stookey GK, Dunipace AJ. Fluoride intake from foods, beverages and dentifrice by young children in communities with negligibly and optimally fluoridated water: a pilot study. Community Dent Oral Epidemiol. (1999) 27:288–97. doi: 10.1111/j.1600-0528.1998.tb02023.x
22. Levy SM, Warren JJ, Davis CS, Kirchner HL, Kanellis MJ, Wefel JS. Patterns of fluoride intake from birth to 36 months. J Public Health Dent. (2001) 61:70–7. doi: 10.1111/j.1752-7325.2001.tb03369.x
23. Murakami T, Narita N, Nakagaki H, Shibata T, Robinson C. Fluoride intake in Japanese children aged 3-5 years by the duplicate-diet technique. Caries Res. (2002) 36:386–90. doi: 10.1159/000066537
24. Levy SM, Warren JJ, Broffitt B. Patterns of fluoride intake from 36 to 72 months of age. J Public Health Dent. (2003) 63:211–20. doi: 10.1111/j.1752-7325.2003.tb03502.x
25. Martínez-Mier EA, Soto-Rojas AE, Ureña-Cirett JL, Stookey GK, Dunipace AJ. Fluoride intake from foods, beverages and dentifrice by children in Mexico. Community Dent Oral Epidemiol. (2003) 31:221–30. doi: 10.1034/j.1600-0528.2003.00043.x
26. Paiva SM, Lima YBO, Cury JA. Fluoride intake by Brazilian children from two communities with fluoridated water. Community Dent Oral Epidemiol. (2003) 31:184–91. doi: 10.1034/j.1600-0528.2003.00035.x
27. Pessan JP, Silva SMB da, Buzalaf MAR. Evaluation of the total fluoride intake of 4-7-year-old children from diet and dentifrice. J Appl Oral Sci Rev FOB. (2003) 11:150–6. doi: 10.1590/S1678-77572003000200012
28. Cardoso VES, Whitford GM, Buzalaf MAR. Relationship between daily fluoride intake from diet and the use of dentifrice and human plasma fluoride concentrations. Arch Oral Biol. (2006) 51:552–7. doi: 10.1016/j.archoralbio.2005.12.003
29. Omena LMF, Silva MF de A, Pinheiro CC, Cavalcante JC, Sampaio FC. Fluoride intake from drinking water and dentifrice by children living in a tropical area of Brazil. J Appl Oral Sci Rev FOB. (2006) 14:382–7. doi: 10.1590/S1678-77572006000500015
30. de Almeida BS, da Silva Cardoso VE, Buzalaf MAR. Fluoride ingestion from toothpaste and diet in 1- to 3-year-old Brazilian children. Community Dent Oral Epidemiol. (2007) 35:53–63. doi: 10.1111/j.1600-0528.2007.00328.x
31. Miziara APB, Philippi ST, Levy FM, Buzalaf MAR. Fluoride ingestion from food items and dentifrice in 2-6-year-old Brazilian children living in a fluoridated area using a semiquantitative food frequency questionnaire. Community Dent Oral Epidemiol. (2009) 37:305–15. doi: 10.1111/j.1600-0528.2009.00477.x
32. Levy SM, Broffitt B, Marshall TA, Eichenberger-Gilmore JM, Warren JJ. Associations between fluorosis of permanent incisors and fluoride intake from infant formula, other dietary sources and dentifrice during early childhood. J Am Dent Assoc 1939. (2010) 141:1190–201. doi: 10.14219/jada.archive.2010.0046
33. Lima-Arsati YBO, Martins CC, Rocha LA, Cury JA. Fingernail may not be a reliable biomarker of fluoride body burden from dentifrice. Braz Dent J. (2010) 21:91–7. doi: 10.1590/S0103-64402010000200001
34. Amaral JG, Freire IR, Valle-Neto EFR, Cunha RF, Martinhon CCR, Delbem ACB. Longitudinal evaluation of fluoride levels in nails of 18-30-month-old children that were using toothpastes with 500 and 1100 μg F/g. Community Dent Oral Epidemiol. (2014) 42:412–9. doi: 10.1111/cdoe.12103
35. Zohoori FV, Whaley G, Moynihan PJ, Maguire A. Fluoride intake of infants living in non-fluoridated and fluoridated areas. Br Dent J. (2014) 216:E3. doi: 10.1038/sj.bdj.2014.35
36. Abuhaloob L, Maguire A, Moynihan P. Total daily fluoride intake and the relative contributions of foods, drinks and toothpaste by 3- to 4-year-old children in the Gaza strip - palestine. Int J Paediatr Dent. (2015) 25:127–35. doi: 10.1111/ipd.12108
37. Lima CV, Cury JA, Vale GC, Lima MDM, Moura L de FAD, Moura MS de. Total fluoride intake by children from a tropical Brazilian city. Caries Res. (2015) 49:640–6. doi: 10.1159/000442029
38. Oliveira PFT de, Cury JA, Lima CV, Vale GC, Lima M de DM de, Moura L de FA de D, et al. Is the fluoride intake by diet and toothpaste in children living in tropical semi-arid city safe? Braz Oral Res. (2018) 32:e26. doi: 10.1590/1807-3107bor-2018.vol32.0026
39. Villa A, Anabalón M, Cabezas L. The fractional urinary fluoride excretion in young children under stable fluoride intake conditions. Community Dent Oral Epidemiol. (2000) 28:344–55. doi: 10.1034/j.1600-0528.2000.028005344.x
40. Zohouri FV, Rugg-Gunn AJ. Total fluoride intake and urinary excretion in 4-year-old Iranian children residing in low-fluoride areas. Br J Nutr. (2000) 83:15–25. doi: 10.1017/S0007114500000040
41. Haftenberger M, Viergutz G, Neumeister V, Hetzer G. Total fluoride intake and urinary excretion in German children aged 3-6 years. Caries Res. (2001) 35:451–7. doi: 10.1159/000047489
42. Pessan JP, Pin MLG, Martinhon CCR, de Silva SMB, Granjeiro JM, Buzalaf M a. R. Analysis of fingernails and urine as biomarkers of fluoride exposure from dentifrice and varnish in 4- to 7-year-old children. Caries Res. (2005) 39:363–70. doi: 10.1159/000086842
43. Maguire A, Zohouri FV, Hindmarch PN, Hatts J, Moynihan PJ. Fluoride intake and urinary excretion in 6- to 7-year-old children living in optimally, sub-optimally and non-fluoridated areas. Community Dent Oral Epidemiol. (2007) 35:479–88. doi: 10.1111/j.1600-0528.2006.00366.x
44. Zohoori FV, Buzalaf MaR, Cardoso CaB, Olympio KPK, Levy FM, Grizzo LT, et al. Total fluoride intake and excretion in children up to 4 years of age living in fluoridated and non-fluoridated areas. Eur J Oral Sci. (2013) 121:457–64. doi: 10.1111/eos.12070
45. Zohoori FV, Walls R, Teasdale L, Landes D, Steen IN, Moynihan P, et al. Fractional urinary fluoride excretion of 6-7-year-old children attending schools in low-fluoride and naturally fluoridated areas in the UK. Br J Nutr. (2013) 109:1903–9. doi: 10.1017/S0007114512003583
46. Idowu OS, Duckworth RM, Valentine RA, Zohoori FV. Biomarkers for the assessment of fluoride exposure in children. Caries Res. (2020) 54:134–43. doi: 10.1159/000504166
47. Idowu OS, Duckworth RM, Valentine RA, Zohoori FV. Biomarkers for the assessment of exposure to fluoride in adults. Caries Res. (2021) 55:292–300. doi: 10.1159/000516091
48. Fejerskov Ole, Ekstrand Jan, Burt Brian A. Fluoride in Dentistry. 2nd edition. Copenhagen: Munksgaard (1996). p. 363.
49. Alaçam A, Ulusu T, Bodur H, Oztaş N, Oren MC. Salivary and urinary fluoride levels after 1-month use of fluoride-releasing removable appliances. Caries Res. (1996) 30:200–3. doi: 10.1159/000262160
50. Altinova YB, Alaçam A, Aydin A, Sanisoglu SY. Evaluation of a new intraoral controlled fluoride release device. Caries Res. (2005) 39:191–4. doi: 10.1159/000084797
51. Caldas da. Rocha DR, Ricomini Filho AP, Cury JA. Soluble fluoride in Na2FPO3/CaCO3-based toothpaste as an indicator of systemically bioavailable fluoride. Caries Res. (2021) 56:55–63. doi: 10.1159/000521068
52. Chung CK, Millett DT, Creanor SL, Gilmour WH, Foye RH. Fluoride release and cariostatic ability of a compomer and a resin-modified glass ionomer cement used for orthodontic bonding. J Dent. (1998) 26:533–8. doi: 10.1016/S0300-5712(98)00017-7
53. Cury JA, Del Fiol FS, Tenuta LMA, Rosalen PL. Low-fluoride dentifrice and gastrointestinal fluoride absorption after meals. J Dent Res. (2005) 84:1133–7. doi: 10.1177/154405910508401208
54. Falcão A, Tenuta LMA, Cury JA. Fluoride gastrointestinal absorption from Na2FPO3/CaCO3- and NaF/SiO2-based toothpastes. Caries Res. (2013) 47:226–33. doi: 10.1159/000346006
55. Forte FDS, Moimaz SAS, Sampaio FC. Urinary fluoride excretion in children exposed to fluoride toothpaste and to different water fluoride levels in a tropical area of Brazil. Braz Dent J. (2008) 19:214–8. doi: 10.1590/S0103-64402008000300007
56. García-Hoyos F, Barbería E, García-Camba P, Varela M. Renal fluoride excretion in children following topical application of fluoride varnish. Eur J Paediatr Dent. (2012) 13:280–4.
57. García-Hoyos F, Cardososilva C, Barbería E. Renal excretion of fluoride after fluoride mouth rinses in children. Eur J Paediatr Dent. (2014) 15:35–8.
58. Lin YS, Rothen ML, Milgrom P. Pharmacokinetics of iodine and fluoride following application of an anticaries varnish in adults. JDR Clin Transl Res. (2018) 3:238–45. doi: 10.1177/2380084418771930
59. Lin YS, Rothen ML, Milgrom P. Pharmacokinetics of 38% topical silver diamine fluoride in healthy adult volunteers. J Am Dent Assoc 1939. (2019) 150:186–92. doi: 10.1016/j.adaj.2018.10.018
60. Lockner F, Twetman S, Stecksén-Blicks C. Urinary fluoride excretion after application of fluoride varnish and use of fluoride toothpaste in young children. Int J Paediatr Dent. (2017) 27:463–8. doi: 10.1111/ipd.12284
61. Martins CC, Paiva SM, Cury JA. Effect of discontinuation of fluoride intake from water and toothpaste on urinary excretion in young children. Int J Environ Res Public Health. (2011) 8:2132–41. doi: 10.3390/ijerph8062132
62. Milgrom P, Taves DM, Kim AS, Watson GE, Horst JA. Pharmacokinetics of fluoride in toddlers after application of 5% sodium fluoride dental varnish. Pediatrics. (2014) 134:e870–874. doi: 10.1542/peds.2013-3501
63. Opydo-Szymaczek J, Ogińska M, Wyrwas B. Fluoride exposure and factors affecting dental caries in preschool children living in two areas with different natural levels of fluorides. J Trace Elem Med Biol Organ Soc Miner Trace Elem GMS. (2021) 65:126726. doi: 10.1016/j.jtemb.2021.126726
64. Vale G, Simões N, Santana G, Mota B, Moura M. Gastrointestinal absorption and renal excretion of fluoride after ingestion of a high-fluoride dentifrice. Biol Trace Elem Res. (2019) 190:24–9. doi: 10.1007/s12011-018-1511-y
65. Martinez-Mier EA, Soto-Rojas AE. Differences in exposure and biological markers of fluoride among white and African American children. J Public Health Dent. (2010) 70:234–40. doi: 10.1111/j.1752-7325.2010.00173.x
66. Olympio KPK, Cardoso VE da S, Bijella MFB, Pessan JP, Delbem ACB, Buzalaf MAR. Urinary fluoride output in children following the use of a dual-fluoride varnish formulation. J Appl Oral Sci Rev FOB. (2009) 17:179–83. doi: 10.1590/S1678-77572009000300009
67. Buzalaf M a. R, Rodrigues MHC, Pessan JP, Leite AL, Arana A, Villena RS, et al. Biomarkers of fluoride in children exposed to different sources of systemic fluoride. J Dent Res. (2011) 90:215–9. doi: 10.1177/0022034510385937
68. Marthaler TM, Steiner M, Menghini G, De Crousaz P. Urinary fluoride excretion in children with low fluoride intake or consuming fluoridated salt. Caries Res. (1995) 29:26–34. doi: 10.1159/000262036
69. Villa A, Anabalón M, Cabezas L, Rugg-Gunn A. Fractional urinary fluoride excretion of young female adults during the diurnal and nocturnal periods. Caries Res. (2008) 42:275–81. doi: 10.1159/000135673
70. Choi AL, Sun G, Zhang Y, Grandjean P. Developmental fluoride neurotoxicity: a systematic review and meta-analysis. Environ Health Perspect. (2012) 120:1362–8. doi: 10.1289/ehp.1104912
71. Kovacs CS. Maternal mineral and bone metabolism during pregnancy, lactation, and post-weaning recovery. Physiol Rev. (2016) 96:449–547. doi: 10.1152/physrev.00027.2015
72. Green R, Rubenstein J, Popoli R, Capulong R, Till C. Sex-specific neurotoxic effects of early-life exposure to fluoride: a review of the epidemiologic and animal literature. Curr Epidemiol Rep. (2020) 7:263–73. doi: 10.1007/s40471-020-00246-1
Keywords: fluoride, drinking water, diet, toothpaste, dental products, urine
Citation: Saad H, Escoube R, Babajko S and Houari S (2022) Fluoride Intake Through Dental Care Products: A Systematic Review. Front. Oral. Health 3:916372. doi: 10.3389/froh.2022.916372
Received: 09 April 2022; Accepted: 04 May 2022;
Published: 10 June 2022.
Edited by:
Rogelio González-González, Juárez University of the State of Durango, MexicoReviewed by:
Jesus Lavalle-Carrasco, Juárez University of the State of Durango, MexicoOmar Tremillo Maldonado, Juárez University of the State of Durango, Mexico
Copyright © 2022 Saad, Escoube, Babajko and Houari. This is an open-access article distributed under the terms of the Creative Commons Attribution License (CC BY). The use, distribution or reproduction in other forums is permitted, provided the original author(s) and the copyright owner(s) are credited and that the original publication in this journal is cited, in accordance with accepted academic practice. No use, distribution or reproduction is permitted which does not comply with these terms.
*Correspondence: Sylvie Babajko, c3lsdmllYmFiYWprb0BnbWFpbC5jb20=