- 1Collaborative Innovation Center of Aquatic Sciences, Institute of Animal Science, Guangdong Academy of Agricultural Sciences, Key Laboratory of Animal Nutrition and Feed Science in South China, Ministry of Agriculture and Rural Affairs, Guangdong Provincial Key Laboratory of Animal Breeding and Nutrition, Guangzhou, China
- 2College of Fisheries, Huazhong Agricultural University, Wuhan, China
- 3State Key Laboratory of Biocontrol, Institute of Aquatic Economic Animals and the Guangdong Province Key Laboratory for Aquatic Economic Animals, Sun Yat-Sen University, Guangzhou, China
- 4Institute of Animal Health, Guangdong Academy of Agricultural Sciences, Guangzhou, China
- 5Guangdong Provincial Engineering Research Center of Prawn Culture, Guangdong Havwii Agricultural Group Co., Ltd., Zhanjiang, China
Soybean meal is considered as one of the major components of Litopenaeus vannamei diets. However, most previous studies have focused on evaluating the effects of soybean meal on L. vannamei from the perspective of growth, physiology, and feed utilization; information regarding the analysis of serum metabolites, antioxidant and immune response, and intestinal microbiota is limited. Five diets were prepared, comprising 20% (T20), 28% (T28), 35% (T35), 42% (T42), and 50% (T50) soybean meal. A total of 600 shrimp were randomly distributed into 20 tanks (i.e., 30 shrimp per tank), with four tanks assigned to each dietary group. Shrimp were fed to apparent satiation during the 42-day feeding trial. The results showed that levels of serum globulin, alanine aminotransferase, and aspartate aminotransferase linearly increased (p < 0.01), but levels of high-density lipoprotein cholesterol linearly decreased (p < 0.001) as the proportion of soybean meal in the diet increased. Supplementation of shrimp diets with soybean meal linearly and quadratically increased (p < 0.05) serum total antioxidant capacity, levels of malondialdehyde, and activities of catalase, nitric oxide synthase and phenoloxidase. Hepatocytes in T35, T42, and T50 were shown to have different degrees of vacuolar degeneration, hepatic corpuscle atrophy, and star-like lumen loss. Dietary inclusion of soybean meal altered the composition of intestinal bacterial microbiota at phylum level, especially increasing the abundance of on other bacterial genera, whereas it had minimal impact on other bacterial genera and had no significant influence on the bacterial diversity. This study suggests that dietary supplementation of L. vannamei diets with soybean meal at concentrations exceeding 28% induces inflammation and oxidant damage of the hepatopancreas, and increases the risk of intestinal disease.
Introduction
The scarcity and rising price of fish meal threaten the sustainable development of aquaculture. How to reduce the amount of fish meal in aquafeed has become an important question in this field. In recent years, soybean meal has been widely used to replace fish meal in both scientific research and commercial production, and has been presented as a promising alternative in aquafeed (Bae et al., 2020; Bruce et al., 2021; Wu et al., 2021a; Zhao et al., 2021a; Ding et al., 2022). Over the last decade, a number of studies have suggested that the replacement of fish meal with soybean meal is feasible when soybean meal is applied in appropriate doses (Yang et al., 2015; Kumar et al., 2017; Wang et al., 2020; Liu T. et al., 2021; Xu et al., 2021). However, dietary supplementation with soybean meal has also been reported to inhibit growth and induce damage in aquatic animals (Yun et al., 2017; Liu et al., 2018; Ding et al., 2019; Zhang et al., 2020; Liu Q. et al., 2021). Similarly, our recent study observed that diets exceeding 28% soybean meal significantly inhibited the growth, digestion, and muscle growth-related gene expression of shrimp (Litopenaeus vannamei) (Peng et al., 2022a). However, these previous studies mostly focused on evaluating the negative effects of soybean meal on aquatic animals from the perspective of growth, physiology, and feed utilization; studies examining multiple factors, such as serum metabolites, antioxidant and immune response, and intestinal microbiota, are rare. Studying the effect of soybean meal on these factors may contribute to further revealing the mechanism of action regarding the growth-inhibiting effect of soybean meal.
It is known that the growth of L. vannamei is highly dependent on fish meal (Suárez et al., 2009). Among the species used globally in aquaculture species, L. vannamei consumes the largest amount of fish meal, accounting for approximately 30% of total fish meal consumption in aquafeed (Gao et al., 2021). Therefore, research on the replacement of fish meal in L. vannamei is comparatively more important than in other species. Soybean meal is considered as one of the major components of shrimp diets (Shao, 2019). Based on previous studies of the application of soybean meal in L. vannamei diets (Amaya et al., 2007; Yang et al., 2015; Xu et al., 2021), our recent study indicated that the growth-inhibiting effect of high doses of dietary soybean meal on L. vannamei is attributed not only to the depression of feed digestibility but also to the inhibition of muscle growth (Peng et al., 2022a). Another study also reported that this growth-inhibiting effect may be due to damage of the hepatopancreas and intestine induced by inclusion of soybean meal in L. vannamei diets (Sun, 2015). This suggests that the influence of soybean meal on the health of L. vannamei manifests in many ways. This study was carried out to evaluate the effects of replacing fish meal with soybean meal on serum metabolites, antioxidants and the immune response, hepatopancreas histomorphology, and the intestinal bacterial microbiota of L. vannamei.
Materials and methods
Experimental diet preparation
Five experimental diets were prepared, comprising 20% (T20), 28% (T28), 35% (T35), 42% (T42), and 50% (T50) soybean meal. The ingredient and nutrient compositions of the diets are shown in Table 1. Diets were prepared and stored as previously detailed by Peng et al. (2022a). Briefly, all ingredients were ground to pass through a 320-μm sieve (AHZC1265 Hammer Mill; Buhler Machinery Co., Ltd., Guanghzou, China), mixed thoroughly (AHML2000 Mixer; Buhler Machinery Co., Ltd.), and then extruded into 2-mm pellets (SLX-80 Twin-screw Extruder; South China University of Technology Machinery Factory, Guangzhou, China). After being pelleted, the feeds were dried at 55°C for 12 h (HMO-205 Oven Dryer; Haiming Electronic Technology Co., Ltd., Dongguan, China) and stored at –20°C until use. Feeds were put into sealed bags and delivered to the fishery when needed.
Experimental procedure
The protocol (no. GAAS20210501) of this study was approved by the Animal Care and Use Committee of Guangdong Academy of Agricultural Sciences (Guangdong, China). The experiment was conducted in an indoor circulating water aquaculture system between September and October 2021.
A total of 600 shrimp (initial body weight of approximately 5.8 g) were randomly distributed into 20 tanks (i.e., 30 shrimp per tank), with four tanks being assigned to each diet. Shrimp were hand fed to apparent satiation (i.e., with feed approximately 4% of their body weight per day) three times a day at 08:00, 14:00, and 20:00. During the 42-day feeding trial, the water temperature was 25–27°C, the dissolved oxygen in the water was above 5.0 mg/L, the water pH was 7.6–8.0, the water salinity was 5‰–6‰, and ammonia nitrogen and nitrite concentrations in the water were below 0.01 mg/L.
Sampling
Before sampling, all shrimp were fasted for 24 h. Blood was collected from the cardio-coelom of 15 shrimp in each tank, pooled, and kept at room temperature for 30 min, then centrifuged at 3,500 rev/min for 10 min. The resultant serum was stored at –80°C for the analysis of serum metabolites and antioxidant and immune parameters.
Three shrimp per tank were randomly selected for the histological examination of the hepatopancreas. Samples were fixed in 4% paraformaldehyde solution for 24 h. Slices were stained with the hematoxylin and eosin (Howard and Smith, 1983) and examined under light microscopy (Wang et al., 2021).
The intestines of three shrimp per tank were sampled on a clean bench, put into a 1.5-mL sterile centrifuge tube, and immediately stored at –80°C for subsequent DNA extraction.
Analysis of nutrient compositions of diets
The nutrient compositions of the diets were analyzed using the methods of the AOAC (1999). Dry matter was measured by drying samples to a constant weight at 105°C (AOAC, #930.15). Crude protein was calculated by determining the total nitrogen (N × 6.25) using the Kjeldahl method (2300-Autoanalyzer, FOSS, Denmark) (AOAC, #2001.11). Crude lipid was measured by gravimetric analysis following ether extraction of lipids according to the Soxhlet method (36680-analyzer, BUCHI, Switzerland) (AOAC, #920.39). Ash was examined by combustion in a muffle furnace at 550°C for 6 h (AOAC, 1999; #942.05). Dietary lysine and methionine contents were determined by chromatography (Xu et al., 2016).
Serum metabolites analysis
The following serum metabolites were measured using a BK-200VET biochemical analyzer (OLABO, China): albumin (ALB), globulin (GLOB), total cholesterol (TCHO), alanine aminotransferase (ALT), aspartate aminotransferase (AST), high-density lipoprotein cholesterol (HDLC), low-density lipoprotein cholesterol (LDLC), and glucose (GLU). The following serum antioxidant and immune parameters were determined by commercial kits provided by Nanjing Jiancheng Bioengineering Institute (Nanjing, China): total antioxidant capacity (TAOC; #A015-1), superoxide dismutase (SOD; #A001-1-2), catalase (CAT; #A007-1-1), glutathione peroxidase (GPx; #A005-1-2), malondialdehyde (MDA; #A003-1-2), alkaline phosphatase (AKP; #A059-2-2), lysozyme (LZM; #A050-1-1), nitric oxide synthase (NOS; #A014-2-2), and phenoloxidase (PPO; #A136-1-1).
Intestinal bacterial communities analysis
The methods of bacterial DNA extraction and 16S rRNA sequencing followed the procedures described by Peng et al. (2021a). The V3–V4 region of 16S was targeted using primers 347F-CS1 (5′-ACACTGACGACATGGTTCTACAGGAGGCAGCAGTRRGGAAT-3′) and 803R-CS2 (5′-TACGGTAGCAGAGACTTGGTCTCTACCRGGGTATCTAATCC-3′). The real-time PCR conditions for 16S rRNA were 3 min at 95°C, followed by 45 cycles of 30 s at 95°C, 30 s at 60°C, and 45 s at 72°C. The composition and diversity of intestinal bacterial communities were analyzed using Illumina PE250 sequencing technology. Sequencing reads were assigned to each sample and analyzed with the QIIME package. Alpha diversity parameters were calculated using the Phyloseq Package (McMurdie and Holmes, 2013). Beta diversity was analyzed using the Pheatmap Package (Kolde and Kolde, 2015). Phenotypic classification prediction (pathogenic) of bacterial communities was conducted using BugBase, based on the Greengenes database (Ward et al., 2017). The 16S sequencing raw sequence reads were deposited in the NCBI sequence read archive (SRA) database, with the BioProject accession number PRJNA911431.
Statistical analysis
IBM SPSS Statistics version 17.0 software (IBM Corporation, Armonk, NY, USA) was used to analyze the experimental data. All data were compared through one-way ANOVA, with the tank as the statistical unit and treatment as a fixed effect. Polynomial contrasts were used to test linear and quadratic responses to dietary soybean meal levels. The level of significance was set at a p-value < 0.05.
Results
Serum metabolites
The levels of GLOB linearly increased (p < 0.01) as dietary soybean meal increased from 200 to 500 g/kg and the increase reached significance at 280, 350, 420, and 500 g/kg (Table 2). Levels of ALT and AST linearly increased (p < 0.001), whereas HDLC levels linearly decreased (p < 0.001), as dietary soybean meal increased. All shrimp had similar (p > 0.05) levels of serum ALB, TCHO, LDLC, and GLU across the groups.
Serum antioxidant and immune response
Activities of serum TAOC and CAT, and levels of MDA linearly (p < 0.001) and quadratically (p ≤ 0.001) increased as dietary soybean meal increased from 200 to 500 g/kg, and the increase reached significance at 350, 420, and 350 g/kg, respectively (Table 3). The activities of NOS linearly (p < 0.001) and quadratically (p < 0.05) increased as dietary soybean meal increased, and the increase reached significance at 280, 350, 420, and 500 g/kg. PPO activities linearly (p < 0.001) increased as dietary soybean meal increased, and the increase reached significance at 350, 420, and 500 g/kg. Dietary treatments did not alter (p > 0.05) the serum activities of SOD, GPx, AKP, and LZM.
Hepatopancreas histological examination
The morphology of shrimp hepatopancreas is shown in Figure 1. The structure of hepatocytes in shrimp fed the T20 and T28 diets was normal, with neatly arranged hepatic corpuscles, complete basement membranes, star-like lumens, and abundant absorption cells. However, hepatocytes in T35, T42, and T50 showed varying degrees of vacuolar degeneration, hepatic corpuscle atrophy, and star-like lumen loss. Clearly, degeneration of or damage to hepatocytes increased as dietary soybean meal increased from 350 to 500 g/kg.
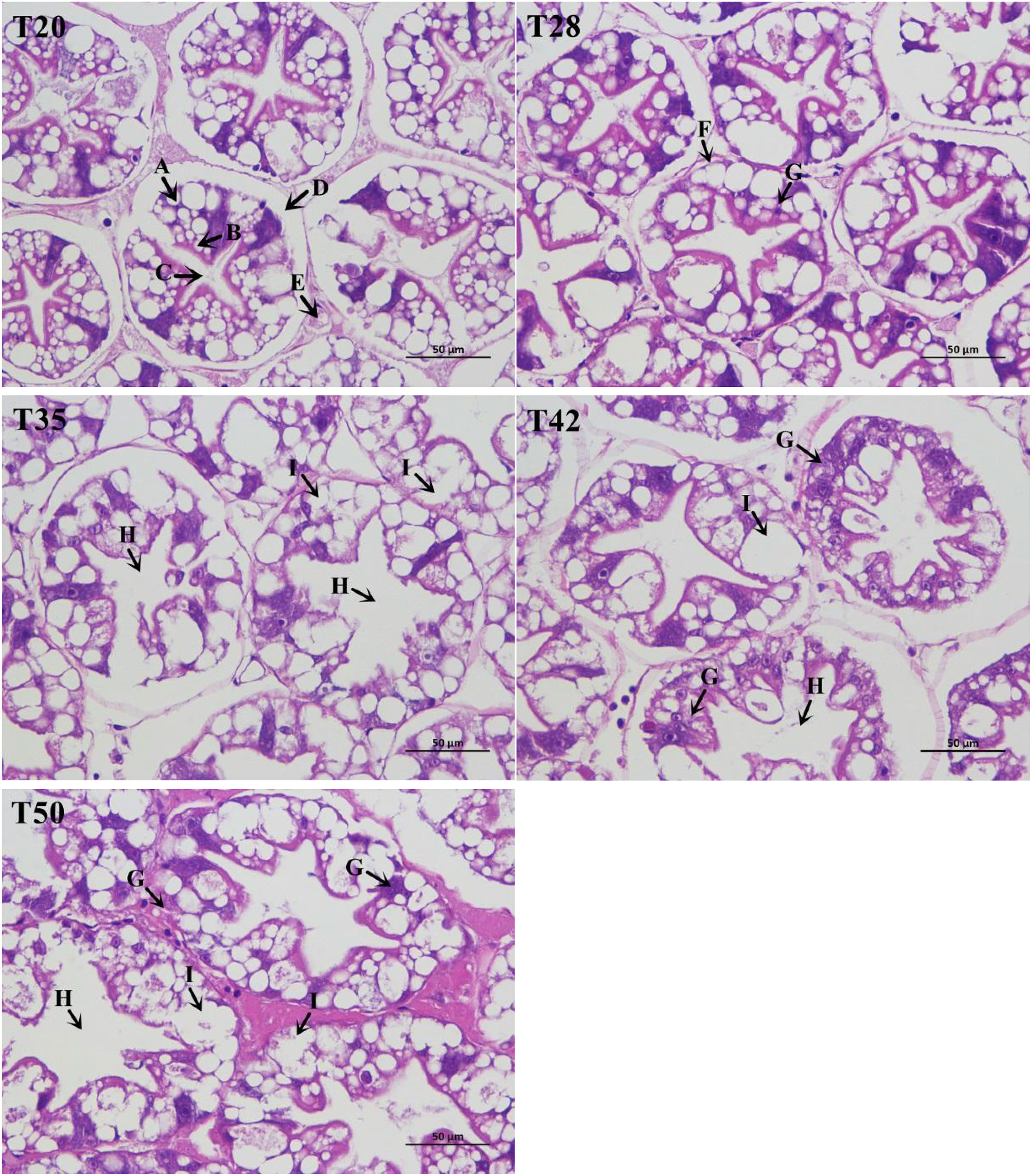
Figure 1 Histological appearance of the hepatopancreas (× 400) stained with hematoxylin and eosin. The proportion of soybean meal in each diet was 20% (T20), 28% (T28), 35% (T35), 42% (T42), or 50% (T50). (A) Absorptive cells; (B) secretory cells; (C) star shape of the lumen; (D) basal membrane; (E) tubules; (F) basal membrane atrophy; (G) inflammatory cell infiltration (unknown substances); (H) star-like lumen loss; and (I) vacuolar degeneration.
Intestinal bacterial microbiota
The Venn diagram shows that 314 operational taxonomic units (OTUs) were shared by five groups (Figure 2). The number of unique OTUs in T20, T28, T35, T42, and T50 groups was 264, 23, 90, 48, and 57, respectively. The top 10 dominant bacterial phyla in the intestine of shrimp (shown in Figure 3) were Proteobacteria, Tenericutes, Firmicutes, Bacteroidetes, Actinobacteria, Cyanobacteria GN02, Fusobacteria, Verrucomicrobia, and Chloroflexi. Compared with the T20 group, the relative abundances of Proteobacteria and Bacteroidetes increased (p < 0.05), but the relative abundance of Tenericutes decreased (p < 0.05) in other groups. The Circos map of the relationship between the groups and relatively abundant bacterial genera (tag number > 2,000) is shown in Figure 4. Relative abundances of the top 10 abundant bacterial genera are shown in Figure 5, i.e., Roseburia, Streptococcus, Bacteroides, Aeromonas, Lactobacillus, Paracoccus, Arcobacter, Photobacterium, Shewanella, and Vibrio.
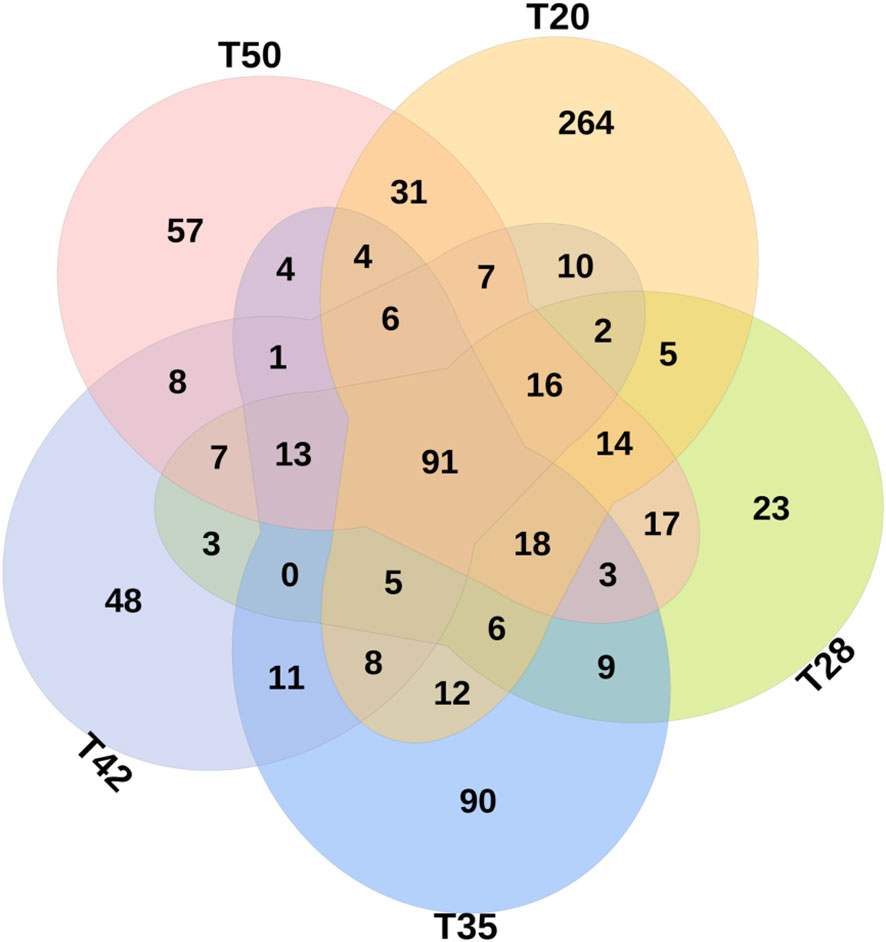
Figure 2 Venn diagrams showing unique and shared operational taxonomic units (OTUs) (at 97% similarity). Values are the numbers of OTUs calculated using the total data set at genus level. The proportion of soybean meal in each diet was 20% (T20), 28% (T28), 35% (T35), 42% (T42), or 50% (T50).
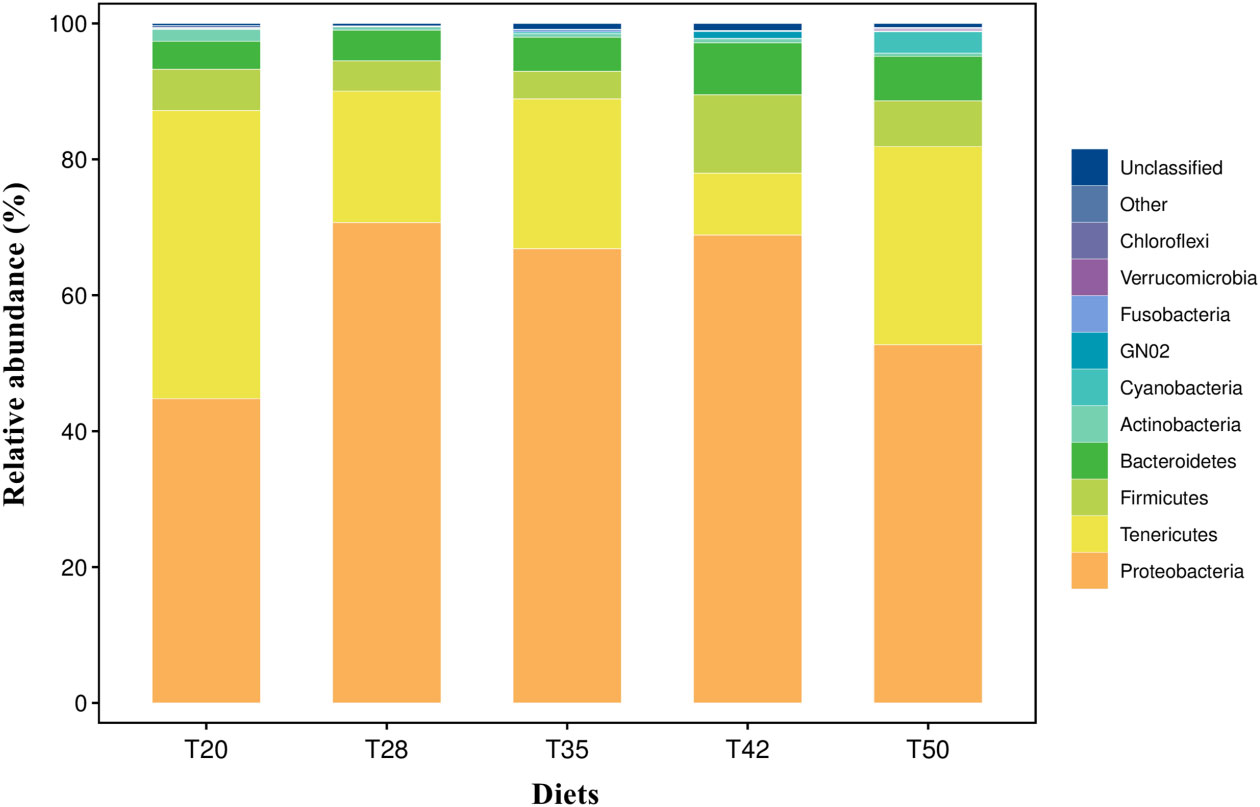
Figure 3 Taxonomic profile and relative abundance of the top 10 bacterial phyla. The proportion of soybean meal in each diet was 20% (T20), 28% (T28), 35% (T35), 42% (T42), or 50% (T50).
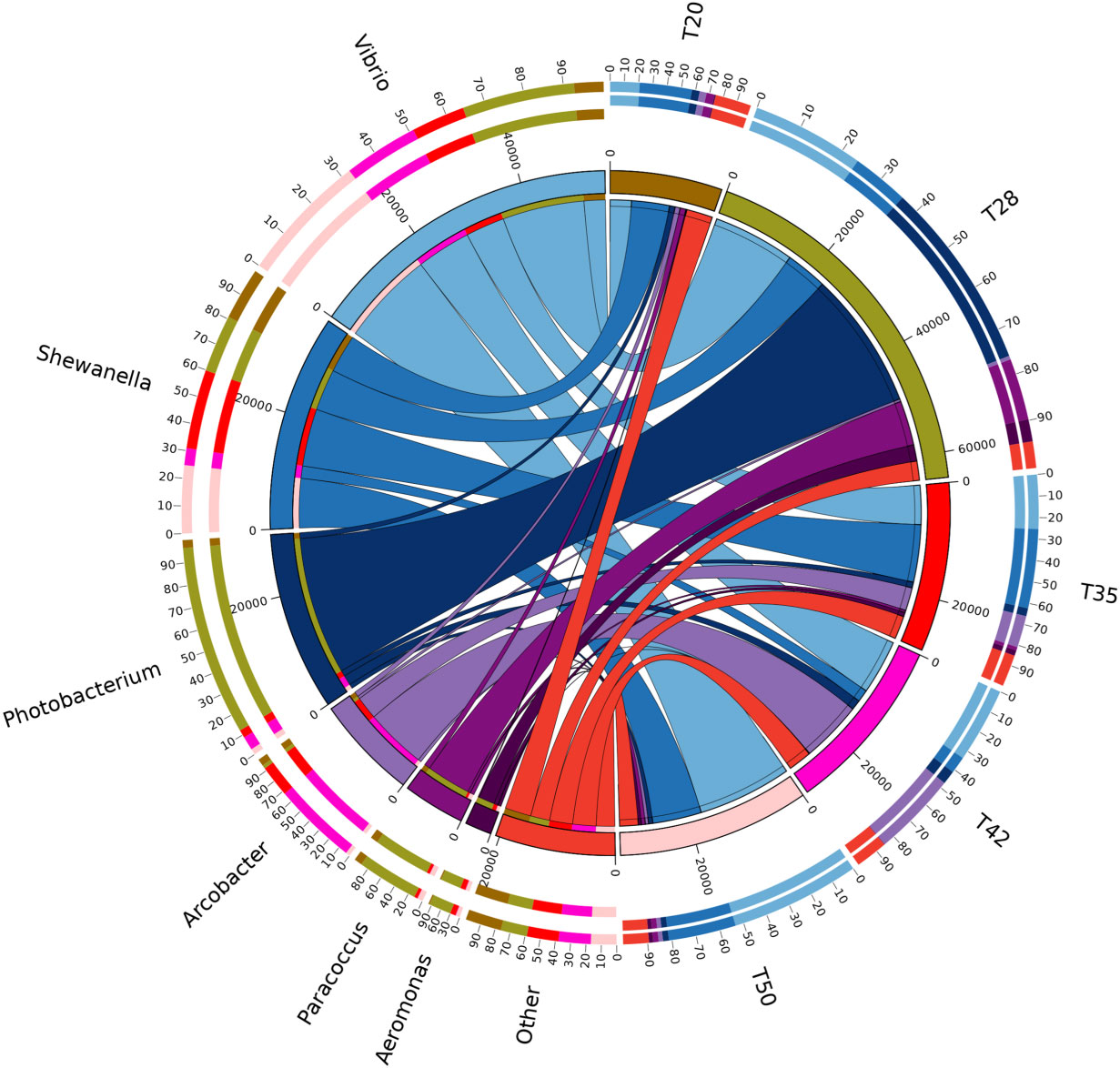
Figure 4 Circos map of the relationship between diets and relatively abundant bacterial genera (tags number > 2,000). The proportion of soybean meal in each diet was 20% (T20), 28% (T28), 35% (T35), 42% (T42), or 50% (T50).
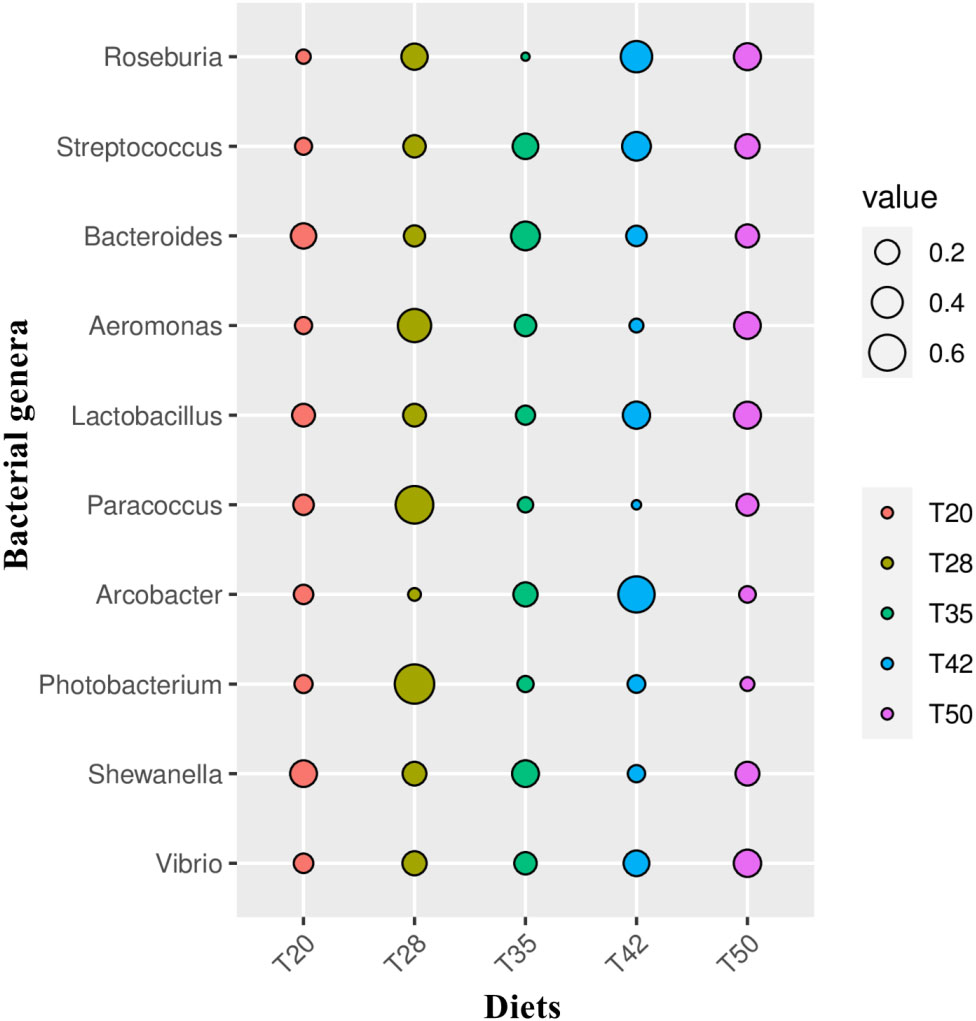
Figure 5 Relative abundances of the 10 most abundant bacterial genera. The indicator value of each bacterial genus in the comparison groups was calculated using the LABDSV package in R. Cross-validation is used for statistical verification to obtain the p-value. As shown in the bubble graph, the biomarkers of each group can be intuitively identified by the bubble size. The proportion of soybean meal in each diet was 20% (T20), 28% (T28), 35% (T35), 42% (T42), or 50% (T50).
The abundance-based coverage estimator (ACE), Chao, Shannon, and Simpson indicators, measures of alpha diversity, were similar (p > 0.05) among groups (Figure 6). In the case of beta diversity, distinction between bacterial communities was low, as most of the plots form separate clusters within each group (Figure 7). Similarly, when the beta diversity of bacterial communities was evaluated by the weighted UniFrac distances, a heatmap analysis showed minimal difference among groups (Figure 8).
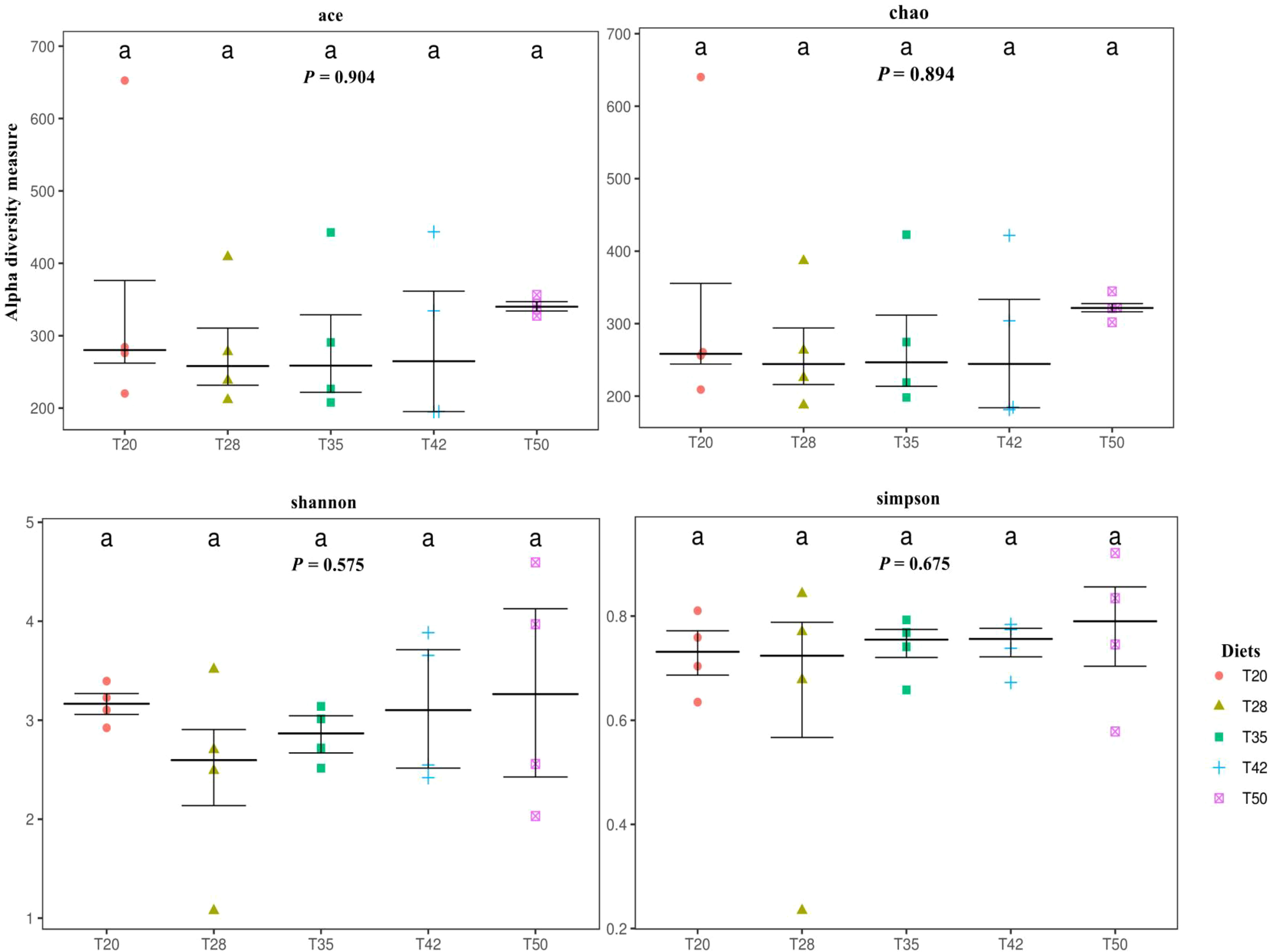
Figure 6 Alpha diversity of bacterial communities. Each panel represents one alpha diversity measure. Abundance-based coverage estimator (ACE) and Chao indices are richness estimators to estimate the total number of operational taxonomic units (OTUs) present in a community. Shannon and Simpson indices are indicators of microbial diversity. The proportion of soybean meal in the diets was 20% (T20), 28% (T28), 35% (T35), 42% (T42), or 50% (T50).
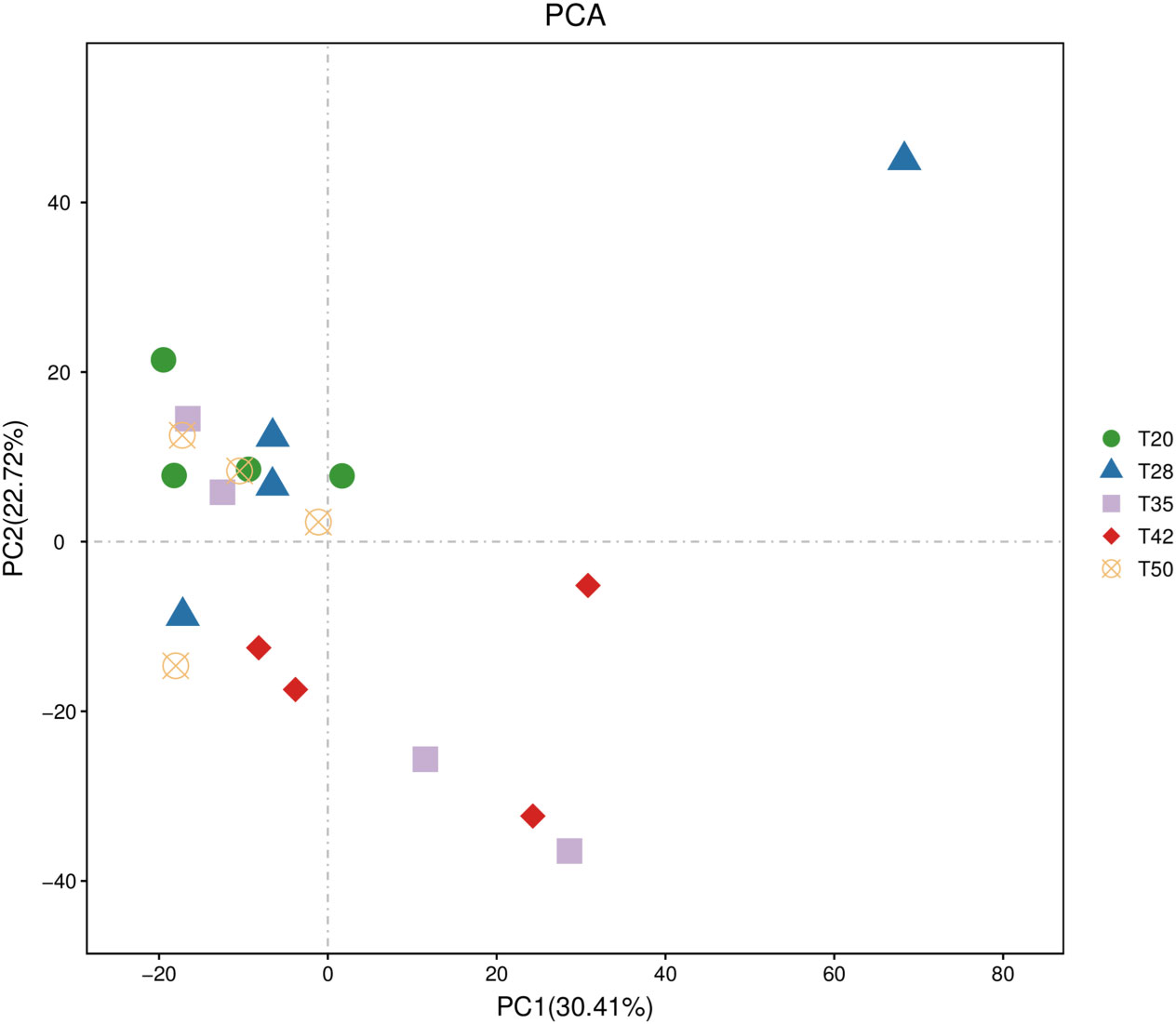
Figure 7 Beta diversity of bacterial communities. The operational taxonomic unit (OTU) principal component analysis (PCA) plots. The proportion of soybean meal in each diet was 20% (T20), 28% (T28), 35% (T35), 42% (T42), or 50% (T50).
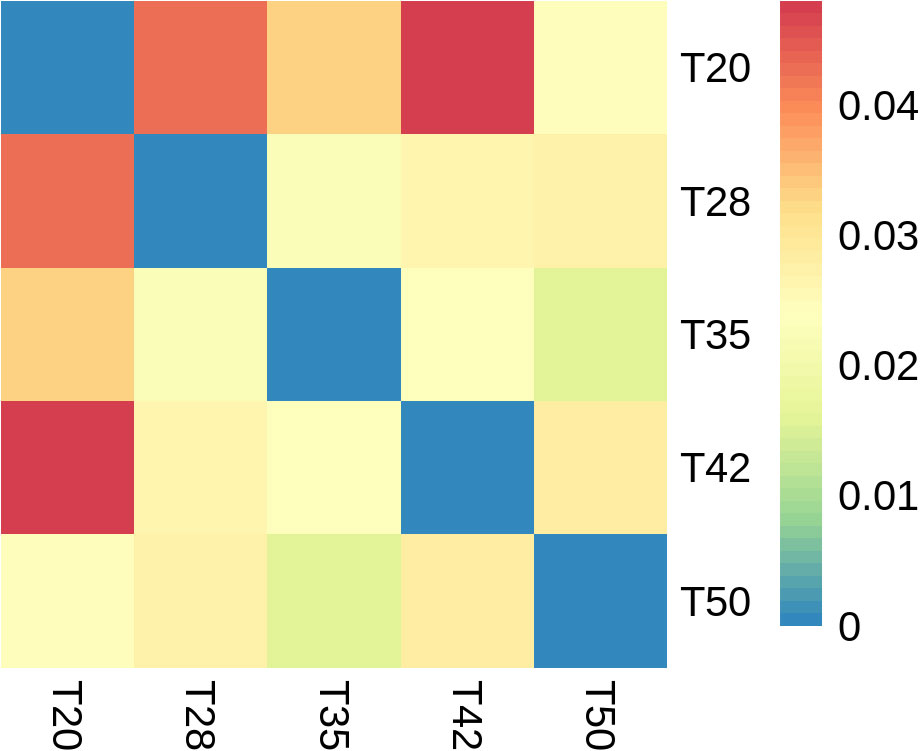
Figure 8 Beta diversity of bacterial communities. Heatmap analysis using the weighted UniFrac distances. The proportion of soybean meal in each diet was 20% (T20),28% (T28), 35% (T35), 42% (T42), or 50% (T50).
The contribution of bacterial communities at a phylum level to the phenotypic function (pathogenic) is shown in Figure 9. In contrast with T20, the major pathogenic bacterial communities in other groups were Proteobacteria, Tenericutes, Bacteroidetes, and Firmicutes.
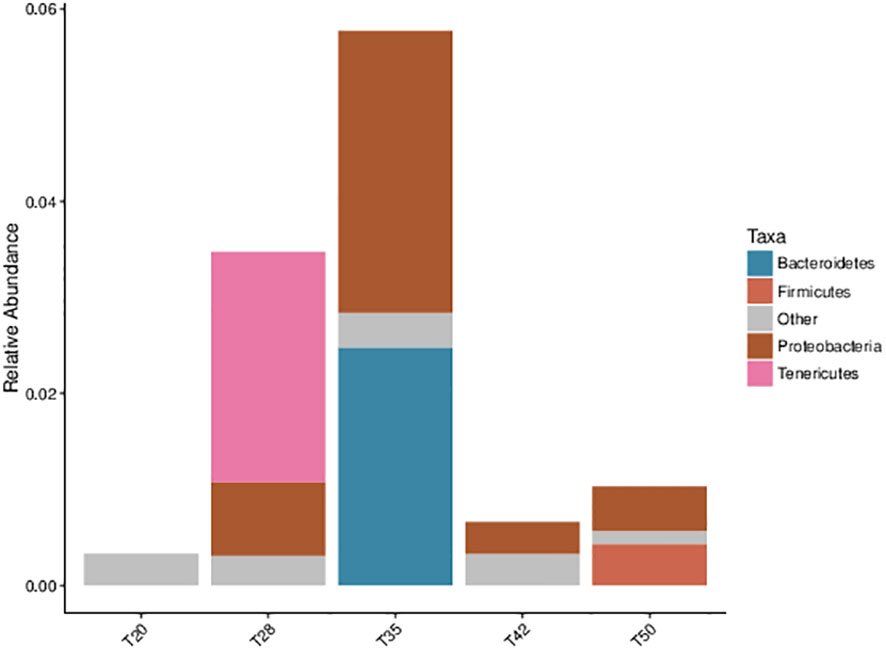
Figure 9 Phenotypic classification prediction of pathogenic bacterial communities using BugBase based on the Greengenes database. The contribution (relative abundance) of bacterial communities at phylum level to the phenotypic function. The proportion of soybean meal in each diet was 20% (T20), 28% (T28), 35% (T35), 42% (T42), or 50% (T50).
Discussion
Serum metabolites
Serum metabolites generally reflect the physiological status, health level, and nutrient metabolism of aquatic animals. In this study, partial changes in serum metabolites suggest that the supplementation of shrimp diets with soybean meal adversely impacted these metabolite profiles. As part of the immune system, concentrations of serum GLOB can reflect the inflammation and nutritional status of animals. Generally, the serum concentration of GLOB is positively correlated with inflammation level (Bu et al., 2018). Therefore, the increased GLOB concentration in the serum of shrimp fed soybean meal-supplemented diets in this study indicates that dietary soybean meal may induce inflammation and thus interfere with the immune system of shrimp. A similar result was also reported by Chen et al. (2014), who found that the inclusion of 60% soybean meal in the diets of grass carp increased their serum GLOB concentration. Both ALT and AST are related to liver damage or necrosis when their measured activity increases in blood, because the increased permeability of the cell membrane induced by injury or stress causes ALT and AST to be released from cells into the blood more rapidly via the cell membrane barrier (Peng et al., 2021b). In this study, the increased serum ALT and AST activities of L. vannamei suggest that dietary supplementation with soybean meal may impair the hepatopancreatic health of shrimp. This is consistent with the histological observation of the hepatopancreas in this study, which found that the degeneration of or damage to hepatocytes increased as the dietary levels of soybean meal increased. Liver injury is also commonly observed in fish when fish meal is replaced by soybean meal (Hernández et al., 2007; Lim et al., 2011; Hu et al., 2018). HDLC, an anti-atherosclerosis lipoprotein, transports cholesterol from other tissues to the liver for metabolism, preventing cholesterol from being deposited in blood vessel walls. Therefore, a low serum HDLC concentration normally indicates an increased risk of atherosclerosis, heart disease, and other conditions. In this study, supplementation of L. vannamei diets with soybean meal linearly decreased serum HDLC concentration, suggesting that soybean meal may cause dyslipidemia in shrimp. Similarly, Liu et al. (2010) documented that replacing dietary fish meal with soybean meal decreased serum HDLC concentration in Japanese flounder (Paralichthys olivaceus).
Antioxidant and immune response
The increase in serum TAOC as dietary levels of soybean meal increased in this study suggests that supplementation with soybean meal enhanced the antioxidant capacity of shrimp. This is also reflected in the increased serum CAT activity of shrimp. The results of this study are consistent with the finding by Li et al. (2020) that a diet in which fish meal was replaced with fermented soybean meal increased TAOC and MDA concentrations in the serum of Anguilla japonica. It has been reported that excessive accumulation of MDA can lead to oxidative damage (Ding et al., 2015). In this study, the increased antioxidant capacity and MDA concentrations in L. vannamei were probably a response to the oxidative damage stimulated by the supplementation of diets with soybean meal. An et al. (2018) reported that replacing more than 20% of fish meal with soybean meal in diets increased serum antioxidant capacity and caused oxidative stress in Epinephelus coioides.
The results of this study indicate that supplementation with soybean meal in shrimp diets increases serum NOS and PPO activity in shrimp. Commonly, NOS is a key rate-limiting factor in the synthesis of nitric oxide, which is a major catalyst of phagocytosis in the immune system (Ignarro, 1996). PPO is the product of the prophenoloxidase activation system of crustaceans, which plays an important role in recognizing foreign bodies, releasing opsonins to promote phagocytosis, and producing lectins or lysozyme to exclude foreign bodies (Liu et al., 2005). Therefore, the expression of NOS and PPO is generally regarded as an indication of immunostimulation or an increased risk of stress. In this study, the increase in serum NOS and PPO activity as dietary levels of soybean meal increased may be attributed to immunostimulation or stress induced by soybean meal in shrimp diets. Similarly, Wu et al. (2008) reported that cadmium stress induced an increase in nitric oxide synthase activity in the serum of L. vannamei. Peng et al. (2015) documented that replacing 6% of fish meal with fermented soybean meal in L. vannamei diets increased the PPO activity in the hemolymph of the shrimp.
Histological appearance of hepatopancreas
Histomorphology is an important part of understanding liver pathology. The observation of hepatopancreas histomorphology in this study suggested that dietary supplementation with soybean meal caused lesions in the hepatopancreas of L. vannamei. This may be due to the presence of antinutritional factors in soybean meal. To the best of our knowledge, this is the first study to evaluate the effects of dietary supplementation with soybean meal on the hepatopancreas histomorphology of L. vannamei. Chen et al. (2014) reported that a diet comprising 60% soybean meal caused liver damage in grass carp (Ctenopharyngodon idellus), as demonstrated by abnormal nuclear morphology, increased cell area and lipid content, and aggravated vacuolation. Similar results were also observed by Zhang et al. (2012) in the large yellow croaker (Pseudosciaena crocea): replacing 45% of fish meal with soybean meal decreased hepatocyte numbers and increased hepatocyte vacuolation. A number of studies have suggested that supplementation with plant protein sources in diets could impair liver function and induce liver damage in aquatic animals, as shown by reduced lipoprotein synthesis and the accumulation of lipids in the liver (Robaina et al., 1995; Feng et al., 2016; Hu et al., 2018; Lin et al., 2019; Chen et al., 2021).
Bacterial microbiota
Although the effects of dietary soybean meal on the growth and physiology of L. vannamei have been well evaluated, little information is available on the response of intestinal bacterial microbiota. It has been reported that the intestinal bacterial microbiota is closely related to the intestinal health of shrimp (Zhao et al., 2021b). In this study, within the phyla identified, over 94% of the sequences were classified within the phyla Proteobacteria, Tenericutes, Firmicutes, and Bacteroidetes. These dominant bacterial phyla identified in the intestine of L. vannamei were consistent with previous studies (Li et al., 2007; Wu et al., 2016; Shao, 2019; Zhao et al., 2021b), suggesting that the composition of the intestinal bacterial microbiota of L. vannamei was conserved to some extent. Among the dominant bacterial phyla, bacteria of the phylum Proteobacteria were the most abundant in the intestine of L. vannamei. This finding is similar to other reports (Cornejo-Granados et al., 2017; Hou et al., 2018; Shao, 2019). Furthermore, this study showed that supplementation with soybean meal in shrimp diets increased the abundance of Proteobacteria and Cyanobacteria but decreased the abundance of Tenericutes. Similarly, Shao (2019) reported that the replacement of fish meal with fermented soybean meal in L. vannamei diets increased the abundance of Proteobacteria, but decreased the abundance of Tenericutes in the intestine of shrimp. Zhao et al. (2021b) documented that dietary inclusion of soybean meal increased the abundance of Cyanobacteria in the intestinal bacterial microbiota of L. vannamei.
At the genus level, the composition of bacterial microbiota in the intestine of L. vannamei has been identified, and the effects of certain feedstuffs and additives on the bacterial genera found in the intestine of L. vannamei have been reported (Wu et al., 2016; Tang et al., 2021; Wu et al., 2021b; Zhu et al., 2022). However, information on the response of the intestinal bacterial genera of L. vannamei to dietary supplementation with soybean meal is scarce. Zhao et al. (2021b) evaluated the effects of replacing fish meal with soybean meal on the composition of intestinal microflora of L. vannamei only at the phylum and family levels. Although Shao (2019) reported the composition of the intestinal bacterial genera of L. vannamei fed with fermented soybean meal, the genera with higher abundance were mostly from unidentified families. Therefore, the 10 most abundant bacterial genera initially identified in the intestine of L. vannamei in this study provide the reference for the subsequent evaluation of the intestinal health of shrimp fed diets supplemented with soybean meal.
Alpha diversity refers to diversity within a certain biotope and is commonly described using specific alpha diversity indices such as ACE, Chao, Shannon, and Simpson (Peng et al., 2022b). This study shows that dietary treatments did not affect the ACE, Chao, Shannon, and Simpson indices of L. vannamei, suggesting that supplementation of L. vannamei diets with soybean meal had no significant effect on the alpha diversity of bacterial microbiota in the intestine of shrimp. Similar results were reported by Zhao et al. (2021b), who found that replacing 50% of fish meal with soybean meal in the diets of L. vannamei had no significant influence on the alpha diversity as measured with the Chao, Shannon, and Simpson indices. Shao (2019) also reported that replacing up to 40% of fish meal with fermented soybean meal in L. vannamei diets did not alter the alpha diversity as measured with the Shao, Shannon, and Simpson indices.
Beta diversity reveals differences in the bacterial community structure and is commonly assessed using the weighted UniFrac distances (Peng et al., 2018) and visualized as principal component analysis plots (Yang et al., 2018). In this study, dietary treatments had no significant influence on the beta diversity of the intestinal bacterial microbiota of L. vannamei. This is consistent with the observation by Shao (2019) that replacing dietary fish meal with fermented soybean meal in L. vannamei diets did not alter the beta diversity of intestinal bacterial communities.
Several large microbial pathway databases, such as the Kyoto Encyclopedia of Genes and Genomes (Kanehisa et al., 2012) and Clusters of Orthologous Groups (Tatusov et al., 1997), can be used to analyze the functionality of a microbiome. However, current tools cannot use sequencing data to readily infer common microbial phenotypes. Although the function prediction of intestinal bacterial microbiota has been reported in L. vannamei (Yu et al., 2018; Shao, 2019), the phenotype analysis of intestinal bacterial microbiota is limited. This is the first study to assess the phenotypes of intestinal bacterial microbiota impacted by soybean meal in L. vannamei diets. BugBase software (a microbiome analysis tool that determines high-level phenotypes present in microbiome samples) is usually used to classify and compare seven phenotypes of intestinal flora: Gram positive, Gram negative, biofilm forming, pathogenic, mobile element containing, oxygen utilizing, and oxidative stress tolerant (Ward et al., 2017; Yi et al., 2021). In this study, the phenotypic classification prediction of pathogenic bacterial communities in the intestine of L. vannamei fed soybean meal-containing diets suggested that the phylum Proteobacteria plays a major role in contributing to pathogenic phenotype, followed by Tenericutes, Bacteroidetes, and Firmicutes. BugBase predicted that L. vannamei fed a diet supplemented with soybean meal, compared with shrimp fed a control diet, would contain significantly more potentially pathogenic bacteria in their intestine. Sun (2015) reported that replacing 50% of fish meal with soybean meal in L. vannamei diets induced injury of the intestinal structure and increased the abundance of pathogenic bacteria. A similar result was also observed by Zhao et al. (2021b) in L. vannamei and by Yin (2019) in grouper (Epinephelus fuscoguttatus ♀ × E. lanceolatu ♂).
Conclusion
Supplementation of L. vannamei diets with soybean meal induced varying degrees of inflammation and injury of the hepatopancreas, as shown by the assessment of serum metabolites and hepatopancreas histomorphology. An increase in serum antioxidant capacity and the immunity of shrimp is probably in response to the oxidative damage stimulated by dietary supplementation with soybean meal. Although inclusion of soybean meal in L. vannamei diets significantly altered the relative abundances of intestinal bacterial microbiota at the phylum level, it had a minimal impact on the top 10 bacterial genera and had no significant influence on bacterial diversity. Phenotype analysis suggested that dietary supplementation with soybean meal led to significantly more potentially pathogenic bacteria in the intestine of L. vannamei.
Data availability statement
The data sets presented in this study can be found in online repositories. The names of the repository/repositories and accession number(s) can be found in the article/supplementary material.
Author contributions
KP and WH conceived the experiment. KP, JQ, CL, HL, ZL, and DL carried out the experiment. KP analyzed the data and wrote the paper. All authors contributed to the article and approved the submitted version.
Funding
This study was funded by the Collaborative Innovation Center Project of Guangdong Academy of Agricultural Sciences (XT202301, XT20230, XT20231), the Special Fund for Rural Revitalization Strategy of Guangdong Province (2022-SPY-00-007) the Innovation Fund project of Guangdong Academy of Agricultural Sciences (202137, 202138), the Special Fund for Scientific Innovation Strategy-Construction of the High-Level Academy of Agriculture Science (R2021PY-QY001), and the Guangdong Academy of Agricultural Science Talent Introduction Program (R2020YJ-QG001).
Acknowledgments
The authors are thankful to the aquafarm staff at the Institute of Animal Science, Guangdong Academy of Agricultural Science for their technical assistance.
Conflict of interest
Author DL is employed by Guangdong Havwii Agricultural Group Co., Ltd.
The remaining authors declare that the research was conducted in the absence of any commercial or financial relationships that could be construed as a potential conflict of interest.
Publisher’s note
All claims expressed in this article are solely those of the authors and do not necessarily represent those of their affiliated organizations, or those of the publisher, the editors and the reviewers. Any product that may be evaluated in this article, or claim that may be made by its manufacturer, is not guaranteed or endorsed by the publisher.
References
Amaya E., Davis D. A., Rouse D. B. (2007). Replacement of fish meal in practical diets for the pacific white shrimp (Litopenaeus vannamei) reared under pond conditions. Aquaculture 262, 393–401. doi: 10.1016/j.aquaculture.2006.11.015
An M., Fan Z., Wang Q., Sun J., Xu D., Guo Y., et al. (2018). Influences of dietary replacement of fishmeal by soybean meal on growth performance, digestive ability and antioxidant ability of Epinephelus malabaricus. Jiangsu Agric. Sci. 46 (16), 128–132. doi: 10.15889/j.issn.1002-1302.2018.16.032
AOAC (1999). Official methods of analysis of the association of official agricultural chemists. 16th ed (Gaithersburg: AOAC International, 5th rev).
Bae J., Hamidoghli A., Djaballah M. S., Maamri S., Hamdi A., Souffi I., et al. (2020). Effects of three different dietary plant protein sources as fishmeal replacers in juvenile whiteleg shrimp, Litopenaeus vannamei. Fish. Aquat. Sci. 23 (1), 2. doi: 10.1186/s41240-020-0148-x
Bruce T. J., Oliver L. P., Ma J., Small B. C., Hardy R. W., Brown M. L., et al. (2021). An initial evaluation of fishmeal replacement with soy protein sources on growth and immune responses of burbot (Lota lota maculosa). Aquaculture 545, 737157. doi: 10.1016/j.aquaculture.2021.737157
Bu Y., Chen J., Li J., Cheng Q., Li H., Liu X. (2018). Effects of enterobacitracin supplementation on performance and serum biochemical indices of weaning piglets. Chin. J. Anim. Nutr. 30 (2), 696–706. doi: 10.3969/j.issn.1006-267x.2018.02.035
Chen X., Chen S., Huang W., Wang G., Huang M., Wu H., et al. (2021). Effects of fishmeal replacement by fermented soybean meal with bacteria and enzyme on growth performance, serum biochemical, immune and antioxidant indexes and hepatic histology of largemouth bass (Micropterus salmonides). Chin. J. Anim. Nutr. 33 (5), 2848–2863. doi: 10.3969/j.issn.1006-267x.2021.05.043
Chen K., Ye Y., Cai C., Huang Y., Liu H., Gong Z., et al. (2014). Effects of dietary soybean meal content on hepatopancreas structure and function of grass carp (Ctenopharyngodon idellus). Chin. J. Anim. Nutr. 26 (7), 1873–1879. doi: 10.3969/j.issn.1006-267x.2014.07.020
Cornejo-Granados F., Lopez-Zavala A. A., Gallardo-Becerra L., Mendoza-Vargas A., Sánchez F., Vichido R., et al. (2017). Microbiome of pacific whiteleg shrimp reveals differential bacterial community composition between wild, aquacultured and AHPND/EMS outbreak conditions. Sci. Rep. 7, 11783. doi: 10.1038/s41598-017-11805-w
Ding L., Wang L., Lu K., Song K., Zhang C. (2019). Effects of replacement of fish meal with soybean meal on growth performance, digestive enzyme activity and intestinal health of Rana catesbeiana. Fresh. Fish. 49 (2), 69–75. doi: 10.13721/j.cnki.dsyy.2019.04.011
Ding Z., Zhang Y., Ye J., Du Z., Kong Y. (2015). An evaluation of replacing fish meal with fermented soybean meal in the diet of Macrobrachium nipponense: growth, nonspecific immunity, and resistance to aeromonas hydrophila. Fish Shellfish Immunol. 44, 295–301. doi: 10.1016/j.fsi.2015.02.024
Ding Z., Zhou D., Zheng J., Chen X., Kong Y., Qi C., et al. (2022). Replacing fishmeal with soybean meal affects survival, antioxidant capacity, intestinal microbiota, and mRNA expression of TOR and S6K1 in Macrobrachium rosenbergii. Chin. J. Oceanol. Limn. 40, 805–817. doi: 10.1007/s00343-021-0494-2
Feng J., Wang P., He J., Lou Y., Dang H., Deng R. (2016). Effects of replacement of fish meal by fermented soybean meal on growth performance, body composition, serum biochemical indices and liver tissue morphology of juvenile large yellow croaker (Larimichthys crocea). Chin. J. Anim. Nutr. 28 (11), 3493–3502. doi: 10.3969/j.issn.1006-267x.2016.11.016
Gao C., Hao T., Yang J., Sun J. (2021). Nutritional requirements analysis of Litopenaeus vannamei. J. Anhui Agric. Sci. 49 (1), 1–10. doi: 10.3969/j.issn.0517-6611.2021.01.001
Hernández M. D., Martínez F. J., Jover M., García B. G. (2007). Effects of partial replacement of fish meal by soybean meal in sharpsnout seabream (Diplodus puntazzo) diet. Aquaculture 263, 159–167. doi: 10.1016/j.aquaculture.2006.07.040
Hou D., Huang Z., Zeng S., Liu J., Wei D., Deng X., et al. (2018). Intestinal bacterial signatures of white feces syndrome in shrimp. Appl. Microbiol. Biotechnol. 102, 3701–3709. doi: 10.1007/s00253-018-8855-2
Howard D. W., Smith C. S. (1983). Histological techniques for marine bivalve molluscs (Woods Hole, MA: NOAA Technical Memorandum NMFS-F/NEC-25), 97.
Hu S., Wang L., Lou B., Zeng W., Tan P., Zhan W., et al. (2018). Effects of replacing fish meal with fermented soybean meal on amino acids in muscle and expression of IGF-I in liver and muscle of juvenile Nibea albiflora. J. Zhejiang Ocean Univ. 37 (3), 196–202.
Ignarro L. J. (1996). Physiology and pathophysiology of nitric oxide. Kidney Int. Suppl.) 55, S2–S5. doi: 10.1016/0891-0618(95)90027-6
Kanehisa M., Goto S., Sato Y., Furumichi M., Tanabe M. (2012). KEGG for integration and interpretation of large-scale molecular data sets. Nucleic Acids Res. 40, D109–D114. doi: 10.1093/nar/gkr988
Kumar S., Sándor Zs J., Nagy Z., Fazekas G., Havasi M., Sinha A. K., et al. (2017). Potential of processed animal protein versus soybean meal to replace fish meal in practical diets for European catfish (Silurus glanis): growth response and liver gene expression. Aquacult. Nutr. 23, 1179–1189. doi: 10.1111/anu.12487
Li N., Liu L., Hua X., Li X., Yao J., Wu M. (2020). Effects of soybean meal on fermented soybean meal partially replacing fish meal in the feed of Anguilla japonica: Growth performance, antioxidant capacity and biochemical indices. Mar. Fish. 42 (3), 352–364. doi: 10.13233/j.cnki.mar.fish.2020.03.011
Li K., Zheng T., Tian W., Yuan J. (2007). Bacterial community structure in intestine of the white shrimp, Litopenaeus vannamei. Acta Microbiol. Sin. 47 (4), 649–653. doi: 10.13343/j.cnki.wsxb.2007.04.016
Lim S., Kim S., Ko G., Song J., Oh D., Kim J., et al. (2011). Fish meal replacement by soybean meal in diets for tiger puffer, Takifugu rubripes. Aquaculture 313, 165–170. doi: 10.1016/j.aquaculture.2011.01.007
Lin Y., Shao X., Jin Y., Zhang Y., Wu C., Wang C., et al. (2019). Effects of fish meal replacement by animal and plant protein mixture on morphology indexes, tissue fatty acid composition, serum biochemical indexes and hepatic histology of juvenile black carp (Mylopharyngodon piceus). Chin. J. Anim. Nutr. 31 (2), 732–745. doi: 10.3969/j.issn.1006-267x.2019.02.030
Liu T., Han T., Wang J., Liu T., Bian P., Wang Y., et al. (2021). Effects of replacing fish meal with soybean meal on growth performance, feed utilization and physiological status of juvenile redlip mullet Liza haematocheila. Aquacult. Rep. 20, 100756. doi: 10.1016/j.aqrep.2021.100756
Liu Y., Meng L., Zhang J. (2018). Effect of partial fish meal replacement by soybean meal on the growth performance, nutritional composition and blood biochemical indices of juvenile Epinephelus fuscoguttatus. China Feed 4, 60–64. doi: 10.15906/j.cnki.cn11-2975/s.20180412
Liu Y., Wang W., Wang A., Miao Y. (2005). Effects of taurine on growth of Macrobrachium nipponense and activity of phenoloxidase. Freshw. Fish. 35 (2), 28–30. doi: 10.3969/j.issn.1000-6907.2005.02.009
Liu Q., Wen B., Man D., Jiang Y., Li X., Zuo R. (2021). Substitution of fish meal with soybean meal for juvenile Chinese mitten crab (Eriocheir sinensis) reared at three salinities: Effects on survival, growth, antioxidant capacity and body composition. Aquacult. Res. 52, 3723–3735. doi: 10.1111/are.15217
Liu X., Ye J., Wang Z., Wang K., Kong J. (2010). Partial replacement of fish meal by soybean meal in diets for juvenile Japanese flounder (Paralichthys olivaceus). J. Fish. China 34 (3), 450–458. doi: 10.3724/SP.J.1231.2010.06541
McMurdie P. J., Holmes S. (2013). Phyloseq: An r package for reproducible interactive analysis and graphics of microbiome census data. PloS One 8, e61217. doi: 10.1371/journal.pone.0061217
Peng K., Chen X., Lu H., Zhao J., Chen Y., Li C., et al. (2022a). Effect of dietary soybean meal on growth performance, apparent digestibility, intestinal digestive enzyme activity, and muscle growth-related gene expression of Litopenaeus vannamei. Front. Mar. Sci. 9, 945417. doi: 10.3389/fmars.2022.945417
Peng K., Chen B., Sun Y., Chen X., Wang Y., Huang W. (2022b). Response of growth performance, serum metabolites, intestinal tight junction structure and bacterial microbiomes to the long-term intervention of aflatoxin B1 in Lateolabrax maculatus diets. Aquaculture 22, 101005. doi: 10.1016/j.aqrep.2022.101005
Peng K., Jin L., Niu Y., Huang Q., McAllister T. A., Yang H. E., et al. (2018). Condensed tannins affect bacterial and fungal microbiomes and mycotoxin production during ensiling and upon aerobic exposure. Appl. Environ. Microbiol. 84, e02274–e02217. doi: 10.1128/AEM.02274-17
Peng K., Xiao H., Mo W., Wang G., Huang Y. (2021b). Effects of dietary black soldier fly larvae meal on serum metabolites, antioxidant and immune indexes and intestinal histological appearance of Micropterus salmoides. Chin. J. Anim. Nutr. 33 (12), 6964–6972. doi: 10.3969/j.issn.1006
Peng S., Zhang M., Li X., Zhao J., Zheng X., Li B., et al. (2015). Effects of replacing fish meal with fermented soybean meal on growth, muscle composition and non-specific immunity of Litopenaeus vannamei. Freshw. Fish. 45 (5), 70–75. doi: 10.13721/j.cnki.dsyy.20150715.015
Peng K., Zhao H., Wang G., Chen B., Mo W., Huang Y. (2021a). Effect of condensed tannins on growth performance, intestinal immune capacity and bacterial microbiomes of Lateolabrax japonicas. Aquacult. Res. 52, 5321–5331. doi: 10.1111/are.15402
Robaina L., Izquierdo M. S., Moyano F. J., Socorro J., Vergara J. M., Montero D., et al. (1995). Soybean and lupin seed meals as protein sources in diets for gilthead seabream (Sparus aurata): nutritional and histological implications. Aquaculture 130, 219–233. doi: 10.1016/0044-8486(94)00225-D
Shao J. (2019). Response mechanism of litopenaeus vannamei to fish meal substitutes in feed. PhD thesis (Beijing, China: University of Chinese Academy of Sciences).
Suárez J. A., Gaxiola G., Mendoza R., Cadavid S., Garcia G., Alanis G., et al. (2009). Substitution of fish meal with plant protein sources and energy budget for white shrimp Litopenaeus vannamei (Boone 1931). Aquaculture 289, 118–123. doi: 10.1016/j.aquaculture.2009.01.001
Sun Y. (2015). Effects of several feed additives on growth performance, immune response and intestinal health of litopenaeus vannamei fed diets with fish meal partially replaced by soybean meal. master thesis (Xiamen, China: Jimei University).
Tang S., Han F., Zhou L., Liu S., Xu C., Chen L., et al. (2021). Effects of dietary mannan oligosaccharide supplementation on growth, health and intestinal microbiota of pacific white shrimp, Litopenaeus vannamei, at low salinity. J. Fish. China 45 (12), 2044–2060. doi: 10.11964/jfc.20210312698
Tatusov R. L., Koonin E. V., Lipman D. J. (1997). A genomic perspective on protein families. Science 278, 631–637. doi: 10.1126/science.278.5338.631
Wang G., Peng K., Hu J., Mo W., Wei Z., Huang Y. (2021). Evaluation of defatted hermetia illucens larvae meal for Litopenaeus vannamei: effects on growth performance, nutrition retention, antioxidant and immune response, digestive enzyme activity and hepatic morphology. Aquacult. Nutr. 27, 986–997. doi: 10.1111/anu.13240
Wang L., Wang J., Lu K., Song K., Mai K., Zhang C., et al. (2020). Total replacement of fish meal with soybean meal in diets for bullfrog (Lithobates catesbeianus): Effects on growth performance and gut microbial composition. Aquaculture 524, 735236. doi: 10.1016/j.aquaculture.2020.735236
Ward T., Larson J., Meulemans J., Hillmann B., Lynch J., Sidiropoulos D., et al. (2017). BugBase predicts organism-level microbiome phenotypes. Biorxiv, 133462. doi: 10.1101/133462
Wu Y., Li R., Shen G., Huang F., Yang Q., Tan B., et al. (2021b). Effects of dietary small peptides on growth, antioxidant capacity, nonspecific immunity and ingut microflora structure of Litopenaeus vannamei. J. Guangdong Ocean Univ. 41 (5), 1–9. doi: 10.3969/j.issn.1673-9159.2021.05.001
Wu T., Li G., Shi S., Wu Z., Zhu C. (2008). Effects of cadmium stress on NOS and SOD activities in Litopeneaus vannamei. J. Trop. Oceanogr. 27 (6), 62–65. doi: 10.3969/j.issn.1009-5470.2008.06.011
Wu Y., Wang Y., Ren X., Huang D., Si G., Chen J. (2021a). Replacement of fish meal with gamma-ray irradiated soybean meal in the diets of largemouth bass Micropterus salmoides. Aquacult. Nutr. 27, 977–985. doi: 10.1111/anu.13239
Wu J., Xiong J., Wang X., Qiu Q., Zheng J., Zhang D. (2016). Intestinal bacterial community is indicative for the healthy status of Litopenaeus vannamei. Chin. J. Appl. Ecol. 27 (2), 611–621. doi: 10.13287/j.1001-9332.201602.039
Xu D., He G., Mai K., Zhou H., Xu W., Song F. (2016). Postprandial nutrient-sensing and metabolic responses after partial dietary fishmeal replacement by soyabean meal in turbot (Scophthalmus maximus l.). Br. J. Nutr. 115, 379–388. doi: 10.1017/S0007114515004535
Xu T., Xu D., Liu X., Zhao W. (2021). Effect of dehulled soybean meal instead of fish meal on growth and body composition of Litopenaeus vannamei. Feed Res. 13, 72–76. doi: 10.13557/j.cnki.issn1002-2813.2021.13.018
Yang P., Hu H., Liu Y., Li Y., Ai Q., Xu W., et al. (2018). Dietary stachyose altered the intestinal microbiota profile and improved the intestinal mucosal barrier function of juvenile turbot, Scophthalmus maximus l. Aquaculture 486, 98–106. doi: 10.1016/j.aquaculture.2017.12.014
Yang Q., Yan B., Dong X., Chi S., Liu H. (2015). Effect of replacing fish meal with extruded soybean meal on growth, feed utilization and apparent nutrient digestibility of juvenile white shrimp (Litopenaeus vannamei). J. Ocean Univ. China 14 (5), 865–872. doi: 10.1007/s11802-015-2572-6
Yi Y., Yu R., Shi G., Ma X., Xiao S., Shui J., et al. (2021). Analysis of the composition and phenotypes of intestinal flora in primary bacteriologically-confirmed pulmonary tuberculosis patients based on high-throughput sequencing of 16S rRNA V4 region. Chin. J. Antituberc. 43 (9), 939–946. doi: 10.3969/j.issn.1000-6621.2021.09.014
Yin B. (2019). Effects of plant protein sources replacing fishmeal on growth performance, intestinal microflora and immunity of juvenile hybrid grouper (Epinephelus fuscoguttatus ♀ × e. lanceolatu ♂). master thesis (Zhanjiang, China: Guangdong Ocean University).
Yu W., Dai W., Tao Z., Xiong J. (2018). Characterizing the compositional and functional structures of intestinal microflora between healthy and diseased Litopenaeus vannamei. J. Fish. China 42 (3), 399–409. doi: 10.11964/jfc.20170310737
Yun H., Shahkar E., Hamidoghli A., Lee S., Won S., Bai S. C. (2017). Evaluation of dietary soybean meal as fish meal replacer for juvenile whiteleg shrimp, Litopenaeus vannamei reared in biofloc system. Int. Aquat. Res. 9, 11–24. doi: 10.1007/s40071-017-0152-7
Zhang X., Han P., Hu J., Liu L., Chen Y., Xu W., et al. (2020). Effects of replacement of fish meal by soybean meal on growth performance, protein utilization and intestinal tissue morphology of Channa argus. Chin. J. Anim. Nutr. 32 (6), 2799–2807. doi: 10.3969/j.issn.1006-267x.2020.06.040
Zhang F., Zhang W., Mai K., Sun R. (2012). Effects of replacement of dietary fish meal by soybean meal on growth, digestive enzyme activity and digestive tract histology of juvenile large yellow croaker, Pseudosciaena crocea r. Periodical Ocean Univ. China 42, 75–82. doi: 10.16441/j.cnki.hdxb.2012.s1.011
Zhao X., Wang Y., Wang X., Ye J. (2021a). Growth performance, plasma components, and intestinal barrier in grouper (Epinephelus coioides) are altered by dietary fish meal replacement with extruded soybean meal. Aquacult. Rep. 21, 100863. doi: 10.1016/j.aqrep.2021.100863
Zhao X., Zheng H., Liu M., Tang Y., Qiao X., Liu Q., et al. (2021b). Effects of dietary fish meal replaced by soybean meal and supplemented with different organic acids on growth, immunity and intestinal microflora of pacific white leg shrimp Litopenaeus vannamei. J. Dalian Ocean Univ. 36 (6), 966–975. doi: 10.16535/j.cnki.dlhyxb.2021-007
Zhu Y., Shen L., Guo R., Jing B., Liang B., Gao J. (2022). Effects of partial fish meal substitution by fish peptide and protease on growth, intestinal digestive enzymes and intestinal microflora of pacific white shrimp Litopenaeus vannamei. J. Dalian Ocean Univ. 37 (5), 756–763. doi: 10.16535/j.cnki.dlhyxb.2021-194
Keywords: Litopenaeus vannamei, soybean meal, fish meal, hepatopancreas health, intestinal microbiota
Citation: Peng K, Qiu J, Li C, Lu H, Liu Z, Liu D and Huang W (2023) A multi-angle analysis of injury induced by supplementation of soybean meal in Litopenaeus vannamei diets. Front. Microbiomes 2:1113635. doi: 10.3389/frmbi.2023.1113635
Received: 01 December 2022; Accepted: 20 February 2023;
Published: 20 March 2023.
Edited by:
Tiffany L. Weir, Colorado State University, United StatesReviewed by:
George Seghal Kiran, Pondicherry University, IndiaShuang Zhang, Guangdong Ocean University, China
Copyright © 2023 Peng, Qiu, Li, Lu, Liu, Liu and Huang. This is an open-access article distributed under the terms of the Creative Commons Attribution License (CC BY). The use, distribution or reproduction in other forums is permitted, provided the original author(s) and the copyright owner(s) are credited and that the original publication in this journal is cited, in accordance with accepted academic practice. No use, distribution or reproduction is permitted which does not comply with these terms.
*Correspondence: Wen Huang, aHVhbmd3ZW41NDlAMTI2LmNvbQ==