- 1African Membrane Society (AMSIC), a Ecole Nationale d’Ingénieurs du Mali Abderhamane Baba Touré, Bamako, Mali
- 2i2i Innovation MegaHub (“i2iMegaHub”), Accra, Ghana
- 3Agence Nationale de la Valorisation des Résultats de la Recherche et du Développement Technologique (ANVREDET), El Djazair, Algeria
The impacts of climate change are real and in many parts of the world testify to its harsh reality, including rampant extreme weather events, droughts, heat, wildfires, and flooding which have recorded in places which have not experienced them in recent memory. In the quest to avert such events, there is a growing awareness and demand for sustainable processes and operations. Today, sustainability encompasses a balance between ecological footprint and human development index, taking into consideration economics, the green environment, safety, quality, ethics, diversity and inclusion (D&I), and communities. This article presents some steps that have been taken by Algeria to balance energetic autonomy and sustainable development, and a case study on green hydrogen production employing membrane processes. Algeria’s objective to join the global fight against climate change is to develop its green hydrogen base. Given its resources, including available solar and wind power, seawater desalination plants, building capacity, and its favorable location, it is developing its green hydrogen economy to supply hydrogen, especially to Europe. This presents an opportunity for other developing nations, especially in Africa, to gain from this experience.
1 Introduction
Hydrogen by nature is a green fuel and produces little to no toxic emissions if used effectively (Wang et al., 2023; Anwar et al., 2021). Hydrogen is projected to be the clean energy of the future and it is expected that green hydrogen will be a decarbonization substitute for much of the fossil fuel energy used today, hence reducing the impacts posed by non-green fuels (Al-Zareer et al., 2018; Lee et al., 2018; Anwar et al., 2021; Nasser and Hassan, 2023).
Hydrogen as a fuel is fundamentally green; however, it is the means of its production that classifies it as green, grey, blue, red/pink, cyan/turquoise, brown and black, green, yellow, and white (Lubbe et al., 2023; Chaudhary et al., 2024). A fully green production process is preferable given the increasing requirements to reduce environmental impacts and decarbonization (Chi and Yu, 2018; Lubbe et al., 2023).
This study will share some of the different non-green and green hydrogen production technologies and color codes. Algeria currently produces grey hydrogen and is investigating ways to change to green hydrogen to tap into the European market. Algeria now is considering strategies to move into this space to reduce environmental impacts.
Here we will discuss the global demand trends for hydrogen, the types of hydrogen, and their production sources. The focus will be on green hydrogen, various technologies used to produce it, its drawbacks, the various cost contributors, and future research focuses.
Most hydrogen currently produced comes from the natural gas or coal industry, as well as steam reforming of natural gas; in general, these are not fully green (Wang et al., 2023). There is, however, a drive to boost green hydrogen production despite its challenges.
The different color coding categorization of produced hydrogen is generally based on the energy source, carbon emission associated with the production process, the overall environmental impacts, and the specific production process (Arcos and Santos, 2023).
Membrane science and technology have and will have a significant role to play in renewable and non-renewable hydrogen production as far as electrolyzers, water treatment, and separation and purification are involved.
2 Literature
2.1 Current uses of hydrogen
Industrial processes that use hydrogen include: hydrodesulfurization, where sulfur is removed from fuels in the petroleum refining industry (Lee et al., 2018); the metal industry for treatment such as sintering, brazing, annealing, powder coating, and metal injection modeling; in the fertilizer industry for ammonia-based fertilizer production (Chaudhary et al., 2024); in the food industry for oil hydrogenation for margarine production (Puprasit et al, 2022). Hydrogen has the potential to replace or supplement natural gas for cooking and heating; hydrogen-powered fuel cells provide the energy to power vehicles, maritime vessels, and aviation, as well as devices such as cell phones, laptops, and back-up and emergency power in buildings and military applications (Chaudhary et al., 2024). More areas of interest are emerging for hydrogen application (Agyekum et al., 2022; Osman et al., 2022; Chaudhary et al., 2024).
2.2 Global demands for hydrogen
Hydrogen Insight projects that the global demand for hydrogen in 2050 is expected to more than triple. This will mainly be driven by aviation, power generation/energy storage, heavy industry (E1A, 2019; Otto et al., 2022; Dally, 2024), water and road transport, and residential/commercial heating. S&P Global Commodity Insights projects that by mid-century, the total global hydrogen produced (249.5 MT) will comprise 67% green hydrogen, 16% blue hydrogen fossil fuel with carbon capture and storage (CCS), and the remaining 17% produced from unabated gas or coal without carbon capture and storage (CCS) technology (Collins, 2023).
Moreover, it is expected that 17% of total global hydrogen produced will be traded across borders from areas with abundant wind and solar power, including Australia, Chile, North Africa, and the Middle East, to carbon conscious regions such as the Europeans Union and East Asia (Collins, 2023).
2.3 Global projection for green hydrogen production
Rystad Energy through Hydrogen Insight projects the top ten green hydrogen producing nations for 2023–2030 to be Australia, the United States, Spain, Canada, Chile, Egypt, Germany, India, Brazil, and Morocco, with Australia, the US, and Spain in the lead (Klevstrand, 2003). Table 1 shows some of the companies in the individual top ten (10) hydrogen producing countries projected for 2023–2030.
2.4 Hydrogen type color coding and production technologies
Several technologies are involved in the production of renewable and non-renewable hydrogen (Anwar et al., 2021; Hydrogen Technologies, 2023). Table 2 shows hydrogen color codes assocaited with the different hydrogen production processes. Ambiguities exist in the literature on hydrogen color coding, highlighting the need for a global uniform color coding.
Figure 1 shows the various non-renewable and renewable hydrogen production technology relative positions on technology maturation versus readiness (Hydrogen Technologies, 2023).
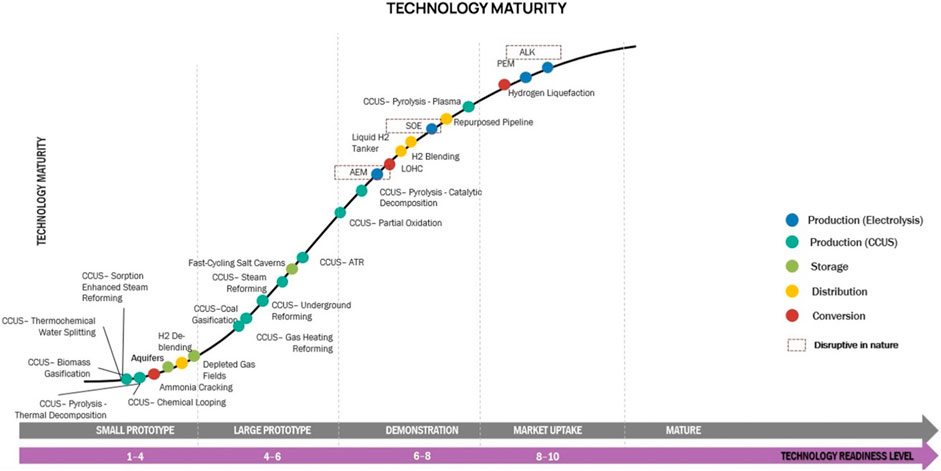
Figure 1. Hydrogen production technology maturation versus technology readiness level (Hydrogen Technologies, 2023).
The demand for green hydrogen is focused the on the four promising electrolyzer types: alkaline (ALK), polymer electrolyte membrane (PEM), anion exchange membrane (AEM), and solid oxide electrolyte (SOE) (Chi and Yu, 2018; Lu et al., 2023). They have advantages and disadvantages, challenges they face and expected future research and development directions (Kumar and Lim, 2022).
2.5 Green hydrogen production technologies involving electrolyzers
Green hydrogen production involves electrolyzers, which use electrodes, membranes, electrolytes, water, and renewable energy, comprising ALK, PEM, AEM, and SOE mentioned above (Chi and Yu, 2018; Kumar and Lim, 2022; Lu et al., 2023). Both ALK and PEM are commercialized while SOE and AEM are still in the pre-commercialization stage, with SOE ahead of AEM in development (Patonia and Poudineh, 2022; Franco and Giovannini, 2023; Hydrogen Technologies, 2023).
Figure 2 shows the operation mechanisms of these four. Each has advantages and disadvantages that need to be understood and explored for their proper selection, application, and operation (Franco and Giovannini, 2023).
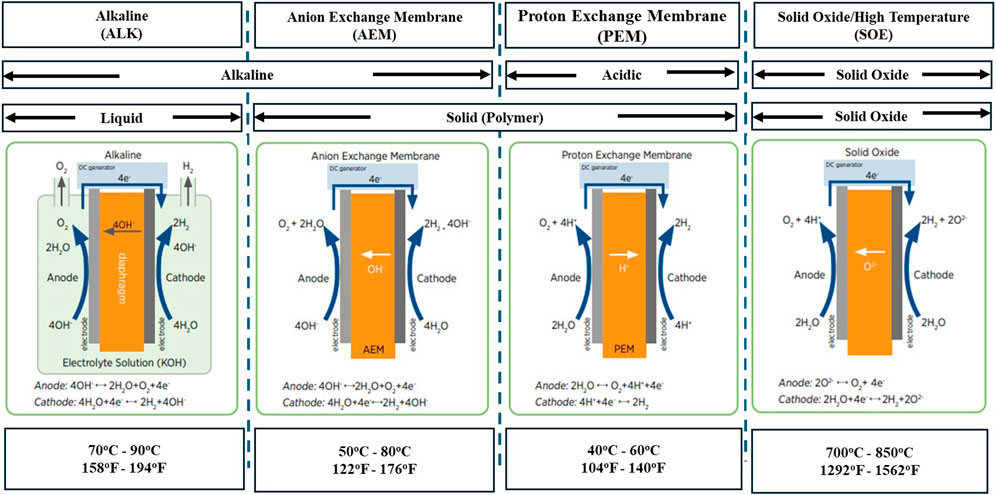
Figure 2. Promising electrolyzer types (IRENA, 2020; El-Shafie, 2023).
2.6 A basic water electrolyzer–green hydrogen production system
A basic water electrolyzer–green hydrogen production system consists of feed water into a gas separator to remove all gases including oxygen (O2) produced from the electrolyzer stack(s) on the input end, and an electrolyzer stack(s) where renewable electricity is supplied to split the feed water. The output end has another gas separator to enhance hydrogen purification, a deoxo unit to further purify the hydrogen produced, and a unit to dry the hydrogen for compression to storage (IRENA, 2020; El-Shafie, 2023).
2.7 Global demand for green hydrogen production electrolyzers and the companies involved
The increasing demand for hydrogen, specifically green hydrogen, has led to an increasing demand for electrolyzers. For the United States and Europe, ALK is dominant and will remain so into the near future, then PEM followed by SOE and then AEM. For manufacturers outside the United States and Europe, a twelve-fold growth (i.e. 1000E to 12,000E) is expected by 2030, driven by ALK, PEM, and SOE. The total global demand for electrolyzers is expected to see a six-fold growth by 2025 and a ten-fold growth by 2035, also mainly driven by ALK, PEM, and SOE (Ernst and Young LLP, 2023).
2.8 Various electrolyzers: advantages, disadvantages, and challenges
These electrolyzers have different electrodes and electrolyte materials, construction, installation, feedwater requirements, and associated operational costs. The electrolyte carries the created chemical charges from one electrode to the other.
From a general and simplistic standpoint, an electrolyzer system is composed of water and electrical supply systems, electrodes comprised of an anode (positive) and cathode (negative), membrane(s), and a separation system for the gases produced.
A general electrolyzer operational flow process is depicted in Figure 3. With the electrical system turned on, (1) the water supplied to the electrolyzer at the anode side undergoes oxidation where both O2 and positively charged hydrogen ions (H+) are produced alongside the release of electrons, and (2) the membrane(s) selectively transport H+ to the cathode to be reduced to hydrogen gas (US Department of Energy, 2024).
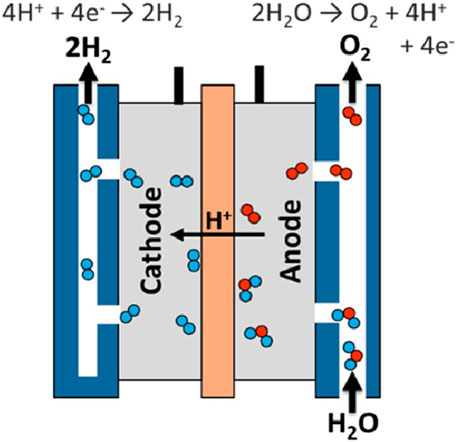
Figure 3. Electrolyzers operational flow process (US Department of Energy, 2024).
Figure 2 shows the various reactions at the anodes and cathodes for the individual PEM, ALK, AEM and SOE electrolyzers.
Both ALK and AEM electrolyzers are considered alkaline systems while PEM as acidic and SOE is a solid oxide or ceramic (IRENA, 2020; Patonia and Poudineh, 2022).
The alkaline systems use liquid electrolytes such as potassium hydroxide (KOH) and sodium hydroxide (NaOH), while PEM uses acids such as perflurosulfonated acids (PFSA) (El-Shafie, 2023). Both AEM and PEM use solid polymers, and SOE uses solid oxide or ceramic. The alkaline systems(i.e., ALK and AEM) are involved in the transport of anions—hydroxide or hydroxyl ions (OH−)—through the electrolyte from the cathode to the anode, while the acidic system (i.e., PEM) selectively transports cations—protons or H+ —through the solid polymer electrolyte from the anode to the cathode. SOE selectively transports anions—charged oxygen ions (O2-)—through the solid oxide or ceramic electrolyte from the cathode to the anode. For all four electrolyzers, hydrogen is generated on the cathode side. ALK is the most mature, durable, and cheapest (Simoes et al., 2021; Nasser and Hassan, 2023; Yang, et al., 2023).
However, the drawbacks of ALK include only pressurized versions being compatible with renewable electricity and having lower purity hydrogen than PEM (Nasser and Hassan, 2023) While AEM could compete with ALK from a cost perspective and better compatibility with renewable electricity, it has lower degradation rates (Miller, 2022; Yang, et al., 2023).
Commercialized PEM, while compatible with renewable electricity, has high material costs (Wang et al., 2023). SOE, the most efficient and compatible with renewable electricity, is associated with very high operating temperature and lower durability (Nasser and Hassan, 2023) and is still in development.
PEM requires the use of pure water while ALK uses a variety of water sources, including seawater and wastewater. PEM’s pure water requirement could make it expensive and, in some cases, limit its use. Similarly, the pressurized ALK version for renewable energy compatibility makes it less efficient than PEM and could limit its use. ALK electrode material includes nickel and iron, making it more durable and less sensitive to feedwater impurities (Hydrogen Newsletter, 2022).
Generally, AEM requiring drinking quality water (Du et al., 2022) produces better results with similar PEM iridium anode (Miller, 2022) while SOE is exploring the use of a wide range of water qualities, especially impure water sources (Maddaloni et al., 2023).
ALK, PEM, and AEM, which operate at lower temperatures compared than SOE, have improved performance, longer device lives, and produce higher quality hydrogen with quality feedwater water. However, quality feedwater adds to the costs, process complexities, and design limitations (Becker et al., 2023).
2.9 Environmental and social impacts of green hydrogen production
Green hydrogen is considered a potential fuel to reduce the overall global carbon footprint; however, the green hydrogen industry is associated with some environmental and social impacts and risks (Vernick, 2024). The hydrogen industry production, storage, and utilization is associated with environmental and social risks and impacts (Vernick, 2024).
However, the resultant impact is expected to be lower. Green hydrogen production involves the use of energy, water, and land. The land acquisition and clearing for green hydrogen production plants is associated with both environmental and social risk and impacts. Environmental risk and impacts include deforestation and habitat loss (Tolba and El-Kholy, 1992), soil erosion and degradation (Lal, 2001), water pollution and decreased water quality (Camara et al., 2019), loss of biodiversity and ecosystem disruption (Mullu, 2016), and increased greenhouse emissions (Romijn, 2011).
Social risks and impacts include displacement of indigenous communities and loss of traditional lands (Moreda, 2017), conflict and human violation (Grant and Das, 2015), economic impacts on local communities, particularly loss of livelihood (Hufe and Heuermann, 2017), cultural heritage destruction (Pankaj et al., 2023), and health impacts, including increased respiratory problems (Myers et al., 2013).
Moreover, energy is required to split water to produce green hydrogen, and the energy source needs to be green (Osman et al., 2022). The equipment used in green hydrogen production itself could be associated with a significant carbon footprint (Hurwitz et al., 2023). Additionally, the production process requires a significant quantity of water which could lead to water scarcity (Vernick, 2024). The different electrolyzers require different water quality, which in turn demands different treatment methods with different environmental or social impacts. Hydrogen stored in high pressure containers and pipelines can leak and cause explosions (Vernick, 2024). Ensuring both environmental and safety integration will lower overall environmental and safety impacts and risks. Moreover, the proper integration of land, energy, and water alongside the selection of a production process and storage systems reduces overall impacts and risks.
3 Green hydrogen production cost and economic viability
There is a general drive to increase green hydrogen adoption, partly due to lower environmental impacts and costs, while driving favorable policies over its non-green hydrogen counterparts (Ishaq et al., 2022).
The electrolyzers and renewable electricity costs (Badgett et al., 2022) are among the factors which may be hindering green hydrogen growth and adoption. Hence, as costs reduce, green hydrogen will be able to compete with the dominant fossil fuel hydrogen.
The total cost of ownership (TCO) of green hydrogen mostly includes the costs of electrolyzers, ancillary equipment, installation, and operational costs. Ancillary equipment to electrolyzers includes cooling equipment, compression, electricity sources, water treatment, purification, dryers, and power electronics (IRENA, 2020).
There are costs associated with installing a complete system, while operating costs involve consumable and unit costs involved in the production process, including the electricity consumption rate and cost, water quality and consumption rate, and stack degradation and replacement rates. Other factors affecting overall production costs include operational efficiency, economy of scale, increasing automation, and design complexities. A large capacity system operating close to maximum capacity for longer hours is preferred for reducing costs and improving return on investment from an operational efficiency standpoint. Improving electrolyzers and their electrical efficiency are expected to reduce electrical consumption and costs. Generally, increasing module and manufacturing plant sizes can all significantly reduce costs in addition to automation (Corbeau and Merz, 2023). Each of the above factors could impact the overall cost differently, given that all the electrolyzers have some distinct differences.
From 2019 to 2022, electrical efficiencies generally improved for each type of electrolyzer. Electrolyzers improved in the following order: SOE > PEM > ALK > AEM. In 2050, system electrical efficiencies expressed in kilowatt-hours per kilogram of green hydrogen produced are expected to be lowest for SOE (<40) and similarly for ALK, PEM and AEM (<45) (IRENA, 2020; Patonia and Poudineh, 2022).
Generally, ALK requiring the use of low-cost steel or nickel alloy-plated material has the lowest cost (Miller et al., 2020). However, costs are associated with controlling the hydroxide electrolyte solution concentration, temperature, corrosion (Lohmann-Richters et al., 2021), and hydrogen produced needing further purification to meet some requirements.
PEM requires the use of expensive platinum-group metals, mostly iridium, to withstand the corrosive acid operating environment. The high voltage applied for high hydrogen production rates results in higher initial costs (Corbeau and Merz, 2023). This restricts opportunities for cost reduction.
SOE, still in development, has the flexibility of being constructed from a variety of materials including steel, nickel, and zirconia (Hauch et al., 2020). This offers some advantages in reducing costs. However, in the current developmental stages, manufacturing process complexities mean that it is more expensive than ALK and PEM. The expectation is that SOE still will implement cost reduction opportunities (Minary-Jolandan, 2022).
AEM is a better alternative to ALK for easy renewable energy compatibility, costs the same as ALK, and performs like PEM. Moreover, AEM is being explored as an alternative to PEM but with lower overall costs. AEM performance with PEM iridium anodes has returned the best AEM results (Miller, 2022; Yang, et al., 2023).
In conclusion, selection of any of these electrolyzers depends on the specific application, availability of resources and infrastructure, and expected project viability (Hydrogen Newsletter, 2022).
While green hydrogen production using an electrolyzer is driven by many factors, available electricity and costs are among the most critical. Industry guides suggest that economic viability could favor higher efficiency and higher capex electrolyzers such as SOE, which may be applicable to areas with a scarcity of renewable electricity, while lower efficiency and lower capex electrolyzers such as ALK may be suitable for areas with sufficient renewable electricity. Driving green hydrogen production costs down depends on the upfront investment, electricity availability and cost, efficiency, stack degradation, and replacement rates. Other considerations include the effects of module size, manufacturing economy of scale, hydrogen produced for specific applications, feed water quality, and the integration of an electrolyzer of interest with intermittent renewable energy operation (Corbeau and Merz, 2023).
Remarkably, some of these parameters may be coupled such that an improvement in any of the parameters could adversely impact the others. This requires making a conscious decision on selection. The ALK, AEM, PEM, and SOE electrolyzers operate differently, hence entailing different cost implications, effectiveness, and production system designs (Kumar and Lim, 2022).
Furthermore, the fast-paced industrial innovation occurs behind closed doors, so up-to-date information is lacking in the public domain. This lack of access to new information means that outdated information is available to academia and the public (Corbeau and Merz, 2023).
The role of membrane science and technology in green hydrogen production using electrolytic splitting of water cannot be overstated, from transporting ionic species from one electrode to the other in electrolyzers, treating the different water sources to meet electrolyzers’ feed water quality to ensure improved productivity and system lives, and producing hydrogen purification.
Increasing use of green hydrogen over established fuels will not only lead to decarbonization of the energy system but a revolution in the water industry, leading to improved water treatment technologies and water use strategies in the face of dwindling clean water resources. The water industry is and will play a vital role in electrolytic green hydrogen production given that water is one of the key raw materials. Hence, countries seeking to adopt net zero strategies should consider a more integrated approach to renewable electricity, water, and green hydrogen (Newborough and Cooley, 2021).
Future green hydrogen development includes increasing adoption of renewable hydrogen by lowering costs and instituting favorable policies to promote it over non-green hydrogen (Kumar and Lim, 2022).
SOE, still in development, is expected to emerge as the best electrolytic solution with lower costs and high efficiency (Miller, 2022). Other green hydrogen developments include exploring (1) photolytic means, where sunlight is directly used to split water into hydrogen and oxygen, (2) thermochemically converting biomass into liquid or gas and separating hydrogen, and (3) biological production of hydrogen by microbes (EIA, 2023).
Growing infrastructure demands include hydrogen refueling stations, storage, and transportation which are crucial for the industry’s success and growth. Economic opportunities associated with green hydrogen include the growth of new industries, job creation, and economic expansion (Hassan et al., 2024; Jones, 2024). The future of hydrogen as part of the global energy mix will depend on continued investment, improvement, lowering of costs, increasing adoption, and policy direction.
4 The Algerian case study: existing capabilities and preparation for its green hydrogen economy
Algeria is a motivated player in the hydrogen industry given that is has many assets for becoming a regional and international player. Currently, Algeria is involved in the blue hydrogen industry. However, it wants to join the fight against climate change and plans to become a leading green hydrogen production country. The road map towards this includes (1) regulatory and institutional adaptation, (2) human capital development, (3) industrial integration and growth, (4) financing mechanisms and incentives, (5) international cooperation and technology transfer, and (6) deployment of the hydrogen sector. The road map involves the following actionable phases with objectives and timelines. (1) Start-up phase (2023–2030) focusing on start-up activities and training, laying the groundwork for building projects and expertise, and initiating pilot projects to explore hydrogen production and utilization. (2) Market expansion and creation phase (2030–2040) that emphasizes the shift to market expansion and creation, scale-up, and the establishment of markets for the applications. (3) Industrialization and export phase (2040–2050) that prioritizes the export of hydrogen derivatives and contributing to global energy markets. (National Hydrogen Development Strategy in Algeria, 2023).
Algeria’s energy transition quest will promote energy transition, innovation, and efficiency (ITA, 2023). Moreover, Algeria seeks to establish a supportive hydrogen sector framework by 2050 dubbed the “The Algerian Strategy on Green Hydrogen 2050”. Algeria’s ambitious target includes reducing its greenhouse gases (GHG) and petroleum products consumption. Algeria projects to export 30–40 TWh of gaseous, liquefied, and derived green hydrogen by 2040 (Stambouli et al., 2024).
Algeria is taking the necessary steps in developing strategies and policies. It is forming internal and external alliances and dealing with some of the existing challenges to make it align with the needs of a green hydrogen economy. Algeria’s plans to appeal to the European market across the Mediterranean. It has acquired land and solved its water scarcity issues by building desalination plants as well as boosting green energy resources with solar and wind energy systems. Moreover, Algeria is considering ways to reduce the impacts of its blue hydrogen industry amid its pursuit of green hydrogen production. Furthermore, it is collaborating with local energy clusters to build and sustain the overall energy ecosystem (National Hydrogen Development Strategy in Algeria, 2023).
Algeria’s Sonatrach state-owned oil company signed a memorandum of understanding (MoU) with Hecate Energy Global Renewables (HGR Energy) to explore both renewable energy and green hydrogen projects in Algeria (Djunisic, 2024).
One of Algeria’s local energy clusters is the Green Energy Cluster Algeria (GEC) which is a collaborative organization including industries, universities, research centers and institutes, and representatives from key government ministries. Its main goal is to foster synergies among its members, promote competitiveness, contribute to sector-specific training to add to its human resource capacity to develop and transfer the knowledge base to support Algeria’s hydrogen economy (Green Energy Cluster Algeria, 2024).
5 Conclusion and directions
Algeria is on track with the start-up phase of its roadmap, building more capability as it positions itself, including lowering costs and moving in the right direction to grow its green hydrogen base. Currently, it is addressing challenges to its water supply by installing desalination plants, designating land, building more solar energy plants, collaborating with local energy clusters, signing MoUs with major global companies for hydrogen projects, and pilot projects to build expertise and training (National Hydrogen Development Strategy in Algeria, 2023).
The above review presents some information on renewable hydrogen production strategies and Algeria’s plans so that other developing nations can decide whether to replace existing non-renewable hydrogen production with renewable hydrogen.
Author contributions
AA-M: Writing–original draft, Writing–review and editing, Formal Analysis, Investigation, Methodology. ND: Conceptualization, Investigation, Methodology, Project administration, Resources, Supervision, Writing–review and editing. WB: Conceptualization, Investigation, Methodology, Project administration, Resources, Supervision, Writing–review and editing.
Funding
The author(s) declare that no financial support was received for the research, authorship, and/or publication of this article.
Conflict of interest
The authors declare that the research was conducted in the absence of any commercial or financial relationships that could be construed as a potential conflict of interest.
Publisher’s note
All claims expressed in this article are solely those of the authors and do not necessarily represent those of their affiliated organizations, or those of the publisher, the editors and the reviewers. Any product that may be evaluated in this article, or claim that may be made by its manufacturer, is not guaranteed or endorsed by the publisher.
References
Agyekum, E. B., Nutakor, C., Agwa, A. M., and Kamel, S. (2022). A critical review of renewable hydrogen production methods: factors affecting their scale-up and its role in future energy generation. Membr 12 (2), 173. doi:10.3390/membranes12020173
Al-Zareer, M., Dincer, I., and Rosen, M. A. (2018). Analysis and assessment of a hydrogen production plant consisting of coal gasification, thermochemical water decomposition and hydrogen compression systems. Energ. Conver. Manage. 157, 600–618. doi:10.1016/j.enconman.2017.11.047
Anez-Lingerfelt, M. (2022). Hydrogen power: why filtration is a key to a green energy future, filtration + separations. Available at: https://www.filtsep.com/content/features/hydrogen-power-why-filtration-is-the-key-to-a-green-energy-future/ (Accessed November 5, 2023).
Anwar, S., Khan, F., Zhang, Y., and Djire, A. (2021). Recent development in electrocatalysts for hydrogen production through water electrolysis. Int. J. Hydrogen Energy 46 (63), 32284–32317. doi:10.1016/j.ijhydene.2021.06.191
Arcos, J. M. M., and Santos, D. M. F. (2023). The hydrogen color spectrum: techno-economic analysis of the available technologies for hydrogen production. Gases 3 (1), 25–46. doi:10.3390/gases3010002
Badgett, A., Ruth, M., and Pivovar, B. (2022). “Economic considerations for hydrogen production with a focus on polymer electrolyte membrane electrolysis,” in Electrochemical power sources: fundamentals, systems, and applications. Editors T. Smolinka, and J. Garche (Elsevier), 327–364. doi:10.1016/B978-0-12-819424-9.00005-7
Becker, H., Murawski, J., Shinde, D. V., Stephens, I. E. L., Hinds, G., and Smith, G. (2023). Impact of impurities on water electrolysis: a review. Sustain. Energy Fuels 7, 1565–1603. doi:10.1039/D2SE01517J
Blackridge Research and Consulting (2024a). Top 10 green hydrogen companies in the world. Available at: https://www.blackridgeresearch.com/blog/list-of-top-green-hydrogen-producing-companies-in-the-world (Accessed May 1, 2024).
Blackridge Research and Consulting (2024b). Top 7 green hydrogen companies in India. Available at: https://www.blackridgeresearch.com/blog/list-of-top-green-hydrogen-producing-manufacturing-companies-in-india (Accessed May 6, 2024).
Brown, J. (2023). Top Canadian hydrogen stocks to watch. Stockhouse. Available at: https://stockhouse.com/news/the-market-herald-news/2023/09/13/top-canadian-hydrogen-stocks-to-watch (Accessed on May 2, 2024).
Camara, M., Jamil, N. R., and Abdullah, A. F. B. (2019). Impact of land uses on water quality in Malaysia: a review. Ecol. Process 8, 10. doi:10.1186/s13717-019-0164-x
Chaudhary, K., Bhardvaj, K., and Chaudhary, A. (2024). A qualitative assessment of hydrogen generation techniques for fuel cell applications. Fuel 358 (A), 130090. doi:10.1016/j.fuel.2023.130090
Chi, J., and Yu, H. (2018). Water electrolysis based on renewable energy for hydrogen production. Chin. J. Catal. 39 (3), 390–394. doi:10.1016/S1872-2067(17)62949-8
Collins, L. (2023). Global hydrogen demand to more than triple by 2050, driven by power generation, aviation, and industry. Anal. Hydrog. Available at: https://www.hydrogeninsight.com/author/l_collins (Accessed November 10, 2023).
Corbeau, A.-S., and Merz, A.-K. (2023). Demystifying electrolyzer production costs. Center on global energy policy SIPA. Columbia University. Available at: https://www.energypolicy.columbia.edu/demystifying-electrolyzer-production-costs/ (Accessed January 11, 2024).
Čučuk, A. (2023a). ACWA Power gearing up to develop $4 billion green hydrogen project in Egypt. Bus. Dev. Proj. Offshore Energy. Available at: https://www.offshore-energy.biz (Accessed May 3, 2024).
Čučuk, A. (2023b). HH2E and LEAG to boost hydrogen economy in Germany, business developments and project, offshore energy. Available at: https://www.offshore-energy.biz/hh2e-and-leag-to-boost-the-hydrogen-economy-in-germany/ (Accessed May 3, 2024).
Cummins, J. (2023). Australia’s going all-in on hydrogen – here are the companies taking development up a notch. Stockhead. Available at: https://stockhead.com.au/energy/australias-going-all-in-on-hydrogen-here-are-the-companies-taking-development-up-a-notch/ (Accessed May 1, 2024).
Dally, B. (2024). The potential role of hydrogen in decarbonizing heavy industry in Saudi Arabia in the Clean Hydrogen Economy and Suadi Arabia. 1st Ed. Routledge Taylor and Francis Group, 584–605. Available at: https://www.taylorfrancis.com/chapters/oa-edit/10.4324/9781003294290-25/potential-role-hydrogen-decarbonizing-heavy-industry-saudi-arabia-bassam-dally.
Daza, M.-L. O., Kamine, J., Vitorla, M., Perze-Marchant, J., Farr, W., and Gallagher, W. L. L. P. (2021). Chile aims to win the green hydrogen race, hydrogen and Chile. Available at: https://www.willkie.com/-/media/files/publications/2021/02/chile-aims-to-win-green-hydrogen-race.pdf (Accessed May 2, 2024).
Djunisic, S. (2024). Sonatrach, hecate energy to explore renewables, green H2 in Algeria, renewable now. Available at: https://renewablesnow.com/news/sonatrach-hecate-energy-to-explore-renewables-green-h2-in-algeria-856287/#:∼:text=Algerian%20state-owned%20oil%20company,hydrogen%20production%20projects%20in%20Algeria (Accessed May 14, 2024).
Du, N., Roy, C., Peach, R., Turnbull, M., Thiele, S., and Bock, C. (2022). Anion-exchange membrane water electrolyzers. Chem. Rev. 122 (13), 11830–11895. doi:10.1021/acs.chemrev.1c00854
E1A (2019) “The future of hydrogen report for the G20,” in Japan Seizing today’s opportunities. Available at: https://iea.blob.core.windows.net/assets/9e3a3493-b9a6-4b7d-b499-7ca48e357561/The_Future_of_Hydrogen.pdf (Accessed April 28, 2024).
EIA (2023). Hydrogen explained. Prod. Hydrogen. Available at: https://www.eia.gov/energyexplained/hydrogen/production-of-hydrogen.php (Accessed December 28, 2023).
El-Shafie, M. (2023). Hydrogen production by water electrolysis technologies: a review, Results Eng., 20, 101426. doi:10.1016/j.rineng.2023.101426
E&M Combustion (2023). The protagonists who lead Green Hydrogen projects in Spain. Available at: https://emcombustion.es/en/the-protagonists-who-lead-green-hydrogen-projects-in-spain/ (Accessed May 1, 2024).
Energy Transition (2024). Egypt targets $40 B of green hydrogen investment – plus other top energy stories. World Econ. Forum. Available at: https://www.weforum.org/agenda/2024/03/egypt-green-hydrogen-investment-plus-other-top-energy-stories/(Accessed May 3, 2024).
Ernst and Young, LLP (2023). Shortage of Electrolyzers for Green Hydrogen. Shortage of electrolyzers for green hydrogen (ey.com) (Accessed on November 25, 2023).
Franco, A., and Giovannini, C. (2023). Recent and future advances in water electrolysis for green hydrogen generation: critical analysis and perspectives. Sustainability 15 (24), 16917. doi:10.3390/su152416917
Garip, P. (2023). Is Chile adrift on green hydrogen? Americas quarterly. Available at: https://www.americasquarterly.org/article/is-chile-adrift-on-green-hydrogen/(Accessed May 2, 2024).
Grant, E., and Das, O. (2015). Land grabbing, sustainable development and human rights. Trans. Environ. Law 4 (2), 289–317. doi:10.1017/S2047102515000023
Green Energy Cluster Algeria (2024). Available at: www.gec-algeria.com (Accessed May 5, 2024).
Hassan, N. S., Jalil, A. A., Rajendran, S., Khusnun, N. F., Bahari, M. B., Johari, A., et al. (2024). Recent review and evaluation of green hydrogen production via water electrolysis for a sustainable and clean energy society. Int. J. Hydrogen Ener. 52 (Part B), 420–441. doi:10.1016/j.ijhydene.2023.09.068
Hauch, A., Küngas, R., Blennow, P., Hansen, A. B., Hansen, J. B., Mathiesen, B. V., et al. (2020). Recent advances in solid oxide cell technology for electrolysis. Science 370 (6513), eaba6118. doi:10.1126/science.aba6118
Hufe, P., and Heuermann, D. F. (2017). The local impacts of large-scale land acquisitions: a review of case study evidence from Sub-Saharan Africa. J. Contemp. Afr. Std. 35 (2), 168–189. doi:10.1080/02589001.2017.1307505
Hurwitz, Z., Bujak, N., Tapia, M., Daza, E., and Gischler, C., (2023). Key aspects for managing the environmental and social risks of green hydrogen Available at: https://blogs.iadb.org/sostenibilidad/en/key-aspects-for-managing-the-environmental-and-social-risks-of-green-hydrogen/ [Accessed May 10, 2024].
Hydrogen Newsletter (2022). PEM (proton exchange membrane) electrolyzers vs. AEL/ALK (alkaline electrolysis) electrolyzers: what is the best selection for a green hydrogen project? Available at: https://www.hydrogennewsletter.com/untitled-2/#google_vignette (Accessed November 4, 2023).
Hydrogen Technologies (2023). Technology maturation, winning imperatives: unleashing the power of hydrogen technologies for A sustainable future. Hydrogen Technol. Available at: https://www.marketsandmarkets.com/industry-practice/hydrogen/hydrogen-technologies-comparison [Accessed in November 16, 2023].
IRENA (2020) “Green hydrogen cost reduction: scaling up electrolysers to meet the 1.5⁰C climate goal, international renewable energy agency,” in Abu Dhabi Green hydrogen cost reduction: scaling up electrolysers to meet the 1.5C climate goal (irena.org) (Accessed October 5, 2024).
Ishaq, H., Dincer, I., and Crawford, C. (2022). A review on hydrogen production and utilization: challenges and opportunities. Int. J. Hyd. Energy 47 (62), 26238–26264. doi:10.1016/j.ijhydene.2021.11.149
ITA (2023). Algeria - renewable energy. Available at: https://www.trade.gov/energy-resource-guide-algeria-renewable-energy (Accessed December 30, 2023).
Jones, R. (2024). The economics of green hydrogen: costs, competitiveness, and ROI. Available at: https://www.azocleantech.com/article.aspx?ArticleID=1826.
Kinch, D. (2003). Brazil 'well-positioned' as green hydrogen exporter to EU steel: energy Transition. Nat. Gas, Metals S P Glob. Commod. Insight. Available at: https://www.spglobal.com/commodityinsights/en/market-insights/latest-news/metals/062223-brazil-well-positioned-as-green-hydrogen-exporter-to-eu-steel-speakers (Accessed May 6, 2003).
Klevstrand, A. (2023). Which ten countries will be the biggest producers of green hydrogen in 2030? Hydrogeninsight. Available at: https://www.hydrogeninsight.com/author/a_klevstrand (Accessed November 14, 2023).
Kumar, S. S., and Lim, H. (2022). An overview of water electrolysis technologies for green hydrogen production. Energy Rep. 8, 13793–13813. doi:10.1016/j.egyr.2022.10.127
Lee, B., Heo, J., Kim, S., Sung, C., Moon, C., Moon, S., et al. (2018). Economic feasibility studies of high pressure PEM water electrolysis for distributed H2 refueling stations. Energ. conver. manage. 162, 139–144. doi:10.1016/j.enconman.2018.02.041
Linde, (2024). Linde to increase green hydrogen production in Brazil. Available at: https://www.linde.com/news-and-media/2024/linde-to-increase-green-hydrogen-production-in-brazil (Accessed May 6, 2024).
Liponi, A., Pasini, G., Baccioli, A., and Ferrari, L. (2023). Hydrogen from renewables: is it always green? The Italian scenario. Ener Conver. Manage. 276, 116525. doi:10.1016/j.enconman.2022.116525
Lohmann-Richters, F. P., Renz, S., Lehnert, W., Müller, M., and Carmo, M. (2021). Review -challenges and opportunities for increased current density in alkaline electrolysis by increasing the operating temperature. J. Electrochem. Soc. 168 (11), 114501. doi:10.1149/1945-7111/ac34cc
Lu, X., Du, B., Zhou, S., Zhu, W., Li, Y., Yang, Y., et al. (2023). Optimization of power allocation for wind-hydrogen system multi-stack PEM water electrolyzer considering degradation conditions. Int. J. Hydro. Energy. 48 (15), 5850–5872. doi:10.1016/j.ijhydene.2022.11.092
Lubbe, F., Rongé, J., Bosserez, T., and Martens, J. A. (2023). Golden hydrogen. Chem 39, 100732. doi:10.1016/j.cogsc.2022.100732
Maddaloni, M., Marchionni, M., Abbá, A., Mascia, M., Tola, V., Carpanese, M. P., et al. (2023). Exploring the viability of utilizing treated wastewater as a sustainable water resource for green hydrogen generation using solid oxide electrolysis cells (SOECs). Water 15 (14), 2569. doi:10.3390/w15142569
Matalucci, S. (2023). The Hydrogen Stream: Brazil reveals $3.6 billion hydrogen production plan. Available at: https://www.pv-magazine.com/2023/11/28/the-hydrogen-stream-brazil-plans-3-6-billion-of-hydrogen-production/ (Accessed May 6, 2024).
Miller, H. A. (2022). Green hydrogen from anion exchange membrane water electrolysis. Cur. Opin. Electrochem 36, 101122. doi:10.1016/j.coelec.2022.101122
Miller, H. A., Bouzek, K., Hnat, J., Loos, S., Bernäcker, C. I., Weißgärber, T., et al. (2020). Green hydrogen from anion exchange membrane water electrolysis: a review of recent developments in critical materials and operating conditions. Sustain. Energy Fuels 4, 2114–2133. doi:10.1039/C9SE01240K
Minary-Jolandan, M. (2022). Formidable challenges in additive manufacturing of solid oxide electrolyzers (SOECs) and solid oxide fuel cells (SOFCs) for electrolytic hydrogen economy toward global decarbonization. Ceramics 5 (4), 761–779. doi:10.3390/ceramics5040055
Moreda, T. (2017). Large-scale land acquisitions, state authority and indigenous local communities: insights from Ethiopia. Third World Q. 38 (3), 698–716. doi:10.1080/01436597.2016.1191941
Mullu, D. (2016). A review on the effect of habitat fragmentation on ecosystem. J. Nat. Sci. Res. 6 (15). Available at: https://www.iiste.org/Journals/index.php/JNSR/article/view/32219/33106.
Myers, S. S., Gaffikin, L., Golden, C. D., Ostfeld, R. S., Redford, K. H., Taylor, H. R., et al. (2013). Human health impacts of ecosystem alteration. PNAS 110 (47), 18753–18760. doi:10.1073/pnas.1218656110
Nasser, M., and Hassan, H. (2023). Techno-enviro-economic analysis of hydrogen production via low and high temperature electrolyzers powered by PV/Wind turbines/Waste heat. Energ. Conver. Manage. 278, 116693. doi:10.1016/j.enconman.2023.116693
National Hydrogen Development Strategy in Algeria, 2023 (2023). Available at: https://www.energy.gov.dz/Media/galerie/doc_strategie_nationale_hydrogene_v.fr_(sept.2023)_65b65e6f0b8eb.pdf (Accessed May 5, 2024).
Newborough, M., and Cooley, G. (2021). Green hydrogen: water use implications and opportunities. Fuel Cells Bull. 12, 12–15. doi:10.1016/S1464-2859(21)00658-1
Osman, A. I., Mehta, N., Elgarahy, A. M., Hefny, M., Al-Hinai, A., Al-Muhtaseb, A. H., et al. (2022). Hydrogen production, storage, utilisation and environmental impacts: a review. Chem. Lett. 20, 153–188. doi:10.1007/s10311-021-01322-8
Otto, M., Chagoya, K. L., Blair, R. G., Hick, S. M., and Kapat, J. S. (2022). Optimal hydrogen carrier: holistic evaluation of hydrogen storage and transportation concepts for power generation, aviation, and transportation. J. Ener Storage 55 (Part D), 105714. doi:10.1016/j.est.2022.105714
Pankaj, S., Sravanthi, G., Khan, I., Pahwa, S., Salman, Z. N., and Sethi, G. (2023). Sustainable utilization of natural stone resources: environmental impacts and preservation of cultural heritage. E3S Web Conf. ICMPC 06, 011. doi:10.1051/e3sconf/202343001106
Patonia, A., and Poudineh, R. (2022). Cost-competitive green hydrogen: how to lower the cost of electrolysers? The Oxford Institute for Energy Studies OIES. Available at: https://www.oxfordenergy.org/wpcms/wp-content/uploads/2022/01/Cost-competitive-green-hydrogen-how-to-lower-the-cost-of-electrolysers-EL47.pdf (Accessed January 5, 2024).
Puprasit, K., Wongsawaeng, D., Ngaosuwan, K., Kiatkittipong, W., and Assabumrungrat, S. (2022). Improved hydrogenation process for margarine production with no trans fatty acid formation by non-thermal plasma with needle-in-tube configuration. J. Food Eng. 334, 111167. doi:10.1016/j.jfoodeng.2022.111167
Reuters (2024). Australia's Fortescue partners with OCP Group for green energy development in Morocco Available at: https://www.reuters.com/sustainability/climate-energy/australias-fortescue-partners-with-ocp-group-green-energy-development-morocco-2024-04-08/ [Accessed May 7, 2024].
Reuters (2024). Morocco partners with Nareva, GE Vernova in green hydrogen project. Available at: https://www.reuters.com/business/energy/morocco-partners-with-nareva-ge-renova-green-hydrogen-project-2024-01-30/ (Accessed May 7, 2024).
Romijn, H. A. (2011). Land clearing and greenhouse gas emissions from Jatropha biofuels on African Miombo Woodlands. Energy Policy 39 (10), 5751–5762. doi:10.1016/j.enpol.2010.07.041
Samir, S. (2022). Infinity power, masdar, hassan allam sign framework agreement for green hydrogen project in SCZONE, green hydrogen, Egypt oil and gas. Available at: https://egyptoil-gas.com/news/infinity-power-masdar-hassan-allam-sign-framework-agreement-for-green-hydrogen-project-in-sczone/ (Accessed May 3, 2024).
Samir, S. (2022). Egypt, CIEG sign two energy MoUs green hydrogen, Egypt oil and gas. Available at: https://egyptoil-gas.com/news/egypt-cieg-sign-two-energy-mous/ (Accessed May 3, 2024).
Samir, S. (2024). India’s Rana group eyes $12 billion investment in Egypt’s green hydrogen production. Green Hydrogen, Egypt Oil Gas. Available at: https://egyptoil-gas.com/tag/green-hydrogen/ (Accessed on May 3, 2024).
Sheikh, S. E., (2022). Fertiglobe, scatec, orascom construction and the sovereign fund of Egypt start commissioning of “Egypt green”, Africa’s first Integr. green hydrogen plant, Dur. U. N. Clim. summit Available at: https://www.globenewswire.com/news-release/2022/11/08/2550912/0/en/Fertiglobe-Scatec-Orascom-Construction-and-The-Sovereign-Fund-of-Egypt-start-commissioning-of-Egypt-Green-Africa-s-first-integrated-green-hydrogen-plant-during-UN-Climate-summit.html [Accessed May 3, 2024].
Simoes, S. G., Catarino, J., Picado, A., Lopes, T. F., Santino, D. B., Amorim, F., et al. (2021). Water availability and water usage solutions for electrolysis in hydrogen production. J. Clean. Prod. 315, 128124. doi:10.1016/j.jclepro.2021.128124
Stambouli, B., Kitamura, Y., Benmessaoud, M. T., and Noureddine, Y. (2024). Algeria's journey towards a green hydrogen future: strategies for renewable energy integration and climate commitments. Int. J. Hydrogen Energy 58, 753–763. doi:10.1016/j.ijhydene.2024.01.119
Tolba, M. K., and El-Kholy, O. A. (1992). “Deforestation and habitat loss,” in The world environment 1972–1992. Editors M. K. Tolba, and O. A. El-Kholy (Dordrecht: Springer). doi:10.1007/978-94-011-2280-1_7
US Department of Energy (2024). Hydrogen and fuel cell technologies office. Hydrogen Prod. Electrolysis. Available at: https://www.energy.gov/eere/fuelcells/hydrogen-production-electrolysis (Accessed April 30, 2024).
U.S. Geological Survey, USGS (2023). The potential for geologic hydrogen for next-generation energy. Available at: https://www.usgs.gov/news/featured-story/potential-geologic-hydrogen-next-generation-energy (Accessed December 14, 2023).
Vernick, D. (2024). What is green hydrogen, and how can it help tackle the climate crisis? Available at: https://www.worldwildlife.org/stories/what-is-green-hydrogen-and-how-can-it-help-tackle-the-climate-crisis (Accessed May 9, 2024).
Wang, Y., Wen, C., Tu, J., Zhan, Z., Zhang, B., Liu, Q., et al. (2023). The multi-scenario projection of cost reduction in hydrogen production by proton exchange membrane (PEM) water electrolysis in the near future (2020–2060) of China. Fuel 354, 129409. doi:10.1016/j.fuel.2023.129409
Keywords: hydrogen, electrolyzers, renewables, energy, sustainability, decarbonization, membranes, clean-fuel
Citation: Anim-Mensah A, Drouiche N and Boulaiche W (2024) Assessment of the economic viability, environmental, and social impacts of green hydrogen production: an Algerian case study. Front. Membr. Sci. Technol. 3:1382651. doi: 10.3389/frmst.2024.1382651
Received: 06 February 2024; Accepted: 17 June 2024;
Published: 07 August 2024.
Edited by:
Diogo Guedes Vidal, University of Coimbra, PortugalCopyright © 2024 Anim-Mensah, Drouiche and Boulaiche. This is an open-access article distributed under the terms of the Creative Commons Attribution License (CC BY). The use, distribution or reproduction in other forums is permitted, provided the original author(s) and the copyright owner(s) are credited and that the original publication in this journal is cited, in accordance with accepted academic practice. No use, distribution or reproduction is permitted which does not comply with these terms.
*Correspondence: Alexander Anim-Mensah, aTJpbWVnYWh1YkBnbWFpbC5jb20=