- 1Department of Laboratory Animals, College of Animal Sciences, Jilin University, Changchun, Jilin, China
- 2Department of Basic Medicine, Changzhi Medical College, Changzhi, Shanxi, China
- 3Department of Plastic Surgery, The First Hospital of Jilin University, Changchun, Jilin, China
Ulcerative colitis (UC) is incurable, and rational dietary interventions are important in preventing UC. Kombucha is a fermented beverage that originated in China and has a variety of activities, including antioxidant, antibacterial and hypoglycemic activities. The aim of this study was to analyze the protective effect of Kombucha polysaccharide (KP) against UC and determine its mechanism of action. The results showed that KP intervention was effective in alleviating dextran sulfate sodium salt (DSS)-induced colitis symptoms and inhibiting DSS-induced inflammation and oxidative stress. Moreover, KP was able to reduce intestinal permeability, promote the expression of tight junction proteins, and help maintain thrush cell numbers and promote mucus secretion. The 16S rDNA results indicated that KP intervention increased the abundance of Rikenellaceae_RC9_gut_group and Clostridiales_unclassified. Untargeted metabolomics techniques revealed that KP can reverse DSS-induced disorders in intestinal metabolism. This study demonstrated that KP alleviated DSS-induced colitis by helping maintain intestinal barrier integrity, regulating the gut microbiota and remodeling metabolism pathways. The findings provide a theoretical basis for the application of KP as a dietary supplement for the prevention of UC.
1 Introduction
Ulcerative colitis (UC) is a chronic inflammatory disease that severely affects the lives of patients. Its incidence is increasing every year, and there were 50,000 cases of UC in 2023 worldwide (Le Berre et al., 2023). The pathogenesis of UC is unclear, with genetics, the environment, the intestinal barrier, and immunity being the main factors that influence the development of UC (Kobayashi et al., 2020). 5-Aminosalicylic acid, thiopurines, biologics, and small molecule drugs have played a significant role in UC remission and maintenance therapy for UC, but rectal resection is still needed in 10-20% of drug-tolerant patients (Bots et al., 2018; Le Berre et al., 2020; Le Berre et al., 2023). Therefore, it is important to develop a low-cost, highly active, and safe dietary supplement for UC prevention.
Polysaccharides, as a kind of biomolecules, are widely found in animals, plants and microorganisms (Yu et al., 2018). Polysaccharides possess various physiological activities such as antioxidant, antitumor, antiviral, and anti-inflammatory (Tang and Huang, 2018; Guo et al., 2021; Cor Andrejc et al., 2022). Polysaccharides have shown great potential in the prevention and treatment of UC.Lycium barbarum polysaccharide has been shown to alleviate DSS-induced UC by repairing the intestinal barrier and modulating the gut microbiota (Li et al., 2023). Atractylodes macrocephala-derived polysaccharide was able to alleviate DSS-induced UC by modulating the ratio of Th17/Treg cells (Yang et al., 2022). Dandelion polysaccharide was able to significantly suppress DSS-induced inflammation by inhibiting the NF-κB/NLRP3 pathway and activating Nrf2 signaling (Wang et al., 2023).
Kombucha is a fermented beverage that originated in China and is traditionally made using black tea and cane sugar as a base, which is fermented in the presence of symbiotic culture of bacteria and yeast (SCOBY) (Emiljanowicz and Malinowska-Panczyk, 2020; Antolak et al., 2021; Abaci et al., 2022). The addition of a wide range of nonclassical matrices, such as fruits, vegetables, plants and herbs, makes the variety of kombucha even richer (Barakat et al., 2022; Anantachoke et al., 2023; Wu et al., 2023). Kombucha is rich in tea polyphenols and organic acids, which have a variety of activities, such as antioxidant (Jakubczyk et al., 2020), liver protection (Moreira et al., 2022), antimicrobial (Nyiew et al., 2022; Vukic et al., 2023) and hypoglycemic (Mendelson et al., 2023) activities. A polysaccharide extract of novel shiitake mushroom kombucha was shown to have immunomodulatory effects (Sknepnek et al., 2021). Several studies have shown that kombucha exerts an antimicrobial effect by regulating the body’s gut microbiota (Wang et al., 2021; Permatasari et al., 2022; Xu et al., 2022). However, it is still unknown whether polysaccharides from kombucha can prevent colitis.
Therefore, in this study, based on a DSS-induced acute mouse model of colitis, we analyzed the effect of prophylactic supplementation with KP on alleviating UC. The effects of KP on the intestinal flora and metabolism were analyzed by 16S rDNA sequencing and metabolomics techniques. The results of this study will provide a theoretical basis for the use of KP as a dietary supplement for the prevention of UC.
2 Materials and methods
2.1 Materials
Dextran sulfate sodium salt (DSS) (molecular weight 36-50 kDa) (MP Biomedicals, CA, USA) and Kombucha polysaccharide (KP) were purchased from HANSUYUAN Biology (Hanzhong, China). Enzyme-linked immunoassay (ELISA) kits for IL-1β, IL-6, TNF-α, superoxide dismutase (SOD), malondialdehyde (MDA), total antioxidant capacity (T-AOC), myeloperoxidase (MPO), and lipopolysaccharide (LPS) were purchased from Shanghai Preferred Biotechnology Co. Streptomycin, ampicillin, gentamicin and vancomycin were purchased from Dalian Meilun Biotechnology Co. The PVDF membrane was purchased from Merck Millipore (Billerica, MA, USA), while the other reagents used in western blotting were obtained from Epizyme (Shanghai, China).
The following antibodies were used in this study:
Anti-Muc2 antibody, anti-Claudin 1 antibody, anti-ZO-1 antibody, and anti-Occludin antibody were purchased from Affinity Biosciences (Cincinnati, OH, USA); anti-GAPDH antibody, and anti-rabbit IgG were purchased from Cell Signaling Technology (Danvers, MA, USA).
2.2 Animals and experimental design
Forty-eight SPF-grade 6-week-old male BALB/c mice were purchased from Liaoning Changsheng Biotechnology Co. and housed in the barrier facility at Jilin University Laboratory Animal Center. The experimental protocol was approved and licensed by the Animal Ethics and Welfare Committee of Jilin University (SY202305008).
After one week of acclimatization, twenty-four mice were randomly divided into three groups, namely, the control group (NC), the model group (DSS) and the KP intervention group (DSS+KP), with 8 mice in each group. The experimental procedure is shown in Figure 1A; the NC and DSS groups were gavaged daily with sterile saline, while the treatment group was gavaged daily with 200 mg/kg KP (after referencing to the literature, the three concentrations 50 mg/kg/d, 100 mg/kg/d, and 200 mg/kg/d were utilized in initial tests, and 200 mg/kg/d was finally selected for the formal experiment) (Xiao et al., 2021; Liu et al., 2022; Yang et al., 2023). UC modeling was established by the addition of 3% DSS to the drinking water of the DSS and DSS+KP groups for 7 consecutive days starting on day 14, and the NC group continued to receive distilled water. Disease activity index (DAI) scores were observed and calculated for each mouse daily during the experimental period (Zhang et al., 2022). Observations included percent weight loss, fecal trait score, and fecal blood, and DAI was calculated using the following equation: DAI = (weight loss score + fecal trait score + fecal blood score). Samples of colon, serum and cecum contents were collected on day 22 for subsequent analysis.
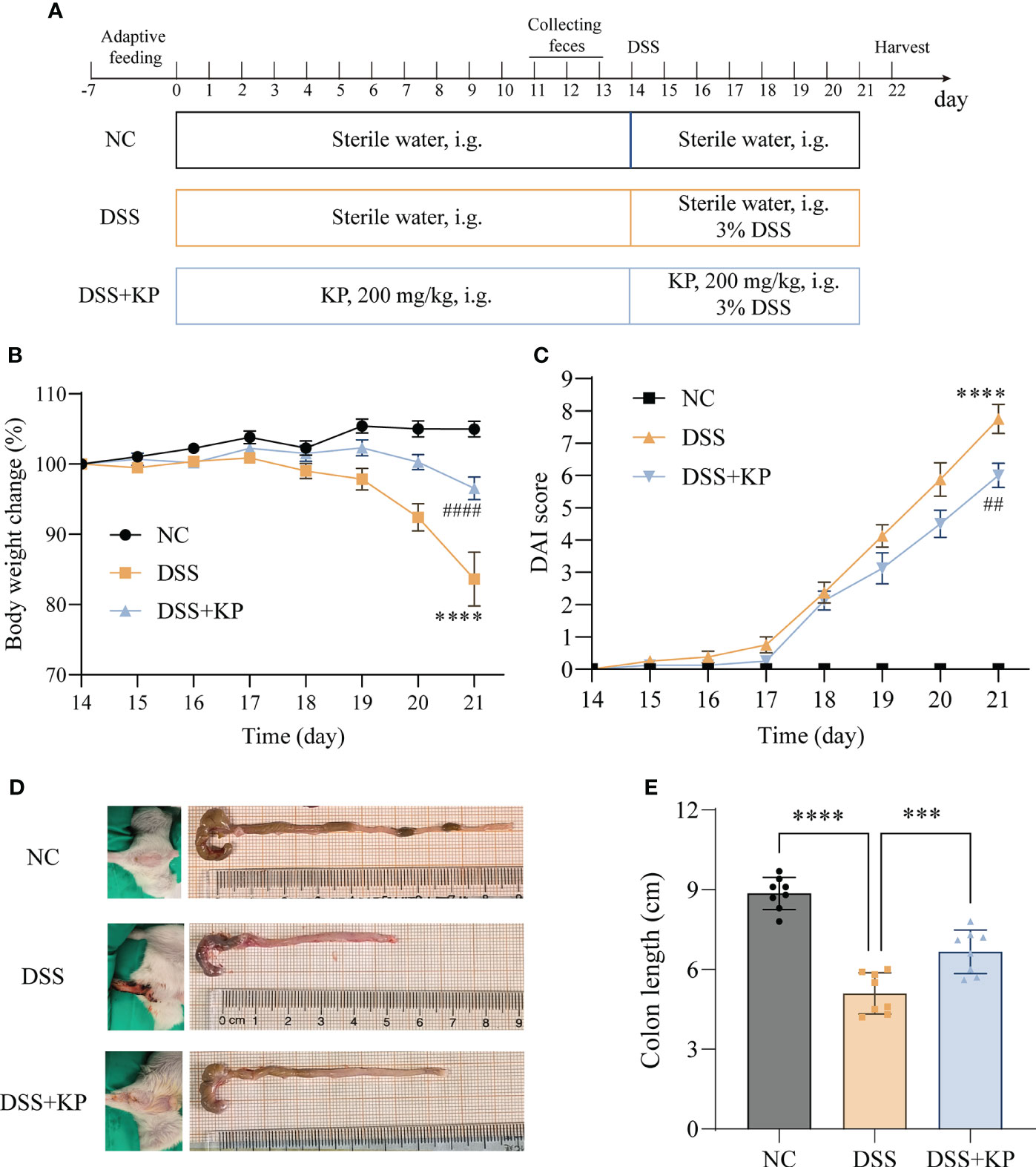
Figure 1 KP relieves DSS-induced colitis symptoms. (A) Experimental flow chart; (B) weight change curves; (C) DAI scores; (D) representative images of perianal and colon; and (E) colon lengths. (n=8) *** P<0.001, **** P<0.0001; ## P<0.01, #### P<0.0001 (* indicates comparison with the NC group and # indicates comparison with the DSS group in graphs B and C).
2.3 Biochemical indicator analysis
The blood samples were collected overnight at 4°C and centrifuged at 3000 rpm for 15 min to collect the supernatant. Colon samples were homogenized using a tissue grinder (1 g of tissue in 9 mL of PBS), and the supernatant was centrifuged at 3000 rpm for 15 min. The levels of IL-1β, IL-6, TNF-α, SOD, MDA and T-AOC in colon tissues and serum were determined, and the levels of MPO and LPS in colon tissues were determined according to the manufacturer’s instructions.
2.4 Histopathological staining
Colon tissues were immersed and fixed in 4% paraformaldehyde for 24 h. Paraffin embedding was performed according to the routine procedure, and 5 μm paraffin sections were used for subsequent staining. Hematoxylin-eosin (HE) staining was used to visualize histopathological damage; Alcian blue (AB) and periodic acid-Schiff (PAS) staining were used to observe goblet cells and mucus secretion (Shimizu et al., 2001).
2.5 Western blotting and immunohistochemistry
The expression of tight junction proteins (ZO-1, claudin 1, and occludin) in colon tissues was determined by WB. The primary antibodies that were used were an anti-ZO-1 antibody (1:1000), anti-Claudin 1 antibody (1:1000), anti-Occludin antibody (1:1000) and anti-GAPDH antibody (1:2000). The secondary antibody that was used was goat anti-rabbit IgG (1:3000). Tissue proteins were extracted using RIPA. To enhance protein stability, 1% protease inhibitor PMSF was added to RIPA. Protein separation was performed using 10% SDS-PAGE with a protein loading amount of 15 μg. The protein was then transferred to a polyvinylidene-difluoride (PVDF) membrane using the wet transfer method. Subsequently, the membrane was blocked with protein-free rapid blocking solution for 30 minutes at room temperature. After blocking, the membrane was incubated with the primary antibody for 2 hours at room temperature. Following this, the membrane was washed three times with TBST for 10 minutes each. Next, the membrane was incubated with the secondary antibody at room temperature for 1 hour. Post-incubation, the membrane was washed three times with TBST for 10 minutes each. Finally, the blots were visualized with ECL reagent.
IHC was utilized to determine Muc2 expression in colonic tissues. The primary antibodies that were used were anti-Muc2 antibodies (1:200). Detection was performed using a DAB colorimetric kit (Boster, Wuhan, China).
2.6 16S rDNA sequencing to analyze the composition of the gut microbiota
The CTAB method was selected for extraction of total DNA from cecum contents. After passing the quality test, the V3+V4 region was amplified using PCR primers (PCR primer sequences: 341F, 5’-CCTACGGGGNGGCWGCAG-3’; and 805R, 5’-GACTACHVGGGTATCTAATCC-3’). PCR amplification products were detected by 2% agarose gel electrophoresis and recovered and purified. Subsequently, 2×250 bp bipartite sequencing was performed using a NovaSeq 6000 sequencer. Bioinformatic analysis was performed using the OmicStudio tools at https://www.omicstudio.cn/. The 16S rRNA sequencing data were analyzed for significant differences using the Kruskal-Wallis test and Dunn’s post-hoc test, and q-values were obtained by correcting for multiple comparisons with the Benjamini-Hochberg test (pVal and qVal count tool was performed using the OmicStudio tools at https://www.omicstudio.cn/tool). Gut microbiota beta diversity was analyzed using principal coordinate analysis (PCoA) based on bray_curtis, jaccard and unweighted unifrac distance matrices. Discrete analyses were performed using Analysis of similarities (Anosim) to obtain R-values and P-values. Where the R-value is closer to 1, indicating that the greater the difference between samples between groups, while the smaller the difference between samples within groups, the better the grouping effect, and the P-value reflects the statistical significance of the results of Anosim’s analysis, with P<0.05 indicating whether or not the statistic is significant. Linear discriminant analysis Effect Size (LEfSe) was used to identify differentially abundant taxa with a LDA score >4.0.
2.7 Analysis of metabolites by untargeted metabolomics
Metabolites were extracted from the contents of the cecum using 80% methanol, and 0.5 mL of 80% methanol was added to each 50 mg sample and left at -20°C for 30 min, followed by centrifugation at 20,000 × g for 15 min. The supernatant was transferred to a new centrifuge tube and lyophilized. Liquid chromatography tandem mass spectrometry (LC-MS) analysis was performed after reintroducing 100 μL of 80% methanol to dissolve the samples. Samples were scanned separately in positive and negative ion mode by mass spectrometry. Differentially abundant metabolites between groups were analyzed using the wilcox.test, and the Benjamini-Hochberg test was used to correct for multiple comparisons with FDR to obtain the q-value. Supervised partial least squares discriminant analysis (PLS-DA) was performed using metaX, and variable importance for projection (VIP) values were calculated. Thresholds for defining differentially abundant metabolites between groups were multiplicity of differences ≥1.5 or ≤1/1.5, P value <0.05, and VIP value ≥1. Spearman correlation heatmap with signs was performed using the OmicStudio tools at https://www.omicstudio.cn. (no multiplicity correction done).
2.8 Statistical analysis
The experimental data are presented as the mean ± standard deviation (SD). One-way ANOVA followed by Dunnett post-hoc test was used to compare multiple groups. The data were analyzed and plotted using GraphPad Prism9.5 (La Jolla, CA, USA). All experiments contained at least 3 biological replicates, and P < 0.05 indicated that the differences were statistically significant.
3 Results
3.1 KP relieves DSS-induced colitis-like symptoms
The effect of dietary supplementation with KP on preventing colitis was evaluated based on a DSS-induced acute colitis mouse model. The results of animal experiments showed that, compared with the NC group, the mice with DSS induction showed weight loss (Figure 1B), DAI score elevation (Figure 1C), perianal hemorrhage, and colon shortening (Figures 1D, E). In contrast, KP intervention was able to significantly alleviate the above symptoms induced by DSS.
3.2 KP inhibits DSS-induced inflammation and oxidative stress
Exacerbation of the proinflammatory response and oxidative stress are typical features of colitis (Recinella et al., 2022; Zhang et al., 2022). The effect of KP intervention on DSS-induced inflammation and oxidative stress was analyzed by measuring the expression levels of IL-1β, IL-6, TNF-α, MDA, SOD, T-AOC, LPS, and MPO in serum and colon samples. The results showed that the levels of LPS and three proinflammatory factors in serum were significantly increased (P < 0.0001) after DSS treatment (Figures 2A–D); the levels of MPO, MDA, and proinflammatory factors expressed in colon tissues were significantly increased (P < 0.0001) (Figures 2E, F, I–K), and the levels of the antioxidant factors SOD and T-AOC were significantly decreased (P < 0.0001) (Figures 2G, H). KP intervention significantly suppressed DSS-induced inflammation and oxidative stress.
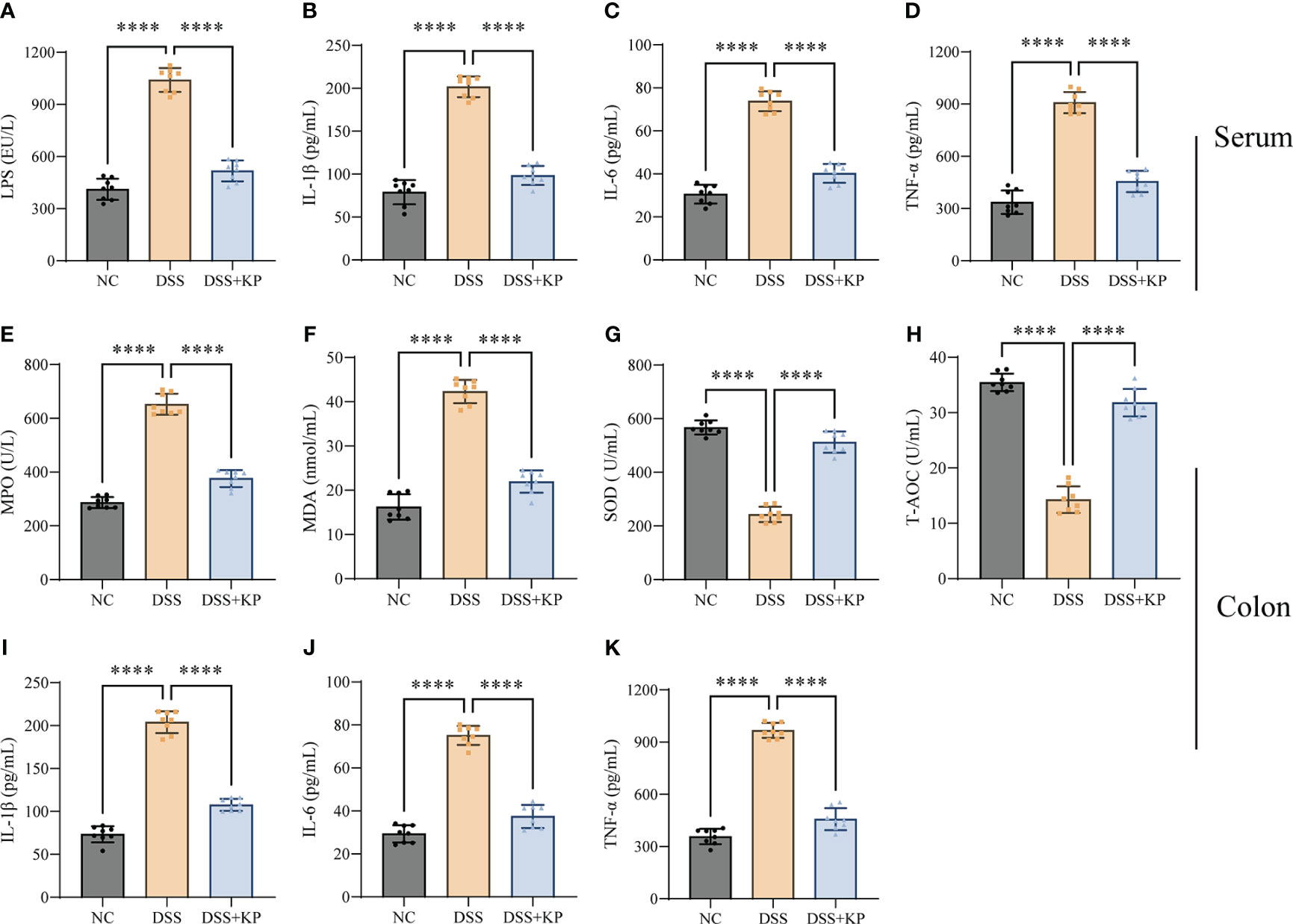
Figure 2 KP inhibits DSS-induced inflammation and oxidative stress. (A–D) Serum levels of LPS, IL-1β, IL-6, and TNF-α; (E–H) levels of MPO, MDA, SOD, and T-AOC in colonic tissues; and (I–K) levels of IL-1β, IL-6, and TNF-α in colonic tissues. (n=8) **** P<0.0001.
3.3 KP alleviates DSS-induced intestinal barrier damage
The physical intestinal barrier is the first barrier that separates the body from harmful substances in the intestines and consists mainly of tight junction proteins, goblet cells and the mucus they secrete (Mehandru and Colombel, 2021; Yao et al., 2022). The effect of KP on intestinal barrier protection was analyzed by pathological staining, IHC and WB. The results showed that DSS induced crypt loss, intestinal wall swelling, inflammatory cell infiltration (Figure 3A), mucin reduction, goblet cells loss (Figures 3B–D), and a significant decrease in the expression of tight junction proteins (P < 0.0001) (Figures 3E–H), whereas KP intervention significantly alleviated DSS-induced intestinal barrier damage and helped maintain intestinal barrier integrity.
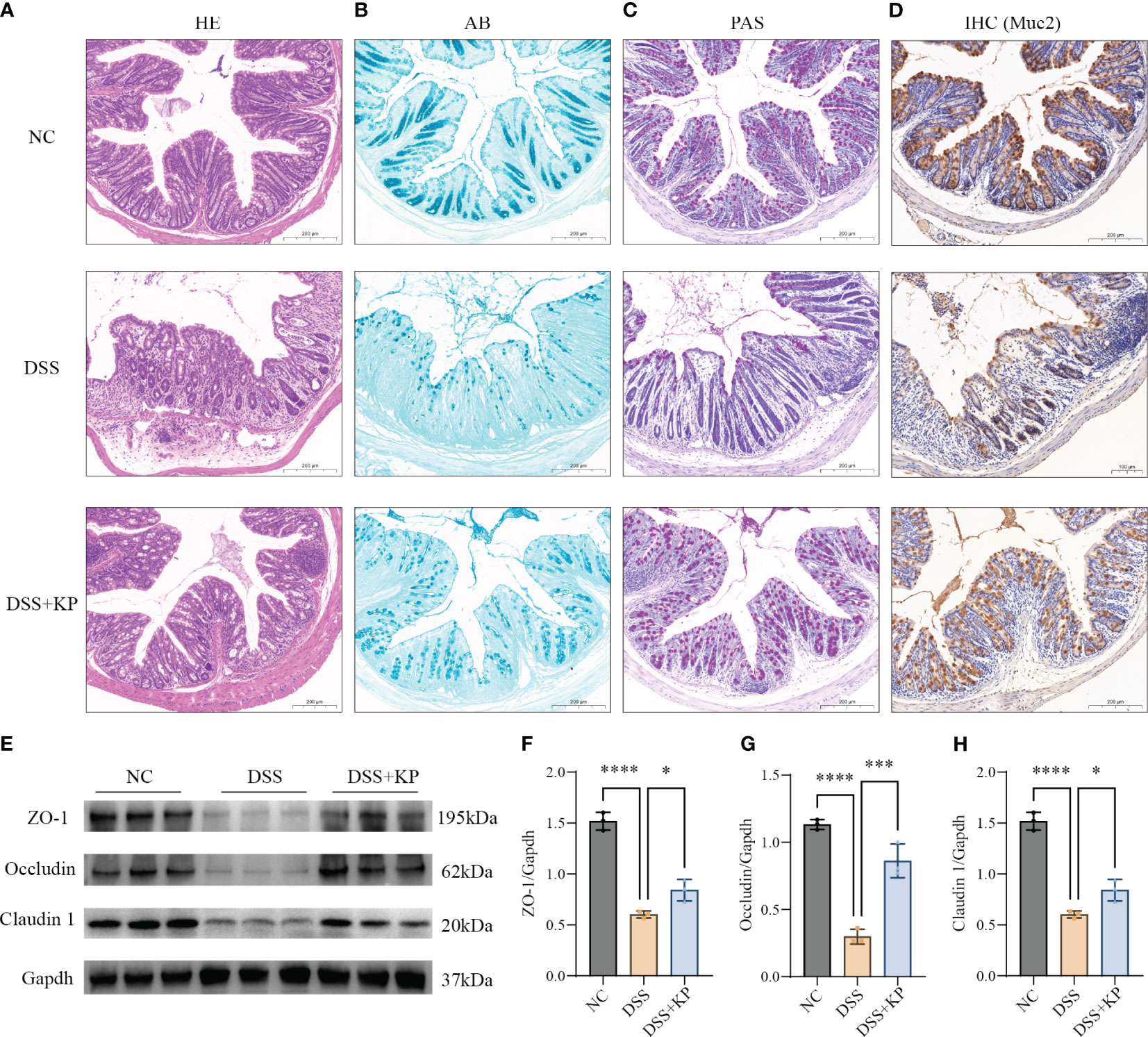
Figure 3 KP alleviates DSS-induced damage to the mechanical intestinal barrier. (A) Representative image of HE staining; (B) representative image of AB staining, AB-stained positive areas indicate mucus secreted by goblet cells; (C) representative image of PAS staining, PAS stains the mucins in the goblet cells a purplish-red color, thus indicating the location of the goblet cells; (D) IHC detection of Muc2 protein expression in colonic tissues, Muc2 is a mucin secreted by goblet cells; (E) WB detection of protein expression of ZO-1, occludin and claudin 1 in colonic tissues; and (F–H) the results of normalization of the ZO-1, occludin and claudin 1 proteins. (n=3) ns P > 0.05, * P < 0.05, *** P < 0.001, **** P < 0.0001.
3.4 KP reversed DSS-induced disorder of the gut microbiota
The gut microbiota is an ecological barrier that maintains intestinal homeostasis, and altered composition and decreased diversity of the gut flora are characteristic of ulcerative colitis, as well as being important factors that influence disease progression and treatment (Costello et al., 2019; Franzosa et al., 2019). 16S rDNA sequencing was applied to analyze gut microbiota composition. The results of alpha diversity analysis showed a significant decrease (P < 0.05) in the Shannon index after DSS induction and a significant increase (P < 0.05) in the Shannon index in the DSS+KP group compared with that of the DSS group (Figure 4A). PCoA analysis based on bray_curtis, jaccard and unweighted unifrac distance matrices., were used to assess the β-diversity of samples, and the results showed that there was a significant separation between the DSS group and the NC group, whereas the microbial composition of the DSS+KP group tended to regress toward that of the NC group (Figures 4B–D). Figures 4E, F show the gut microbiota composition at the phylum and genus levels, more detailed information is shown in Supplementary Table 1. LEfSe analysis revealed 9 and 11 differentially abundant genera and species, respectively (LDA score > 4.0) (Figures 4G, H). Among them, Clostridiales_unclassified and Rikenellaceae_RC9_gut_group were the biomarkers of DSS+KP group. In conclusion, these results indicate that KP intervention might effectively reverse at least some aspects of DSS-induced disorder.
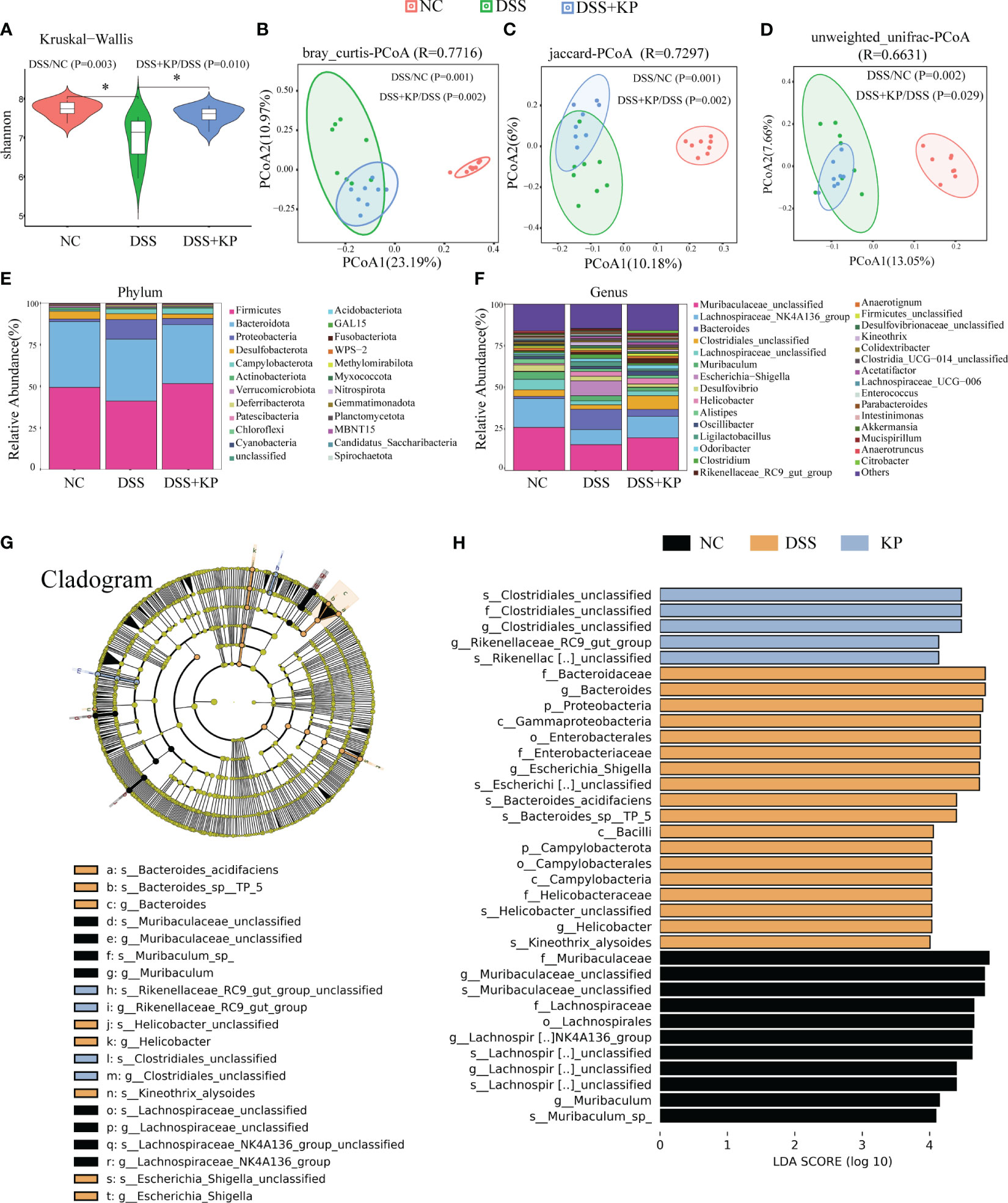
Figure 4 KP alleviates DSS-induced gut microbiota. (A) Shannon index; (B) PCoA analysis based on bray_curtis distance matrices; (C) PCoA analysis based on jaccard distance matrices; (D) PCoA analysis based on unweighted unifrac distance matrices; (E) microbial composition at the phylum level; (F) microbial composition at the genus level; and (G) The cladogram of LEfSe analysis; (H) LDA scores in differentially abundant taxa (LDA > 4.0). (n=8) * P < 0.05.
3.5 KP alleviates DSS-induced disorder of intestinal metabolism
LC-MS analysis of metabolites in the cecum contents of the three groups of mice. With a multiplicity of difference ≥1.5 or ≤1/1.5, P <0.05, and a VIP value ≥1 as the defining thresholds, in negative ion mode, the expressions of 2056 metabolites were downregulated and 1698 metabolites were upregulated in the DSS group compared with the NC group; the expressions of 1332 metabolites were downregulated and 1266 metabolites were upregulated in the DSS+KP group compared to the DSS group. In positive ion mode, the expressions of 1413 metabolites were downregulated and 1263 metabolites were upregulated in the DSS group compared with the NC group, and the expressions of 953 metabolites were downregulated and 996 metabolites were upregulated in the DSS+KP group compared with the DSS group (Figure 5A). Figures 5B, C shows the clustering of differentially abundant metabolites between the two groups in the form of a heatmap. PLS-DA analysis showed that the three groups of mice had different metabolic profiles (Figures 5D, E). Figure 5F shows the composition of metabolites in the three groups in the form of a heat map and shows that KP intervention can reverse DSS-induced disorder of intestinal metabolism. Figures 5G–K specifically shows the abundance of five metabolites (Ganoderic Acid A, Ganoderic acid beta, Indole-3-carboxyaldehyde, Indole-3-propionic acid and Sulfanilamide) in DSS and DSS+KP groups, more detailed information is shown in Supplementary Table 2.
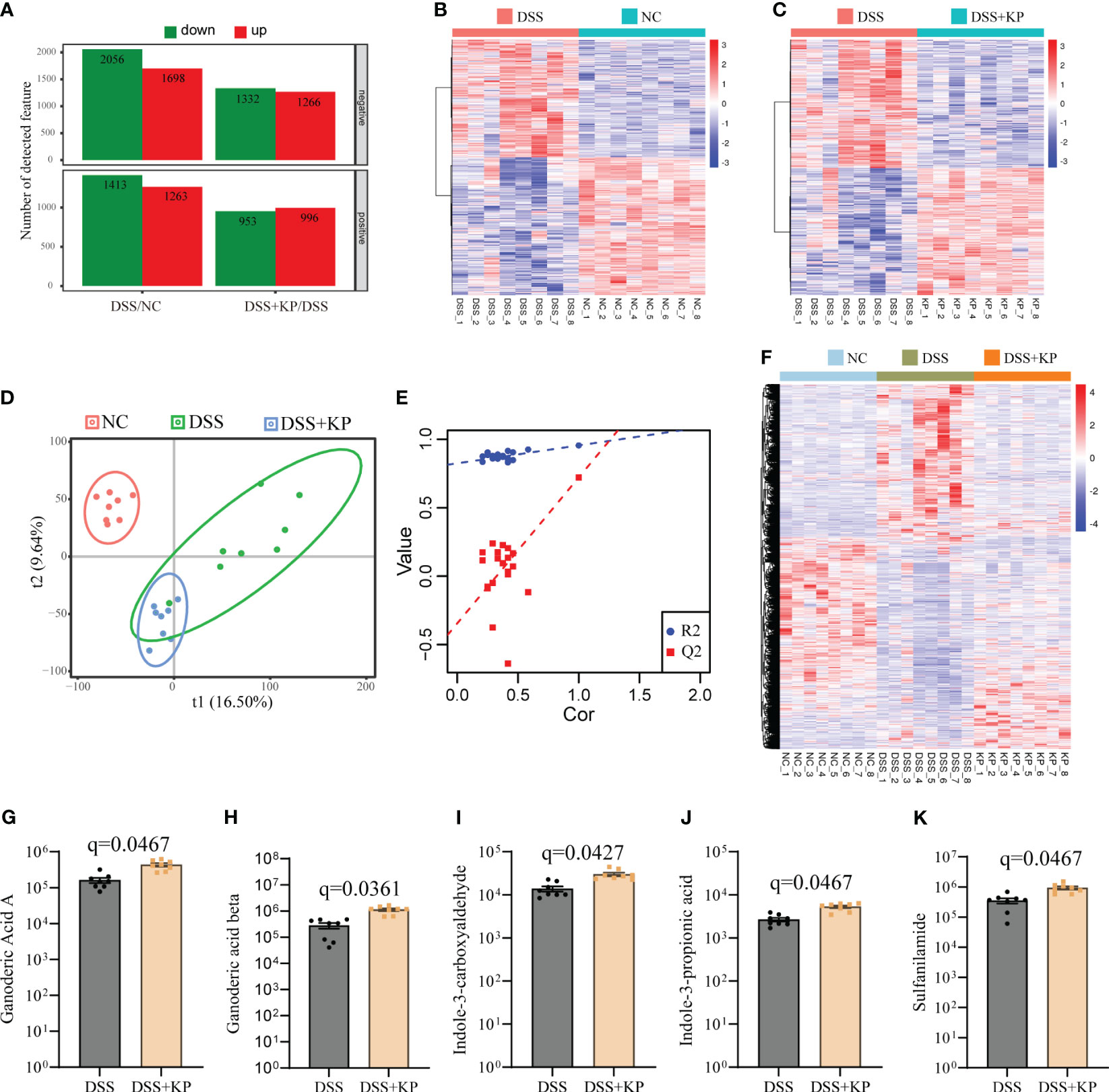
Figure 5 Effect of KP on intestinal metabolism in DSS-induced colitis mice. (A) Differentially abundant metabolites between groups in positive and negative ion mode, with the criteria for determining differences as fold change ≥1.5 or ≤1/1.5, P value <0.05, and VIP value ≥1; (B) heatmap of differentially abundant metabolites between the DSS group and the NC group; (C) heatmap of differentially abundant metabolites between the DSS group and the DSS+KP group; (D) PLS-DA score plot; (E) PLA-DA analysis of the permutation test results; (F) heatmap showing the composition of metabolites in the three groups; and (G-K) Ganoderic Acid A, Ganoderic acid beta, Indole-3-carboxyaldehyde, Indole-3-propionic acid and Sulfanilamide 5 metabolites in DSS and DSS+KP groups. (n=8).
Spearman analysis was used to reveal correlations between key bacteria, metabolites, and physiological and biochemical indicators. The results showed that Rikenellaceae_RC9_gut_group and Clostridiales_unclassified were negatively correlated with proinflammatory factors and oxidative indices and positively correlated with antioxidant factors and differentially abundant metabolites (Figure 6).
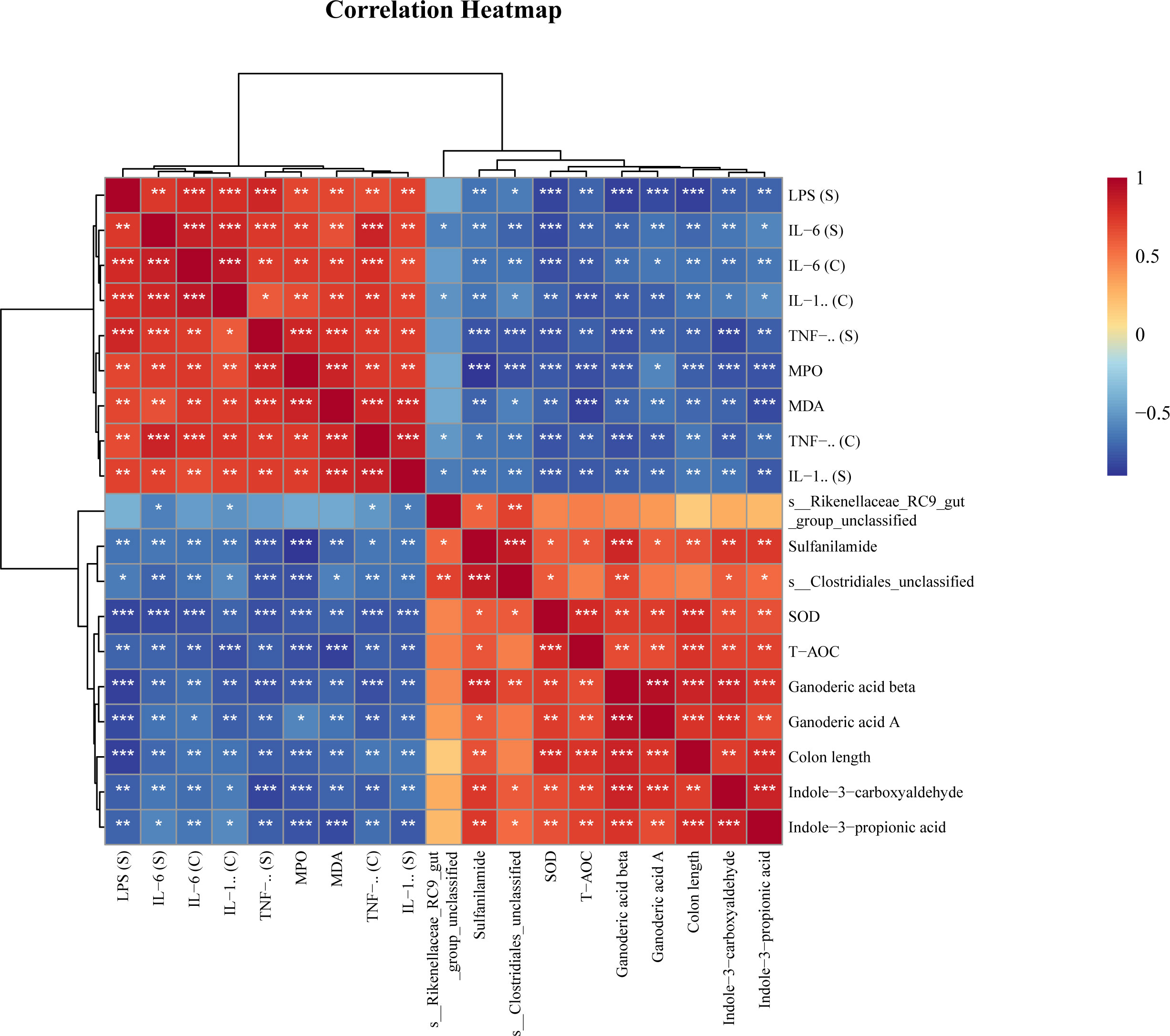
Figure 6 Spearman correlation analysis of key bacteria, metabolites and physiological and biochemical indicators. * P<0.05, ** P<0.01, *** P<0.001.
4 Discussion
UC is one of the most common inflammatory bowel diseases, with an incidence rate that increases year after year, and its course is characterized by alternating remission-relapse states (Le Berre et al., 2023). There is not yet a cure for UC, and the current goal is to alleviate symptoms and prevent and treat complications (Gros and Kaplan, 2023). The introduction of the food-as-medicine concept has led to the realization that improved health can be achieved through nutrition (Mozaffarian et al., 2022). Numerous studies have shown that natural polysaccharides exhibit great potential in the prevention and treatment of UC by influencing the intestinal microflora (Guo et al., 2021; Xu et al., 2023), regulating metabolism (Liu et al., 2022; Xu et al., 2023), maintaining the integrity of the intestinal barrier (Xiao et al., 2021), and improving immunity (Zou et al., 2023). Kombucha is a fermented beverage that has several beneficial effects in the body, and in this study, based on a DSS-induced acute UC model, we found that dietary supplementation with KP significantly alleviated the symptoms of DSS-induced colitis and suppressed inflammation and oxidative stress.
Impaired intestinal barrier integrity is a typical feature of UC. The intestinal barrier includes the mechanical intestinal barrier, the microbial barrier, and the immune barrier. Our findings demonstrate that dietary supplementation with KP reduces intestinal permeability, promotes the expression of tight junction proteins, helps maintain goblet cells numbers and promoting mucus secretion. Additionally, dietary supplementation with KP increases the diversity of the gut microbiota and modulates the composition of gut microbiota. Rikenellaceae_RC9_gut_group produces propionic acid and butyric acid using succinic acid or lactic acid as substrates (Hosomi et al., 2022). Functionally, Rikenellaceae_RC9_gut_group regulates lipid metabolism and glucose metabolism homeostasis (Zhou et al., 2018; Sebastia et al., 2023) and alleviates oxidative stress (Tang et al., 2024). In addition, ginsenoside Rg1 was reported to alleviate ulcerative colitis in obese mice, whereas ginsenoside Rg1 was able to up-regulate the abundance of the Rikenellaceae_RC9_gut_group in terms of gut microbiota composition (Zhong et al., 2023). The function of Clostridiales_unclassified is associated with short-chain fatty acid metabolism (Tsukuda et al., 2021; Tian et al., 2022). The abundance of Rikenellaceae_RC9_gut_group and Clostridiales_unclassified were differentially abundant after KP intervention. These results suggest that KP can alleviate gut microbiota imbalance and prevent colitis.
Metabolic disorders occur in patients with IBD, and metabolites show promise for the diagnosis, staging, and treatment of IBD (Williams et al., 2009; Martin et al., 2016; Bjerrum et al., 2021). Multiple drugs inhibit intestinal inflammatory responses by remodeling tryptophan metabolism and bile acid metabolism (Liu et al., 2022; Xiao et al., 2022; Wang et al., 2023; Yan et al., 2023). KP intervention was able to significantly increase the abundance of metabolites such as Ganoderic Acid A, Ganoderic acid beta, Indole-3-carboxyaldehyde, Indole-3-propionic acid and Sulfanilamide. Ganoderic Acid A inhibits the NF-κB pathway and the TLR4/NLRP3 pathway (Wang et al., 2022; Zheng et al., 2022). Ganoderic acid A protects against inflammatory diseases by regulating the intestinal flora, and the T helper cell 17/regulatory T-cell balance (Zhang et al., 2021; Lv et al., 2022). In addition, ganoderic acid beta promotes the production of the anti-inflammatory factor IL-10 (Liu et al., 2022). Indole-3-propionic Acid maintains intestinal barrier integrity by promoting tight junction protein expression and mucus secretion (Li et al., 2021). Indole-3-carboxaldehyde activates the Nrf2/HO-1 signaling pathway, inhibits ROS production and exerts antioxidant functions (Lu et al., 2023). Therefore, we hypothesized that KP may inhibit inflammation and oxidative stress and maintain intestinal barrier homeostasis by remodeling metabolism and increasing the levels of beneficial metabolites such as Ganoderic Acid A, Ganoderic acid beta, Indole-3-carboxyaldehyde, and Indole-3-propionic acid.
Using the body surface area method to convert equivalent doses between mice and humans, it is determined that the dose for mice is approximately 12.3 times higher than that for humans. Specifically, the dose administered to mice is 200mg/kg, thus resulting in a dose of 1138mg for a person weighing 70kg. Furthermore, it is worth noting that the aforementioned dose of 200 mg/kg in mice did not elicit any discernible acute toxic side effects. Nevertheless, to comprehensively assess its toxicological and side effects, further investigations involving chronic toxicity experiments are imperative.
In conclusion, in this study, we demonstrated that KP alleviated DSS-induced colitis by helping maintain intestinal barrier integrity, modulating the gut microbiota and remodeling metabolism pathways. The results of the study will provide a theoretical basis for the use of KP as a dietary supplement for the prevention of UC.
Data availability statement
The data presented in the study are deposited in the National Genomics Data Center repository, accession number CRA013559.
Ethics statement
The animal study was approved by Animal Ethics and Welfare Committee of Jilin University. The study was conducted in accordance with the local legislation and institutional requirements.
Author contributions
Z-HJ: Conceptualization, Formal analysis, Methodology, Writing – original draft. W-YX: Data curation, Methodology, Writing – original draft. P-SZ: Data curation, Methodology, Visualization, Writing – review & editing. W-ZR: Supervision, Writing – review & editing. H-JJ: Conceptualization, Funding acquisition, Writing – review & editing. BY: Conceptualization, Funding acquisition, Supervision, Writing – review & editing.
Funding
The author(s) declare financial support was received for the research, authorship, and/or publication of this article. This work was supported by the Changchun Science and Technology Bureau (21ZY19); the China Agriculture Research System (CARS-37).
Conflict of interest
The authors declare that the research was conducted in the absence of any commercial or financial relationships that could be construed as a potential conflict of interest.
Publisher’s note
All claims expressed in this article are solely those of the authors and do not necessarily represent those of their affiliated organizations, or those of the publisher, the editors and the reviewers. Any product that may be evaluated in this article, or claim that may be made by its manufacturer, is not guaranteed or endorsed by the publisher.
Supplementary material
The Supplementary Material for this article can be found online at: https://www.frontiersin.org/articles/10.3389/frmbi.2024.1341824/full#supplementary-material
Supplementary Table 1 | Results of the analysis of differences in microbial composition among the three groups.
Supplementary Table 2 | Differential metabolites between DSS and DSS+KP groups (q<0.05).
Abbreviations
WB, western blot; AB, alcian blue; DAI, disease activity index; DSS, dextran sulfate sodium salt; ELISA, enzyme linked immunosorbent assay; HE, haematoxylin–eosin; IBD, inflammatory bowel disease; IHC, immunohistochemistry; KP, Kombucha polysaccharide; LC-MS, Liquid chromatography tandem mass spectrometry; LEfSe, Linear discriminant analysis Effect Size; LPS, lipopolysaccharide; MDA, malondialdehyde; MPO, myeloperoxidase; PAS, periodic acid-schiff; PCoA, Principal coordinate analysis; PLS-DA, Partial Least Squares Discriminant Analysis; PVDF, polyvinylidene-difluoride; RT-qPCR, real time quantitative PCR; SCFA, short chain fatty acid; SOD, superoxide dismutase; SPF, specific pathogen free; T-ACO, total antioxidant capacity; UC, ulcerative colitis; VIP, Variable Important for the Projection; SCOBY, symbiotic culture of bacteria and yeast.
References
Abaci N., Senol Deniz F. S., Orhan I. E. (2022). Kombucha - an ancient fermented beverage with desired bioactivities: A narrowed review. Food Chem. X 14, 100302. doi: 10.1016/j.fochx.2022.100302
Anantachoke N., Duangrat R., Sutthiphatkul T., Ochaikul D., Mangmool S. (2023). Kombucha beverages produced from fruits, vegetables, and plants: A review on their pharmacological activities and health benefits. Foods 12 (9), 1818. doi: 10.3390/foods12091818
Antolak H., Piechota D., Kucharska A. (2021). Kombucha tea-a double power of bioactive compounds from tea and symbiotic culture of bacteria and yeasts (Scoby). Antioxidants (Basel) 10 (10), 1541. doi: 10.3390/antiox10101541
Barakat N., Beaufort S., Rizk Z., Bouajila J., Taillandier P., El Rayess Y. (2022). Kombucha analogues around the world: A review. Crit. Rev. Food Sci. Nutr. 63, 1–25. doi: 10.1080/10408398.2022.2069673
Bjerrum J. T., Wang Y. L., Seidelin J. B., Nielsen O. H. (2021). Ibd metabonomics predicts phenotype, disease course, and treatment response. EBioMedicine 71, 103551. doi: 10.1016/j.ebiom.2021.103551
Bots S., Gecse K., Barclay M., D'Haens G. (2018). Combination immunosuppression in ibd. Inflamm Bowel Dis. 24 (3), 539–545. doi: 10.1093/ibd/izx065
Cor Andrejc D., Knez Z., Knez Marevci M. (2022). Antioxidant, antibacterial, antitumor, antifungal, antiviral, anti-inflammatory, and nevro-protective activity of ganoderma lucidum: an overview. Front. Pharmacol. 13. doi: 10.3389/fphar.2022.934982
Costello S. P., Hughes P. A., Waters O., Bryant R. V., Vincent A. D., Blatchford P., et al. (2019). Effect of fecal microbiota transplantation on 8-week remission in patients with ulcerative colitis: A randomized clinical trial. JAMA 321 (2), 156–164. doi: 10.1001/jama.2018.20046
Emiljanowicz K. E., Malinowska-Panczyk E. (2020). Kombucha from alternative raw materials - the review. Crit. Rev. Food Sci. Nutr. 60 (19), 3185–3194. doi: 10.1080/10408398.2019.1679714
Franzosa E. A., Sirota-Madi A., Avila-Pacheco J., Fornelos N., Haiser H. J., Reinker S., et al. (2019). Gut microbiome structure and metabolic activity in inflammatory bowel disease. Nat. Microbiol. 4 (2), 293–305. doi: 10.1038/s41564-018-0306-4
Gros B., Kaplan G. G. (2023). Ulcerative colitis in adults: A review. JAMA 330 (10), 951–965. doi: 10.1001/jama.2023.15389
Guo C., Guo D., Fang L., Sang T., Wu J., Guo C., et al. (2021). Ganoderma lucidum polysaccharide modulates gut microbiota and immune cell function to inhibit inflammation and tumorigenesis in colon. Carbohydr. Polym. 267, 118231. doi: 10.1016/j.carbpol.2021.118231
Hosomi K., Saito M., Park J., Murakami H., Shibata N., Ando M., et al. (2022). Oral administration of blautia wexlerae ameliorates obesity and type 2 diabetes via metabolic remodeling of the gut microbiota. Nat. Commun. 13 (1), 4477. doi: 10.1038/s41467-022-32015-7
Jakubczyk K., Kaldunska J., Kochman J., Janda K. (2020). Chemical profile and antioxidant activity of the kombucha beverage derived from white, green, black and red tea. Antioxidants (Basel) 9 (5), 447. doi: 10.3390/antiox9050447
Kobayashi T., Siegmund B., Le Berre C., Wei S. C., Ferrante M., Shen B., et al. (2020). Ulcerative colitis. Nat. Rev. Dis. Primers 6 (1), 74. doi: 10.1038/s41572-020-0205-x
Le Berre C., Honap S., Peyrin-Biroulet L. (2023). Ulcerative colitis. Lancet 402 (10401), 571–584. doi: 10.1016/S0140-6736(23)00966-2
Le Berre C., Roda G., Nedeljkovic Protic M., Danese S., Peyrin-Biroulet L. (2020). Modern use of 5-aminosalicylic acid compounds for ulcerative colitis. Expert Opin. Biol. Ther. 20 (4), 363–378. doi: 10.1080/14712598.2019.1666101
Li J., Zhang L., Wu T., Li Y., Zhou X., Ruan Z. (2021). Indole-3-propionic acid improved the intestinal barrier by enhancing epithelial barrier and mucus barrier. J. Agric. Food Chem. 69 (5), 1487–1495. doi: 10.1021/acs.jafc.0c05205
Li Z. Y., Lin L. H., Liang H. J., Li Y. Q., Zhao F. Q., Sun T. Y., et al. (2023). Lycium barbarum polysaccharide alleviates dss-induced chronic ulcerative colitis by restoring intestinal barrier function and modulating gut microbiota. Ann. Med. 55 (2), 2290213. doi: 10.1080/07853890.2023.2290213
Liu C., Cao M., Yang N., Reid-Adam J., Tversky J., Zhan J., et al. (2022). Time-dependent dual beneficial modulation of interferon-gamma, interleukin 5, and treg cytokines in asthma patient peripheral blood mononuclear cells by ganoderic acid B. Phytother. Res. 36 (3), 1231–1240. doi: 10.1002/ptr.7266
Liu T., Ning Z., Liu P., Gao H. (2022). Cassane diterpenoid ameliorates dextran sulfate sodium-induced experimental colitis by regulating gut microbiota and suppressing tryptophan metabolism. Front. Immunol. 13. doi: 10.3389/fimmu.2022.1045901
Liu X., Zhang Y., Li W., Zhang B., Yin J., Liuqi S., et al. (2022). Fucoidan ameliorated dextran sulfate sodium-induced ulcerative colitis by modulating gut microbiota and bile acid metabolism. J. Agric. Food Chem. 70 (47), 14864–14876. doi: 10.1021/acs.jafc.2c06417
Lu Y., Yang W., Qi Z., Gao R., Tong J., Gao T., et al. (2023). Gut microbe-derived metabolite indole-3-carboxaldehyde alleviates atherosclerosis. Signal Transduct Target Ther. 8 (1), 378. doi: 10.1038/s41392-023-01613-2
Lv X. C., Wu Q., Cao Y. J., Lin Y. C., Guo W. L., Rao P. F., et al. (2022). Ganoderic Acid a from Ganoderma Lucidum Protects against Alcoholic Liver Injury through Ameliorating the Lipid Metabolism and Modulating the Intestinal Microbial Composition. Food Funct. 13 (10), 5820–5837. doi: 10.1039/d1fo03219d
Martin F. P., Ezri J., Cominetti O., Da Silva L., Kussmann M., Godin J. P., et al. (2016). Urinary metabolic phenotyping reveals differences in the metabolic status of healthy and inflammatory bowel disease (Ibd) children in relation to growth and disease activity. Int. J. Mol. Sci. 17 (8), 1310. doi: 10.3390/ijms17081310
Mehandru S., Colombel J. F. (2021). The intestinal barrier, an arbitrator turned provocateur in ibd. Nat. Rev. Gastroenterol. Hepatol. 18 (2), 83–84. doi: 10.1038/s41575-020-00399-w
Mendelson C., Sparkes S., Merenstein D. J., Christensen C., Sharma V., Desale S., et al. (2023). Kombucha tea as an anti-hyperglycemic agent in humans with diabetes - a randomized controlled pilot investigation. Front. Nutr. 10. doi: 10.3389/fnut.2023.1190248
Moreira G. V., Araujo L. C. C., Murata G. M., Matos S. L., Carvalho C. R. O. (2022). Kombucha tea improves glucose tolerance and reduces hepatic steatosis in obese mice. BioMed. Pharmacother. 155, 113660. doi: 10.1016/j.biopha.2022.113660
Mozaffarian D., Blanck H. M., Garfield K. M., Wassung A., Petersen R. (2022). A food is medicine approach to achieve nutrition security and improve health. Nat. Med. 28 (11), 2238–2240. doi: 10.1038/s41591-022-02027-3
Nyiew K. Y., Kwong P. J., Yow Y. Y. (2022). An overview of antimicrobial properties of kombucha. Compr. Rev. Food Sci. Food Saf. 21 (2), 1024–1053. doi: 10.1111/1541-4337.12892
Permatasari H. K., Nurkolis F., Gunawan W. B., Yusuf V. M., Yusuf M., Kusuma R. J., et al. (2022). Modulation of gut microbiota and markers of metabolic syndrome in mice on cholesterol and fat enriched diet by butterfly pea flower kombucha. Curr. Res. Food Sci. 5, 1251–1265. doi: 10.1016/j.crfs.2022.08.005
Recinella L., Gorica E., Chiavaroli A., Fraschetti C., Filippi A., Cesa S., et al. (2022). Anti-inflammatory and antioxidant effects induced by allium sativum L. Extracts on an ex vivo experimental model of ulcerative colitis. Foods 11 (22), 3559. doi: 10.3390/foods11223559
Sebastia C., Folch J. M., Ballester M., Estelle J., Passols M., Munoz M., et al. (2023). Interrelation between gut microbiota, scfa, and fatty acid composition in pigs. mSystems 9, e0104923. doi: 10.1128/msystems.01049-23
Shimizu T., Hirano H., Shimizu S., Kishioka C., Sakakura Y., Majima Y. (2001). Differential properties of mucous glycoproteins in rat nasal epithelium. A comparison between allergic inflammation and lipopolysaccharide stimulation. Am. J. Respir. Crit. Care Med. 164 (6), 1077–1082. doi: 10.1164/ajrccm.164.6.2012058
Sknepnek A., Tomic S., Miletic D., Levic S., Colic M., Nedovic V., et al. (2021). Fermentation characteristics of novel coriolus versicolor and lentinus edodes kombucha beverages and immunomodulatory potential of their polysaccharide extracts. Food Chem. 342, 128344. doi: 10.1016/j.foodchem.2020.128344
Tang Q., Huang G. (2018). Preparation and antioxidant activities of cuaurbit polysaccharide. Int. J. Biol. Macromol. 117, 362–365. doi: 10.1016/j.ijbiomac.2018.05.213
Tang W., Xiang X., Wang H., Zhou W., He L., Yin Y., et al. (2024). Zinc lactate alleviates oxidative stress by modulating crosstalk between constitutive androstane receptor signaling pathway and gut microbiota profile in weaned piglets. Anim. Nutr. 16, 23–33. doi: 10.1016/j.aninu.2023.10.001
Tian B., Geng Y., Wang P., Cai M., Neng J., Hu J., et al. (2022). Ferulic acid improves intestinal barrier function through altering gut microbiota composition in high-fat diet-induced mice. Eur. J. Nutr. 61 (7), 3767–3783. doi: 10.1007/s00394-022-02927-7
Tsukuda N., Yahagi K., Hara T., Watanabe Y., Matsumoto H., Mori H., et al. (2021). Key bacterial taxa and metabolic pathways affecting gut short-chain fatty acid profiles in early life. ISME J. 15 (9), 2574–2590. doi: 10.1038/s41396-021-00937-7
Vukic V., Vukic D., Pavlic B., Ilicic M., Kocic-Tanackov S., Kanuric K., et al. (2023). Antimicrobial potential of kombucha fresh cheese with the addition of sage (Salvia officinalis L.) and its preparations. Food Funct. 14 (7), 3348–3356. doi: 10.1039/d2fo01774a
Wang D., Cai X., Xu F., Kang H., Li Y., Feng R. (2022). Ganoderic acid a alleviates the degeneration of intervertebral disc via suppressing the activation of tlr4/nlrp3 signaling pathway. Bioengineered 13 (5), 11684–11693. doi: 10.1080/21655979.2022.2070996
Wang P., Feng Z., Sang X., Chen W., Zhang X., Xiao J., et al. (2021). Kombucha ameliorates lps-induced sepsis in a mouse model. Food Funct. 12 (20), 10263–10280. doi: 10.1039/d1fo01839f
Wang T., Song Y., Ai Z., Liu Y., Li H., Xu W., et al. (2023). Pulsatilla chinensis saponins ameliorated murine depression by inhibiting intestinal inflammation mediated ido1 overexpression and rebalancing tryptophan metabolism. Phytomedicine 116, 154852. doi: 10.1016/j.phymed.2023.154852
Wang S., Wu P., Fan Z., He X., Liu J., Li M., et al. (2023). Dandelion polysaccharide treatment protects against dextran sodium sulfate-induced colitis by suppressing nf-kappab/nlrp3 inflammasome-mediated inflammation and activating nrf2 in mouse colon. Food Sci. Nutr. 11 (11), 7271–7282. doi: 10.1002/fsn3.3653
Williams H. R., Cox I. J., Walker D. G., North B. V., Patel V. M., Marshall S. E., et al. (2009). Characterization of inflammatory bowel disease with urinary metabolic profiling. Am. J. Gastroenterol. 104 (6), 1435–1444. doi: 10.1038/ajg.2009.175
Wu S. X., Xiong R. G., Cheng J., Xu X. Y., Tang G. Y., Huang S. Y., et al. (2023). Preparation, antioxidant activities and bioactive components of kombucha beverages from golden-flower tea (Camellia petelotii) and honeysuckle-flower tea (Lonicera japonica). Foods 12 (16), 3010. doi: 10.3390/foods12163010
Xiao H., Feng J., Peng J., Wu P., Chang Y., Li X., et al. (2022). Fuc-S-a new ultrasonic degraded sulfated alpha-L-fucooligosaccharide-alleviates dss-inflicted colitis through reshaping gut microbiota and modulating host-microbe tryptophan metabolism. Mar. Drugs 21 (1), 16. doi: 10.3390/md21010016
Xiao H., Li H., Wen Y., Jiang D., Zhu S., He X., et al. (2021). Tremella fuciformis polysaccharides ameliorated ulcerative colitis via inhibiting inflammation and enhancing intestinal epithelial barrier function. Int. J. Biol. Macromol. 180, 633–642. doi: 10.1016/j.ijbiomac.2021.03.083
Xu S., Wang Y., Wang J., Geng W. (2022). Kombucha reduces hyperglycemia in type 2 diabetes of mice by regulating gut microbiota and its metabolites. Foods 11 (5), 754. doi: 10.3390/foods11050754
Xu D., Wu Q., Liu W., Hu G., Meng H., Wang J. (2023). Therapeutic efficacy and underlying mechanisms of gastrodia elata polysaccharides on dextran sulfate sodium-induced inflammatory bowel disease in mice: modulation of the gut microbiota and improvement of metabolic disorders. Int. J. Biol. Macromol. 248, 125919. doi: 10.1016/j.ijbiomac.2023.125919
Yan Y., Lei Y., Qu Y., Fan Z., Zhang T., Xu Y., et al. (2023). Bacteroides uniformis-induced perturbations in colonic microbiota and bile acid levels inhibit th17 differentiation and ameliorate colitis developments. NPJ Biofilms Microbiomes 9 (1), 56. doi: 10.1038/s41522-023-00420-5
Yang W., Ren D., Shao H., Zhang X., Li T., Zhang L., et al. (2023). Theabrownin from fu brick tea improves ulcerative colitis by shaping the gut microbiota and modulating the tryptophan metabolism. J. Agric. Food Chem. 71 (6), 2898–2913. doi: 10.1021/acs.jafc.2c06821
Yang M., Zhang Q., Taha R., Abdelmotalab M. I., Wen Q., Yuan Y., et al. (2022). Polysaccharide from atractylodes macrocephala koidz. Ameliorates dss-induced colitis in mice by regulating the th17/treg cell balance. Front. Immunol. 13. doi: 10.3389/fimmu.2022.1021695
Yao Y., Kim G., Shafer S., Chen Z., Kubo S., Ji Y., et al. (2022). Mucus sialylation determines intestinal host-commensal homeostasis. Cell 185 (7), 1172–88 e28. doi: 10.1016/j.cell.2022.02.013
Yu Y., Shen M., Song Q., Xie J. (2018). Biological activities and pharmaceutical applications of polysaccharide from natural resources: A review. Carbohydr. Polym. 183, 91–101. doi: 10.1016/j.carbpol.2017.12.009
Zhang J., Liang F., Chen Z., Chen Y., Yuan J., Xiong Q., et al. (2022). Vitexin protects against dextran sodium sulfate-induced colitis in mice and its potential mechanisms. J. Agric. Food Chem. 70 (38), 12041–12054. doi: 10.1021/acs.jafc.2c05177
Zhang Y., Wang X., Yang X., Yang X., Xue J., Yang Y. (2021). Ganoderic acid a to alleviate neuroinflammation of alzheimer's disease in mice by regulating the imbalance of the th17/tregs axis. J. Agric. Food Chem. 69 (47), 14204–14214. doi: 10.1021/acs.jafc.1c06304
Zheng S., Ma J., Zhao X., Yu X., Ma Y. (2022). Ganoderic acid a attenuates il-1beta-induced inflammation in human nucleus pulposus cells through inhibiting the nf-kappab pathway. Inflammation 45 (2), 851–862. doi: 10.1007/s10753-021-01590-0
Zhong Y., Xiao Q., Huang J., Yu S., Chen L., Wan Q., et al. (2023). Ginsenoside rg1 alleviates ulcerative colitis in obese mice by regulating the gut microbiota-lipid metabolism-th1/th2/th17 cells axis. J. Agric. Food Chem. 71 (50), 20073–20091. doi: 10.1021/acs.jafc.3c04811
Zhou L., Xiao X., Zhang Q., Zheng J., Li M., Yu M., et al. (2018). Improved glucose and lipid metabolism in the early life of female offspring by maternal dietary genistein is associated with alterations in the gut microbiota. Front. Endocrinol. (Lausanne) 9. doi: 10.3389/fendo.2018.00516
Zou M. Y., Wang Y. J., Liu Y., Xiong S. Q., Zhang L., Wang J. H. (2023). Huangshan floral mushroom polysaccharide ameliorates dextran sulfate sodium-induced colitis in mice by modulating th17/treg balance in a gut microbiota-dependent manner. Mol. Nutr. Food Res. 67 (2), e2200408. doi: 10.1002/mnfr.202200408
Keywords: ulcerative colitis, kombucha polysaccharide, gut microbiota, intestinal barrier, tight junction protein
Citation: Ji Z-H, Xie W-Y, Zhao P-S, Ren W-Z, Jin H-J and Yuan B (2024) Kombucha polysaccharide alleviates DSS-induced colitis in mice by modulating the gut microbiota and remodeling metabolism pathways. Front. Microbiomes 3:1341824. doi: 10.3389/frmbi.2024.1341824
Received: 21 November 2023; Accepted: 18 January 2024;
Published: 07 February 2024.
Edited by:
David C. B. Taras, Boehringer Ingelheim, GermanyReviewed by:
Kit Leong Cheong, Guangdong Ocean University, ChinaBenjamin P. Willing, University of Alberta, Canada
Copyright © 2024 Ji, Xie, Zhao, Ren, Jin and Yuan. This is an open-access article distributed under the terms of the Creative Commons Attribution License (CC BY). The use, distribution or reproduction in other forums is permitted, provided the original author(s) and the copyright owner(s) are credited and that the original publication in this journal is cited, in accordance with accepted academic practice. No use, distribution or reproduction is permitted which does not comply with these terms.
*Correspondence: Hong-Juan Jin, amhqQGpsdS5lZHUuY24=; Bao Yuan, eXVhbl9iYW9Aamx1LmVkdS5jbg==