- 1Department of Medical, The First Affiliated Hospital of Henan University of Chinese Medicine, Zhengzhou, China
- 2Department of the First Clinical Medical College, Henan University of Chinese Medicine, Zhengzhou, China
- 3Department of AIDS Clinical Research Center, Henan University of Chinese Medicine, Zhengzhou, China
- 4Department of Graduate, Henan University of Chinese Medicine, Zhengzhou, China
- 5Department of Zhongjing Academy of Traditional Chinese Medicine, Henan University of Chinese Medicine, Zhengzhou, China
Akkermansia muciniphila is considered the “paradigm for next-generation beneficial microorganisms” and has been reported to help alleviat immune-related diseases. Evidence shows that herbal medicine can treat disease by regulating the abundance of A. muciniphila. Recent studies have revealed a link between A. muciniphila and immune-related diseases. Here, we systematically reviewed the association between A. muciniphila, herbal medicine, and immune-related diseases (including inflammatory bowel disease, human immunodeficiency virus, cancer immunotherapy, and immune-related liver injury). We also summarize the potential mechanisms of action of A. muciniphila and offer perspectives for future studies.
1 Introduction
Immune-related diseases (IRDs) prevalence widely around the world, with an increasing incidence of autoimmune disease in recent years (Brodin, 2022). The mechanisms of some IRDs might be related to alterations in immune–microbe interactions and impaired immune function, leading to recurrent infections, chronic inflammation, and nutritional deficiency. The gut microbiota affects host health, and changes in the abundance of organisms within the gut microbiota have been linked with cancer, type 2 diabetes, obesity, intestinal bowel disease, and neurodegenerative diseases (Derosa et al., 2022; Bi et al., 2023; Ghotaslou et al., 2023).
The intestine is predominantly colonized by four bacterial phyla: Firmicutes, Bacteroidetes, Actinobacteria, and Verrucomicrobia (Bibbò et al., 2017). Akkermansia muciniphila is the only representative of the Verrucomicrobiota phylum and is highly effective at mucin degradation and considered the “paradigm for next-generation beneficial microorganisms”. Since A. muciniphila was discovered and characterized two decades ago, changes in this commensal bacterium have led to it being linked to multiple diseases in humans, such as IRDs and metabolic disorders (Cani et al., 2022). A. muciniphila shows alterations in abundance (in some cases a significant reduction) in patients with IRDs (Scher et al., 2015; Tan et al., 2018; Stoll et al., 2023; Zhao et al., 2023). These findings suggest that A. muciniphila may confer a clinical benefit in patients with IRDs.
Herbal medicine is gaining increasing attention worldwide because of its powerful therapeutic effects and minimal adverse effects. Herbal medicine can regulate the composition and metabolism of intestinal flora and inhibits disease by regulating the abundance of A. muciniphila (Feng et al., 2019; Su et al., 2020; Zhang et al., 2022b). Herbal medicine might have beneficial biogenic effects on A. muciniphila, and it plays an important therapeutic role by regulating the population, distribution, and metabolites of intestinal microbiota to promote its probiotic function. Herbal medicine also affects systemic immunity by increasing the abundance of A. muciniphila in the intestine (Barratt et al., 2022), suggesting that upregulation of A. muciniphila abundance may be strongly associated with IRDs, making the impact of herbal medicine on A. muciniphila and IRDs a promising area with potentially far-reaching implications. In this review, we will discuss the close relationship between herbal medicine, A. muciniphila and IRDs by examining the biological characteristics of A. muciniphila, the effects of herbal medicine in regulating the abundance of A. muciniphila, and the mechanisms underlying the role of A. muciniphila in IRDs.
2 Characteristics of A. muciniphila
A. muciniphila is an oval-shaped Gram-negative bacterium that is strictly anaerobic, non-motile, and non-endospore-forming. A. muciniphila can produce acetate, butyrate, and propionate in the gut as short-chain fatty acids via the process of mucin fermentation. Genome analysis of Akkermansia revealed four phylogroups (AmI, AmII, AmIII, and AmIV) (Kirmiz et al., 2020). Phylogroups AmIV and AmII outcompete AmI strains in antibiotic-treated mice, AmIII is predominant in the Chinese population, AmIV is predominant in Western populations, while AmI strains are most prominent in infants, children, and adolescents (Becken et al., 2021; Luna et al., 2022). A. muciniphila was initially considered a strictly anaerobic bacterium, but one study found that it could survive under microaerophilic conditions to produce additional energy relative to its oxygen sensitivity (Ghaffari et al., 2022). Phenotypes of A. muciniphila are resistant to gentamicin, penicillin G, vancomycin, kanamycin, streptomycin, tetracycline, and ciprofloxacin (Filardi et al., 2022; MaChado et al., 2022). One study found that in healthy middle-aged and older adults in southwest China, the abundance of Akkermansia positively correlated with IgA levels and the percentage of CD8+ T cells, and negatively correlated with the percentage of CD4+ T cells and the CD4+/CD8+ ratio, indicating that the intestinal flora correlates to some extent with immunity (Shen et al., 2018).
A. muciniphila affects the composition of immune cells and enhances immune regulation by regulating pleiotropic cytokines, including interferon (IFN)-γ, tumor necrosis factor (TNF)-α, Th17, interleukin (IL)10, IL33, and IL4, with multiple immunomodulatory effects (Derrien et al., 2010; Li et al., 2019; Chen et al., 2020; Katiraei et al., 2020). Preliminary evidence from preclinical studies has revealed the immune-regulatory potential of A. muciniphila. Supplementation with A. muciniphila increases the thickness of the intestinal mucus layer and improves the systemic immune status of mice (van der Lugt et al., 2019). A. muciniphila supplementation also improves immune cell chemotaxis, phagocytosis, natural killer cell activity, proliferative capacity, and reduces oxidative stress parameters and pro-inflammatory cytokines in aged mice (Cerro et al., 2022). These studies collectively support the immune-regulation potential of A. muciniphila and offer new directions for immune-related research.
3 A. muciniphila, herbal medicine, and immunomodulation
Probiotics are live organisms that confer a health benefit to the host when administered in adequate amounts (Cani, 2018). Probiotics play an important role in immune system modulation and the anti-inflammatory response (Plaza-Díaz et al., 2017). A. muciniphila alleviates persistent inflammation, mediates immunosuppression, and protects against catabolism syndrome by reshaping the microbial community. A. muciniphila is one of the most abundant microorganisms in humans with an immunoregulatory function (Sanders et al., 2019; Terrisse et al., 2022). Recent studies have demonstrated that a decreased abundance or lack of A. muciniphila is closely linked with increased inflammation in the context of multiple diseases, such as Crohn’s disease, ulcerative colitis, human immunodeficiency virus (HIV), diabetes, and obesity (Plovier et al., 2017; Depommier et al., 2019) Recently, herbal medicine has been shown to have immunomodulatory effects by regulating the abundance of A. muciniphila (Feng et al., 2019; Alharris et al., 2022).
A. muciniphila plays a key role in maintaining intestinal health by inducing intestinal adaptive immune responses and immune responses (Ansaldo et al., 2019; Wang et al., 2020). A. muciniphila can be used as a treatment strategy for IRDs by regulating the immune system and restoring balance to the intestinal flora (Table 1). Herbal medicine can be used as an immunomodulator in IRDs such as inflammatory bowel disease (IBD), rheumatoid arthritis, and hypersensitivity reactions (Teng et al., 2023). Furthermore, there is a correlation between the regulation of intestinal flora by herbal medicine and the prevention and control of IRDs (Table 2). The link between A. muciniphila and herbal medicine in IRDs has long been a topic of great interest. These studies support the interaction of A. muciniphila with herbal medicine in the treatment of IRDs and introduce new perspectives for future IRD research.
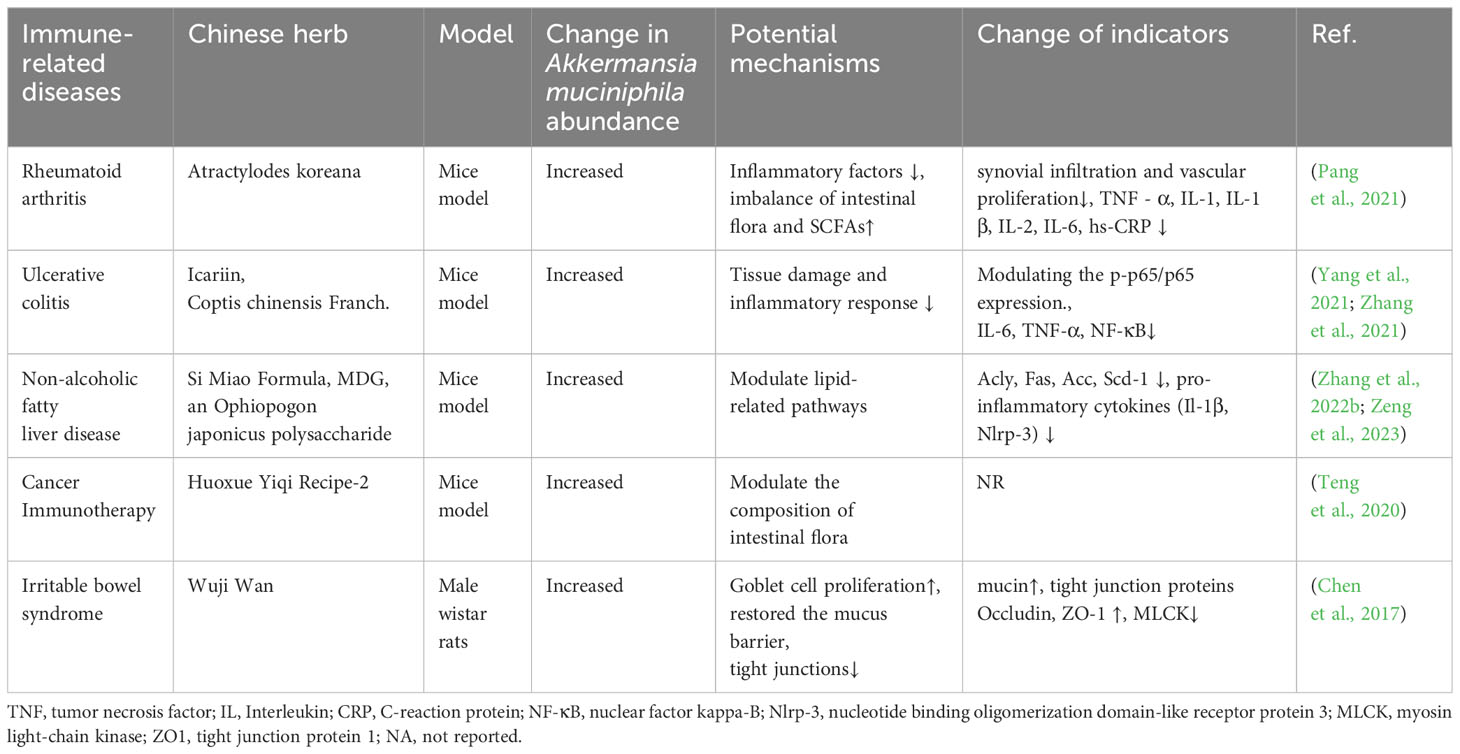
Table 2 Regulation of the intestinal flora by the active ingredients in herbal medicine for the treatment of immune-related diseases.
4 A. muciniphila, herbal medicine, and immune-related diseases
4.1 A. muciniphila, herbal medicine, and inflammatory bowel disease
IBD is a chronic, relapsing gastrointestinal disease that develops an inappropriate immune response to environmental factors in genetically-susceptible individuals (Wright et al., 2018). The onset of IBD is caused by combining the effects of barrier functions, intestinal microecology, and mucosal immunity (Vindigni et al., 2016). Reduced diversity of the intestinal flora leads to microbial dysbiosis, resulting in the occurrence of IBD (Marchesi et al., 2016; Thaiss et al., 2016). The abundance of A. muciniphila a is considered crucial in the occurrence and development of IBD. A. muciniphil, a promising probiotic, could protect against colitis via the regulation of the immune response. Integrative analysis of fecal metagenomes and serum metabolomes revealed that A. muciniphila significantly offsets the reduction in indoleacetic acid concentrations, increases the serum concentrations of indole acrylic acid, and upregulates aryl hydrocarbon receptor target genes, including CYP1A1, IL-10 and IL-22, thereby attenuating colonic inflammation (Gu et al., 2021). Additionally, the administration of A. muciniphila reduces the number of infiltrating macrophages and CD8+ cytotoxic T lymphocytes in the colon, which may improve colitis (Wang et al., 2020). A study of an acute colitis mice model found that gavage feeding of A. muciniphila decreased intestinal permeability and the level of inflammatory cytokines in serum and tissue. Analysis of 16S rDNA sequences showed that A. muciniphila induced significant gut microbiota alterations (Bian et al., 2019). Amucc_2109, an enzyme secreted by A. muciniphila, attenuates dextran sulfate sodium-induced colitis in mice, possibly in association with inhibition of the overexpression of inflammatory cytokines (Qian et al., 2022). In clinical practice, fecal microbiota transplantation has been used to treat IBD and has shown certain effects. Metagenomic sequencing indicated higher species diversity and higher abundance of anti-inflammatory bacteria in the fecal microbiota transplantation intervention group, including Alistipes putredinis, A. muciniphila, Bifidobacterium adolescentis, SCFAs-producing bacterium Christensenella minuta, and secondary bile acids-producing bacterium Clostridium leptum. Metabolomics analysis showed that indoleacetic acid and unsaturated fatty acids (DHA, DPA, and EPA) with anti-inflammatory effects were significantly enriched (Yang et al., 2022). These studies suggest that A. muciniphila could ameliorate mucosal inflammation either via microbe-host interactions, which protect the gut barrier function and reduce the levels of inflammatory cytokines, or by improving the microbial community.
Si-Ni-San (SNS) is a herbal medicine that modulates the gut microbial community and markedly inhibits inflammatory responses by improving intestinal flora dysbiosis, reducing the abundance of pro-inflammatory flora, and upregulating the abundance of anti-inflammatory species (Wang et al., 2021; Cai et al., 2023). A study exploring the potential modulatory effects of GeGen QinLian decoction on intestinal flora found that the NOD/RIP2/NF-κB signaling pathway is inhibited in the mesenteric lymph nodes and serum of mice that received fecal microbiota transplantation from mice fed GeGen QinLian decoction, these changes are associated with changes in A. muciniphila (Deng et al., 2021). Coptis chinensis is a Chinese herb that can improve the intestinal barrier by increasing the abundance of Akkermansia (Yang et al., 2023; Zhao et al., 2023). Codonopsis pilosula extract could alleviate the symptoms of acute colitis in mice by regulating the intestinal microbiota. Bacterial 16S rRNA sequencing analyzed showed that addition of Codonopsis pilosula extract stimulating the growth of three important probiotics, Bifidobacterium spp., Lactobacillus spp., and A. muciniphila. Gas chromatography determined the content of SCFAs in feces found that Codonopsis pilosula extract has selectively increased the bacteria that produced SCFAs, and promoted the production of SCFAs, alleviated malnutrition symptoms in colitis (Jing et al., 2018). These findings imply that the administration of herb medicine can ameliorates the symptoms of acute colitis.
The relationship between A. muciniphila and herb medicine play an important role in maintaining intestinal barrier integrity and intestinal microenvironment homeostasis. More animal trials combined with clinical studies are urgently needed to further elucidate the mechanisms for the effect of specific probiotic bacteria in preventing IBD.
4.2 A. muciniphila, herbal medicine, and human immunodeficiency virus
The CC chemokine receptor 5 is an attractive target for HIV as it is expressed at high levels in intestinal CD4 T cells, which are severely depleted during infection (Brenchley and Douek, 2008; Weichseldorfer et al., 2022). HIV infection disrupts the intestinal barrier, leading to translocation of microbial products. Although antiretroviral therapy (ART) can control the viral load and CD4 T cell count, it is difficult to normalize gut dysbiosis and chronic immune activation, which affect disease progression (Marchetti et al., 2013; Zicari et al., 2019). Evidence has shown that gut damage and microbial translocation contribute to the risk of non-AIDS comorbidity and mortality (Ouyang et al., 2023). Therefore, upregulation of intestinal barrier function may be a promising strategy in people living with HIV (PLWH).
It is particularly important to explore the gut microbiota mechanisms in PLWH (Schretter, 2020). A reduction in A. muciniphila in the gut is one of the changes associated with ART-naïve and ART-treated PLWH compared with noninfected people (Rocafort et al., 2019). Furthermore, the abundance of A. muciniphila in 27 chronically HIV-1-infected patients treated with ART was similar to the abundance in noninfected people (Rocafort et al., 2019). A Phase 2b trial studying the ability of supplementation with probiotics to reduce disease-associated systemic immune activation in an immune-unresponsive phenotype of PLWH showed that 18 patients in the probiotic group had increased blood CD8 and CD4+ T cell activation compared with 10 patients in the placebo group (Rousseau et al., 2022).
A study of seven chronically simian immunodeficiency virus-infected pigtail macaques showed that ART plus probiotics/prebiotics increases the frequency and functionality of the gastrointestinal tract by upregulating the expression of APC-related genes, enhancing the reconstitution and functionality of CD4+ T cells, and reducing the fibrosis of colonic lymphoid follicles (Klatt et al., 2013). Thus, ART combined with probiotic/prebiotic symbiotic supplementation in PLWH may promote increased intestinal CD4+ T cell reconstitution and mitigate inflammatory sequelae, significantly improve disease prognosis, and provide a new perspective for the management of HIV (Klatt et al., 2013; Ortiz et al., 2016).
Herbal medicine regulates the intestinal flora, inhibits the abnormal proliferation of opportunistic pathogens, and delays the clinical progression of AIDS. 16S rRNA gene sequencing of 30 patients with post-ART immunodeficiency who were treated with Shenlingguben immune granules or Artesunate tablets plus ART showed that treated patients possessed a higher abundance of Sutteralla species and Verrucomicrobiota after treatment, which was positively correlated with enhancements in immune function and the CD4+ T cell count (Wu, 2021). In a mouse model of immunosuppression established by cyclophosphamide, the administration of dandelion and Codonopsis significantly improved the immune organ index, immunoglobulin levels, and the white blood cell count, possibly in association with the abundance of Bifidobacterium and Lactobacillus and an increase in intestinal flora diversity (Gu et al., 2019). The relationship between A. muciniphila abundance and herbal medicine in PLWH requires further elucidation.
4.3 A. muciniphila, herbal medicine, and cancer immunotherapy
Cancer immunotherapy is an innovative treatment and its effectiveness depends on the activity of the host immune system. The intestinal microbiota plays an important role in immune regulation, the immune response, and tumor immunity by affecting the tumor microenvironment. Disturbance of the intestinal microbiota promotes tumor formation. In addition, the microbiota may play an important role in ameliorating tumorigenesis (Janney et al., 2020; Wang et al., 2020; Matson et al., 2021; Schmitt and Greten, 2021). The intestinal microbiota is closely associated with various immune cells. Some bacteria, such as A. muciniphila, Clostridiales, and Ruminococcaceae, have been shown to prevent systemic immune suppression by strengthening intestinal barrier integrity and systematically reducing inflammation. Furthermore, the microbiome governs the gut ecosystem to circumvent primary resistance to immune checkpoint inhibitors (Routy et al., 2018).
One study investigated whether the gut microbiome affects the response to anti-PD-1 immunotherapy in patients with hepatocellular carcinoma using metagenomic sequencing. The results showed that the intestinal microbiome, specifically A. muciniphila and Ruminococcaceae spp., can improve the efficacy of PD-1 by enhancing immune metabolism during the treatment of hepatocellular carcinoma. In addition, changes in the abundance of the gut microbiome may provide an early prediction of the outcome of immunotherapy in hepatocellular carcinoma (Zheng et al., 2019), and the long-term survival prospects (Kharofa et al., 2023). Analysis of the fecal metagenome in long-term survivors of pancreas adenocarcinoma showed that patients cured of this cancer had a greater abundance of A. muciniphila, compared with patients who completed pancreatectomy and chemotherapy (Kharofa et al., 2023). Amuc_2172, a newly discovered antitumor component of A. muciniphila, inhibits the viability of cells by promoting the cytotoxic T lymphocyte-related immune response (Jiang et al., 2023). A subcutaneous melanoma and colorectal tumor-bearing mouse model showed that IL-2 combined with the oral administration of A. muciniphila strengthens antitumor immune surveillance by activating the Toll-like receptor 2 signaling pathway (Shi et al., 2020a). These findings propose that A. muciniphila in cancer treatment, is a novel therapeutic strategy with prospecting application for cancer treatment in clinical practice.
The active ingredients of herb medicine or compound interact with intestinal flora on target organs to enhance immunity against tumors. A recent attempt to evaluate the effects of sini Decoction(SND) and gut microbes on colorectal cancer revealed that SND upregulates the expression of CD8+ T lymphocytes, increases the relative contents of beneficial bacteria (including A. muciniphila), and ameliorates the degree of malignancy of the tumor, which demonstrates that SND changes the intestinal microbiota composition in mice (Wang et al., 2021). Huoxue Yiqi Recipe-2 is a classic herbal medicine prescription described in the “Synopsis of Prescriptions of the Golden Chamber”, which increases the abundance of A. muciniphila and may therefore enhance the therapeutic effect of PD-L1 (Teng et al., 2020). Wenzi Jiedu Recipe (WJR) has been proven to be clinically useful in the treatment of colorectal cancer. The 16S rDNA sequencing method was used to analyze the changes of gut microbes revealed that WJR significantly enriched for Oscillibacter and Bacteroides_acidifacien in tumor-bearing mice with colorectal cancer. Meanwhile, WJR significantly increased the proportion of CD8+ T cells and the expression of immune-associated cytokines IL-10, IFN-γ, and TNF-α (Qiu et al., 2022). The above studies suggest that traditional Chinese medicine can improve tumor immunotherapy by adjusting the intestinal microecological structure. It is anticipated that clinical outcomes for patients with cancer will improve in the near future with the introduction of cancer immunotherapy combined with A. muciniphila.
Recently, medical researchers have proved that herb medicine can enrich intestinal bacteria and enhance immunity. However, the relationship between intestinal flora and TCM syndrome type in immunotherapy needs further research.
4.4 A. muciniphila, herbal medicine, and immune-related liver injury
The liver is a key, frontline immune tissue that maintains the homeostasis of the systemic immune response and overall tissue health (Li et al., 2015). Immune-related liver injury(IRLI) is mediated by the immune response and characterized by inflammatory cell infiltration, inflammatory granuloma formation, and damage to the structure of the hepatocyte cords (Nakano et al., 2010). IRLI is an important factor in the development of liver fibrosis, cirrhosis, and even liver tumors, and determines the outcome of the disease (Zhan and An, 2010). Various lines of evidence have linked gut microbiota dysbiosis with barrier autoimmunity and beyond, especially in the setting of immune-related liver injury (Abe et al., 2018; Wei et al., 2020). The gut microbiota and harmful substances initiate the downstream immune signal of liver cells through toll-like receptor 4 and other pattern recognition receptors, directly cause an inflammatory response, thereby aggravating inflammation-induced liver injury (Hritz et al., 2008).
Accumulating evidence indicates that the severity of immune-mediated liver injury is related to the microbiome. Dysbiosis is the cause of the development of immune hepatitis. Klebsiella and Enterococcus are up-regulated and bifidobacteria, ruminococcus and Lactobacillus are down-regulated in patients with immune hepatitis, which leads to a decrease in the ratio of bifidobacteria to Enterococci (Liu et al., 2022). An animal model of acute liver injury was induced by the injection of concanavalin A into the tail vein. Administration of A. muciniphila decreased hepatocellular apoptosis and the concentrations of pro-inflammatory cytokines and chemokines. This may be because the decreased concentrations of Fas, DR 5, and CB1 receptor enhanced the expression of Bcl-2, occludin, and Tjp-1 (Wu et al., 2017). Amuc_1100 (Amuc) may exert an immunomodulatory function by upregulating the mRNA levels of Nlrp3 and Asc in the liver of S. typhimurium-infected mice (Shi et al., 2020b; Song et al., 2023)
In concanavalin A-treated mice, the administration of zhenqiyigan decoction results in decreased serum concentrations of ALT, AST, and superoxide dismutase, increased expression levels of Fas, FasL, Bax, and PCNA, and regulates the balance of Thl/Th2, indicating that zhenqiyigan decoction plays a protective role in promoting the apoptosis of damaged hepatocytes and stimulating hepatocyte regeneration (Xiaoli, 2016).A study found that geniposide and chlorogenic acid, the active ingredients of Qushi Huayu decoction, can reduce the expression of genes required for lipid synthesis in the liver of rats with non-alcoholic fatty liver disease, and reduce serum LPS levels in rats. This may be because the increased abundance of Bacteroides and Clostridium induces Treg cell production that inhibits intestinal inflammation and improves intestinal barrier function in rats (Feng et al., 2017).
Research on the regulation of gut microbiota dysbiosis in IRLI by herb medicine is well underway, which will provide a novel direction for targeting the gut microbiota to explore potential therapeutic strategies for IRLI. However, more studies are still at the efficacy observation stage and fail to address the in-depth mechanism.
5 Mechanisms of action of A. muciniphila and herbal medicine in immune-related diseases
A large body of experimental and clinical evidence has accumulated on the use of gut microbiota strategies to improve health. Several models have been proposed based on the available evidence for the mechanisms underlying the effects of A. muciniphila in the treatment of IRDs (Figure 1).
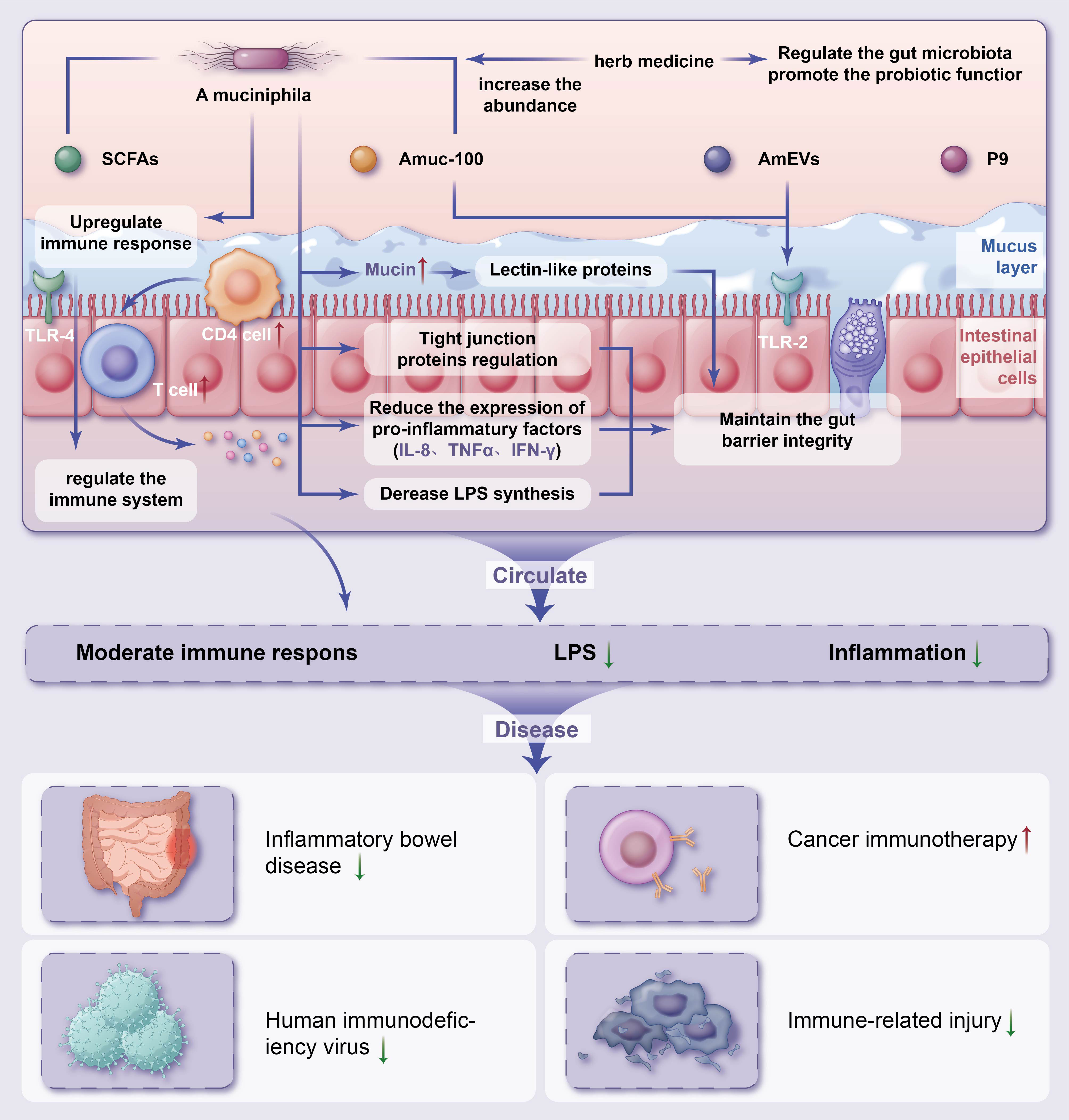
Figure 1 Potential mechanisms of herb medicine and action of Akkermansia muciniphila in immune-related diseasesThe potential mechanisms of herb medicine and action of A. muciniphila in controlling IRDs are (1) regulate the gut microbiota promote the probiotic functor and reduce chronic inflammation (2); intestinal homeostasis are associated with increased numbers of goblet cells, enhanced mucus barrier (3); A. muciniphila derived extracellular vesicles (AmEVs), a bilayer structure composed of lipid, protein, lipopolysaccharides as well as other molecules, and Amuc_1100 also regulate intestinal barrier permeability by altering tight junction protein expression through activating TLR2 pathway (4); maintenance of gut barrier integrity through the reduction of LPS synthesis and reduce the express of pro-inflammatory factors (5); increase the differentiation of Tregs in CD4+ T cell population, reduce chronic inflammation and upregulate TLR4-mediated immune response, and regulate the immune system. IRDs, immune-related diseases; SCFAs, short-chain fatty acids; LPS, lipopolysaccharide; IL, interleukin; TNF, tumor necrosis factor; IFN, interferon; TLR, Toll-like receptor.
5.1 Maintenance of the intestinal barrier
The gut microbiota exerts a number of functions including preventing epithelial damage, maintaining the integrity of the intestinal barrier, and preventing pathogens from invading the mucosal tissues. The intestinal mucous layer plays an important role in protecting against mechanical, chemical, and biological attack, and contributes to maintaining a steady state (Cornick et al., 2015; Etienne-Mesmin et al., 2019). The mucus layer can directly attach to lectin-like proteins by immune cells as a result of the glycan immune effect. Mucus is part of the innate intestinal mucosal barrier that reduces the exposure of antigens and bacteria to the intestinal epithelial cell-based immune system, and its protective effect is also due to its synergistic action with the immune system to serve as the first line of immune defense against potentially harmful compounds (Pelaseyed et al., 2014; Corfield, 2015; Johansson and Hansson, 2016). The gut microbiota is important in the formation and regulation of the intestinal mucus layer. The mucosal surface is enriched in A. muciniphila, which protect against pathogen adhesion by increasing mucus production and occupying available binding sites on the mucins, thus preventing pathogen invasion (Kim and Ho, 2010; Donaldson et al., 2016). Probiotics might also affect the mucus barrier. A. muciniphila is a key bacterium that modifies the mucus layer to communicate with host cells and stimulates the production of mucus (Everard et al., 2013; Shin et al., 2014; Wu et al., 2017; Alvarado et al., 2019; Bárcena et al., 2019; van der Lugt et al., 2019). A muciniphila expresses specific outer membrane proteins, thus potentially strengthening the intestinal barrier (Paone and Cani, 2020). A. muciniphila-derived extracellular vesicles influence gut permeability through the regulation of tight junctions (Chelakkot et al., 2018). A recent study revealed that after gavage of A. muciniphila into ApcMin/+ mice, the thickness of the intestinal mucus layer returned to normal, accompanied by an increase in the number of A. muciniphila in the mucus layer and goblet cells, which might be related to changes in the intestinal immune system, permeability, and microbial metabolites caused by A. muciniphila colonization (Dingemanse et al., 2015). Similarly, a study found that supplementation with A. muciniphila improved glucose sensitivity, inflammation, antioxidant capacity, and intestinal barrier function in mice (Ma et al., 2023). Herbal medicine has been shown to be involved in the repair of epithelial barrier integrity by upregulating the expression of the tight junction protein zonula occludens protein 1 (ZO-1) and occludin contents (Alharris et al., 2022). This implies that A. muciniphila may exert a beneficial regulatory role in host immune function by protecting the intestinal barrier. Herbal medicine can increase the thickness of the mucus layer, the expression of tight junction proteins, and the population of A. muciniphila in the intestine. Meanwhile, supplementation with herbal medicine reduced endotoxemia and the expression of various pro-inflammatory factors in mice (Zhu et al., 2018). Therefore, it is reasonable to believe that there is a crucial link between A. muciniphila and herbal medicine that maintains the integrity of the intestinal barrier.
5.2 Adjusted microbiome
Disorders of the gut microbiota increase intestinal permeability and lead to systemic inflammation by activating immunity, ultimately causing disease. Oral administration of A. muciniphila significantly decreased inflammatory cell infiltration, the mRNA expression of inflammatory factors, and improved dysbiosis of the intestinal flora in mice (Mulhall et al., 2022; Zhang et al., 2022a). Analysis of 16S rRNA amplicon sequences showed that Akkermansia induced significant intestinal microbiota alterations, including the increased abundance of Akkermansia, Muribaculaceae, and Parabacterbides goldsteinii, and the decreased abundance of Escherichia_Shigella and Enterobacteriaceae (Xu et al., 2023). Proteomic and metabolomic analyses revealed that A. muciniphila activates glucose and lipid metabolism in gut epithelial cells, leading to an increase in ATP production (Song et al., 2021). Herbal medicine acts through a variety of immunomodulatory pathways to suppress inflammation, including modulating microbial composition and reducing intestinal inflammation and permeability (Ma et al., 2018; Chhabra et al., 2021; Alharris et al., 2022; Medina-Larqué et al., 2022; Lu et al., 2023). Andrographolide prevents type 2 diabetes by modifying the gut microbiota composition, elevating the Bacteroidetes/Firmicutes ratio, enriching microbial species such as A. muciniphila, and increasing the short-chain fatty acid concentration (Su et al., 2020). Ootheca mantidis, a commonly prescribed herbal medicine for chronic kidney disease, mitigates renal fibrosis in mice by modulating glutamine metabolism, remodeling the gut microbiota, increasing the levels of some probiotics (including A. muciniphila), and downregulating apoptosis and inflammation-associated pathways (Wang et al., 2023). Dysbiosis of the gut microbiome and related metabolites has been intimately associated with disease. In a 5/6 nephrectomized rat model, 16S rRNA sequencing and untargeted metabolomic analysis showed a marked decline in microbial diversity and richness, accompanied by significant changes in 291 serum metabolites, which were mediated by altered enzymatic activities and dysregulation of lipids, amino acids, bile acids, and polyamine metabolism. Administration of poricoic acid A and Poria cocos ameliorated microbial dysbiosis and lowered the serum levels of microbial-derived products, including glycine-conjugated compounds and polyamine metabolites (Feng et al., 2019). Sodium houttuyfonate (SH), a derivative of the medicinal herb Houttuynia cordata Thunb, could maintain gut microbiota homeostasis, thereby improving intestinal function. Administration with SH weaken the oxidative stress and inflammatory response and enhance the intestinal mucosal integrity in mice model. 16S rRNA gene sequencing results showed that SH regulate the abundance and diversity of microbiota with an increase of beneficial bacteria, including SCFAs producing bacteria and probiotics (Cheng et al., 2023). Taken together, research shows that herbal medicine might have beneficial biological effects on A. muciniphila, which plays an important therapeutic role by regulating the population, distribution, and metabolites of intestinal microbiota to promote its probiotic function.
5.3 Enhancement of immune function
A. muciniphila strains increase the differentiation of Tregs from CD4+ T cell populations and alleviate chronic inflammation by reducing the concentrations of IL-8, TNF-α, and IFN-γ through the enhancement of anti-inflammatory Tregs (Zhai et al., 2019). Toll-like receptor 4 functions as a sensor mediating the crosstalk between the intestinal commensal microbiome and host immunity. A study exploring the relationship between Toll-like receptor 4 and intestinal microbial ecology found that A. muciniphila ameliorated colitis by upregulating the immune responses mediated by RORγt+ Treg cells (Liu et al., 2022). Butyrate serves as an energy source for intestinal epithelial cells and has anti-inflammatory effects. A. muciniphila is beneficial in maintaining the homeostasis of the intestinal microbiome by producing butyrate, thus promoting anti-inflammatory immune functions (Huang et al., 2022). A study found that the therapeutic outcome of IL-2 was significantly potentiated when A. muciniphila was increased in mice with subcutaneous melanomas and colorectal tumors. Mechanistically, Amuc, derived from the outer membrane protein of A. muciniphila, activates the Toll-like receptor 2 signaling pathway, which is conducive to the antitumor immune response (Shi et al., 2020a). Although research shows that A. muciniphila regulates the immune system, the mechanisms underlying the effects of A. muciniphila on the regulation of anti-inflammatory immune functions require further investigation.
Herbal medicines can improve the body’s immune response by indirectly exerting an immune regulation effect or directly acting on the intestinal epithelial cells (Xu et al., 2015). Yi-Yi-Fu-Zi-Bai-Jiang-San (YYFZBJS) is a classical prescription that can regulate T lymphocytes and improve immunity. Diversity analysis of fecal samples demonstrated that YYFZBJS regulated animal’s natural gut flora, including Bacteroides fragilis, Lachnospiraceae and so on. Intestinal lymphatic, and mesenteric lymph nodes, accumulated CD4+ CD25+ Foxp3 positive Treg cells were reduced by YYFZBJS in Apc Min/+ mice. In conclusion, YYFZBJS regulate inflammation expression by the gut microbiota mediated immune cells and increased immune function (Sui et al., 2020). GeGen QinLian decoction (GQD), a Chinese herb formula. 16S rRNA sequencing revealed that GQD can restore the intestinal flora, resulting in an increase in A. muciniphila, Desulfovibrio_C21_c20 and Lactobacillus_salivarius, and a decrease in Escherichia_coli. GQD inhibited the NOD/RIP2/NF-κB signaling pathway in the intestine and affected the expression of downstream related inflammatory cytokines in mesenteric lymph nodes and serum in mouse. In addition, GQD treatment showed systemic protection by restraining the inflammatory differentiation of CD4 + T cells. In conclusion, GQD can affect systemic immunity by restore intestinal flora (Deng et al., 2021).
In summary, herb medicinal can positively regulate the intestinal microorganisms, promotes probiotic colonization, inhibit the growth of pathogenic microorganisms, influence the differentiation and apoptosis of intestinal cells, thereby improve the intestinal barrier function and immune function. However, the intestinal microbiota is a dynamically changing and complex population. In-depth research is needed in the study of herbal medicines acting on the gut flora to modulate the immune system for the treatment of IRDs.
6 Conclusions and perspectives
When the intestinal barrier is destroyed, the permeability of intestinal mucosa increases, bacterial translocation causes systemic inflammatory response, while the occurrence and development of IRDs are mostly closely related to the inflammatory response of the body. A. muciniphila plays an important role in protecting the intestinal barrier. It is crucial to understand the link between A. muciniphila and IRDs. The abundance of A. muciniphila may be reduced by the interaction between the organism and the gut microbiota when the host’s immune function is suppressed. A. muciniphila has a complex relationship with the immune responses of the host and is a potential therapeutic target for IRDs linked to the microbiome. A. muciniphila also exerts probiotic properties and has been used in therapeutic interventions with satisfactory results. The mechanism of action of herbal medicine in treating IRDs is associated with repairing the intestinal barrier, regulating the intestinal microbiota and its metabolites, or regulating the immune response to alleviate disease. Using herb medicine regulating the gut microbiota to maintain it in a relatively stable state has great potential and clinical research value in the treatment of IRDs. However, several key issues remain unresolved. Most of the mechanistic studies on the related effect of A. muciniphila and herb medicine have been performed using animal models. Given the differences in the genetics and external environment between animal models and humans, the mechanisms of action of A. muciniphila in humans are yet to be proven. There is an urgent need for more animal trials combined with clinical studies to further elucidate the mechanistic basis for the effects of A. muciniphila in the treatment of IRDs and to develop novel therapeutic targets. Future studies should focus on how the active components of herbal medicine are metabolized by intestinal flora, and whether these metabolites have synergistic or antagonistic effects on the treatment of autoimmune diseases, this will enable the discovery of new beneficial metabolites of the intestinal flora, which will provide a new direction for clinical drug treatment of IRDs.
Author contributions
XD: Writing – original draft. P-FM: Conceptualization, Writing – review & editing. X-XM: Conceptualization, Writing – review & editing. J-YY: Conceptualization, Writing – review & editing. L-PL: Conceptualization, Writing – review & editing. L-RX: Writing – review & editing.
Funding
The author(s) declare financial support was received for the research, authorship, and/or publication of this article. This work was supported by Henan Province COVID-19 Traditional Chinese Medicine Scientific Research Special Project (2022ZYFY02), Henan Province Special Project of Traditional Chinese Medicine Scientific Research (2019AZB006, 2019JDZX2096, 2022JDZX154), and the Science and Technology Research Project of Henan Province (222102310570).
Conflict of interest
The authors declare that the research was conducted in the absence of any commercial or financial relationships that could be construed as a potential conflict of interest.
Publisher’s note
All claims expressed in this article are solely those of the authors and do not necessarily represent those of their affiliated organizations, or those of the publisher, the editors and the reviewers. Any product that may be evaluated in this article, or claim that may be made by its manufacturer, is not guaranteed or endorsed by the publisher.
References
Abe K., Takahashi A., Fujita M., Imaizumi H., Hayashi M., Okai K., et al. (2018). Dysbiosis of oral microbiota and its association with salivary immunological biomarkers in autoimmune liver disease. PloS One 13 (7), e0198757. doi: 10.1371/journal.pone.0198757
Alharris E., Mohammed A., Alghetaa H., Zhou J., Nagarkatti M., Nagarkatti P. (2022). The ability of resveratrol to attenuate ovalbumin-mediated allergic asthma is associated with changes in microbiota involving the gut-lung axis, enhanced barrier function and decreased inflammation in the lungs. Front. Immunol. 13, 805770. doi: 10.3389/fimmu.2022.805770
Alvarado D. M., Chen B., Iticovici M., Thaker A. I., Dai N., VanDussen K. L., et al. (2019). Epithelial indoleamine 2,3-dioxygenase 1 modulates aryl hydrocarbon receptor and notch signaling to increase differentiation of secretory cells and alter mucus-associated microbiota. Gastroenterology 157 (4), 1093–108.e11. doi: 10.1053/j.gastro.2019.07.013
Ansaldo E., Slayden L. C., Ching K. L., Koch M. A., Wolf N. K., Plichta D. R., et al. (2019). Akkermansia muciniphila induces intestinal adaptive immune responses during homeostasis. Science 364 (6446), 1179–1184. doi: 10.1126/science.aaw7479
Bárcena C., Valdés-Mas R., Mayoral P., Garabaya C., Durand S., Rodríguez F., et al. (2019). Healthspan and lifespan extension by fecal microbiota transplantation into progeroid mice. Nat. Med. 25 (8), 1234–1242. doi: 10.1038/s41591-019-0504-5
Barratt M. J., Nuzhat S., Ahsan K., Frese S. A., Arzamasov A. A., Sarker S. A., et al. (2022). Bifidobacterium infantis treatment promotes weight gain in Bangladeshi infants with severe acute malnutrition. Sci. Transl. Med. 14 (640), eabk1107. doi: 10.1126/scitranslmed.abk1107
Becken B., Davey L., Middleton D. R., Mueller K. D., Sharma A., Holmes Z. C., et al. (2021). Genotypic and Phenotypic Diversity among Human Isolates of Akkermansia muciniphila. mBio 12 (3). doi: 10.1128/mBio.00478-21
Bi M., Liu C., Wang Y., Liu S. J. (2023). Therapeutic prospect of new probiotics in neurodegenerative diseases. Microorganisms 11 (6). doi: 10.3390/microorganisms11061527
Bian X., Wu W., Yang L., Lv L., Wang Q., Li Y., et al. (2019). Administration of akkermansia muciniphila ameliorates dextran sulfate sodium-induced ulcerative colitis in mice. Front. Microbiol. 10, 2259. doi: 10.3389/fmicb.2019.02259
Bibbò S., Dore M. P., Pes G. M., Delitala G., Delitala A. P. (2017). Is there a role for gut microbiota in type 1 diabetes pathogenesis? Ann. Med. 49 (1), 11–22. doi: 10.1080/07853890
Brenchley J. M., Douek D. C. (2008). HIV infection and the gastrointestinal immune system. Mucosal Immunol. 1 (1), 23–30. doi: 10.1038/mi.2007.1
Brodin P. (2022). Immune-microbe interactions early in life: A determinant of health and disease long term. Science 376 (6596), 945–950. doi: 10.1126/science.abk2189
Cai Y., Li X., Han Q., Bai J., Zheng Q., Sun R., et al. (2023). Si-Ni-San improves experimental colitis by favoring Akkermensia colonization. J. Ethnopharmacol. 305, 116067. doi: 10.1016/j.jep.2022.116067
Cani P. D. (2018). Human gut microbiome: hopes, threats and promises. Gut 67 (9), 1716–1725. doi: 10.1136/gutjnl-2018-316723
Cani P. D., Depommier C., Derrien M., Everard A., de Vos W. M. (2022). Akkermansia muciniphila: paradigm for next-generation beneficial microorganisms. Nat. Rev. Gastroenterol. Hepatol. 19 (10), 625–637. doi: 10.1038/s41575-022-00631-9
Cerro E. D., Lambea M., Félix J., Salazar N., Gueimonde M., de la Fuente M. (2022). Daily ingestion of Akkermansia mucciniphila for one month promotes healthy aging and increases lifespan in old female mice. Biogerontology 23 (1), 35–52. doi: 10.1007/s10522-021-09943-w
Chelakkot C., Choi Y., Kim D. K., Park H. T., Ghim J., Kwon Y., et al. (2018). Akkermansia muciniphila-derived extracellular vesicles influence gut permeability through the regulation of tight junctions. Exp. Mol. Med. 50 (2), e450. doi: 10.1038/emm.2017.282
Chen Z., Qian X., Chen S., Fu X., Ma G., Zhang A. (2020). Akkermansia muciniphila enhances the antitumor effect of cisplatin in lewis lung cancer mice. J. Immunol. Res. 2020, 2969287. doi: 10.1155/2020/2969287
Chen Y., Xiao S., Gong Z., Zhu X., Yang Q., Li Y., et al. (2017). Wuji wan formula ameliorates diarrhea and disordered colonic motility in post-inflammation irritable bowel syndrome rats by modulating the gut microbiota. Front. Microbiol. 8, 2307. doi: 10.3389/fmicb.2017.02307
Cheng T., Xu C., Wu D., Yan G., Wang C., Wang T., et al. (2023). Sodium houttuyfonate derived from Houttuynia cordata Thunb improves intestinal malfunction via maintaining gut microflora stability in Candida albicans overgrowth aggravated ulcerative colitis. Food Funct. 14 (2), 1072–1086. doi: 10.1039/D2FO02369E
Chhabra G., Singh C. K., Amiri D., Akula N., Ahmad N. (2021). Recent advancements on immunomodulatory mechanisms of resveratrol in tumor microenvironment. Molecules 26 (5). doi: 10.3390/molecules26051343
Corfield A. P. (2015). Mucins: a biologically relevant glycan barrier in mucosal protection. Biochim. Biophys. Acta 1850 (1), 236–252. doi: 10.1016/j.bbagen.2014.05.003
Cornick S., Tawiah A., Chadee K. (2015). Roles and regulation of the mucus barrier in the gut. Tissue Barriers 3 (1-2), e982426. doi: 10.4161/21688370.2014.982426
Demirci M., Tokman H. B., Uysal H. K., Demiryas S., Karakullukcu A., Saribas S., et al. (2019). Reduced Akkermansia muciniphila and Faecalibacterium prausnitzii levels in the gut microbiota of children with allergic asthma. Allergol Immunopathol. (Madr). 47 (4), 365–371. doi: 10.1016/j.aller.2018.12.009
Deng L., Shi Y., Liu P., Wu S., Lv Y., Xu H., et al. (2021). GeGen QinLian decoction alleviate influenza virus infectious pneumonia through intestinal flora. BioMed. Pharmacother. 141, 111896. doi: 10.1016/j.biopha.2021.111896
Depommier C., Everard A., Druart C., Plovier H., Van Hul M., Vieira-Silva S., et al. (2019). Supplementation with Akkermansia muciniphila in overweight and obese human volunteers: a proof-of-concept exploratory study. Nat. Med. 25 (7), 1096–1103. doi: 10.1038/s41591-019-0495-2
Derosa L., Routy B., Thomas A. M., Iebba V., Zalcman G., Friard S., et al. (2022). Intestinal Akkermansia muciniphila predicts clinical response to PD-1 blockade in patients with advanced non-small-cell lung cancer. Nat. Med. 28 (2), 315–324. doi: 10.1038/s41591-021-01655-5
Derrien M., van Passel M. W., van de Bovenkamp J. H., Schipper R. G., de Vos W. M., Dekker J. (2010). Mucin-bacterial interactions in the human oral cavity and digestive tract. Gut Microbes 1 (4), 254–268. doi: 10.4161/gmic.1.4.12778
Dingemanse C., Belzer C., van Hijum S. A., Günthel M., Salvatori D., den Dunnen J. T., et al. (2015). Akkermansia muciniphila and Helicobacter typhlonius modulate intestinal tumor development in mice. Carcinogenesis 36 (11), 1388–1396. doi: 10.1093/carcin/bgv120
Donaldson G. P., Lee S. M., Mazmanian S. K. (2016). Gut biogeography of the bacterial microbiota. Nat. Rev. Microbiol. 14 (1), 20–32. doi: 10.1038/nrmicro3552
Etienne-Mesmin L., Chassaing B., Desvaux M., De Paepe K., Gresse R., Sauvaitre T., et al. (2019). Experimental models to study intestinal microbes-mucus interactions in health and disease. FEMS Microbiol. Rev. 43 (5), 457–489. doi: 10.1093/femsre/fuz013
Everard A., Belzer C., Geurts L., Ouwerkerk J. P., Druart C., Bindels L. B., et al. (2013). Cross-talk between Akkermansia muciniphila and intestinal epithelium controls diet-induced obesity. Proc. Natl. Acad. Sci. U S A. 110 (22), 9066–9071. doi: 10.1073/pnas.1219451110
Feng W., Ao H., Peng C., Yan D. (2019). Gut microbiota, a new frontier to understand traditional Chinese medicines. Pharmacol. Res. 142, 176–191. doi: 10.1016/j.phrs.2019.02.024
Feng Y. L., Cao G., Chen D. Q., Vaziri N. D., Chen L., Zhang J., et al. (2019). Microbiome-metabolomics reveals gut microbiota associated with glycine-conjugated metabolites and polyamine metabolism in chronic kidney disease. Cell Mol. Life Sci. 76 (24), 4961–4978. doi: 10.1007/s00018-019-03155-9
Feng Q., Liu W., Baker S. S., Li H., Chen C., Liu Q., et al. (2017). Multi-targeting therapeutic mechanisms of the Chinese herbal medicine QHD in the treatment of non-alcoholic fatty liver disease. Oncotarget 8 (17), 27820–27838. doi: 10.18632/oncotarget.15482
Filardi R., Gargari G., Mora D., Arioli S. (2022). Characterization of antibiotic-resistance traits in Akkermansia muciniphila strains of human origin. Sci. Rep. 12 (1), 19426. doi: 10.1038/s41598-022-23980-6
Ghaffari S., Abbasi A., Somi M. H., Moaddab S. Y., Nikniaz L., Kafil H. S., et al. (2022). Akkermansia muciniphila: from its critical role in human health to strategies for promoting its abundance in human gut microbiome. Crit. Rev. Food Sci. Nutr. 63 (25), 7357–7377. doi: 10.1080/10408398.2022.2045894
Ghotaslou R., Nabizadeh E., Memar M. Y., Law W. M. H., Ozma M. A., Abdi M., et al. (2023). The metabolic, protective, and immune functions of Akkermansia muciniphila. Microbiol. Res. 266, 127245. doi: 10.1016/j.micres.2022.127245
Gu Z., Pei W., Shen Y., Wang L., Zhu J., Zhang Y., et al. (2021). Akkermansia muciniphila and its outer protein Amuc_1100 regulates tryptophan metabolism in colitis. Food Funct. 12 (20), 10184–10195. doi: 10.1039/D1FO02172A
Gu W., Sun M., Wang L., Chen Z., Zeng J., Chen J., et al. (2019). Effects of four kinds of traditional chinese medicine on immune performance and intestinal flora in immunosuppressed mice. Chian Anim. Husbandry&Veterinary Med. 46 (01), 147–156.
Guo M., Lu M., Chen K., Xu R., Xia Y., Liu X., et al. (2023). Akkermansia muciniphila and Lactobacillus plantarum ameliorate systemic lupus erythematosus by possibly regulating immune response and remodeling gut microbiota. mSphere 8 (4), e0007023. doi: 10.1128/msphere.00070-23
Hong R. P., Hou Y. Y., Xu X. J., Lang J. D., Jin Y. F., Zeng X. F., et al. (2022). The difference of gut microbiota and their correlations with urinary organic acids between autistic children with and without atopic dermatitis. Front. Cell Infect. Microbiol. 12, 886196. doi: 10.3389/fcimb.2022.886196
Hritz I., Mandrekar P., Velayudham A., Catalano D., Dolganiuc A., Kodys K., et al. (2008). The critical role of toll-like receptor (TLR) 4 in alcoholic liver disease is independent of the common TLR adapter MyD88. Hepatology 48 (4), 1224–1231. doi: 10.1002/hep.22470
Huang C., Sun Y., Liao S. R., Chen Z. X., Lin H. F., Shen W. Z. (2022). Suppression of Berberine and Probiotics (in vitro and in vivo) on the Growth of Colon Cancer With Modulation of Gut Microbiota and Butyrate Production. Front. Microbiol. 13, 869931. doi: 10.3389/fmicb.2022.869931
Isnard S., Lin J., Fombuena B., Ouyang J., Varin T. V., Richard C., et al. (2020). Repurposing metformin in nondiabetic people with HIV: influence on weight and gut microbiota. Open Forum Infect. Dis. 7 (9), ofaa338. doi: 10.1093/ofid/ofaa338
Janney A., Powrie F., Mann E. H. (2020). Host-microbiota maladaptation in colorectal cancer. Nature 585 (7826), 509–517. doi: 10.1038/s41586-020-2729-3
Jiang Y., Xu Y., Zheng C., Ye L., Jiang P., Malik S., et al. (2023). Acetyltransferase from Akkermansia muciniphila blunts colorectal tumourigenesis by reprogramming tumour microenvironment. Gut 72 (7), 1308–1318. doi: 10.1136/gutjnl-2022-327853
Jing Y., Li A., Liu Z., Yang P., Wei J., Chen X., et al. (2018). Absorption of codonopsis pilosula saponins by coexisting polysaccharides alleviates gut microbial dysbiosis with dextran sulfate sodium-induced colitis in model mice. BioMed. Res. Int. 2018, 1781036. doi: 10.1155/2018/1781036
Johansson M. E., Hansson G. C. (2016). Immunological aspects of intestinal mucus and mucins. Nat. Rev. Immunol. 16 (10), 639–649. doi: 10.1038/nri.2016.88
Katiraei S., de Vries M. R., Costain A. H., Thiem K., Hoving L. R., van Diepen J. A., et al. (2020). Akkermansia muciniphila Exerts Lipid-Lowering and Immunomodulatory Effects without Affecting Neointima Formation in Hyperlipidemic APOE*3-Leiden. CETP Mice. Mol. Nutr. Food Res. 64 (15), e1900732. doi: 10.1002/mnfr.201900732
Kharofa J., Haslam D., Wilkinson R., Weiss A., Patel S., Wang K., et al. (2023). Analysis of the fecal metagenome in long-term survivors of pancreas cancer. Cancer 129 (13), 1986–1994. doi: 10.1002/cncr.34748
Kim Y. S., Ho S. B. (2010). Intestinal goblet cells and mucins in health and disease: recent insights and progress. Curr. Gastroenterol. Rep. 12 (5), 319–330. doi: 10.1007/s11894-010-0131-2
Kirmiz N., Galindo K., Cross K. L., Luna E., Rhoades N., Podar M., et al. (2020). Comparative genomics guides elucidation of vitamin B(12) biosynthesis in novel human-associated akkermansia strains. Appl. Environ. Microbiol. 86 (3). doi: 10.1128/AEM.02117-19
Klatt N. R., Canary L. A., Sun X., Vinton C. L., Funderburg N. T., Morcock D. R., et al. (2013). Probiotic/prebiotic supplementation of antiretrovirals improves gastrointestinal immunity in SIV-infected macaques. J. Clin. Invest. 123 (2), 903–907. doi: 10.1172/JCI66227
Lee Y., Byeon H. R., Jang S. Y., Hong M. G., Kim D., Lee D., et al. (2022). Oral administration of Faecalibacterium prausnitzii and Akkermansia muciniphila strains from humans improves atopic dermatitis symptoms in DNCB induced NC/Nga mice. Sci. Rep. 12 (1), 7324. doi: 10.1038/s41598-022-11048-4
Li S., Tan H. Y., Wang N., Zhang Z. J., Lao L., Wong C. W., et al. (2015). The role of oxidative stress and antioxidants in liver diseases. Int. J. Mol. Sci. 16 (11), 26087–26124. doi: 10.3390/ijms161125942
Li J., Xia N., Wen S., Li D., Lu Y., Gu M., et al. (2019). IL (Interleukin)-33 suppresses abdominal aortic aneurysm by enhancing regulatory T-cell expansion and activity. Arterioscler. Thromb. Vasc. Biol. 39 (3), 446–458. doi: 10.1161/ATVBAHA.118.312023
Liu Q., He W., Tang R., Ma X. (2022). Intestinal homeostasis in autoimmune liver diseases. Chin. Med. J. (Engl) 135 (14), 1642–1652. doi: 10.1097/CM9.0000000000002291
Liu Y., Yang M., Tang L., Wang F., Huang S., Liu S., et al. (2022). TLR4 regulates RORγt(+) regulatory T-cell responses and susceptibility to colon inflammation through interaction with Akkermansia muciniphila. Microbiome 10 (1), 98. doi: 10.1186/s40168-022-01296-x
Lu J., Gong Y., Gao Y., Yang Y., Zhang Y., Zhang Z., et al. (2023). Wolfberry, Yam, and Chrysanthemum polysaccharides increased intestinal Akkermansia muciniphila abundance and hepatic YAP1 expression to alleviate DILI. FASEB J. 37 (12), e23286. doi: 10.1096/fj.202301388R
Luna E., Parkar S. G., Kirmiz N., Hartel S., Hearn E., Hossine M., et al. (2022). Utilization efficiency of human milk oligosaccharides by human-associated akkermansia is strain dependent. Appl. Environ. Microbiol. 88 (1), e0148721. doi: 10.1128/AEM.01487-21
Ma J., Liu Z., Gao X., Bao Y., Hong Y., He X., et al. (2023). Gut microbiota remodeling improves natural aging-related disorders through Akkermansia muciniphila and its derived acetic acid. Pharmacol. Res. 189, 106687. doi: 10.1016/j.phrs.2023.106687
Ma D. S. L., Tan L. T., Chan K. G., Yap W. H., Pusparajah P., Chuah L. H., et al. (2018). Resveratrol-potential antibacterial agent against foodborne pathogens. Front. Pharmacol. 9, 102. doi: 10.3389/fphar.2018.00102
MaChado D., Barbosa J. C., Almeida D., Andrade J. C., Freitas A. C., Gomes A. M. (2022). Insights into the Antimicrobial Resistance Profile of a Next Generation Probiotic Akkermansia muciniphila DSM 22959. Int. J. Environ. Res. Public Health 19 (15). doi: 10.3390/ijerph19159152
Marchesi J. R., Adams D. H., Fava F., Hermes G. D., Hirschfield G. M., Hold G., et al. (2016). The gut microbiota and host health: a new clinical frontier. Gut 65 (2), 330–339. doi: 10.1136/gutjnl-2015-309990
Marchetti G., Tincati C., Silvestri G. (2013). Microbial translocation in the pathogenesis of HIV infection and AIDS. Clin. Microbiol. Rev. 26 (1), 2–18. doi: 10.1128/CMR.00050-12
Matson V., Chervin C. S., Gajewski T. F. (2021). Cancer and the microbiome-influence of the commensal microbiota on cancer, immune responses, and immunotherapy. Gastroenterology 160 (2), 600–613. doi: 10.1053/j.gastro.2020.11.041
Medina-Larqué A. S., Rodríguez-Daza M. C., Roquim M., Dudonné S., Pilon G., Levy É, et al. (2022). Cranberry polyphenols and agave agavins impact gut immune response and microbiota composition while improving gut barrier function, inflammation, and glucose metabolism in mice fed an obesogenic diet. Front. Immunol. 13, 871080. doi: 10.3389/fimmu.2022.871080
Michalovich D., Rodriguez-Perez N., Smolinska S., Pirozynski M., Mayhew D., Uddin S., et al. (2019). Obesity and disease severity magnify disturbed microbiome-immune interactions in asthma patients. Nat. Commun. 10 (1), 5711. doi: 10.1038/s41467-019-13751-9
Mulhall H., DiChiara J. M., Huck O., Amar S. (2022). Pasteurized Akkermansia muciniphila reduces periodontal and systemic inflammation induced by Porphyromonas gingivalis in lean and obese mice. J. Clin. Periodontol. 49 (7), 717–729. doi: 10.1111/jcpe.13629
Nakano T., Goto S., Lai C. Y., Hsu L. W., Takaoka Y., Kawamoto S., et al. (2010). Immunological aspects and therapeutic significance of an autoantibody against histone H1 in a rat model of concanavalin A-induced hepatitis. Immunology 129 (4), 547–555. doi: 10.1111/j.1365-2567.2009.03149.x
Ortiz A. M., Klase Z. A., DiNapoli S. R., Vujkovic-Cvijin I., Carmack K., Perkins M. R., et al. (2016). IL-21 and probiotic therapy improve Th17 frequencies, microbial translocation, and microbiome in ARV-treated, SIV-infected macaques. Mucosal Immunol. 9 (2), 458–467. doi: 10.1038/mi.2015.75
Ouyang J., Yan J., Zhou X., Isnard S., Harypursat V., Cui H., et al. (2023). Relevance of biomarkers indicating gut damage and microbial translocation in people living with HIV. Front. Immunol. 14, 1173956. doi: 10.3389/fimmu.2023.1173956
Pang J., Ma S., Xu X., Zhang B., Cai Q. (2021). Effects of rhizome of Atractylodes koreana (Nakai) Kitam on intestinal flora and metabolites in rats with rheumatoid arthritis. J. Ethnopharmacol. 281, 114026. doi: 10.1016/j.jep.2021.114026
Paone P., Cani P. D. (2020). Mucus barrier, mucins and gut microbiota: the expected slimy partners? Gut 69 (12), 2232–2243. doi: 10.1136/gutjnl-2020-322260
Pelaseyed T., Bergström J. H., Gustafsson J. K., Ermund A., Birchenough G. M., Schütte A., et al. (2014). The mucus and mucins of the goblet cells and enterocytes provide the first defense line of the gastrointestinal tract and interact with the immune system. Immunol. Rev. 260 (1), 8–20. doi: 10.1111/imr.12182
Plaza-Díaz J., Ruiz-Ojeda F. J., Vilchez-Padial L. M., Gil A. (2017). Evidence of the anti-inflammatory effects of probiotics and synbiotics in intestinal chronic diseases. Nutrients 9 (6). doi: 10.3390/nu9060555
Plovier H., Everard A., Druart C., Depommier C., Van Hul M., Geurts L., et al. (2017). A purified membrane protein from Akkermansia muciniphila or the pasteurized bacterium improves metabolism in obese and diabetic mice. Nat. Med. 23 (1), 107–113. doi: 10.1038/nm.4236
Qian K., Chen S., Wang J., Sheng K., Wang Y., Zhang M. (2022). A β-N-acetylhexosaminidase Amuc_2109 from Akkermansia muciniphila protects against dextran sulfate sodium-induced colitis in mice by enhancing intestinal barrier and modulating gut microbiota. Food Funct. 13 (4), 2216–2227. doi: 10.1039/D1FO04094D
Qiu W., Sang T., Chen H., Zhou H., Wang Z., Zhou H. (2022). Wenzi Jiedu Recipe ameliorates colorectal cancer by remodeling the gut microbiota and tumor microenvironment. Front. Oncol. 12, 915498. doi: 10.3389/fonc.2022.915498
Rocafort M., Noguera-Julian M., Rivera J., Pastor L., Guillén Y., Langhorst J., et al. (2019). Evolution of the gut microbiome following acute HIV-1 infection. Microbiome 7 (1), 73. doi: 10.1186/s40168-019-0687-5
Rousseau R. K., Walmsley S. L., Lee T., Rosenes R., Reinhard R. J., Malazogu F., et al. (2022). Randomized, blinded, placebo-controlled trial of de simone formulation probiotic during HIV-associated suboptimal CD4+ T cell recovery. J. Acquir. Immune Defic. Syndr. 89 (2), 199–207. doi: 10.1097/QAI.0000000000002840
Routy B., Le Chatelier E., Derosa L., Duong C. P. M., Alou M. T., Daillère R., et al. (2018). Gut microbiome influences efficacy of PD-1-based immunotherapy against epithelial tumors. Science 359 (6371), 91–97. doi: 10.1126/science.aan3706
Sanders M. E., Merenstein D. J., Reid G., Gibson G. R., Rastall R. A. (2019). Probiotics and prebiotics in intestinal health and disease: from biology to the clinic. Nat. Rev. Gastroenterol. Hepatol. 16 (10), 605–616. doi: 10.1038/s41575-019-0173-3
Scher J. U., Ubeda C., Artacho A., Attur M., Isaac S., Reddy S. M., et al. (2015). Decreased bacterial diversity characterizes the altered gut microbiota in patients with psoriatic arthritis, resembling dysbiosis in inflammatory bowel disease. Arthritis Rheumatol. 67 (1), 128–139. doi: 10.1002/art.38892
Schmitt M., Greten F. R. (2021). The inflammatory pathogenesis of colorectal cancer. Nat. Rev. Immunol. 21 (10), 653–667. doi: 10.1038/s41577-021-00534-x
Schretter C. E. (2020). Links between the gut microbiota, metabolism, and host behavior. Gut Microbes 11 (2), 245–248. doi: 10.1080/19490976.2019.1643674
Shen X., Miao J., Wan Q., Wang S., Li M., Pu F., et al. (2018). Possible correlation between gut microbiota and immunity among healthy middle-aged and elderly people in southwest China. Gut Pathog. 10, 4. doi: 10.1186/s13099-018-0231-3
Shi L., Sheng J., Chen G., Zhu P., Shi C., Li B., et al. (2020a). Combining IL-2-based immunotherapy with commensal probiotics produces enhanced antitumor immune response and tumor clearance. J. Immunother. Cancer 8 (2). doi: 10.1136/jitc-2020-000973
Shi L., Zhang S., Huang Z., Hu F., Zhang T., Wei M., et al. (2020b). Baicalin promotes liver regeneration after acetaminophen-induced liver injury by inducing NLRP3 inflammasome activation. Free Radic. Biol. Med. 160, 163–177. doi: 10.1016/j.freeradbiomed.2020.05.012
Shin N. R., Lee J. C., Lee H. Y., Kim M. S., Whon T. W., Lee M. S., et al. (2014). An increase in the Akkermansia spp. population induced by metformin treatment improves glucose homeostasis in diet-induced obese mice. Gut 63 (5), 727–735. 10.1136/gutjnl-2012-303839
Song W. S., Shin S. G., Jo S. H., Lee J. S., Jeon H. J., Kwon J. E., et al. (2021). Development of an in vitro coculture device for the investigation of host-microbe interactions via integrative multiomics approaches. Biotechnol. Bioeng. 118 (4), 1612–1623. doi: 10.1002/bit.27676
Song Z., Si X., Zhang X., Chen J., Jia H., He Y., et al. (2023). Amuc prevents liver inflammation and oxidative stress in mice challenged with salmonella typhimurium. J. Nutr. 153 (2), 532–542. doi: 10.1016/j.tjnut.2022.12.004
Sonomoto K., Song R., Eriksson D., Hahn A. M., Meng X., Lyu P., et al. (2023). High-fat-diet-associated intestinal microbiota exacerbates psoriasis-like inflammation by enhancing systemic γδ T cell IL-17 production. Cell Rep. 42 (7), 112713. doi: 10.1016/j.celrep.2023.112713
Stoll M. L., Sawhney H., Wells P. M., Sternes P. R., Reveille J. D., Morrow C. D., et al. (2023). The faecal microbiota is distinct in HLA-B27+ ankylosing spondylitis patients versus HLA-B27+ healthy controls. Clin. Exp. Rheumatol. 41 (5), 1096–1104. doi: 10.55563/clinexprheumatol/nlsj0o
Su H., Mo J., Ni J., Ke H., Bao T., Xie J., et al. (2020). Andrographolide Exerts Antihyperglycemic Effect through Strengthening Intestinal Barrier Function and Increasing Microbial Composition of Akkermansia muciniphila. Oxid. Med. Cell Longev. 2020, 6538930. doi: 10.1155/2020/6538930
Sui H., Zhang L., Gu K., Chai N., Ji Q., Zhou L., et al. (2020). YYFZBJS ameliorates colorectal cancer progression in Apc(Min/+) mice by remodeling gut microbiota and inhibiting regulatory T-cell generation. Cell Commun. Signal. 18 (1), 113. doi: 10.1186/s12964-020-00596-9
Tan L., Zhao S., Zhu W., Wu L., Li J., Shen M., et al. (2018). The Akkermansia muciniphila is a gut microbiota signature in psoriasis. Exp. Dermatol. 27 (2), 144–149. doi: 10.1111/exd.13463
Teng L., Wang K., Chen W., Wang Y. S., Bi L. (2020). HYR-2 plays an anti-lung cancer role by regulating PD-L1 and Akkermansia muciniphila. Pharmacol. Res. 160, 105086. doi: 10.1016/j.phrs.2020.105086
Teng S., Yaqi Z., Guangxi Z., Fengqin Z. (2023). Research progress of the action of shikonin in immune-related diseases. China Med. 18 (04), 629–632.
Terrisse S., Goubet A. G., Ueda K., Thomas A. M., Quiniou V., Thelemaque C., et al. (2022). Immune system and intestinal microbiota determine efficacy of androgen deprivation therapy against prostate cancer. J. Immunother. Cancer 10 (3). doi: 10.1136/jitc-2021-004191
Thaiss C. A., Zmora N., Levy M., Elinav E. (2016). The microbiome and innate immunity. Nature 535 (7610), 65–74. doi: 10.1038/nature18847
van der Lugt B., van Beek A. A., Aalvink S., Meijer B., Sovran B., Vermeij W. P., et al. (2019). Akkermansia muciniphila ameliorates the age-related decline in colonic mucus thickness and attenuates immune activation in accelerated aging Ercc1 (-/Δ7) mice. Immun. Ageing. 16, 6. doi: 10.1186/s12979-019-0145-z
Vindigni S. M., Zisman T. L., Suskind D. L., Damman C. J. (2016). The intestinal microbiome, barrier function, and immune system in inflammatory bowel disease: a tripartite pathophysiological circuit with implications for new therapeutic directions. Therap Adv. Gastroenterol. 9 (4), 606–625. doi: 10.1177/1756283X16644242
Wang J., Guo X., Zou Z., Yu M., Li X., Xu H., et al. (2023). Ootheca mantidis mitigates renal fibrosis in mice by the suppression of apoptosis via increasing the gut microbe Akkermansia muciniphila and modulating glutamine metabolism. BioMed. Pharmacother. 166, 115434. doi: 10.1016/j.biopha.2023.115434
Wang L., Tang L., Feng Y., Zhao S., Han M., Zhang C., et al. (2020). A purified membrane protein from Akkermansia muciniphila or the pasteurised bacterium blunts colitis associated tumourigenesis by modulation of CD8(+) T cells in mice. Gut 69 (11), 1988–1997. doi: 10.1136/gutjnl-2019-320105
Wang Y., Zhang X., Li J., Zhang Y., Guo Y., Chang Q., et al. (2021). Sini decoction ameliorates colorectal cancer and modulates the composition of gut microbiota in mice. Front. Pharmacol. 12, 609992. doi: 10.3389/fphar.2021.609992
Wei Y., Li Y., Yan L., Sun C., Miao Q., Wang Q., et al. (2020). Alterations of gut microbiome in autoimmune hepatitis. Gut 69 (3), 569–577. doi: 10.1136/gutjnl-2018-317836
Weichseldorfer M., Tagaya Y., Reitz M., DeVico A. L., Latinovic O. S. (2022). Identifying CCR5 coreceptor populations permissive for HIV-1 entry and productive infection: implications for in vivo studies. J. Transl. Med. 20 (1), 39. doi: 10.1186/s12967-022-03243-8
Wright E. K., Ding N. S., Niewiadomski O. (2018). Management of inflammatory bowel disease. Med. J. Aust. 209 (7), 318–323. doi: 10.5694/mja17.01001
Wu X. (2021). Clinical observation on the effect of regulating intestinal flora with traditional Chinese medicine on improving the immune reconstitution insufficiency of AIDS (China Academy of Chinese Medical Sciences).
Wu W., Lv L., Shi D., Ye J., Fang D., Guo F., et al. (2017). Protective Effect of Akkermansia muciniphila against Immune-Mediated Liver Injury in a Mouse Model. Front. Microbiol. 8, 1804. doi: 10.3389/fmicb.2017.01804
Xiaoli J. (2016). Clinical and experimental study of zhenqiyigan decoction on protective effect of acute immune-mediated liver injury (Guangzhou University of Chinese Medicine).
Xu Y., Duan J., Wang D., Liu J., Chen X., Qin X. Y., et al. (2023). Akkermansia muciniphila alleviates persistent inflammation, immunosuppression, and catabolism syndrome in mice. Metabolites 13 (2). doi: 10.3390/metabo13020194
Xu J., Lian F., Zhao L., Zhao Y., Chen X., Zhang X., et al. (2015). Structural modulation of gut microbiota during alleviation of type 2 diabetes with a Chinese herbal formula. Isme J. 9 (3), 552–562. doi: 10.1038/ismej.2014.177
Yanavich C., Perazzo H., Li F., Tobin N., Lee D., Zabih S., et al. (2022). A pilot study of microbial signatures of liver disease in those with HIV mono-infection in Rio de Janeiro, Brazil. Aids 36 (1), 49–58. doi: 10.1097/QAD.0000000000003084
Yang Y., Su W., Zang C., Tan Y., Zhang Y., Zhou Z., et al. (2023). Traditional Chinese medicines (TCMs) with varied meridians (Gui-Jing) differentially alleviate the adverse impact of Coptis chinensis on gut microbiota. J. Ethnopharmacol. 307, 116256. doi: 10.1016/j.jep.2023.116256
Yang G., Tu X., You F., He Y., Yu L., Tian W., et al. (2021). The effect of Coptis coptis on Akermansia mucophilus in the intestinal tract of mice with ulcerative colitis was analyzed by qPCR. Acta Chin. Med. Pharmacol. 49 (11), 18–23.
Yang Y., Zheng X., Wang Y., Tan X., Zou H., Feng S., et al. (2022). Human fecal microbiota transplantation reduces the susceptibility to dextran sulfate sodium-induced germ-free mouse colitis. Front. Immunol. 13, 836542. doi: 10.3389/fimmu.2022.836542
Zeng S. Y., Liu Y. F., Liu J. H., Zeng Z. L., Xie H., Liu J. H. (2023). Potential effects of akkermansia muciniphila in aging and aging-related diseases: current evidence and perspectives. Aging Dis. 14 (6), 2015–2027. doi: 10.14336/AD.2023.0325
Zhai R., Xue X., Zhang L., Yang X., Zhao L., Zhang C. (2019). Strain-specific anti-inflammatory properties of two akkermansia muciniphila strains on chronic colitis in mice. Front. Cell Infect. Microbiol. 9, 239. doi: 10.3389/fcimb.2019.00239
Zhan Y. T., An W. (2010). Roles of liver innate immune cells in nonalcoholic fatty liver disease. World J. Gastroenterol. 16 (37), 4652–4660. doi: 10.3748/wjg.v16.i37.4652
Zhang L., Liu J., Jin T., Qin N., Ren X., Xia X. (2022a). Live and pasteurized Akkermansia muciniphilaattenuate hyperuricemia in mice through modulating uric acid metabolism, inflammation, and gut microbiota. Food Funct. 13 (23), 12412–12425. doi: 10.1039/D2FO02702J
Zhang L., Wang Y., Wu F., Wang X., Feng Y., Wang Y. (2022b). MDG, an Ophiopogon japonicus polysaccharide, inhibits non-alcoholic fatty liver disease by regulating the abundance of Akkermansia muciniphila. Int. J. Biol. Macromol. 196, 23–34. doi: 10.1016/j.ijbiomac.2021.12.036
Zhang H., Zhuo S., Song D., Wang L., Gu J., Ma J., et al. (2021). Icariin inhibits intestinal inflammation of DSS-induced colitis mice through modulating intestinal flora abundance and modulating p-p65/p65 molecule. Turk J. Gastroenterol. 32 (4), 382–392. doi: 10.5152/tjg.2021.20282
Zhao Q., Yu J., Hao Y., Zhou H., Hu Y., Zhang C., et al. (2023). Akkermansia muciniphila plays critical roles in host health. Crit. Rev. Microbiol. 49 (1), 82–100. doi: 10.1080/1040841X.2022.2037506
Zheng Y., Wang T., Tu X., Huang Y., Zhang H., Tan D., et al. (2019). Gut microbiome affects the response to anti-PD-1 immunotherapy in patients with hepatocellular carcinoma. J. Immunother. Cancer. 7 (1), 193. doi: 10.1186/s40425-019-0650-9
Zhu L., Zhang D., Zhu H., Zhu J., Weng S., Dong L., et al. (2018). Berberine treatment increases Akkermansia in the gut and improves high-fat diet-induced atherosclerosis in Apoe(-/-) mice. Atherosclerosis 268, 117–126. doi: 10.1016/j.atherosclerosis.2017.11.023
Keywords: Akkermansia muciniphila, immune-related disease, inflammation, herbal medicine, immune system
Citation: Ding X, Meng P-F, Ma X-X, Yue J-Y, Li L-P and Xu L-R (2024) Akkermansia muciniphila and herbal medicine in immune-related diseases: current evidence and future perspectives. Front. Microbiomes 3:1276015. doi: 10.3389/frmbi.2024.1276015
Received: 17 October 2023; Accepted: 15 January 2024;
Published: 05 February 2024.
Edited by:
Shenghui Li, Puensum Genetech Institute, ChinaReviewed by:
Zhiming Li, Beijing Genomics Institute (BGI), ChinaAhmad Ud Din, North Carolina State University, United States
Copyright © 2024 Ding, Meng, Ma, Yue, Li and Xu. This is an open-access article distributed under the terms of the Creative Commons Attribution License (CC BY). The use, distribution or reproduction in other forums is permitted, provided the original author(s) and the copyright owner(s) are credited and that the original publication in this journal is cited, in accordance with accepted academic practice. No use, distribution or reproduction is permitted which does not comply with these terms.
*Correspondence: Li-Ran Xu, eHVsaXJhbkBoYWN0Y20uZWR1LmNu