- 1School of Biomedicine, University of Adelaide, Adelaide, SA, Australia
- 2UniSA Online, University of South Australia, Adelaide, SA, Australia
- 3Precision Medicine Theme (Cancer), South Australian Health and Medical Research Institute, Adelaide, SA, Australia
Oral mucositis (OM) is one of the most common and debilitating oral complications of cancer treatments including chemotherapy, radiotherapy, and hematopoietic stem cell transplantation. It is associated with severe pain and difficulties in chewing, swallowing, and speech. This leads to impairment of basic oral functions and could result in unplanned treatment interruption or modification. As such, OM negatively impacts both patients' quality of life as well as tumor prognostic outcomes. Understanding pathways underlying OM pathogenesis help identify new targets for intervention or prevention. The pathophysiology of OM has been widely studied over past decades with several pathways related to oxidative stress, inflammation, and molecular and cellular signaling being implicated. In this mini-review, we will discuss the emerging role of the oral-gut microbiome axis in the development of OM. Particularly, we will elaborate on how the alterations in the oral and gut microbiota as well as intestinal dysfunction caused by cancer treatments could contribute to the pathogenesis of OM. Further, we will briefly discuss the potential methods for targeting the oral-gut microbiome axis to improve OM outcomes.
Introduction
The oral/oropharyngeal mucosa is highly sensitive to cytotoxic anti-cancer agents causing profound inflammation and breakdown of the mucosal barrier [1]. The resulting ulcerative lesions, termed oral mucositis (OM), is one of the most frequent oral complications affecting 80–100% of patients with head and neck cancer (HNC) treated with radiotherapy [2, 3], up to 40% of patients receiving chemotherapy [4], and 70–87% of patients undergoing hematopoietic stem cell transplantation (HSCT) [5, 6]. OM has been identified as one of the most debilitating toxicities that significantly impact patients' quality of life due to its associated pain, difficulty chewing and swallowing, weight loss, and infection [2, 7–9]. In cases where these cannot be optimally managed, treatment is often withheld or the dose reduced, which therefore negatively impacts patient prognosis [10, 11]. In addition to clinical consequences, OM is also associated with a significant economic cost as patients often require intensive medical interventions for symptoms management [2, 7, 12].
OM pathophysiology is a complex multifactorial process involving direct and indirect injury pathways including DNA damage, oxidative stress, inflammatory responses, and bacterial translocation [13]. OM develops through five phases i.e., initiation, signal upregulation and amplification, ulceration, and healing [14–19]. Briefly, exposure to cytotoxic agents initiates epithelial cell death through direct DNA damage and the production of reactive oxygen species causing tissue damage and activating subsequent molecular pathways including nuclear factor kappa-B (NF-κB). This results in the production of pro-inflammatory mediators such as tumor necrosis factor-α, interleukin (IL)-1β, and IL-6 leading to the ulcerative phase in which painful deep ulcers are formed creating a thriving environment for bacterial colonization which in turn exacerbate inflammatory responses. During the healing phase, signals from the submucosa and extracellular matrix stimulate proliferation and differentiation of epithelial cells allowing the restoration of the normal tissue structure [14–19].
The significant pathological changes in the oral cavity have led to the assumption that alterations in the oral microbes following cancer treatments could contribute to OM development. As such, the role of oral microbiota in the pathogenesis of OM has been an area of interest for several decades, with changes in oral microbiota following radiation therapy documented as early as the 1980s [20]. The significant increase in bacterial load in the ulcerated epithelium, and the correlation between bacterial load and OM peak severity [19], has suggested a causal relationship between oral bacteria and OM [19, 21, 22]. Hence, multiple clinical trials have used antimicrobial agents targeting oral bacteria to reduce OM severity; however, these attempts have failed to achieve positive outcomes [23]. This might be due to non-selective targeting of the oral microbiota and a lack of understanding of which specific microbes are contributing to OM. The recent advances in culture-independent microbial detection technologies (e.g., 16S rRNA sequencing) have allowed for extensive characterization of oral microbiota and subsequent investigation of its association with OM [24].
In addition to oral pathology, cancer treatments are also associated with major pathological changes in the lower gastrointestinal tract including intestinal inflammation, and disruption of intestinal barrier integrity and functions [13, 25]. These are often accompanied by changes in the gut microbiota, which serve to exacerbate gastrointestinal dysfunction [26]. In addition to disrupting local gut homeostasis, these changes are thought to impact organ systems at distant sites and therefore have prompted speculation that disruption of intestinal homeostasis could also contribute to OM pathogenesis. This mini-review focuses on the role of oral-gut microbiome axis pathways including oral and gut microbiota dysbiosis, intestinal dysfunction, and gut microbiota oralization in OM pathogenesis and briefly discusses potential methods to target these pathways to prevent or reduce the severity of OM.
Oral-Gut Microbiome Axis in OM
Oral Microbiota Dysbiosis and OM
The oral microbiota, a collection of microorganisms residing in the oral cavity, is composed of more than 700 bacterial species representing the second-largest microbial community in the human body after the gut microbiota [27]. Different bacterial populations are found in different oral cavity sites with a distinctive microbial community found in saliva, oral mucosa, and dental plaque [28, 29]. Oral microbiota plays a key role in maintaining oral homeostasis and preventing the colonization of exogenous pathogenic microorganisms [28, 30]. However, disruption of the oral microbial ecosystem could contribute to local and systemic diseases, with a growing body of evidence implicating the oral microbiota with oral diseases (periodontitis, dental caries, and oral cancer) and systemic conditions (colorectal cancer, diabetes, Alzheimer's disease, and cardiovascular diseases) [31].
Exposure to cytotoxic cancer therapies is widely associated with changes in the oral microbiota, directly caused by bactericidal or bacteriostatic anti-cancer agents, and indirectly through the breakdown of the mucosal lining and alteration of immunological properties of the oral environment [32–35]. Similarly, changes in saliva production and composition, xerostomia, are also associated with microbial changes in the mouth [36, 37]. Alterations in the oral microbiota have been extensively studied using both culture-dependent and independent methods. While culture-based studies confirmed the alterations in oral microbiota following cancer treatments, they failed to demonstrate an association between the oral microbiota and OM severity as the analysis was limited to cultivated microorganisms [32]. The rapid advances in culture-independent molecular and next-generation sequencing techniques have allowed for more efficient detection of low abundance and non-cultivable taxa and helped overcome the detection limitations of culture-based methods [34, 38]. Hence, multiple studies have used these methods to characterize the oral microbiota in patients undergoing cancer treatments [39–41]. For instance, using 16S rRNA sequencing, Napeñas et al. reported a shift in the oral microbial community, which was dominated by Streptococcus mitis and Gemella haemolysans in patients with breast cancer treated with chemotherapy [39]. The same method was used by Hu et al. and demonstrated a temporal shift in the relative abundance of core oral microbiota throughout radiotherapy with a negative correlation between radiation doses and the oral microbial richness in patients with HNC undergoing radiotherapy [40]. Studies also attempted to identify a specific oral microbial signature associated with the risk or severity of OM (Table 1) [6, 33, 41–50]. Although no clear microbial signature was identified across these studies, one of the consistent observations is the enrichment of oral pathobiont Fusobacterium (F. nucleatum) in patients with severe OM [6, 41, 44–46, 48]. Interestingly, patients who experienced more severe OM had more profound changes in the oral microbiota while a more resilient oral microbiota, minimal alterations, and faster recovery of the microbial community were observed in those with less severe OM [33, 46, 49, 50]. Collectively, the current evidence suggests that oral microbiota alterations are associated with OM onset and severity; however, a clear microbial pattern is yet to be established. This might be due to the variation in study subjects, samples collection time, sampling sites and methodology, or OM scoring methods. Thus, there is a need for a standardized methodology for oral microbiota sampling and analysis to obtain more consistent results.
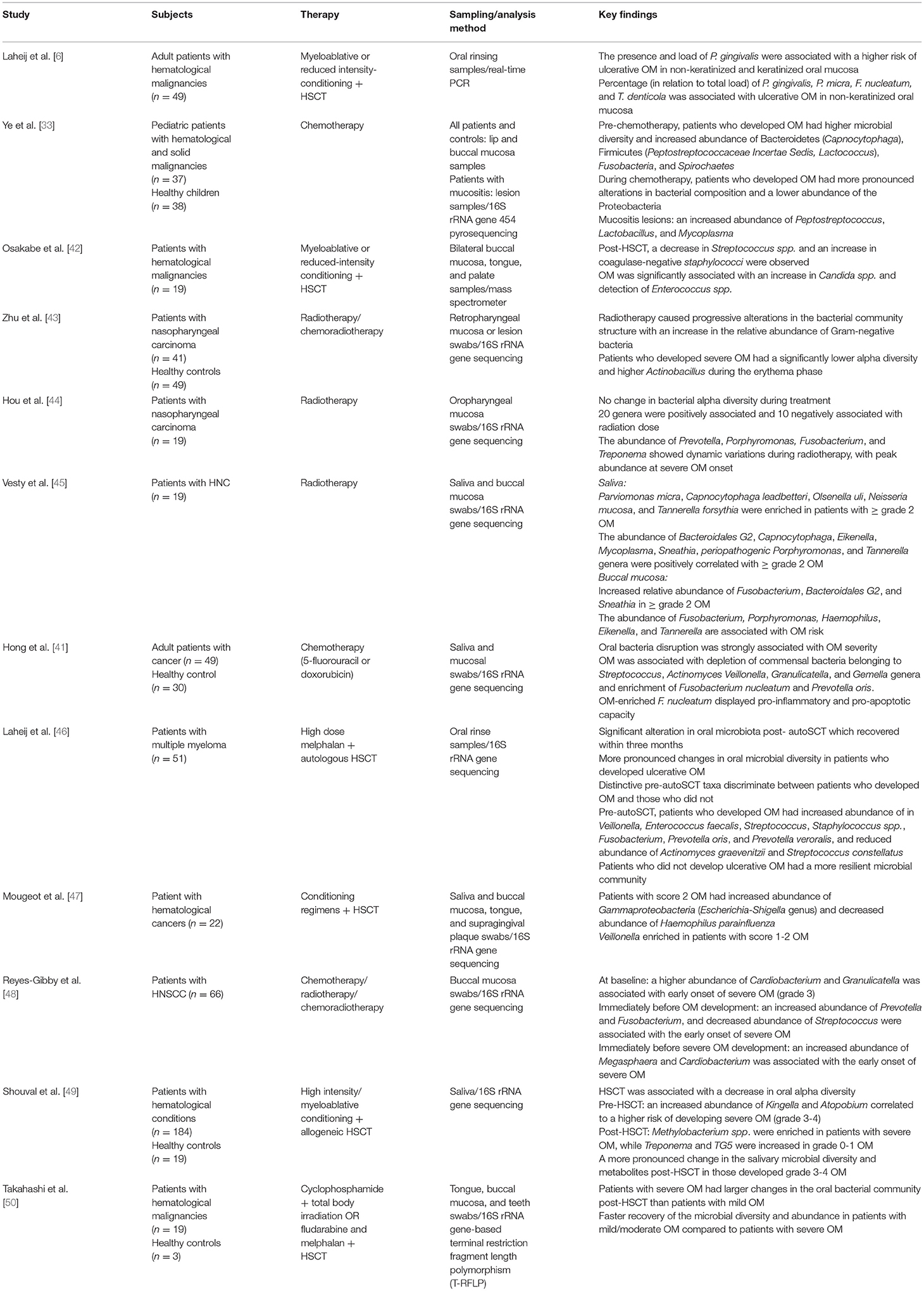
Table 1. Studies investigated the association between the oral microbiota and the development of OM (studies that used culture-independent methods only were included).
Most of the present research has focused on the association between oral microbiota and OM; however, the causal relationship remains poorly understood. Only one study has been conducted and demonstrated that germ-free mice treated with chemotherapy had less oral epithelial tissue injury and lower levels of pro-inflammatory cytokines and matrix metalloproteinases in the tongue tissues compared to specific pathogen-free mice [51]. Although the authors suggested that these outcomes are mediated by the oral microbiota, this does not exclude the impact of the gut microbiota as germ-free mice are completely free of all microbes. Overall, despite limited research, current evidence suggests that oral microbiota may contribute to OM through the regulation of oral innate immune pathways including NF-κB and toll-like receptors (TLRs) [22]. Microbiota-derived molecules like lipopolysaccharides can interact with TLRs in infiltrating immune cells leading to the further activation of NF-κB and, therefore, exacerbating inflammatory signals [21]. Further, the oral microbiota could influence OM healing phase by regulating the rate of mucosal recovery and restoration. It has been demonstrated that co-culturing the oral microbiota biofilms and epithelial cell layer alters its wound healing capacity [52]. Moreover, oral pathobiont associated with OM e.g., Porphyromonas gingivalis (P. gingivalis) has been found to inhibit cell migration in an in vitro assay of human buccal epithelial cells, suggesting the oral microbiota could contribute to the epithelial wound healing process [53, 54].
OM-Associated Intestinal Dysfunction
It is well-documented that systemic chemotherapy and HSCT myeloablative regimes cause significant gastrointestinal toxicities characterized by gastrointestinal mucositis, diarrhea, nausea, vomiting, and abdominal pain [55]. These toxicities are often associated with major gastrointestinal pathological changes including gut microbial dysbiosis, disruption of barrier functions, and intestinal inflammation [13, 25]. While these are expected consequences in patients receiving systemic therapies, local radiotherapy to the head and neck could also cause intestinal inflammation and disrupt intestinal barriers. For instance, Fernández-Gil et al. demonstrated that irradiation of the rat oral cavity was associated with intestinal damage, oxidative stress, and reduction in intestinal tight junction protein, Zonula occludens-1 [56]. Gastrointestinal toxicity characterized by disruption of intestinal barriers can lead to increased translocation of bacterial endotoxins into the circulation, activation of systemic inflammation, and eventually aggravating tissue injury in other parts of the body such as the brain [57, 58], liver [59], and heart [60]. Similarly, these pathological changes could enhance the severity of OM by enhancing systemic inflammatory responses; however, this is yet to be investigated. Nevertheless, reduced intestinal inflammation and increased expression of tight junction proteins were associated with lower severity of radiation-induced OM in a rat model suggesting that intestinal homeostasis is a potential target for alleviating OM [56]. Together, intestinal pathologies during cancer therapies may contribute to OM development and severity through activating systemic inflammation (Figure 1), and hence further research is warranted.
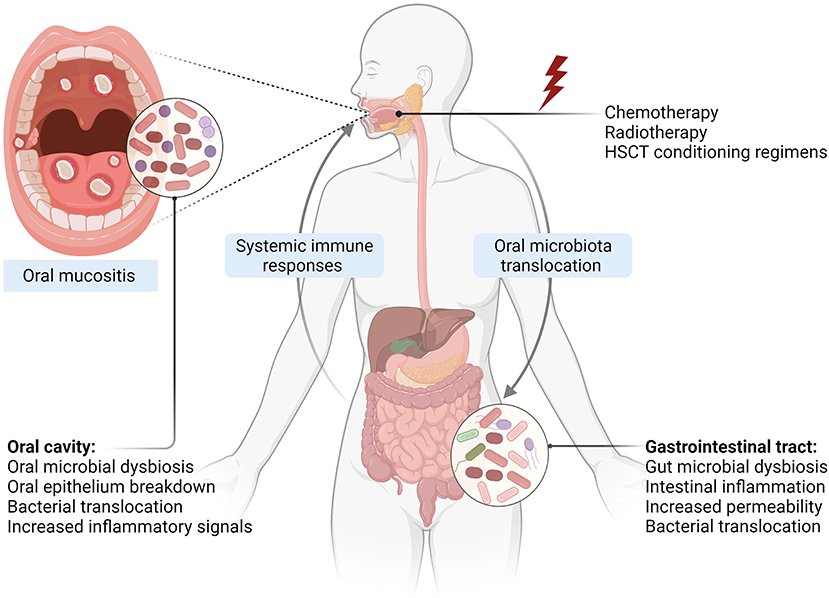
Figure 1. Oral-gut microbiome axis in the development of OM. Exposure to cytotoxic cancer treatments causes direct tissue injury and subsequent inflammatory responses leading to epithelial damage. Changes in the oral environment result in oral microbiota dysbiosis, which can cross through the damaged and ulcerated mucosa, interacting with immune cells and enhancing inflammatory responses. Intestinal pathological changes including gut microbiota dysbiosis, caused by anticancer agents and oral microbiota translocation into the gut, disrupt intestinal homeostasis and facilitate bacterial translocation into circulation and activation of systemic immune responses, which in turn aggravate OM severity (Created with Biorender.com).
Bottom-Up: Gut Microbiota Dysbiosis and Its Impact on OM
The gut microbiota refers to trillions of microorganisms found along the gastrointestinal tract [61]. Our understanding of these microbes has evolved enormously, and it is now well established that the gut microbiota controls the development and modulation of several host physiological processes including intestinal homeostasis, immune responses, and host metabolism [62]. As such, perturbations in the gut microbiota have been implicated in several intestinal and extraintestinal conditions at distant sites [63]. It has been widely demonstrated that the gut microbiota is disrupted in patients at high risk of OM, including those undergoing systemic chemotherapy or HSCT [64, 65]. While HNC local radiotherapy is not expected to cause a significant change in the gut microbiota, chemoradiotherapy regimens may lead to gut microbial dysbiosis in patients with HNC. Currently, only one study has investigated the impact of chemoradiotherapy on the gut microbiota of patients with oropharyngeal cancer and reported no changes in the gut microbiota post chemoradiotherapy [66]. However, this was only evaluated in a small sample size (N = 22) with limited resolution; hence further research is required.
Since the gut microbiota plays a critical role in modulating systemic immune and inflammatory responses, it may influence the development and/or severity of OM [34, 67]. However, the current evidence supporting this is limited. As discussed earlier, germ-free mice (lacking both oral and gut microbiota) are less susceptible to oral injury and inflammation following chemotherapy [51]. Similarly, we have shown that treating rats with broad-spectrum antibiotics in drinking water, to deplete the gut microbiota, decreased radiation-induced OM severity by reducing the inflammatory cytokines in tongue tissues [68]. Although it is difficult to dissect whether these findings are due to changes in the oral or gut microbiota, the immunomodulatory capacity of the gut microbiota is undoubtedly larger than that of the oral microbiota. As such, it is likely that the gut microbiota plays an important role in OM pathobiology. In fact, this concept is supported by more recent evidence which used a more targeted approach to deplete the gut microbiota using intragastric antibiotics. Mice exposed to antibiotics had reduced epithelial damage and immune cell infiltration in the tongue after irradiation, indicating that gut microbiota is implicated in OM development [69]. Minimal effects of intragastric antibiotics on the oral microbiota were reported suggesting that the protective effect is mainly mediated by the gut microbiota depletion independent of the oral microbiota [69].
Mechanistically, it is well-known that gut microbiota plays a pivotal role in maintaining intestinal homeostasis and enhancing intestinal barrier integrity [62]. Therefore, the disruption of the steady-state balance of the gut microbiota could indirectly influence OM by further aggravating the disruption of intestinal integrity caused by anticancer agents and hence activating systemic immune responses [70]. A recent study demonstrated that the restoration of the gut microbiota using ingested probiotics reduced the severity of OM in patients with nasopharyngeal cancer receiving chemoradiotherapy and in a rat model of radiation-induced OM through reducing of OM-associated inflammation [71]. Collectively, growing research indicates that gut microbiota could play a role in OM pathology (Figure 1); however, further research in this field is needed.
Top-Down: Interaction Between the Oral and Gut Microbiota During OM
The oral and gut microbiota are composed of distinctive microbial load and taxa. However, the interconnected nature of the alimentary tract provides a potential route of oral bacteria transfer into the distal digestive tract. It has been hypothesized that oral microbiota can translocate into the gut through either the enteral (gastrointestinal tract) or the hematological route (blood) [72, 73]. Current evidence suggests that more than half of oral microbes are subjected to oral-gut translocation even in healthy individuals [74]. However, more pronounced ectopic displacement occurs under pathological conditions such as periodontitis and severe systemic inflammatory disorders [75]. Oral microbiota colonization of the gut, also known as the oralization of the gut microbiota, has been linked to several conditions including liver cirrhosis [76] and colorectal cancer [77]. The translocation of oral pathobionts could result in gut microbial dysbiosis and potentially disrupt intestinal immune homeostasis, hence affecting gastrointestinal [78] and systemic inflammatory diseases [79]. For instance, the administration of P. gingivalis was found to cause a significant gut microbiota dysbiosis, reduce the expression of intestinal tight junction proteins and increase the risk of endotoxemia [80, 81]. Collectively, oral bacteria translocation is increased in pathological conditions and could cause gut microbiota dysbiosis and disruption of intestinal homeostasis.
Oral microbiota translocation during OM is yet to be investigated. Nevertheless, an increase in oral bacteria in the gut has been reported following cancer treatments [82, 83]. It has been demonstrated that oral Firmicutes (Veillonella parvula and Solobacterium moorei) and Actinobacteria (Rothia mucilaginosa) are detected in the stool of patients undergoing HSCT and are associated with the severity of acute graft-vs.-host disease [82, 83]. Since OM is associated with major changes in the oral environment and oral microbial community, translocation of dysbiotic oral bacteria into the gut is likely to occur. This in turn could contribute to pathological changes in the gut and activation of systemic immune responses and hence negatively affect OM (Figure 1). As such, further research investigating oral microbial translocation in patients at risk of OM and whether that has any implications in OM pathogenies is warranted.
Targeting The Oral-Gut Microbiota Axis in OM
Since the recognition of the potential role of oral bacteria in the pathogenesis of OM, multiple attempts to use antiseptic and antimicrobial agents to treat or prevent OM in patients undergoing cancer treatments have been made with limited success [84–87]. The lack of benefit seen in these studies could be due to the use of non-targeted antimicrobial agents. Further, the use of antibiotics could disrupt the oral microbial ecosystem affecting both commensal and pathobiont microbes and hence may have overall detrimental effects on OM. As such, the use of alternative methods such as probiotics has been explored [21, 88, 89]. In a recent systematic review, which included five clinical trials, probiotics reduced the risk of all OM grades with a more significant result for grade ≥3 [90]. Probiotics could be used to manipulate oral and gut microbiota to improve both oral and intestinal homeostasis. For instance, administration of probiotic feed containing Bacillus subtilis, Bifidobacterium bifidum, Enterococcus faecium, and Lactobacilllus acidophilus, has been shown to enhance OM regression and reduce both oral and intestinal inflammation and intestinal villus-related damage in a rat model of chemotherapy-induced OM [91]. Probiotics are a safe method for modulating the microbiota; however, the risk of infections should be taken into consideration, especially in immunocompromised patients. Although, it should be appreciated that a damaged microbiota is predictive of infection in immunocompromised patients, and as such, probiotics may counterintuitively serve to reduce infection risk.
Another way to modulate gut microbiota is through diet. Given that reduction of oral intake is one of the main OM complications, changes in dietary habits are likely to have a significant impact on the gut microbiota. Andersen et al. demonstrated that reduced oral intake post hematopoietic progenitor cell transplantation was associated with a shift in the microbial composition with a lower gut microbial diversity and lower abundance of Blautia and Faecalibacterium prausnitzii [92]. Furthermore, compared to parenteral nutrition, enteral nutrition was associated higher abundance of short-chain fatty acids-producing Faecalibacterium and Ruminococcus bromii [92] and faster recovery of the gut microbiota structure [93]. Therefore, further research is needed to determine the best nutritional support that enriches the oral and gut microbiota symbiosis of patients suffering from OM.
Fecal microbiota transplant (FMT) and more recently oral microbiota transplant (OMT) are also possible ways to restore microbial symbiosis. While FMT is a more well-established procedure, it is yet to be investigated for mitigation of OM. Further, only one study has demonstrated that OMT from healthy mice into irradiated mice was able to reduce OM-associated epithelial injury and oral and systemic inflammation by mitigating irradiation-induced alteration in both oral and gut microbiota [69]. Further research is warranted for both FMT and OMT as they hold significant potential as do other emerging strategies such as photobiomodulation [94, 95].
Conclusion
Cancer treatment-induced OM remains a major complication with significant personal, clinical, and economic burdens. Growing evidence indicates that the oral microbiota is altered following cancer treatment and may be involved in OM pathogenesis. Further, there is mounting evidence for the role of the gut microbiota contributing to OM pathogenesis through the regulation of systemic immune responses. Moreover, intestinal dysfunction caused by cancer treatment or oralization of gut microbiota could exacerbate the severity of OM. Further research is warranted to further investigate these oral-gut microbiome axis pathways and identify the best targeting intervention to prevent or reduce the severity of OM.
Author Contributions
GA-Q conceptualized, wrote, and contributed to editing the manuscript. HW, YV, and JB contributed to drafting and revising the manuscript. All authors contributed to the article and approved the submitted version.
Conflict of Interest
The authors declare that the research was conducted in the absence of any commercial or financial relationships that could be construed as a potential conflict of interest.
Publisher's Note
All claims expressed in this article are solely those of the authors and do not necessarily represent those of their affiliated organizations, or those of the publisher, the editors and the reviewers. Any product that may be evaluated in this article, or claim that may be made by its manufacturer, is not guaranteed or endorsed by the publisher.
References
1. Sonis ST. Regimen-related gastrointestinal toxicities in cancer patients. Curr Opin Support Palliat Care. (2010) 4:26.iat doi: 10.1097/SPC.0b013e328335fb76
2. Elting LS, Cooksley CD, Chambers MS, Garden AS. Risk, outcomes, and costs of radiation-induced oral mucositis among patients with head-and-neck malignancies. Int J Radiat Oncol Biol Phys. (2007) 68:1110.. Phdoi: 10.1016/j.ijrobp.2007.01.053
3. Maria OM, Eliopoulos N, Muanza T. Radiation-induced oral mucositis. Front Oncol. (2017) 7:89. doi: 10.3389/fonc.2017.00089
4. Villa A, Sonis ST. Pharmacotherapy for the management of cancer regimen-related oral mucositis. Expert Opin Pharmacother. (2016) 17:1801er.cdoi: 10.1080/14656566.2016.1217993
5. Chaudhry HM, Bruce AJ, Wolf RC, Litzow MR, Hogan WJ, Patnaik MS, et al. The incidence and severity of oral mucositis among allogeneicllogeneicand severity of oral mucositis among allogeneicogeneicmong alloBiol Blood Marrow Transplant. (2016) 22:605–16. doi: 10.1016/j.bbmt.2015.09.014
6. Laheij AMGA, de Soet JJ., von dem Borne PA, Kuijper EJ, Kraneveld EA, van Loveren C, et al. Oral bacteria and yeasts in relationship to oral ulcerations in hematopoietic stem cell transplant recipients. Support Care Cancer. (2012) 20:3231ancerdoi: 10.1007/s00520-012-1463-2
7. Trotti A, Bellm LA, Epstein JB, Frame D, Fuchs HJ, Gwede CK, et al. Mucositis incidence, severity and associated outcomes in patients with head and neck cancer receiving radiotherapy with or without chemotherapy: a systematic literature review. Radiother Oncol. (2003) 66:253l.ritdoi: 10.1016/S0167-8140(02)00404-8
8. Elad S, Zadik Y, Hewson I, Hovan A, Correa ME, Logan R, et al. A systematic review of viral infections associated with oral involvement in cancer patients: a spotlight on Herpesviridea. Support Care Cancer. (2010) 18:993ancer. doi: 10.1007/s00520-010-0900-3
9. Lalla RV, Latortue MC, Hong CH, Ariyawardana A, D'Amato-Palumbo S, Fischer DJ, et al. A systematic review of oral fungal infections in patients receiving cancer therapy. Support Care Cancer. (2010) 18:985ance
10. Thomas K, Martin T, Gao A, Ahn C, Wilhelm H, Schwartz DL. Interruptions of head and neck radiotherapy across insured and indigent patient populations. J Oncol Pract. (2017) 13:e319Schwardoi: 10.1200/JOP.2016.017863
11. Russo G, Haddad R, Posner M, Machtay M. Radiation treatment breaks and ulcerative mucositis in head and neck cancer. Oncologist. (2008) 13:886eck cdoi: 10.1634/theoncologist.2008-0024
12. Elting LS, Chang YC. Costs of oral complications of cancer therapies: estimates and a blueprint for future study. J Natl Cancer Inst Monogr. (2019) 2019:lgz010. doi: 10.1093/jncimonographs/lgz010
13. Bowen J, Al-Dasooqi N, Bossi P, Wardill H, Van Sebille Y, Al-Azri A, et al. The pathogenesis of mucositis: updated perspectives and emerging targets. Support Care Cancer. (2019) 27:4023ncer. doi: 10.1007/s00520-019-04893-z
15. Sonis ST, Elting LS, Keefe D, Peterson DE, Schubert M, HauerKeefe D, P D, Peterson DE, Schubert M Hauer ositiscositisucositiscositisCancer. (2004) 100:1995terson doi: 10.1002/cncr.20162
16. Sonis ST. Pathobiology of oral mucositis: novel insights and opportunities. J Support Oncol. (2007) 5(Suppl. 4):3–11.
17. Cinausero M, Aprile G, Ermacora P, Basile D, Vitale MG, Fanotto V, et al. New frontiers in the pathobiology and treatment of cancer regimen-related mucosal injury. Front Pharmacol. (2017) 8:354. doi: 10.3389/fphar.2017.00354
18. Oronsky B, Goyal S, Kim MM, Cabrales P, Lybeck M, Caroen S, et al. A review of clinical radioprotection and chemoprotection for oral mucositis. Transl Oncol. (2018) 11:771sit doi: 10.1016/j.tranon.2018.03.014
19. Sonis ST. Mucositis: The impact, biology and therapeutic opportunities of oral mucositis. Oral Oncol. (2009) 45:1015iologdoi: 10.1016/j.oraloncology.2009.08.006
20. Rice DH, Gill G. The effect of irradiation upon the bacterial flora in patients with head and neck cancer. Laryngoscope. (1979) 89:1839. Thedoi: 10.1288/00005537-197911000-00018
21. Vasconcelos RM, Sanfilippo N, Paster BJ, Kerr AR Li Y, Ramalho L, et al. Host-microbiome cross-talk in oral mucositis. J Dent Res. (2016) 95:725 oraldoi: 10.1177/0022034516641890
22. Stringer AM, Logan RM. The role of oral flora in the development of chemotherapy-induced oral mucositis. J Oral Pathol Med. (2015) 44:81 Me doi: 10.1111/jop.12152
23. Saunders DP, Epstein JB, Elad S, Allemano J, Bossi P, van de Wetering MD, et al. Systematic review of antimicrobials, mucosal coating agents, anesthetics, and analgesics for the management of oral mucositis in cancer patients. Support Care Cancer. (2013) 21:3191ncer. doi: 10.1007/s00520-013-1871-y
24. Mougeot J-LC, Stevens CB, Morton DS, Brennan MT, Mougeot FB. Oral microbiome and cancer therapy-induced oral mucositis. JNCI Monographs. (2019) 2019:lgz002. doi: 10.1093/jncimonographs/lgz002
25. Al-Dasooqi N, Sonis ST, Bowen JM, Bateman E, Blijlevens N, Gibson RJ, et al. Emerging evidence on the pathobiology of mucositis. Support Care Cancer. (2013) 21:2075ncer.doi: 10.1007/s00520-013-1810-y
26. Wardill HR, van der Aa SAR, da Silva Ferreira AR, Havinga R, Tissing WJE, Harmsen HJM. Antibiotic-induced disruption of the microbiome exacerbates chemotherapy-induced diarrhoea and can be mitigated with autologous faecal microbiota transplantation. Eur J Cancer. (2021) 153:27on. adoi: 10.1016/j.ejca.2021.05.015
27. Deo PN, Deshmukh R. Oral microbiome: unveiling the fundamentals. J Oral Maxillofac Pathol. (2019) 23:122hol doi: 10.4103/jomfp.JOMFP_304_18
28. Arweiler NB, Netuschil L. The oral microbiota. Adv Exp Med Biol. (2016) 902:45ol. Tdoi: 10.1007/978-3-319-31248-4_4
29. Segata N, Haake SK, Mannon P, Lemon KP, Waldron L, Gevers D, et al. Composition of the adult digestive tract bacterial microbiome based on seven mouth surfaces, tonsils, throat and stool samples. Genome Biol. (2012) 13:R42. doi: 10.1186/gb-2012-13-6-r42
30. Marsh PD, Percival RS. The oral microflora–friend or foe? Can we decide? Int Dent J. (2006) 56(Suppl. 1):233–9. doi: 10.1111/j.1875-595X.2006.tb00107.x
31. Willis JR, Gabald J T. The human oral microbiome in health and disease: from sequences to ecosystems. Microorganisms. (2020) 8:308. doi: 10.3390/microorganisms8020308
32. Nape.33 JJ, Brennan MT, Bahrani-Mougeot FK, Fox PC, Lockhart PB. Relationship between mucositis and changes in oral microflora during cancer chemotherapy. Oral Surg Oral Med Oral Pathol Oral Radiol Endod. (2007) 103:48ndod.doi: 10.1016/j.tripleo.2005.12.016
33. Ye Y, Carlsson G, Agholme MB, Wilson JA, Roos A, Henriques-Normark B, et al. Oral bacterial community dynamics in paediatric patients with malignancies in relation to chemotherapy-related oral mucositis: a prospective study. Clin Microbiol Infect. (2013) 19:E559t..ecdoi: 10.1111/1469-0691.12287
34. Laheij AM, de Soet JJ. Can the oral microflora affect oral ulcerative mucositis? Curr Opin Support Palliat Care. (2014) 8:180iat doi: 10.1097/SPC.0000000000000053
35. Vanhoecke B, De Ryck T, Stringer A, Van de Wiele T, Keefe D. Microbiota and their role in the pathogenesis of oral mucositis. Oral Dis. (2015) 21:17le indoi: 10.1111/odi.12224
36. Guobis uobKareivien V, Basevisevio N, Paipalienl P, Niedzelskienk I, Sabalys G, et al. Microflora of the oral cavity in patients with xerostomia. Medicina (Kaunas). (2011) 47:646a51. doi: 10.3390/medicina47120094
37. Ingrosso G, Saldi S, Marani S, Wong AYW, Bertelli M, Aristei C, et al. Breakdown of symbiosis in radiation-induced oral mucositis. J Fungi. (2021) 7:290. doi: 10.3390/jof7040290
38. Yadav BS, Ronda V, Vashista DP, Sharma B. Sequencing and computational approaches to identification and characterization of microbial organisms. Biomed Eng Comput Biol. (2013) 5:43.ol.doi: 10.4137/BECB.S10886
39. Nape.41 JJ, Brennan MT, Coleman S, Kent ML, Noll J, Frenette G, et al. Molecular methodology to assess the impact of cancer chemotherapy on the oral bacterial flora: a pilot study. Oral Surg Oral Med Oral Pathol Oral Radiol Endod. (2010) 109:554dod.Edoi: 10.1016/j.tripleo.2009.11.015
40. Hu YJ, Shao ZY, Wang Q, Jiang YT, Ma R, Tang ZS, et al. Exploring the dynamic core microbiome of plaque microbiota during head-and-neck radiotherapy using pyrosequencing. PLoS ONE. (2013) 8:e56343. doi: 10.1371/journal.pone.0056343
41. Hong BY, Sobue T, Choquette L, Dupuy AK, Thompson A, Burleson JA, et al. Chemotherapy-induced oral mucositis is associated with detrimental bacterial dysbiosis. Microbiome. (2019) 7:66. doi: 10.1186/s40168-019-0679-5
42. Osakabe L, Utsumi A, Saito B, Okamatsu Y, Kinouchi H, Nakamaki T, et al. Influence of oral anaerobic bacteria on hematopoietic stem cell transplantation patients: oral mucositis and general condition. Transplant Proc. (2017) 49:2176.ditidoi: 10.1016/j.transproceed.2017.09.012
43. Zhu XX, Yang XJ, Chao YL, Zheng HM, Sheng HF, Liu HY, et al. The potential effect of oral microbiota in the prediction of mucositis during radiotherapy for nasopharyngeal carcinoma. EBioMedicine. (2017) 18:23theradoi: 10.1016/j.ebiom.2017.02.002
44. Hou J, Zheng H, Li P, Liu H, Zhou H, Yang X. Distinct shifts in the oral microbiota are associated with the progression and aggravation of mucositis during radiotherapy. Radiother Oncol. (2018) 129:44l.rapdoi: 10.1016/j.radonc.2018.04.023
45. Vesty A, Gear K, Biswas K, Mackenzie BW, Taylor MW, Douglas RG. Oral microbial influences on oral mucositis during radiotherapy treatment of head and neck cancer. Support Care Cancer. (2020) 28:2683ncer.doi: 10.1007/s00520-019-05084-6
46. Laheij A, Raber-Durlacher JE, Koppelmans RGA, Huysmans M, Potting C, van Leeuwen SJM, et al. Microbial changes in relation to oral mucositis in autologous hematopoietic stem cell transplantation recipients. Sci Rep. (2019) 9:16929. doi: 10.1038/s41598-019-53073-w
47. Mougeot JC, Beckman MF, Stevens CB, Almon KG, Morton DS, Von BKG Morton DS I, et al. Lasting Gammaproteobacteria profile changes characterized hematological cancer patients who developed oral mucositis following conditioning therapy. J Oral Microbiol. (2020) 12:1761135. doi: 10.1080/20002297.2020.1761135
48. Reyes-Gibby CC, Wang J, Zhang L, Peterson CB, Do KA, Jenq RR, et al. Oral microbiome and onset of oral mucositis in patients with squamous cell carcinoma of the head and neck. Cancer. (2020) 126:5124h squdoi: 10.1002/cncr.33161
49. Shouval R, Eshel A, Dubovski B, Kuperman AA, Danylesko I, Fein JA, et al. Patterns of salivary microbiota injury and oral mucositis in recipients of allogeneic hematopoietic stem cell transplantation. Blood Adv. (2020) 4:2912 of doi: 10.1182/bloodadvances.2020001827
50. Takahashi M, Toyosaki M, Matsui K, Machida S, Kikkawa E, Ota Y, et al. An analysis of oral microbial flora by T-RFLP in patients undergoing hematopoietic stem cell transplantation. Int J Hematol. (2020) 112:690l. Adoi: 10.1007/s12185-020-02958-y
51. Gupta N, Quah SY, Yeo JF, Ferreira J, Tan KS, Hong CHL. Role of oral flora in chemotherapy-induced oral mucositis in vivo. Arch Oral Biol. (2019) 101:51.vo.doi: 10.1016/j.archoralbio.2019.03.008
52. Vanlancker E, Vanhoecke B, Sieprath T, Bourgeois J, Beterams A, De Moerloose B, et al. Oral microbiota reduce wound healing capacity of epithelial monolayers, irrespective of the presence of 5-fluorouracil. Exp Biol Med. (2018) 243:350ealindoi: 10.1177/1535370217753842
53. Haverman TM, Laheij AMGA, de Soet JJ, de Lange J, Rozema FR. Candida and Porphyromonas gingivalis: the effect on wound closure in vitro. J Oral Microbiol. (2017) 9:1328266. doi: 10.1080/20002297.2017.1328266
54. Laheij AMGA, de Soet JJ, Veerman ECI, Bolscher JGM, van Loveren C. The influence of oral bacteria on epithelial cell migration < i>in vitro < /i>. Mediators Inflamm. (2013) 2013:154532. doi: 10.1155/2013/154532
55. Boussios S, Pentheroudakis G, Katsanos K, Pavlidis N. Systemic treatment-induced gastrointestinal toxicity: incidence, clinical presentation and management. Ann Gastroenterol. (2012) 25:106rol.
56. Fern12) 2-Gil B, Moneim AE, Ortiz F, Shen YQ, Soto-Mercado V, Mendivil-Perez M, et al. Melatonin protects rats from radiotherapy-induced small intestine toxicity. PLoS ONE. (2017) 12:e0174474. doi: 10.1371/journal.pone.0174474
57. Wardill HR, Van Sebille YZ, Mander KA, Gibson RJ, Logan RM, Bowen JM, et al. Toll-like receptor 4 signaling: a common biological mechanism of regimen-related toxicities: an emerging hypothesis for neuropathy and gastrointestinal toxicity. Cancer Treat Rev. (2015) 41:122ev.odoi: 10.1016/j.ctrv.2014.11.005
58. Wardill HR, Mander KA, Van Sebille YZ, Gibson RJ, Logan RM, Bowen JM, et al. Cytokine-mediated blood brain barrier disruption as a conduit for cancer/chemotherapy-associated neurotoxicity and cognitive dysfunction. Int J Cancer. (2016) 139:2635Logandoi: 10.1002/ijc.30252
59. Jordan K, Pontoppidan P, Uhlving HH, Kielsen K, Burrin DG, Weischendorff S, et al. Gastrointestinal toxicity, systemic inflammation, and liver biochemistry in allogeneic hematopoietic stem cell transplantation. Biol Blood Marrow Transplant. (2017) 23:1170ransdoi: 10.1016/j.bbmt.2017.03.021
60. An L, Wuri J, Zheng Z, Li W, Yan T. Microbiota modulate Doxorubicin induced cardiotoxicity. Eur J Pharm Sci. (2021) 166:105977. doi: 10.1016/j.ejps.2021.105977
61. Marchesi JR, Ravel J. The vocabulary of microbiome research: a proposal. Microbiome. (2015) 3:31. doi: 10.1186/s40168-015-0094-5
62. Sommer F BF, Br F. The gut microbiota–masters of host development and physiology. Nat Rev Microbiol. (2013) 11:227iol.ddoi: 10.1038/nrmicro2974
63. Lazar V, Ditu L-M, Pircalabioru GG, Gheorghe I, Curutiu C, Holban AM, et al. Aspects of gut microbiota and immune system interactions in infectious diseases, immunopathology, and cancer. Front Immunol. (2018) 9:1830. doi: 10.3389/fimmu.2018.01830
64. Secombe KR, Coller JK, Gibson RJ, Wardill HR, Bowen JM. The bidirectional interaction of the gut microbiome and the innate immune system: Implications for chemotherapy-induced gastrointestinal toxicity. Int J Cancer. (2019) 144:2365immundoi: 10.1002/ijc.31836
65. Zama D, Bossù G, Leardini D, Muratore E, Biagi E, Prete A, et al. Insights into the role of intestinal microbiota in hematopoietic stem-cell transplantation. Ther Adv Hematol. (2020) 11:2040620719896961. doi: 10.1177/2040620719896961
66. Oliva M, Schneeberger PHH, Rey V, Cho M, Taylor R, Hansen AR, et al. Transitions in oral and gut microbiome of HPV+ oropharyngeal squamous cell carcinoma following definitive chemoradiotherapy (ROMA LA-OPSCC study). Br J Cancer. (2021) 124:1543studydoi: 10.1038/s41416-020-01253-1
67. Al-Qadami G, Van Sebille Y, Le H, Bowen J. Gut microbiota: implications for radiotherapy response and radiotherapy-induced mucositis. Expert Rev Gastroenterol Hepatol. (2019) 13:485Hepatdoi: 10.1080/17474124.2019.1595586
68. Al-Qadami G, Verma G, Van Sebille Y, Le H, Hewson I, Bowen J. Antibiotic-induced depletion of gut microbiota reduces injury area and duration of radiation-induced oral mucositis in rats. Supportive Care in Cancer. (2021) 29:S106–S107. doi: 10.1007/s00520-021-06285-8
69. Xiao H, Fan Y, Li Y, Dong J, Zhang S, Wang B, et al. Oral microbiota transplantation fights against head and neck radiotherapy-induced oral mucositis in mice. Comput Struct Biotechnol J. (2021) 19:5898ol J.doi: 10.1016/j.csbj.2021.10.028
70. van Vliet MJ, Harmsen HJ, de Bont ES, Tissing WJ. The role of intestinal microbiota in the development and severity of chemotherapy-induced mucositis. PLoS Pathog. (2010) 6:e1000879. doi: 10.1371/journal.ppat.1000879
71. Xia C, Jiang C, Li W, Wei J, Hong H, Li J, et al. A phase II randomized clinical trial and mechanistic studies using improved probiotics to prevent oral mucositis induced by concurrent radiotherapy and chemotherapy in nasopharyngeal carcinoma. Front Immunol. (2021) 12:618150. doi: 10.3389/fimmu.2021.618150
72. Lu M, Xuan S, Wang Z. Oral microbiota: a new view of body health. Food Sci Hum Wellness. (2019) 8:8ss.ssdoi: 10.1016/j.fshw.2018.12.001
73. Khor B, Snow M, Herrman E, Ray N, Mansukhani K, Patel KA, et al. Interconnections between the oral and gut microbiomes: reversal of microbial dysbiosis and the balance between systemic health and disease. Microorganisms. (2021) 9:496. doi: 10.3390/microorganisms9030496
74. Schmidt TS, Hayward MR, Coelho LP, Li SS, Costea PI, Voigt AY, et al. Extensive transmission of microbes along the gastrointestinal tract. Elife. (2019) 8:e42693. doi: 10.7554/eLife.42693
75. Olsen I, Yamazaki K. Can oral bacteria affect the microbiome of the gut? J Oral Microbiol. (2019) 11:1586422. doi: 10.1080/20002297.2019.1586422
76. Qin N, Yang F, Li A, Prifti E, Chen Y, Shao L, et al. Alterations of the human gut microbiome in liver cirrhosis. Nature. (2014) 513:59hosisdoi: 10.1038/nature13568
77. Flemer B, Warren RD, Barrett MP, Cisek K, Das A, Jeffery IB, et al. The oral microbiota in colorectal cancer is distinctive and predictive. Gut. (2018) 67:1454cancedoi: 10.1136/gutjnl-2017-314814
78. Park S-Y, Hwang B-O, Lim M, Ok S-H, Lee S-K, Chun K-S, et al. Oral-gut microbiome axis in gastrointestinal disease and cancer. Cancers. (2021) 13:2124. doi: 10.3390/cancers13092124
79. du Teil Espina M, Gabarrini G, Harmsen HJM, Westra J, van Winkelhoff AJ, van Dijl JM. Talk to your gut: the oral-gut microbiome axis and its immunomodulatory role in the etiology of rheumatoid arthritis. FEMS Microbiol Rev. (2019) 43:1v. Redoi: 10.1093/femsre/fuy035
80. Arimatsu K, Yamada H, Miyazawa H, Minagawa T, Nakajima M, Ryder MI, et al. Oral pathobiont induces systemic inflammation and metabolic changes associated with alteration of gut microbiota. Sci rep. (2014) 4:4828. doi: 10.1038/srep04828
81. Nakajima M, Arimatsu K, Kato T, Matsuda Y, Minagawa T, Takahashi N, et al. Oral Administration of P. gingivalis Induces Dysbiosis of Gut Microbiota and Impaired Barrier Function Leading to Dissemination of Enterobacteria to the Liver. PLoS ONE. (2015) 10:e0134234. doi: 10.1371/journal.pone.0134234
82. Beckman MF, Morton DS, Bahrani Mougeot F, Mougeot JC. Allogenic stem cell transplant-associated acute graft versus host disease: a computational drug discovery text mining approach using oral and gut microbiome signatures. Support Care Cancer. (2021) 29:1765ncer.doi: 10.1007/s00520-020-05821-2
83. Golob JL, Pergam SA, Srinivasan S, Fiedler TL, Liu C, Garcia K, et al. Stool microbiota at neutrophil recovery is predictive for severe acute graft vs host disease after hematopoietic cell transplantation. Clin Infect Dis. (2017) 65:1984.ter doi: 10.1093/cid/cix699
84. Spijkervet FK, van Saene HK, Panders AK, Vermey A, van Saene JJ, Mehta DM, et al. Effect of chlorhexidine rinsing on the oropharyngeal ecology in patients with head and neck cancer who have irradiation mucositis. Oral Surg Oral Med Oral Pathol. (1989) 67:154 Pathdoi: 10.1016/0030-4220(89)90321-6
85. Pitten FA, Kiefer T, Buth C, Doelken G, Kramer A. Do cancer patients with chemotherapy-induced leukopenia benefit from an antiseptic chlorhexidine-based oral rinse? A double-blind, block-randomized, controlled study. J Hosp Infect. (2003) 53:283. blodoi: 10.1053/jhin.2002.1391
86. Wijers OB, Levendag PC, Harms ER, Gan-Teng AM, Schmitz PI, Hendriks WD, et al. Mucositis reduction by selective elimination of oral flora in irradiated cancers of the head and neck: a placebo-controlled double-blind randomized study. Int J Radiat Oncol Biol Phys. (2001) 50:343s.. Pdoi: 10.1016/S0360-3016(01)01444-4
87. Stokman MA, Spijkervet FK, Burlage FR, Dijkstra PU, Manson WL, de Vries EG, et al. Oral mucositis and selective elimination of oral flora in head and neck cancer patients receiving radiotherapy: a double-blind randomised clinical trial. Br J Cancer. (2003) 88:1012. aldoi: 10.1038/sj.bjc.6600824
88. Cereda E, Caraccia M, Caccialanza R. Probiotics and mucositis. Curr Opin Clin Nutr Metab Care. (2018) 21:399 Meer doi: 10.1097/MCO.0000000000000487
89. Yarom N, Hovan A, Bossi P, Ariyawardana A, Jensen SB, Gobbo M, et al. Systematic review of natural and miscellaneous agents, for the management of oral mucositis in cancer patients and clinical practice guidelines - part 2: honey, herbal compounds, saliva stimulants, probiotics, and miscellaneous agents. Support Care Cancer. (2020) 28:2457ncer.doi: 10.1007/s00520-019-05256-4
90. Shu Z, Li P, Yu B, Huang S, Chen Y. The effectiveness of probiotics in prevention and treatment of cancer therapy-induced oral mucositis: a systematic review and meta-analysis. Oral Oncol. (2020) 102:104559. doi: 10.1016/j.oraloncology.2019.104559
91. Gerhard D, Sousa F, Andraus RAC, Pardo PE, Nai GA, Neto HB, et al. Probiotic therapy reduces inflammation and improves intestinal morphology in rats with induced oral mucositis. Braz Oral Res. (2017) 31:e71. doi: 10.1590/1807-3107bor-2017.vol31.0071
92. Andersen S, Staudacher H, Weber N, Kennedy G, Varelias A, Banks M, et al. Pilot study investigating the effect of enteral and parenteral nutrition on the gastrointestinal microbiome post-allogeneic transplantation. Br J Haematol. (2020) 188:570c tradoi: 10.1111/bjh.16218
93. D'Amico F, Biagi E, Rampelli S, Fiori J, Zama D, Soverini M, et al. Enteral nutrition in pediatric patients undergoing hematopoietic SCT promotes the recovery of gut microbiome homeostasis. Nutrients. (2019) 11:2958. doi: 10.3390/nu11122958
94. Zanotta N, Ottaviani G, Campisciano G, Poropat A, Bovenzi M, Rupel K, et al. Photobiomodulation modulates inflammation and oral microbiome: a pilot study. Biomarkers. (2020) 25:677y. madoi: 10.1080/1354750X.2020.1825812
Keywords: oral mucositis, chemotherapy, radiotherapy, HSCT, oral microbiota, gut microbiota, oral-gut microbiome axis
Citation: Al-Qadami G, Van Sebille Y, Bowen J and Wardill H (2022) Oral-Gut Microbiome Axis in the Pathogenesis of Cancer Treatment-Induced Oral Mucositis. Front. Oral. Health 3:881949. doi: 10.3389/froh.2022.881949
Received: 23 February 2022; Accepted: 07 March 2022;
Published: 28 March 2022.
Edited by:
Nathaniel Simon Treister, Brigham and Women's Hospital and Harvard Medical School, United StatesReviewed by:
Ourania Nicolatou-Galitis, University of Athens, GreeceCopyright © 2022 Al-Qadami, Van Sebille, Bowen and Wardill. This is an open-access article distributed under the terms of the Creative Commons Attribution License (CC BY). The use, distribution or reproduction in other forums is permitted, provided the original author(s) and the copyright owner(s) are credited and that the original publication in this journal is cited, in accordance with accepted academic practice. No use, distribution or reproduction is permitted which does not comply with these terms.
*Correspondence: Ghanyah Al-Qadami, ghanyah.al-qadami@adelaide.edu.au