- 1Department of Periodontology, Justus-Liebig-University of Giessen, Giessen, Germany
- 2Department of Orthodontics, Justus-Liebig-University of Giessen, Giessen, Germany
Periodontitis is an oral chronic inflammatory disease and may cause tooth loss in adults. Oral epithelial cells provide a barrier for bacteria and participate in the immune response. Fusobacterium nucleatum (F. nucleatum) is one of the common inhabitants of the oral cavity and has been identified as a potential etiologic bacterial agent of oral diseases, such as periodontitis and oral carcinomas. F. nucleatum has been shown to be of importance in the development of diverse human cancers. In the dental biofilm, it exhibits a structural role as a bridging organism, connecting primary colonizers to the largely anaerobic secondary colonizers. It expresses adhesins and is able to induce host cell responses, including the upregulation of defensins and the release of chemokines and interleukins. Like other microorganisms, its detection is achieved through germline-encoded pattern-recognition receptors (PRRs) and pathogen-associated molecular patterns (PAMPs). By identification of the pathogenic mechanisms of F. nucleatum it will be possible to develop effective methods for the diagnosis, prevention, and treatment of diseases in which a F. nucleatum infection is involved. This review summarizes the recent progress in research targeting F. nucleatum and its impact on oral epithelial cells.
Introduction
The oral epithelium as part of the masticatory mucosa, the lining mucosa, and the specialized mucosa, provides a barrier that separates the oral soft tissues from the environment. This barrier is the result of a number of functional and structural protein interactions that result in the capability to react to numerous exogenous, possibly toxic, influences [1]. It is actually known that epithelial cells are not only passive bystanders, but are able of responding to external stimuli by producing a number of cytokines, adhesion molecules, growth factors, chemokines, and matrix metalloproteases [2]. Fusobacterium nucleatum, a Gram-negative obligate anaerobic bacterium, belongs to the genus Fusobacterium, which normally lives parasitically in the oral cavity, urogenital tract, soil, intestinal tract, and upper digestive tract, but most commonly in oral plaque. It is frequently present in the oral cavity, in diseased as well as healthy individuals [3].
In the past, F. nucleatum has been regarded as a component of the normal flora of the human body. Due to the continuous isolation of the bacterium from clinical samples, F. nucleatum has attracted attention from researchers and has been recognized as a bacterium that should not be ignored. It was verified that F. nucleatum is an opportunistic pathogen with strong pathogenicity. F. nucleatum is frequently detected in oral and systemic infections. It is associated with various human diseases, such as periodontitis, angina, lung abscesses, chronic otitis, sinusitis, peritonsillar abscesses, cerebral abscesses, inflammatory bowel disease, ulcerative colitis, Crohn's disease, gynecological abscesses, neonatal sepsis, Lemierre's syndrome, and infective endocarditis [4–9].
In oral infections, it is widely present in infected dental pulp, periodontal, and other inflammatory lesions. Most importantly, F. nucleatum is implicated in different kinds of periodontal disease from the reversible and rather mild form of gingivitis to the more severe forms of periodontitis such as chronic and aggressive periodontitis [3]. The prevalence of F. nucleatum is higher in severe forms of the disease, with progressive inflammatory responses, and increased pocket depth. [10].
F. nucleatum is not only detected in periodontal sites but additionally in saliva, with higher prevalence in patients exhibiting gingivitis and periodontitis, in comparison to healthy controls [11]. The results of animal studies suggest a causative role of F. nucleatum in periodontal infections [3]. A mono-infection of mice with F. nucleatum induces periodontal bone loss or abscess formation [12]. Together with other oral species, synergistic effects of virulence are detected, resulting in increased bone loss, abscess development, or death [13]. It has been shown that F. nucleatum passes through the gingival epithelial cells to the endocytic pathway of degradation after the invasion. After this event, no cytopathic effect on gingival epithelial cells persists, which may be associated with the host evasion strategies of F. nucleatum in the pathogenesis of periodontitis [14]. This oral gram-negative bacterium can enter connective tissue and enhance tissue destruction through proteases as well as by inducing abundant inflammatory responses [14]. Figure 1 shows an overview of the multiple forms and components of F. nucleatum and their interactions with oral epithelial cells.
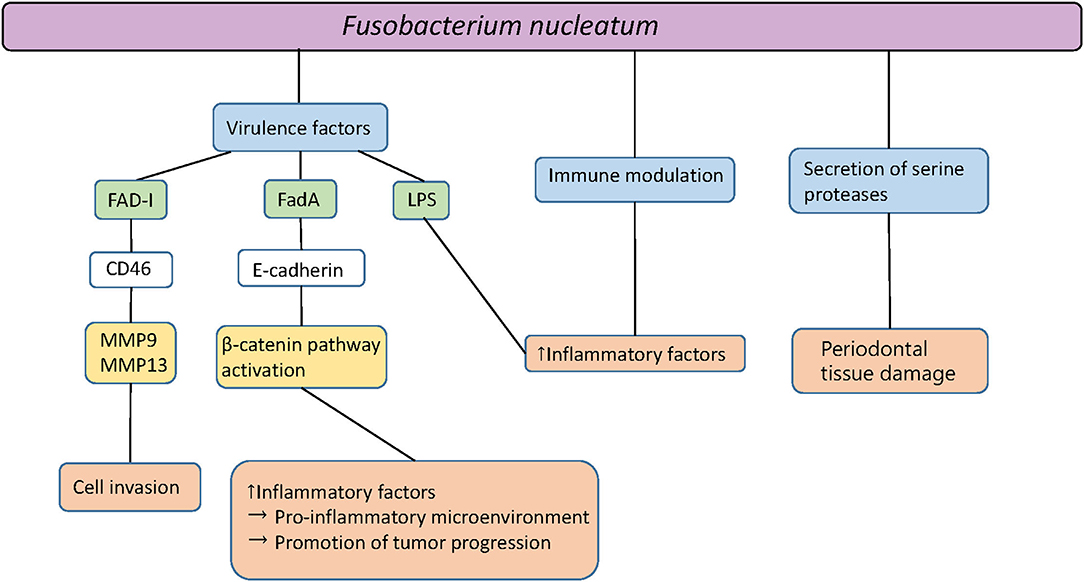
Figure 1. Pathogenicity of Fusobacterium nucleatum. Fad-I, Fusobacterium-associated defensing inducer; FadA, Fusobacterial adhesion; MMP, Matrix metalloproteinase; LPS, Lipopolysaccharides.
F. nucleatum—A Bridging Organism
In the dental bacterial biofilm, F. nucleatum plays a structural role by acting as a bridging organism, connecting the primary colonizers such as the Streptococcus species to the mostly anaerobic secondary colonizers to which it can bind as well, i.e., Porphyromonas gingivalis (P. gingivalis) and Aggregatibacter actinomycetemcomitans (A. actinomycetemcomitans) [3, 15, 16]. F. nucleatum exists in the intermediate layer of tooth-attached human plaque samples, as proposed by Kolenbrander and London [17], which also was supported by an in vivo study [18]. It can co-aggregate with almost all bacterial species that participate in oral plaque formation [19]. F. nucleatum is able to bind to and transport Streptococcus cristatus, a non-invasive bacterial species, into host cells, working as a shuttle [20]. In general, known fusobacterial adhesins (Aid1, CmpA, Fap2, FomA, FadA, and RadD) play a vital role in microbial coaggregation, mediating the invasion and facilitating the spread of bacteria [3, 6, 21–24].
RadD plays an eclectic role in fusobacterial adhesion, which has been shown as the main adhesin that mediates the attachment of numerous gram-positive early colonizers [23] and promotes fusobacterial adherence to biofilms [25]. It can bind to the S. mutans adhesin SpaP to mediate the co-aggregation of these two bacterial species and progressive biofilm organization [15, 26]. It conveys interactions not only to bacterial species but also to Candida albicans, which can also be part of the oral microbiota [15, 23, 27]. According to a microarray analysis, Kaplan et al. found that a small hypothetical protein encoded by FN1253, which they designated as Adhesion Inducing Determinant 1 (Aid1), is induced in F. nucleatum single-species biofilms. Aid1 appears to be unique to fusobacteria and potentially plays a vital role in facilitating RadD-mediated interaction with oral streptococci [28]. The interaction between F. nucleatum ATCC 23726 and S. gordonii V288 is mediated by RadD and another second outer membrane protein called Coaggregation-mediated Protein A (CmpA) [29]. While CmpA increased the expression under biofilm conditions, RadD expression was decreased, indicating that these two proteins may be involved in different physiological processes [29].
The fusobacterial adhesin (FadA) is the only adhesin that has been demonstrated to bind to host cells and, until now, remains to be the virulence factor from F. nucleatum that has been characterized the best [30]. FadA plays an essential role in inducing the tumorigenic responses and binding and invasion of host cells by the organism [31]. A synthetic peptide that can prevent FadA from binding to E-cadherin inhibits tumorigenic responses in colorectal cancer [32]. FadA binds to E-cadherin, activates β-catenin signaling, and regulates the inflammatory and oncogenic reactions in a differential manner [32–35]. F. nucleatum activates p38 MAP kinase followed by the secretion of matrix metalloproteinase (MMP)−9 and MMP-13 [33, 36–38]. MMP-9 and MMP-13 induce invasion and metastasis manifestation [6, 39]. This supports the migration of cells over the stimulation of Etk/BMX, S6 kinase p70, and RhoA kinase [40]. Liu et al. assessed the prevalence of F. nucleatum and its virulence factor FadA adhesion gene (fadA) in subgingival biofilm samples from patients with gingivitis or periodontitis with or without fixed orthodontic appliances. It was found that F. nucleatum fadA was detected in higher amounts in the periodontitis group and non-orthodontic gingivitis group compared to the other groups. It was also increased in the orthodontic gingivitis group but only compared to healthy subjects. The authors concluded that F. nucleatum carrying fadA probably plays a role in the development of non-orthodontic gingivitis and periodontitis compared with orthodontic gingivitis [41].
FomA is a major outer membrane pore protein of F. nucleatum. The increased abundance of FomA may be related to the promotion of biofilm formation [42]. FomA was also identified as the target antigen bound to F. nucleatum-specific IgA [43]. The production of anti-FomA IgG antibodies was dependent on TLR2 expression [44]. F. nucleatum invades host cells through a “zipper” mechanism that relies on a large number of adhesins [45]. However, a study found that P. gingivalis outer membrane vesicles (OMVs), reduced the expression levels of FadA and FomA through protease components and further inhibited the invasion of F. nucleatum into the oral epithelial cells [46].
Fibroblast activation protein 2 (Fap2) is a galactose-sensitive hemagglutinin and adhesin that probably participates in the virulence of Fusobacteriae [22]. Its function is critical in mediating colorectal cancer (CRC) development through binding with acetylgalactosamine (Gal-GalNAc), which is overexpressed in human metastases and colorectal adenocarcinoma [47]. It was demonstrated that the Fap2 protein of F. nucleatum directly interacts with the T cell immune receptor with Immunoglobulin G (TIGIT) expressed on NK cells and tumor-infiltrating lymphocytes, causing inhibition of natural killer (NK) cell cytotoxicity and lymphocytes activity, thus inducing the development of CRC [48, 49]. FadA interacts with endothelial cells and epithelial cells, while Fap-2 binds only to Gal-GalNAc [6, 50].
Compared with P. gingivalis, F. nucleatum demonstrated a highly invasive capacity [14]. The coinfection with F. nucleatum can enhance the adhesion and invasion of P. gingivalis and A. actinomycetemcomitans to human gingival epithelial cells and inhibits host innate immune responses [51]. The green and black tea extracts, epigallocatechin-3-gallate (EGCC), and theaflavins diminish the adherence of F. nucleatum to oral epithelial cells and matrix proteins [52]. Furthermore, these tea components also inhibit F. nucleatum-mediated hemolysis and hydrogen sulfide production, which represent two further virulence factors expressed by this bacterium [52].
F. nucleatum—Receptor Interactions
Microbial detection is achieved through germline-encoded pattern-recognition receptors (PRRs) and pathogen-associated molecular patterns (PAMPs) that surveil the extracellular as well as the intracellular area for conserved microbial components that indicate infection [53–55]. Representative bacterial PAMPs include lipid A of lipopolysaccharides (LPS), lipopeptide, and peptidoglycans (PGNs) [54]. The classification of the most PRRs is based on protein domain homology, discriminating them into one of five families, including Toll-like receptors (TLRs) and nucleotide-binding domain, leucine-rich repeat (LRR)-containing [or nucleotide-binding oligomerization domain-containing proteins (NOD)-like] receptors (NLRs) [55, 56]. TLRs are membrane-bound inborn receptors, while NLRs form the unbound intracellular receptor class [55].
Toll-like receptors are an essential class of protein molecules that participate in innate immunity, and they are also the bridge connecting innate and adaptive immune responses. TLR 1, 2, 4, 5, 6, and 10 are expressed on the cell surface and are transferred to phagosomes after being activated, whereas TLR 3, 7, 8, and 9 are expressed in intracellular compartments in nearly all cell types, principally in the endosomes and the endoplasmic reticulum, with the ligand-binding domains sampling the lumen of the vesicle [57]. In the oral cavity, both immune cells and cells of the periodontium express TLRs [58]. Gingival keratinocytes express TLR2 [59], which interacts with either TLR1 or TLR6 [54]. TLR2 recognizes gram-positive and gram-negative bacterial PAMPs and activates intracellular signaling pathways, which could then induce antimicrobial peptides, like human beta defensins (hBDs) and inflammatory markers like cytokines or MMPs [54, 59, 60]. TLRs on the epithelial cell surface recognize F. nucleatum which activates pro-inflammatory signaling pathways [33]. The hBD2 and hBD3 are induced in oral epithelial cells via TLR2 and neutrophilic alkaline phosphatase (NALP) 2 [14, 53, 61]. Ji et al. demonstrated that TLR2 and NALP2 mediate the induction of hBDs, but not IL-8, and hBD2 and hBD3 are divergently regulated [53]. Fusobacterium-associated defensin inducer (FAD-I) is the principal F. nucleatum agent for hBD2 induction in human oral epithelial cells via TLR-1/2 and TLR-2/6 [31].
NOD-like receptors are normally composed of a central conserved NOD domain, a C-terminal LRR, and an N-terminal effector domain [62, 63]. Depending on the N-terminal effector domain, NLRs can be categorized into varying groups, such as NLRP, NLRC, and NLRX [64]. Every NLR is essential and not redundant in recognition of specific PAMPs or damage-associated molecular patterns (DAMPs). The responses that are induced can be classified as inflammasome-dependent or -independent [62]. NLRP1, NLRP3, and NLRC4 for example can build inflammasomes to activate caspase-1 after the sensing of PAMPs and DAMPs that results in the release of IL-1β [62, 65]. NLRP10 is the smallest human nucleotide-binding and leucine-rich repeat (NLR) protein which has anti- and pro-inflammatory functions [66]. It takes part in activating the extracellular signal-regulated kinases (ERK) signaling pathway in human epithelial cells infected with F. nucleatum and augments the pro-inflammatory cytokine IL-1α levels [66]. NOD proteins are cytosolic pattern recognition molecules that belong to the family of NLRs, recognizing PGN, a component of bacterial cell walls [67]. NOD1, NOD2, and NLRX1 induce pro-inflammatory responses through nuclear factor kappa B (NF-κB) or mitogen-activated protein kinase (MAPK) signaling during microbial infection [56, 64]. NOD1 is mostly involved in sensing components of Gram-negative bacteria, while NOD2 is able to recognize both Gram-negative and Gram-positive bacteria [68]. Both NOD1 and NOD2 might have played a role in recognizing periodontal pathogens, but the stimulatory activities of P. gingivalis are weaker than those of other periodontal pathogens [69]. In addition, the NLR family acts as an essential regulator of inflammatory and innate immune response, which can control IL-1, NF-κB, and host response to pathogens including distinct forms of cell death [70]. Depletion of NLRX1 can decrease F. nucleatum infection-activated NLRP3 in gingival epithelial cells (GECs). It is proposed that NLRX1 should enhance the innate immune response during infection by pathogens but behave as a break to prevent excessive inflammation under normal circumstances [62]. A study also found that F.nucleatum can stimulate NLRP3, activate upstream signal molecules of ATR-CHK1, and inhibit the activation of CHK1, promoting tumor growth and proliferation in squamous cell carcinomas (SCCs) [71].
Previous studies show that F. nucleatum can inhibit proliferation, apoptosis, reactive oxygen species (ROS), and inflammatory cytokine production partly of human gingival fibroblasts by activating the AKT/MAPK and NF-κB signaling pathways [72]. It is also reported that a large number of different genes are enriched in the PI3K/AKT signaling pathway after stimulation with F. nucleatum [72]. The activation of the PI3K/AKT pathway may induce cell growth [73, 74]. NF-κB, as the downstream molecule of the PI3K/AKT signaling pathway, acts synergetically in regulating cell proliferation, apoptosis, ROS generation, and the inflammatory respose [72], and it also mediates oral infections and periodontitis [75].
A recent study showed that F. nucleatum aggravated colitis by induction of the Th1-related cytokine IFN-γ over the AKT2 signaling pathway in vitro and in vivo. The group demonstrated that F. nucleatum could support the progression of colitis by proinflammatory M1 macrophage twisting. Therefore, F. nucleatum or AKT2 signaling may be therapeutic targets in order to inhibit the development of the disease [76].
Some signaling pathways that are related to F. nucleatum are shown in Figure 2.
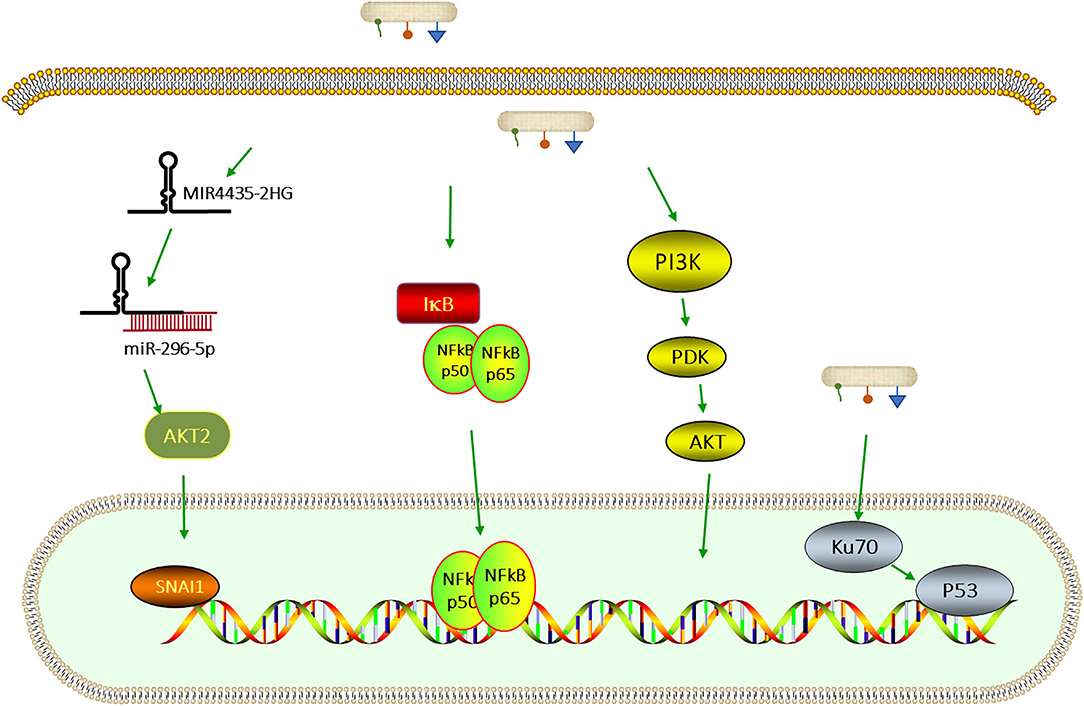
Figure 2. Signaling pathways that are related to F. nucleatum infection. F. nucleatum upregulates MIR4435-2HG, which binds miR-296-5p and weakens the inhibitory effect of miR-296-5p on SNAI1 via AKT2 [77]. F. nucleatum can also activate the PI3K/AKT—nuclear factor kappa B (NF-κB) signaling pathway which regulates cell proliferation, apoptosis, and the inflammatory response [72]. The infection with F. nucleatum promotes the capability of proliferation by leading to DNA damage through the Ku70/p53 pathway [78].
F. nucleatum and Defensins
The barrier function of epithelial results from the unique structural integrity and the production of antimicrobial peptides, such as hBDs and a cathelicidin, LL-37 [79]. Human beta defensins are a family of epithelial cell-derived antimicrobial peptides which are of importance in immune defense against challenging pathogens. The epithelia of many body sites express hBD1 constitutively but express hBD2 and hBD3 under conditions of infection or inflammation [53, 80]. The influences of oral bacteria on the expression of hBDs in epithelial cells and the signaling pathway have been studied extensively. hBD2 was found to have strong bactericidal effects against gram-negative periodontal bacteria [81], while hBD3 also demonstrated activity against Gram-positive as well as Gram-negative bacteria [82]. Moreover, hBD2 and hBD3 can furthermore attract different immune cells and link together the innate and adaptive immune responses [83]. However, gingival epithelium can also express hBD2 in the absence of inflammation, presumably due to the constant exposure to oral bacteria [53]. The promoter region of hBD2 contains numerous regulatory elements, including the binding sites for NF-κB, activator protein (AP)-1, AP-2, and nuclear factor for IL-6 expression (NF-IL-6), whereas the promoter of hBD3 contains no discernible NF-κB binding elements [53, 84]. F. nucleatum and F. nucleatum cell wall (FnCW) extracts induce expression of hBD2 and hBD3 in cultured primary human GECs in vitro [85, 86]. The induction of hBD2 in GECs in response to FnCW occurs mainly through MAPK signaling, not NF-κB [87]. Krisanaprakornkit et al. demonstrated that hBD2 and Interleukin-8 (IL)-8 could also be induced by LPS extracted from the cell wall of F. nucleatum [85]. Another study supported that F. nucleatum infection induced the expression of hBD2 and hBD3 in gingival cells [86].
LL-37, the only cathelicidin-derived antimicrobial peptide found in humans [88], is expressed by neutrophils and epithelial cells, and in the gingiva, it is localized in the junctional epithelium [89]. The local deficiency of LL-37 in the gingival crevicular fluid is suggested to be a supporting factor in the pathogenesis of severe cases of periodontitis [90]. Direct killing of microbes by antimicrobial peptides, including LL-37, is thought to serve as a crucial innate immune defense mechanism to prevent the growth of microbes in the gingival sulcus [91]. In addition to its antimicrobial effects, LL-37 suppresses inflammatory responses and modulates the apoptotic behavior of neutrophils [91]. Studies found that LL-37 is able to suppress the effect of P. gingivalis-induced proinflammatory responses of human gingival fibroblasts in a paracrine manner, suggesting that inflammatory responses to P. gingivalis in the gingival tissue are suppressed by LL-37 in vivo [91]. Moreover, LL-37 has the ability to suppress periodontopathogenic LPS-induced IL-8 production in both human periodontal ligament fibroblasts and gingival fibroblasts [92]. However, after stimulation with F. nucleatum, LL-37 is only weakly expressed in an organotypic dento-epithelial model, which is used to mimic the dento-gingival junction in vitro [93]. That is a coherent observation since LL-37 is mostly released by neutrophils and doesn't originate from epithelial cells [94].
F. nucleatum and Immune Responses
The gingival epithelium is the tissue that is primarily challenged by plaque-associated bacteria [86]. Mucosal epithelia not only are a passive protective barrier but also can initiate immune responses over secretion of a number of cytokines and chemokines [79, 86]. F. nucleatum can induce significant changes in the expression of genes related to immune defense responses [95]. Here, we reviewed the inflammatory and immune responses due to F. nucleatum.
Gingival epithelial cells gene expression was investigated after the stimulation with a commensal FnCW preparation and hBD2 peptide. The results revealed significant changes in the expression levels of genes associated with immune and defense responses. The 20 most highly up-regulated genes included CC chemokine-ligand 20 (CCL20), calcium-binding protein S100A7, skin-derived antileukoprotease (SKALP), IL 1 family member 9 (IL1F9), IL-8, chemokine (C-X-C motif) ligand (CXCL) 5, complement factor 3 (C3), IL-32, serum amyloid A (SAA) 1, small proline-rich protein (SPRR) 2C, and CXCL1. Fourteen out of 20 are cytokines, components of the innate immune system or inflammatory markers, antimicrobials, or protease inhibitors. Two genes that were also strongly up-regulated (small proline-rich proteins, SPRR2B, and SPRR2C) are associated with structural issues of the epithelial barrier. The most obvious down-regulated genes included cell cycle regulatory genes cell division cycle (CDC) 20, S-phase kinase-associated protein (SKP) 2, proliferating cell nuclear antigen (PCNA), polymerase epsilon (POLE) 2), and ubiquitin-proteasome-associated genes [ubiquitin associated protein 2 like (UBAP2L), proteasome 26S subunit, non-ATPase 11(PSMD11)] [96]. Genes up-regulated by FnCW include encoding antimicrobial peptides and proteins. Up-regulated genes of defense responses included chemokines IL-8, CXCL1, CXCL3, CXCL5, and CXCL10, which attract neutrophils, monocytes, macrophages, and lymphocytes. Furthermore, colony-stimulating factor (CSF) 2, and CSF3 that stimulate neutrophil development were up-regulated [96].
Neutrophils are involved in inflammatory processes and release proteases that induce tissue damage. Numerous protease inhibitors are strongly up-regulated as a reaction to FnCW [96]. The targets of these inhibitors are proteases released by neutrophils. This promotes the control of tissue damage and represents a protective mechanism if commensal bacteria are present [96, 97]. In addition to providing protection against neutrophil proteases, these protease inhibitors may also protect against bacterial proteases released by pathogens, such as P. gingivalis [96]. Genes that reduce NF-κB function, a major transcription factor participating in inflammatory responses, are up-regulated in GECs after stimulation with FnCW [96].
In conclusion, F. nucleatum not only induces the antimicrobial peptide hBD2 but also affects immune responses over the induction of chemokines as well as the apparent impairment of NF-κB function. F. nucleatum supports the maintenance of an intact mucosal surface by enhancing the transcription of a number of protease inhibitors that, when translated, work as active inhibitors that impede tissue damage by proteases secreted from neutrophils, which constantly migrate into the oral cavity by passing through the gingival crevice.
CCL20 is a 70-amino-acid chemokine that attracts immature dendritic cells and T cells via the chemokine receptor CCR6. It plays a role in the specific differentiation of lymphocytes, such as developing Th17 and Treg cells that migrate into inflamed periodontal tissues [98, 99]. Ghosh et al. detected that primary oral epithelial cells release CCL20 as a reaction to F. nucleatum [100]. They also demonstrated in vitro that the inducible defensins hBD-2, hBD-3, tumor necrosis factor-α (TNF-α), and IL-1 β could induce the release of CCL20 by human oral epithelial cells. ERK 1/2 and p38 are required in this process [100]. Interestingly, Yin et al. provided a new aspect of the bacteria-specific innate immune responses by epigenetic regulation [101]. They demonstrated that DNA methyltransferase (DNMT) and histone deacetylase expression were impaired in GECs treated with the oral pathogens P. gingivalis and F. nucleatum [101]. Pretreatment with DNMT inhibitor 5'-azacytidine enhanced hBD2 and CCL20 expression in F. nucleatum infected GECs [101].
In human epithelial cells, infection with F. nucleatum induces the upregulation of 12 kinases involved in cell migration, proliferation, and cell survival signaling, as assessed by the Kinetworks immunoblotting system [40, 102]. IL-1, IL-6, and IL-8 are pro-inflammatory cytokines induced in immune and non-immune cells, like gingival keratinocytes [54]. F. nucleatum effectively stimulates inflammatory cytokines, IL-6, IL-8, and TNF-α [3, 36, 103, 104]. F. nucleatum infection in gingival epithelial cells can also activate NF-κB, which as consequence translocates to the nucleus, and there stimulates the expression of pro-inflammatory genes, such as genes encoding pro-IL-1β [39, 65]. Inflammatory cytokines such as IL-1β, IL-6, and TNF- α mainly cause periodontal tissue damage. IL-1β may also be involved in bone resorption and attachment loss which are characteristic properties of periodontitis.
Lipopolysaccharides from F. nucleatum is responsible for activating the immune system at the cellular level in periodontitis [33]. It can drive the production of inflammatory cytokines, such as IL-1α, IL-1β, IL-6, IL-8, and MMPs through the activation of the translocation of the NF-κB gene into the nucleus, activating immunological response and leading to the loss of periodontal attachment and tissue damage [6, 33, 104–108].
A recent study revealed that periodontitis enhanced gingival levels of IL-6 and CXCL2 in an animal model. Orthodontic tooth movement enhanced microbial-induced periodontal destruction and gingival IL-6 gene expression. Enhanced IL-6 and CXCL2 levels have also been detected in the gingiva in human periodontitis. Moreover, mechanical stress enhanced the stimulatory impact of F. nucleatum on IL-6 production in vitro [109].
Fusobacterium nucleatum infection can induce some DAMPs that mediate the formation of inflammasomes such as the high-mobility group box-1 protein (HMGB1) and apoptosis-associated speck-like protein (ASP) with a similar time-course as caspase-1 activation [6, 65, 110]. These data are consistent with animal studies in BALB/c mice infected with F. nucleatum, which suggested that infection with F. nucleatum is followed by fast induction of inflammation, the release of DAMPs, and macrophage infiltration in gingival tissues. The results also suggested that osteoclasts possibly drive bone resorption in the early stages of the inflammatory process [111]. HMGB1, a DNA-binding nuclear protein, is released actively after cytokine stimulation and passively during cell death; it is involved in several inflammatory disorders, cell adhesion, and cell migration [112]. HMGB1 can link with other molecules, including cytokines and TLR ligands. It activates cells by the differential engagement of numerous surface receptors such as TLR2, TLR4, and receptor of advanced glycation end-product (RAGE) [112]. RAGE is a receptor that binds structurally diverse molecules, and its signaling pathway includes the activation of MAPKs, NF-κB, PI3K/AKT, JAK/STAT [6]. The interaction between HMGB1 and RAGE may contribute to oral inflammation and oral cancer [6].
A disintegrin and metalloproteinase 8 (ADAM8), which is localized within the gingival epithelium, exhibits enhanced expression in inflamed tissues affected with chronic periodontitis [113]. ADAM8 mRNA expression in GECs is significantly induced by stimulation with F. nucleatum [113]. These findings suggest a possible role of ADAM8 in the innate immunity of periodontal tissues [113]. Moreover, ADAM8 is found significantly elevated in the gingival crevicular fluid of patients with chronic periodontitis [114] and synovia of patients with rheumatoid arthritis [115]. This is consistent with previous experiments which demonstrated that overexpression of ADAM8 may increase in vitro osteoclast development and function and cause bone resorption in mouse models [116, 117], implying a possible role of ADAM8 in the promotion of bone destruction [113].
The Role of F. nucleatum in Oral Cancer
It is not a new idea that the microbiota plays a role in cancer. Bacteria, parasites, and viruses are all linked to the initiation and progression of cancer [15]. Chronic infections and persistent inflammation are linked to an augmented risk of cancer [1]. Persisting bacterial components may lead to up-regulation of immune-suppressing receptors, which can facilitate the ability of cancer cells to escape from anti-tumor responses of the host [1]. During the past decade, it was shown that oral bacteria might promote different oral diseases. The role of F. nucleatum as an inflammation-causing and cancer-inducing agent in oral epithelial cells is still emerging.
In a murine model of periodontitis-associated oral tumorigenesis that Binder Gallimidi et al. used, it was demonstrated that chronic bacterial infection promotes oral squamous carcinoma cells (OSCC) and intensified signaling over the IL-6-STAT3 axis [39]. P. gingivalis and F. nucleatum can induce tumorigenesis by directly interacting with oral epithelial cells via TLRs [39]. In contrast to P. gingivalis, which has been demonstrated to exhibit specific virulence factors that are probably involved in different stages of carcinogenesis, the presumed carcinogenic potential of F. nucleatum has been mostly explained over its pro-inflammatory effect by inducing different cytokines, including TNF-α, IL-6, IL-8, IL-10, IL-12, and production of ROS within the colon lining epithelial cells. This process ultimately can cause dysplasia and the development of cancer [3, 118]. F.nucleatum was also highly abundant in salivary samples and tumor sites of patients suffering from oral/head and neck squamous cell carcinoma [119, 120]. Tumor lesions showed a 6% higher abundance of Fusobacterium (95% CI, 3–9) than in non-tumor lesions and a 2.93 times higher chance of Fusobacterium being present in lesions [121]. These findings suggest that Fusobacterium infection might promote oral/head and neck cancer [121]. Recent studies demonstrated that F. nucleatum infection might impact cell migration, proliferation, invasion, and apoptosis, in/of gingival epithelial cells or OSCC [72, 78, 122].
In 2020, a cellular model of human immortalized oral epithelial cells (HIOECs) with F. nucleatum infection was established. The study group reported that F. nucleatum facilitated the functional loss of E-cadherin, cell migration, and the up-regulation of Snail family transcriptional repressor 1 (SNAI1) in non-cancerous as well as cancerous oral epithelial cells, which is regarded as an indicator for epithelial-mesenchymal transition (EMT) [77]. This model was used to perform high-throughput sequencing of cells infected by F. nucleatum. The function of the database of essential genes (DEGs) and cis targeted genes of differentially expressed long non-coding RNAs (lncRNAs) were shown to be significantly enriched in biological processes of DNA-templated transcription. In contrast, the trans-targeted genes of lncRNAs showed a main association with cell-cell adhesion and cell division. The tumor-associated genes were specifically analyzed and the top 10 hub genes related to tumor progression were identified. Some of the hub genes were also demonstrated to be aberrantly expressed in clinical samples of OSCC [123]. F. nucleatum infection was also found to promote the proliferative ability of oral carcinoma cells by causing DNA damage through the Ku70/p53 pathway [78].
Conclusion
Fusobacterium nucleatum, as one of the resident members of the oral microflora, plays a significant role in the pathogenesis and progression of periodontitis. Long-term infection with F. nucleatum may lead to the development of cancer. Several studies demonstrated that F. nucleatum could be a causative constituent of oral squamous cell carcinoma. There are some underlying molecular mechanisms about F. nucleatum and oral epithelial cells, but we tried to review almost all known mechanisms, which are summarized in Figure 3. F. nucleatum adheres to and invades oral epithelial cells by binding host receptors, modulating host signaling pathway and cytokine network. This induced an inflammatory environment in the host and promoted the secretion of inflammatory factors and serine proteases, disrupting the immune-inflammatory balance and damaging periodontal tissue. Moreover, chronic infections are related to the increased risk of cancer. The interaction between F. nucleatum and oral epithelial cells creates a microenvironment that promotes tumor growth. Although growing evidence has contributed to a better understanding of the F. nucleatum-GEC interactions and how this dialog modulates host responses leading to inflammatory diseases, future studies are needed to identify new potential molecular targets for preventing and treating F. nucleatum-induced diseases.
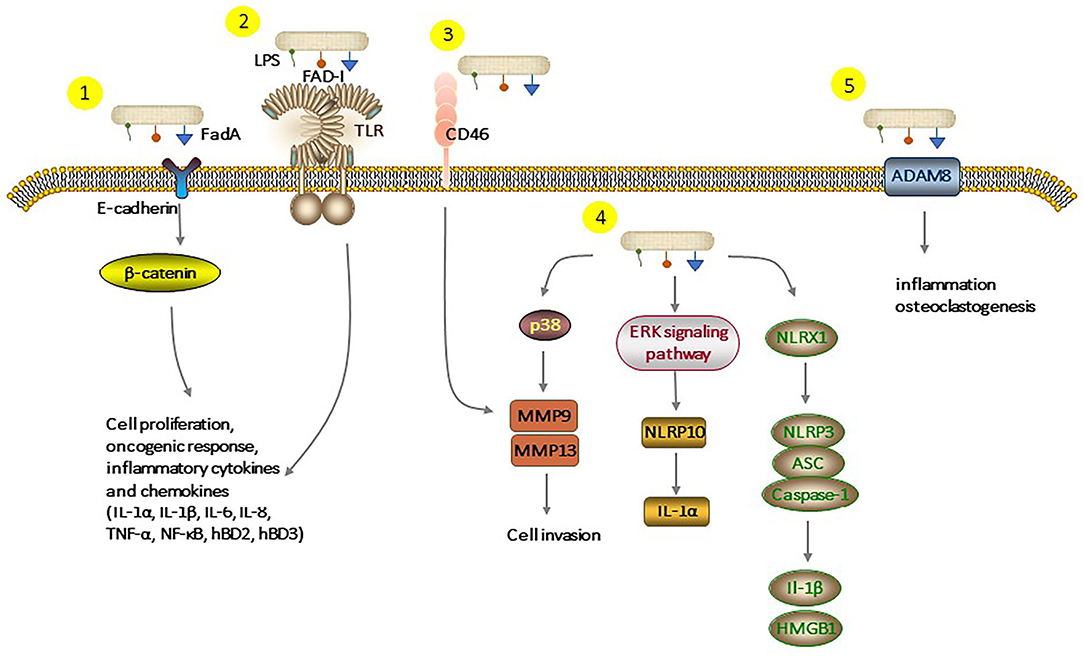
Figure 3. Different pathways of interaction of F. nucleatum with epithelial cells. (1), FadA binding to E-cadherin activates β-catenin signaling, leads to cell proliferation, oncogenic, and inflammatory responses. (2), FAD-I can induce hBD2 expression via both TLR-1/2 and TLR-2/6. LPS and cell extracts of F. nucleatum can also increase production of inflammatory cytokines and chemokines. (3), F. nucleatum binds to CD46 which results in the overexpression of MMP-9. (4), F. nucleatum triggers the expression of MMP-9 and MMP-13 over the p38 signaling pathway, which causes invasion into the epithelial cell. Activation of the extraregular regulated protein kinases (ERK) signaling pathway augments the pro-inflammatory cytokine IL-1α levels. F. nucleatum infection-triggered inflammatory response activates the NLRP3 inflammasome, which is enhanced by NLRX1. (5), Stimulation by F. nucleatum leads to upregulation of ADAM8 expression which is involved in inflammation and essential for osteoclastogenesis. LPS, Lipopolysaccharides; Fad-I, Fusobacterium-associated defensing inducer; FadA, Fusobacterial adhesion; NLRP, Nucleotide-binding oligomerization domain-like repeat protein; NLRX, Nucleotide-binding domain and leucine-rich-repeat-containing family member X; ADAM8, A disintegrin and metalloproteinase 8; ASC, Apoptosis-associated speck-like protein containing a carboxy-terminal CARD; MMPs, Matrix metalloproteinase.
Author Contributions
SG wrote the manuscript together with YZ. SR and JM helped to prepare the manuscript. All authors contributed to the article and approved the submitted version.
Conflict of Interest
The authors declare that the research was conducted in the absence of any commercial or financial relationships that could be construed as a potential conflict of interest.
Publisher's Note
All claims expressed in this article are solely those of the authors and do not necessarily represent those of their affiliated organizations, or those of the publisher, the editors and the reviewers. Any product that may be evaluated in this article, or claim that may be made by its manufacturer, is not guaranteed or endorsed by the publisher.
References
1. Groeger S, Meyle J. Oral mucosal epithelial cells. Front Immunol. (2019) 10:208. doi: 10.3389/fimmu.2019.00208
2. Groeger SE, Meyle J. Epithelial barrier and oral bacterial infection. Periodontol. (2015) 69:46–67. doi: 10.1111/prd.12094
3. Han YW. Fusobacterium nucleatum: a commensal-turned pathogen. Curr Opin Microbiol. (2015) 23:141–7. doi: 10.1016/j.mib.2014.11.013
4. Kostic AD, Xavier RJ, Gevers D. The microbiome in inflammatory bowel disease: current status and the future ahead. Gastroenterology. (2014) 146:1489–99. doi: 10.1053/j.gastro.2014.02.009
5. Han XY, Weinberg JS, Prabhu SS, Hassenbusch SJ, Fuller GN, Tarrand JJ, et al. Fusobacterial brain abscess: a review of five cases and an analysis of possible pathogenesis. J Neurosurg. (2003) 99:693–700. doi: 10.3171/jns.2003.99.4.0693
6. Gholizadeh P, Eslami H, Kafil HS. Carcinogenesis mechanisms of Fusobacterium nucleatum. Biomed Pharmacother. (2017) 89:918–25. doi: 10.1016/j.biopha.2017.02.102
7. Wang X, Buhimschi CS, Temoin S, Bhandari V, Han YW, Buhimschi IA. Comparative microbial analysis of paired amniotic fluid and cord blood from pregnancies complicated by preterm birth and early-onset neonatal sepsis. PLoS ONE. (2013) 8:e56131. doi: 10.1371/journal.pone.0056131
8. Brook I. Fusobacterial infections in children. Curr Infect Dis Rep. (2013) 15:288–94. doi: 10.1007/s11908-013-0340-6
9. Didilescu AC, Rusu D, Anghel A, Nica L, Iliescu A, Greabu M, et al. Investigation of six selected bacterial species in endo-periodontal lesions. Int Endod J. (2012) 45:282–93. doi: 10.1111/j.1365-2591.2011.01974.x
10. Yang NY, Zhang Q, Li JL, Yang SH, Shi Q. Progression of periodontal inflammation in adolescents is associated with increased number of Porphyromonas gingivalis, Prevotella intermedia, Tannerella forsythensis, and Fusobacterium nucleatum. Int J Paediatr Dent. (2014) 24:226–33. doi: 10.1111/ipd.12065
11. Zhou X, Liu X, Li J, Aprecio RM, Zhang W, Li Y. Real-time PCR quantification of six periodontal pathogens in saliva samples from healthy young adults. Clin Oral Investig. (2015) 19:937–46. doi: 10.1007/s00784-014-1316-0
12. Chaushu S, Wilensky A, Gur C, Shapira L, Elboim M, Halftek G, et al. Direct recognition of Fusobacterium nucleatum by the NK cell natural cytotoxicity receptor NKp46 aggravates periodontal disease. PLoS Pathog. (2012) 8:e1002601. doi: 10.1371/journal.ppat.1002601
13. Polak D, Wilensky A, Shapira L, Halabi A, Goldstein D, Weiss EI, et al. Mouse model of experimental periodontitis induced by Porphyromonas gingivalis/Fusobacterium nucleatum infection: bone loss and host response. J Clin Periodontol. (2009) 36:406–10. doi: 10.1111/j.1600-051X.2009.01393.x
14. Ji S, Shin JE, Kim YC, Choi Y. Intracellular degradation of Fusobacterium nucleatum in human gingival epithelial cells. Mol Cells. (2010) 30:519–26. doi: 10.1007/s10059-010-0142-8
15. Brennan CA, Garrett WS. Fusobacterium nucleatum - symbiont, opportunist and oncobacterium. Nat Rev Microbiol. (2019) 17:156–66. doi: 10.1038/s41579-018-0129-6
16. Kolenbrander PE, Palmer RJ Jr, Periasamy S, Jakubovics NS. Oral multispecies biofilm development and the key role of cell-cell distance. Nat Rev Microbiol. (2010) 8:471–80. doi: 10.1038/nrmicro2381
17. Kolenbrander PE, London J. Adhere today, here tomorrow: oral bacterial adherence. J Bacteriol. (1993) 175:3247–52. doi: 10.1128/jb.175.11.3247-3252.1993
18. Zijnge V, van Leeuwen MB, Degener JE, Abbas F, Thurnheer T, Gmur R, et al. Oral biofilm architecture on natural teeth. PLoS ONE. (2010) 5:e9321. doi: 10.1371/journal.pone.0009321
19. Rizzato C, Torres J, Kasamatsu E, Camorlinga-Ponce M, Bravo MM, Canzian F, et al. Potential role of biofilm formation in the development of digestive tract cancer with special reference to Helicobacter pylori infection. Front Microbiol. (2019) 10:846. doi: 10.3389/fmicb.2019.00846
20. Edwards AM, Grossman TJ, Rudney JD. Fusobacterium nucleatum transports noninvasive Streptococcus cristatus into human epithelial cells. Infect Immun. (2006) 74:654–62. doi: 10.1128/IAI.01559-06
21. Kaplan CW, Ma X, Paranjpe A, Jewett A, Lux R, Kinder-Haake S, et al. Fusobacterium nucleatum outer membrane proteins Fap2 and RadD induce cell death in human lymphocytes. Infect Immun. (2010) 78:4773–8. doi: 10.1128/IAI.00567-10
22. Coppenhagen-Glazer S, Sol A, Abed J, Naor R, Zhang X, Han YW, et al. Fap2 of Fusobacterium nucleatum is a galactose-inhibitable adhesin involved in coaggregation, cell adhesion, and preterm birth. Infect Immun. (2015) 83:1104–13. doi: 10.1128/IAI.02838-14
23. Kaplan CW, Lux R, Haake SK, Shi W. The Fusobacterium nucleatum outer membrane protein RadD is an arginine-inhibitable adhesin required for inter-species adherence and the structured architecture of multispecies biofilm. Mol Microbiol. (2009) 71:35–47. doi: 10.1111/j.1365-2958.2008.06503.x
24. Koike R, Cueno ME, Nodomi K, Tamura M, Kamio N, Tanaka H, et al. Heat-killed Fusobacterium nucleatum triggers varying heme-related inflammatory and stress responses depending on primary human respiratory epithelial cell type. Molecules. (2020) 25:173839. doi: 10.3390/molecules25173839
25. Shokeen B, Park J, Duong E, Rambhia S, Paul M, Weinberg A, et al. Role of FAD-I in fusobacterial interspecies interaction and biofilm formation. Microorganisms. (2020) 8:10070. doi: 10.3390/microorganisms8010070
26. Guo L, Shokeen B, He X, Shi W, Lux R. Streptococcus mutans SpaP binds to RadD of Fusobacterium nucleatum ssp. Polymorphum. Mol Oral Microbiol. (2017) 32:355–64. doi: 10.1111/omi.12177
27. Wu T, Cen L, Kaplan C, Zhou X, Lux R, Shi W, et al. Cellular components mediating coadherence of Candida albicans and Fusobacterium nucleatum. J Dent Res. (2015) 94:1432–8. doi: 10.1177/0022034515593706
28. Kaplan A, Kaplan CW, He X, McHardy I, Shi W, Lux R. Characterization of aid1, a novel gene involved in Fusobacterium nucleatum interspecies interactions. Microb Ecol. (2014) 68:379–87. doi: 10.1007/s00248-014-0400-y
29. Lima BP, Shi W, Lux R. Identification and characterization of a novel Fusobacterium nucleatum adhesin involved in physical interaction and biofilm formation with Streptococcus gordonii. Microbiologyopen. (2017) 6:444. doi: 10.1002/mbo3.444
30. Han Y. Oral Microbial Communities: Genomic Inquiry and Interspecies Communication. Chichester: Whiley publishing (2011).
31. Bhattacharyya S, Ghosh SK, Shokeen B, Eapan B, Lux R, Kiselar J, et al. FAD-I, a Fusobacterium nucleatum cell wall-associated diacylated lipoprotein that mediates human beta defensin 2 induction through toll-like receptor-1/2 (TLR-1/2) and TLR-2/6. Infect Immun. (2016) 84:1446–56. doi: 10.1128/IAI.01311-15
32. Rubinstein MR, Wang X, Liu W, Hao Y, Cai G, Han YW. Fusobacterium nucleatum promotes colorectal carcinogenesis by modulating E-cadherin/beta-catenin signaling via its FadA adhesin. Cell Host Microbe. (2013) 14:195–206. doi: 10.1016/j.chom.2013.07.012
33. Chattopadhyay I, Verma M, Panda M. Role of oral microbiome signatures in diagnosis and prognosis of oral cancer. Technol Cancer Res Treat. (2019) 18:1533033819867354. doi: 10.1177/1533033819867354
34. Ma CT, Luo HS, Gao F, Tang QC, Chen W. Fusobacterium nucleatum promotes the progression of colorectal cancer by interacting with E-cadherin. Oncol Lett. (2018) 16:2606–12. doi: 10.3892/ol.2018.8947
35. Silva BS, Castro CA, Von Zeidler SL, Sousa SC, Batista AC, Yamamoto-Silva FP. Altered beta-catenin expression in oral mucosal dysplasia: a comparative study. J Appl Oral Sci. (2015) 23:472–8. doi: 10.1590/1678-775720150150
36. Gholizadeh P, Eslami H, Yousefi M, Asgharzadeh M, Aghazadeh M, Kafil HS. Role of oral microbiome on oral cancers, a review. Biomed Pharmacother. (2016) 84:552–8. doi: 10.1016/j.biopha.2016.09.082
37. Montreekachon P, Chotjumlong P, Reutrakul V, Krisanaprakornkit S. Involvement of cytosolic phospholipase A2alpha in MMP-9 up-regulation. J Dent Res. (2009) 88:1031–6. doi: 10.1177/0022034509345967
38. Gursoy UK, Könönen E, Uitto VJ. Stimulation of epithelial cell matrix metalloproteinase (MMP-2,−9,−13) and interleukin-8 secretion by fusobacteria. Oral Microbiol Immunol. (2008) 23:432–4. doi: 10.1111/j.1399-302X.2008.00453.x
39. Binder Gallimidi A, Fischman S, Revach B, Bulvik R, Maliutina A, Rubinstein AM, et al. Periodontal pathogens Porphyromonas gingivalis and Fusobacterium nucleatum promote tumor progression in an oral-specific chemical carcinogenesis model. Oncotarget. (2015) 6:22613–23. doi: 10.18632/oncotarget.4209
40. Uitto VJ, Baillie D, Wu Q, Gendron R, Grenier D, Putnins EE, et al. Fusobacterium nucleatum increases collagenase 3 production and migration of epithelial cells. Infect Immun. (2005) 73:1171–9. doi: 10.1128/IAI.73.2.1171-1179.2005
41. Liu P, Liu Y, Wang J, Guo Y, Zhang Y, Xiao S. Detection of Fusobacterium nucleatum and fadA adhesin gene in patients with orthodontic gingivitis and non-orthodontic periodontal inflammation. PLoS ONE. (2014) 9:e85280. doi: 10.1371/journal.pone.0085280
42. Chew J, Zilm PS, Fuss JM, Gully NJ. A proteomic investigation of Fusobacterium nucleatum alkaline-induced biofilms. BMC Microbiol. (2012) 12:189. doi: 10.1186/1471-2180-12-189
43. Gu X, Song LJ, Li LX, Liu T, Zhang MM, Li Z, et al. Fusobacterium nucleatum causes microbial dysbiosis and exacerbates visceral hypersensitivity in a colonization-independent manner. Front Microbiol. (2020) 11:1281. doi: 10.3389/fmicb.2020.01281
44. Toussi DN, Liu X, Massari P. The FomA porin from Fusobacterium nucleatum is a Toll-like receptor 2 agonist with immune adjuvant activity. Clin Vaccine Immunol. (2012) 19:1093–101. doi: 10.1128/CVI.00236-12
45. Han YW, Shi W, Huang GT, Kinder Haake S, Park NH, Kuramitsu H, et al. Interactions between periodontal bacteria and human oral epithelial cells: Fusobacterium nucleatum adheres to and invades epithelial cells. Infect Immun. (2000) 68:3140–6. doi: 10.1128/IAI.68.6.3140-3146.2000
46. Zhang Z, Liu S, Zhang S, Li Y, Shi X, Liu D, et al. Porphyromonas gingivalis outer membrane vesicles inhibit the invasion of Fusobacterium nucleatum into oral epithelial cells by downregulating FadA and FomA. J Periodontol. (2021). doi: 10.1002/JPER.21-0144
47. Hussan H, Clinton SK, Roberts K, Bailey MT. Fusobacterium's link to colorectal neoplasia sequenced: a systematic review and future insights. World J Gastroenterol. (2017) 23:8626–50. doi: 10.3748/wjg.v23.i48.8626
48. Zhang S, Cai S, Ma Y. Association between Fusobacterium nucleatum and colorectal cancer: progress and future directions. J Cancer. (2018) 9:1652–9. doi: 10.7150/jca.24048
49. Gur C, Ibrahim Y, Isaacson B, Yamin R, Abed J, Gamliel M, et al. Binding of the Fap2 protein of Fusobacterium nucleatum to human inhibitory receptor TIGIT protects tumors from immune cell attack. Immunity. (2015) 42:344–55. doi: 10.1016/j.immuni.2015.01.010
50. Ganesan K, Guo S, Fayyaz S, Zhang G, Xu B. Targeting programmed Fusobacterium nucleatum Fap2 for colorectal cancer therapy. Cancers. (2019) 11:1592. doi: 10.3390/cancers11101592
51. Li Y, Guo H, Wang X, Lu Y, Yang C, Yang P. Coinfection with Fusobacterium nucleatum can enhance the attachment and invasion of Porphyromonas gingivalis or Aggregatibacter actinomycetemcomitans to human gingival epithelial cells. Arch Oral Biol. (2015) 60:1387–93. doi: 10.1016/j.archoralbio.2015.06.017
52. Ben Lagha A, Haas B, Grenier D. Tea polyphenols inhibit the growth and virulence properties of Fusobacterium nucleatum. Sci Rep. (2017) 7:44815. doi: 10.1038/srep44815
53. Ji S, Shin JE, Kim YS, Oh JE, Min BM, Choi Y. Toll-like receptor 2 and NALP2 mediate induction of human beta-defensins by Fusobacterium nucleatum in gingival epithelial cells. Infect Immun. (2009) 77:1044–52. doi: 10.1128/IAI.00449-08
54. Peyret-Lacombe A, Brunel G, Watts M, Charveron M, Duplan H. TLR2 sensing of F. nucleatum and S sanguinis distinctly triggered gingival innate response. Cytokine. (2009) 46:201–10. doi: 10.1016/j.cyto.2009.01.006
55. Kumar H, Kawai T, Akira S. Pathogen recognition by the innate immune system. Int Rev Immunol. (2011) 30:16–34. doi: 10.3109/08830185.2010.529976
56. Brubaker SW, Bonham KS, Zanoni I, Kagan JC. Innate immune pattern recognition: a cell biological perspective. Annu Rev Immunol. (2015) 33:257–90. doi: 10.1146/annurev-immunol-032414-112240
57. Kanzler H, Barrat FJ, Hessel EM, Coffman RL. Therapeutic targeting of innate immunity with Toll-like receptor agonists and antagonists. Nat Med. (2007) 13:552–9. doi: 10.1038/nm1589
58. Mahanonda R, Pichyangkul S. Toll-like receptors and their role in periodontal health and disease. Periodontology. (2007) 43:41–55. doi: 10.1111/j.1600-0757.2006.00179.x
59. Asai Y, Jinno T, Ogawa T. Oral treponemes and their outer membrane extracts activate human gingival epithelial cells through toll-like receptor 2. Infect Immun. (2003) 71:717–25. doi: 10.1128/IAI.71.2.717-725.2003
60. Sugawara Y, Uehara A, Fujimoto Y, Kusumoto S, Fukase K, Shibata K, et al. Toll-like receptors, NOD1, and NOD2 in oral epithelial cells. J Dent Res. (2006) 85:524–9. doi: 10.1177/154405910608500609
61. Gupta S, Ghosh SK, Scott ME, Bainbridge B, Jiang B, Lamont RJ, et al. Fusobacterium nucleatum-associated beta-defensin inducer (FAD-I): identification, isolation, and functional evaluation. J Biol Chem. (2010) 285:36523–31. doi: 10.1074/jbc.M110.133140
62. Hung SC, Huang PR, Almeida-da-Silva CLC, Atanasova KR, Yilmaz O, Ojcius DM. NLRX1 modulates differentially NLRP3 inflammasome activation and NF-kappaB signaling during Fusobacterium nucleatum infection. Microbes Infect. (2018) 20:615–25. doi: 10.1016/j.micinf.2017.09.014
63. Proell M, Riedl SJ, Fritz JH, Rojas AM, Schwarzenbacher R. The Nod-like receptor (NLR) family: a tale of similarities and differences. PLoS ONE. (2008) 3:e2119. doi: 10.1371/journal.pone.0002119
64. Barbe F, Douglas T, Saleh M. Advances in Nod-like receptors (NLR) biology. Cytokine Growth Factor Rev. (2014) 25:681–97. doi: 10.1016/j.cytogfr.2014.07.001
65. Bui FQ, Johnson L, Roberts J, Hung SC, Lee J, Atanasova KR, et al. Fusobacterium nucleatum infection of gingival epithelial cells leads to NLRP3 inflammasome-dependent secretion of IL-1beta and the danger signals ASC and HMGB1. Cell Microbiol. (2016) 18:970–81. doi: 10.1111/cmi.12560
66. Lee SJ, Choi BK. Involvement of NLRP10 in IL-1alpha induction of oral epithelial cells by periodontal pathogens. Innate Immun. (2017) 23:569–77. doi: 10.1177/1753425917722610
67. Chung WO, An JY, Yin L, Hacker BM, Rohani MG, Dommisch H, et al. Interplay of protease-activated receptors and NOD pattern recognition receptors in epithelial innate immune responses to bacteria. Immunol Lett. (2010) 131:113–9. doi: 10.1016/j.imlet.2010.02.006
68. Inohara C, McDonald C, Nunez G. NOD-LRR proteins: role in host-microbial interactions and inflammatory disease. Ann Rev Biochem. (2005) 74:355–83. doi: 10.1146/annurev.biochem.74.082803.133347
69. Okugawa T, Kaneko T, Yoshimura A, Silverman N, Hara Y. NOD1 and NOD2 mediate sensing of periodontal pathogens. J Dent Res. (2010) 89:186–91. doi: 10.1177/0022034509354843
70. Ye Z, Ting JP. NLR the nucleotide-binding domain leucine-rich repeat containing gene family. Curr Opin Immunol. (2008) 20:3–9. doi: 10.1016/j.coi.2008.01.003
71. Yao Y, Shen X, Zhou M, Tang B. Periodontal pathogens promote oral squamous cell carcinoma by regulating ATR and NLRP3 inflammasome. Front Oncol. (2021) 11:722797. doi: 10.3389/fonc.2021.722797
72. Kang W, Jia Z, Tang D, Zhang Z, Gao H, He K, et al. Fusobacterium nucleatum facilitates apoptosis, ROS generation, and inflammatory cytokine production by activating AKT/MAPK and NF-kappaB signaling pathways in human gingival fibroblasts. Oxid Med Cell Longev. (2019) 2019:1681972. doi: 10.1155/2019/1681972
73. Fulda S. The PI3K/Akt/mTOR pathway as therapeutic target in neuroblastoma. Curr Cancer Drug Targets. (2009) 9:729–37. doi: 10.2174/156800909789271521
74. Yang J-h, Yu K, Si X-k, Li S, Cao Y-j, Li W, et al. Liensinine inhibited gastric cancer cell growth through ROS generation and the PI3K/AKT pathway. J Cancer. (2019) 10:6431–8. doi: 10.7150/jca.32691
75. Abu-Amer Y. NF-kappaB signaling and bone resorption. Osteoporos Int. (2013) 24:2377–86. doi: 10.1007/s00198-013-2313-x
76. Liu L, Liang L, Liang H, Wang M, Lu B, Xue M, et al. Fusobacterium nucleatum aggravates the progression of colitis by regulating M1 macrophage polarization via AKT2 pathway. Front Immunol. (2019) 10:1324. doi: 10.3389/fimmu.2019.01324
77. Zhang S, Li C, Liu J, Geng F, Shi X, Li Q, et al. Fusobacterium nucleatum promotes epithelial-mesenchymal transiton through regulation of the lncRNA MIR4435-2HG/miR-296-5p/Akt2/SNAI1 signaling pathway. FEBS J. (2020) 287:4032–47. doi: 10.1111/febs.15233
78. Geng F, Zhang Y, Lu Z, Zhang S, Pan Y. Fusobacterium nucleatum caused DNA damage and promoted cell proliferation by the Ku70/p53 pathway in oral cancer cells. DNA Cell Biol. (2020) 39:144–51. doi: 10.1089/dna.2019.5064
79. Acheson DW, Luccioli S. Microbial-gut interactions in health and disease. Mucosal immune responses. Best Pract Res Clin Gastroenterol. (2004) 18:387–404. doi: 10.1016/j.bpg.2003.11.002
80. Putsep K, Carlsson G, Boman HG, Andersson M. Deficiency of antibacterial peptides in patients with morbus Kostmann: an observation study. Lancet. (2002) 360:1144–9. doi: 10.1016/S0140-6736(02)11201-3
81. Scott MG, Hancock RE. Cationic antimicrobial peptides and their multifunctional role in the immune system. Crit Rev Immunol. (2000) 20:407–31. doi: 10.1615/CritRevImmunol.v20.i5.40
82. Harder J, Bartels J, Christophers E, Schroder JM. Isolation and characterization of human beta -defensin-3, a novel human inducible peptide antibiotic. J Biol Chem. (2001) 276:5707–13. doi: 10.1074/jbc.M008557200
83. Marshall RI. Gingival defensins: linking the innate and adaptive immune responses to dental plaque. Periodontology. (2004) 35:14–20. doi: 10.1111/j.0906-6713.2004.003568.x
84. Jia HP, Schutte BC, Schudy A, Linzmeier R, Guthmiller JM, Johnson GK, et al. Discovery of new human beta-defensins using a genomics-based approach. Gene. (2001) 263:211–8. doi: 10.1016/S0378-1119(00)00569-2
85. Krisanaprakornkit S, Kimball JR, Weinberg A, Darveau RP, Bainbridge BW, Dale BA. Inducible expression of human beta-defensin 2 by Fusobacterium nucleatum in oral epithelial cells: multiple signaling pathways and role of commensal bacteria in innate immunity and the epithelial barrier. Infect Immun. (2000) 68:2907–15. doi: 10.1128/IAI.68.5.2907-2915.2000
86. Ji S, Kim Y, Min BM, Han SH, Choi Y. Innate immune responses of gingival epithelial cells to nonperiodontopathic and periodontopathic bacteria. J Periodontal Res. (2007) 42:503–10. doi: 10.1111/j.1600-0765.2007.00974.x
87. Krisanaprakornkit S, Kimball JR, Dale BA. Regulation of human beta-defensin-2 in gingival epithelial cells: the involvement of mitogen-activated protein kinase pathways, but not the NF-kappaB transcription factor family. J Immunol. (2002) 168:316–24. doi: 10.4049/jimmunol.168.1.316
88. Durr UH, Sudheendra US, Ramamoorthy A. LL-37, the only human member of the cathelicidin family of antimicrobial peptides. Biochim Biophys Acta. (2006) 1758:1408–25. doi: 10.1016/j.bbamem.2006.03.030
89. Nizet V, Ohtake T, Lauth X, Trowbridge J, Rudisill J, Dorschner RA, et al. Innate antimicrobial peptide protects the skin from invasive bacterial infection. Nature. (2001) 414:454–7. doi: 10.1038/35106587
90. Puklo M, Guentsch A, Hiemstra PS, Eick S, Potempa J. Analysis of neutrophil-derived antimicrobial peptides in gingival crevicular fluid suggests importance of cathelicidin LL-37 in the innate immune response against periodontogenic bacteria. Oral Microbiol Immunol. (2008) 23:328–35. doi: 10.1111/j.1399-302X.2008.00433.x
91. Inomata M, Into T, Murakami Y. Suppressive effect of the antimicrobial peptide LL-37 on expression of IL-6, IL-8 and CXCL10 induced by Porphyromonas gingivalis cells and extracts in human gingival fibroblasts. Eur J Oral Sci. (2010) 118:574–81. doi: 10.1111/j.1600-0722.2010.00775.x
92. Suphasiriroj W, Mikami M, Shimomura H, Sato S. Specificity of antimicrobial peptide LL-37 to neutralize periodontopathogenic lipopolysaccharide activity in human oral fibroblasts. J Periodontol. (2013) 84:256–64. doi: 10.1902/jop.2012.110652
93. Gursoy UK, Pollanen M, Kononen E, Uitto VJ. A novel organotypic dento-epithelial culture model: effect of Fusobacterium nucleatum biofilm on B-defensin-2,−3, and LL-37 expression. J Periodontol. (2012) 83:242–7. doi: 10.1902/jop.2011.110177
94. Turkoglu O, Kandiloglu G, Berdeli A, Emingil G, Atilla G. Antimicrobial peptide hCAP-18/LL-37 protein and mRNA expressions in different periodontal diseases. Oral Dis. (2011) 17:60–7. doi: 10.1111/j.1601-0825.2010.01704.x
95. Signat B, Roques C, Poulet P, Duffaut D. Fusobacterium nucleatum in periodontal health and disease. Curr Issues Mol Biol. (2011) 13:25–36. doi: 10.21775/cimb.013.025
96. Yin L, Dale BA. Activation of protective responses in oral epithelial cells by Fusobacterium nucleatum and human beta-defensin-2. J Med Microbiol. (2007) 56:976–87. doi: 10.1099/jmm.0.47198-0
97. Magert HJ, Drogemuller K, Raghunath M. Serine proteinase inhibitors in the skin: role in homeostasis and disease. Curr Protein Pept Sci. (2005) 6:241–54. doi: 10.2174/1389203054065374
98. Schutyser E, Struyf S, Van Damme J. The CC chemokine CCL20 and its receptor CCR6. Cytokine Growth Factor Rev. (2003) 14:409–26. doi: 10.1016/S1359-6101(03)00049-2
99. Peyyala R, Emecen-Huja P, Ebersole JL. Environmental lead effects on gene expression in oral epithelial cells. J Periodontal Res. (2018) 53:961–71. doi: 10.1111/jre.12594
100. Ghosh SK, Gupta S, Jiang B, Weinberg A. Fusobacterium nucleatum and human beta-defensins modulate the release of antimicrobial chemokine CCL20/macrophage inflammatory protein 3alpha. Infect Immun. (2011) 79:4578–87. doi: 10.1128/IAI.05586-11
101. Yin L, Chung WO. Epigenetic regulation of human beta-defensin 2 and CC chemokine ligand 20 expression in gingival epithelial cells in response to oral bacteria. Mucosal Immunol. (2011) 4:409–19. doi: 10.1038/mi.2010.83
102. Perera M, Al-hebshi NN, Speicher DJ, Perera I, Johnson NW. Emerging role of bacteria in oral carcinogenesis: a review with special reference to perio-pathogenic bacteria. J Oral Microbiol. (2016) 8:32762. doi: 10.3402/jom.v8.32762
103. Park SR, Kim DJ, Han SH, Kang MJ, Lee JY, Jeong YJ, et al. Diverse Toll-like receptors mediate cytokine production by Fusobacterium nucleatum and Aggregatibacter actinomycetemcomitans in macrophages. Infect Immun. (2014) 82:1914–20. doi: 10.1128/IAI.01226-13
104. Dickinson BC, Moffatt CE, Hagerty D, Whitmore SE, Brown TA, Graves DT, et al. Interaction of oral bacteria with gingival epithelial cell multilayers. Mol Oral Microbiol. (2011) 26:210–20. doi: 10.1111/j.2041-1014.2011.00609.x
105. Peyyala R, Kirakodu SS, Novak KF, Ebersole JL. Oral microbial biofilm stimulation of epithelial cell responses. Cytokine. (2012) 58:65–72. doi: 10.1016/j.cyto.2011.12.016
106. Zhang G, Chen R, Rudney JD. Streptococcus cristatus modulates the Fusobacterium nucleatum-induced epithelial interleukin-8 response through the nuclear factor-kappa B pathway. J Periodontal Res. (2011) 46:558–67. doi: 10.1111/j.1600-0765.2011.01373.x
107. Karpinski TM. Role of oral microbiota in cancer development. Microorganisms. (2019) 7:10020. doi: 10.3390/microorganisms7010020
108. Hamada S, Koga T, Nishihara T, Fujiwara T, Okahashi N. Characterization and immunobiologic activities of lipopolysaccharides from periodontal bacteria. Adv Dent Res. (1988) 2:284–91. doi: 10.1177/08959374880020021301
109. Rath-Deschner B, Nogueira AVB, Beisel-Memmert S, Nokhbehsaim M, Eick S, Cirelli JA, et al. Interaction of periodontitis and orthodontic tooth movement-an in vitro and in vivo study. Clin Oral Investig. (2021) 26:171–81. doi: 10.1007/s00784-021-03988-4
110. de Andrade KQ, Almeida-da-Silva CLC, Coutinho-Silva R. Immunological pathways triggered by Porphyromonas gingivalis and Fusobacterium nucleatum: therapeutic possibilities? Mediators Inflamm. (2019) 2019:7241312. doi: 10.1155/2019/7241312
111. Johnson L, Almeida-da-Silva CLC, Takiya CM, Figliuolo V, Rocha GM, Weissmuller G, et al. Oral infection of mice with Fusobacterium nucleatum results in macrophage recruitment to the dental pulp and bone resorption. Biomedical J. (2018) 41:184–93. doi: 10.1016/j.bj.2018.05.001
112. Sims GP, Rowe DC, Rietdijk ST, Herbst R, Coyle AJ. HMGB1 and RAGE in inflammation and cancer. Annu Rev Immunol. (2010) 28:367–88. doi: 10.1146/annurev.immunol.021908.132603
113. Aung WPP, Chotjumlong P, Pata S, Montreekachon P, Supanchart C, Khongkhunthian S, et al. Inducible expression of A Disintegrin and Metalloproteinase 8 in chronic periodontitis and gingival epithelial cells. J Periodontal Res. (2017) 52:582–93. doi: 10.1111/jre.12426
114. Khongkhunthian S, Techasatian P, Supanchart C, Bandhaya P, Montreekachon P, Thawanaphong S, et al. Elevated levels of a disintegrin and metalloproteinase 8 in gingival crevicular fluid of patients with periodontal diseases. J Periodontol. (2013) 84:520–8. doi: 10.1902/jop.2012.120262
115. Ainola M, Li TF, Mandelin J, Hukkanen M, Choi SJ, Salo J, et al. Involvement of a disintegrin and a metalloproteinase 8 (ADAM8) in osteoclastogenesis and pathological bone destruction. Ann Rheum Dis. (2009) 68:427–34. doi: 10.1136/ard.2008.088260
116. Ishizuka H, Garcia-Palacios V, Lu G, Subler MA, Zhang H, Boykin CS, et al. ADAM8 enhances osteoclast precursor fusion and osteoclast formation in vitro and in vivo. J Bone Miner Res. (2011) 26:169–81. doi: 10.1002/jbmr.199
117. Choi SJ, Han JH, Roodman GD. ADAM8: a novel osteoclast stimulating factor. J Bone Miner Res. (2001) 16:814–22. doi: 10.1359/jbmr.2001.16.5.814
118. Atanasova KR, Yilmaz O. Prelude to oral microbes and chronic diseases: past, present and future. Microbes Infect. (2015) 17:473–83. doi: 10.1016/j.micinf.2015.03.007
119. Guerrero-Preston R, White JR, Godoy-Vitorino F, Rodriguez-Hilario A, Navarro K, Gonzalez H, et al. High-resolution microbiome profiling uncovers Fusobacterium nucleatum, Lactobacillus gasseri/johnsonii, and Lactobacillus vaginalis associated to oral and oropharyngeal cancer in saliva from HPV positive and HPV negative patients treated with surgery and chemo-radiation. Oncotarget. (2017) 8:110931–48. doi: 10.18632/oncotarget.20677
120. Schmidt BL, Kuczynski J, Bhattacharya A, Huey B, Corby PM, Queiroz EL, et al. Changes in abundance of oral microbiota associated with oral cancer. PLoS ONE. (2014) 9:e98741. doi: 10.1371/journal.pone.0098741
121. Bronzato JD, Bomfim RA, Edwards DH, Crouch D, Hector MP, Gomes B. Detection of Fusobacterium in oral and head and neck cancer samples: a systematic review and meta-analysis. Arch Oral Biol. (2020) 112:104669. doi: 10.1016/j.archoralbio.2020.104669
122. Abdulkareem AA, Shelton RM, Landini G, Cooper PR, Milward MR. Periodontal pathogens promote epithelial-mesenchymal transition in oral squamous carcinoma cells in vitro. Cell Adh Migr. (2018) 12:127–37. doi: 10.1080/19336918.2017.1322253
Keywords: F. nucleatum, oral epithelial cells, immune response, cytokines, receptors, infection, cancer, periodontitis
Citation: Groeger S, Zhou Y, Ruf S and Meyle J (2022) Pathogenic Mechanisms of Fusobacterium nucleatum on Oral Epithelial Cells. Front. Oral. Health 3:831607. doi: 10.3389/froh.2022.831607
Received: 08 December 2021; Accepted: 28 February 2022;
Published: 05 April 2022.
Edited by:
Octavio Alberto Gonzalez, University of Kentucky, United StatesReviewed by:
Giorgio Gabarrini, Karolinska Institutet (KI), SwedenFermín E. González, University of Chile, Chile
Copyright © 2022 Groeger, Zhou, Ruf and Meyle. This is an open-access article distributed under the terms of the Creative Commons Attribution License (CC BY). The use, distribution or reproduction in other forums is permitted, provided the original author(s) and the copyright owner(s) are credited and that the original publication in this journal is cited, in accordance with accepted academic practice. No use, distribution or reproduction is permitted which does not comply with these terms.
*Correspondence: Sabine Groeger, sabine.e.groeger@dentist.med.uni-giessen.de