- 1Department of Clinical Sciences, Hematology, Faculty of Medicine, Titu Maiorescu University of Bucharest, Bucharest, Romania
- 2Department of Hematology, Colentina Clinical Hospital, Bucharest, Romania
Immune thrombocytopenia (ITP) manifests as depleted platelet reserves, primarily due to the immune-mediated destruction of platelets. The pathogenesis of ITP is complex and involves dysregulation of the immune system. This review aimed to summarize the current knowledge of the cytokine profile in ITP and its potential implications for diagnosis, treatment, and prognosis. Several studies have reported that ITP patients have an altered cytokine profile from that of healthy individuals. Specifically, there is evidence of an imbalance of pro-inflammatory (interleukin (IL)-6, tumor necrosis factor (TNF)-α, interferon (IFN)-γ) and anti-inflammatory cytokines (IL-10, TGF-β). The cytokine profile in ITP appears to be heterogeneous, with different patterns observed in different subsets of patients. For example, some studies have reported a Th1-type cytokine profile, characterized by elevated levels of IFN-γ and TNF-α, while others have reported a Th2-type cytokine profile, characterized by elevated levels of IL-4 and IL-10. There is also evidence of a shift from a Th1 to a Th2 cytokine profile in some patients over time. The cytokine profile in ITP may have important implications for diagnosis, treatment, and prognosis. Targeting specific cytokines or cytokine pathways may also represent a promising therapeutic approach for ITP. Further studies are needed to better understand the heterogeneity of the cytokine profile in ITP and its potential implications for clinical management.
1 Introduction
Immune thrombocytopenia (ITP) is a type of autoimmune disorder that involves the destruction of platelets and their precursor cells, megakaryocytes (1, 2). This not only results in low-circulating platelets but also impairs the production of new platelets (3, 4). Previously, this disorder was referred to as idiopathic thrombocytopenic purpura due to the unknown origin of the disease. However, this term is no longer used since it has been determined that the underlying cause involves immune dysfunction. The incidence rate of ITP is 16–27 new cases per million annually, with a greater propensity observed in the female population (5, 6). Patients presenting with a platelet count below 100,000 platelets per microliter are typically diagnosed with primary ITP (7). Secondary ITP, which accounts for 20% of ITP cases, is induced by various factors such as autoimmune disorders, infections, or drug treatments (8). Based on duration, ITP can be classified as newly diagnosed (up to 3 months), persistent (up to 12 months), or chronic (longer than 12 months). In adults, 80% of ITP cases are chronic in nature, characterized by recurring episodes, and often exhibit resistance to therapeutic interventions (9).
Although platelet destruction mediated by autoantibodies has traditionally been considered the primary pathophysiological mechanism, the underlying mechanisms that trigger the development of autoimmunity against platelets remains unclear (10). Antiplatelet antibodies lead platelet destruction by targeting platelet surface antigens. These antibodies are mainly directed against glycoproteins on the platelet surface, such as glycoprotein IIb/IIIa (GP IIb/IIIa) and glycoprotein Ib/IX (GP Ib/IX). The presence of these antibodies in ITP leads to several mechanisms that contribute to platelet destruction, including opsonization, Fc receptor-mediated clearance, antibody-mediated cellular cytotoxicity (ADCC), and complement system activation (11). A study by Iraqi et al. reported that antiplatelet autoantibodies inhibit proplatelet formation by megakaryocytes and impair platelet production in vitro (12).
Initially, Harrington and Hollingsworth proposed that ITP was caused by plasma factor circulating in the blood, which was later identified as immunoglobulin type G (IgG) specific to platelets (13). Furthermore, in roughly two-thirds of ITP cases, a deficiency of endogenous TPO (eTPO) is observed (14). The deficit of eTPO is due to functional impairment rather than compensatory increase, as seen in megakaryocytic thrombocytopenia.
In addition to autoantibodies, T lymphocytes and cytokine imbalances have also been implicated in the pathogenesis of ITP (15). Elevated numbers of T-cell receptor (TCR) g/d-positive T lymphocytes have been reported in chronic ITP patients (16), and elevated levels of soluble interleukin (IL)-2 receptors (sIL-2R) have been observed in serum (17). Several studies have documented the existence of stimulated T cells that are reactive to platelets in patients with ITP. These patients show a disturbance in the cytokine balance, which favors the production of IL-2 and interferon (IFN)-γ (6, 18). Furthermore, helper T lymphocytes polarize into Th1 and Th2 immune responses and are involved in the aberrant immune responses seen in ITP (19, 20). Figure 1 summarizes multiple factors involved in the pathogenesis of ITP.
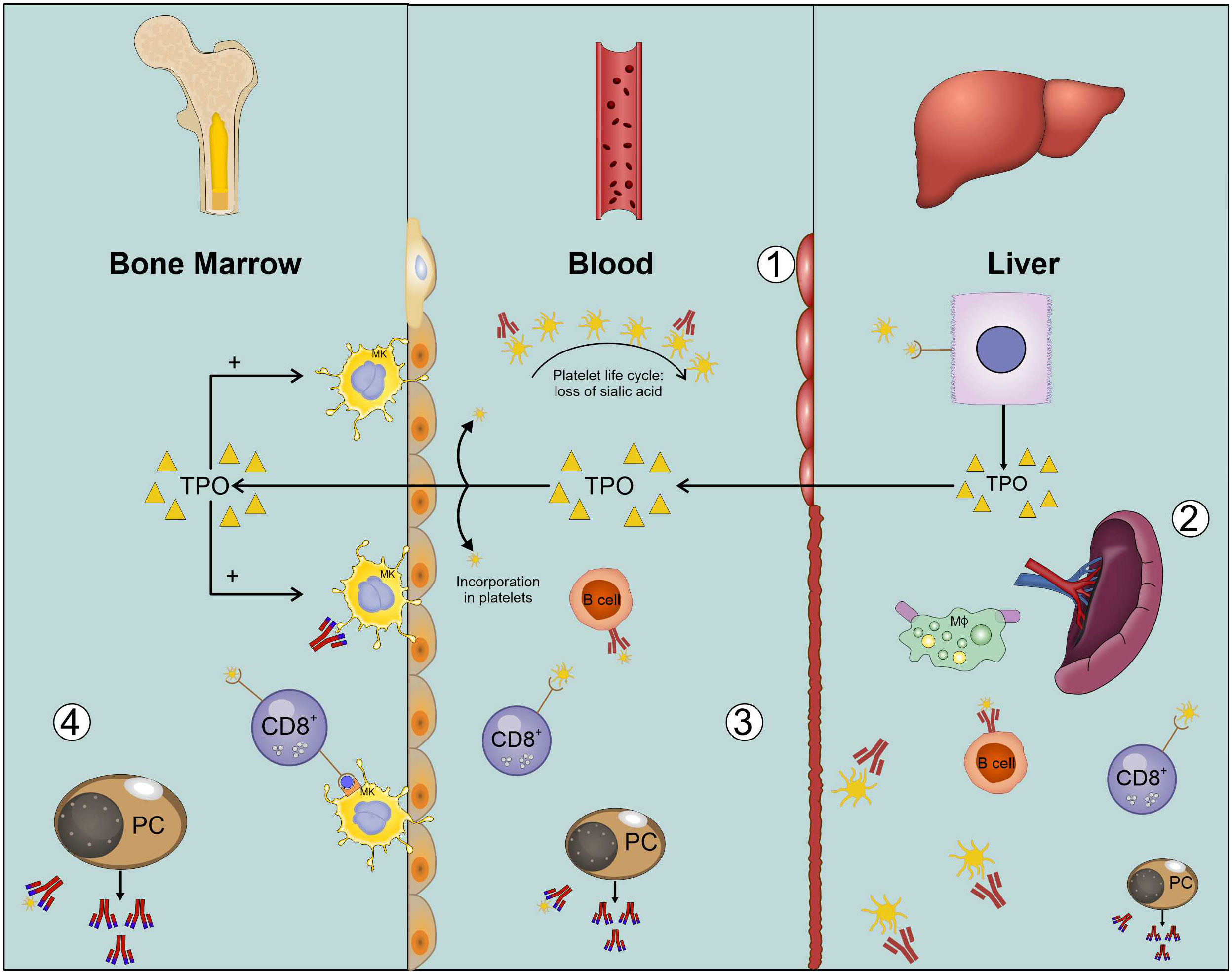
Figure 1 Platelet lifecycle explained in ITP. (1) Platelets (yellow) are produced in bone marrow. When platelets age, they lose terminal sialic acid (curved arrow). Ultimately, they are cleared in the liver. Platelet clearing also leads to the formation of new TPO (yellow triangles). (2) Macrophages (green) can also phagocytose platelets whereas cytotoxic cells (blue) also lyze platelets. (3) In blood, the autoimmune response is carried out by cytotoxic T cells. Apart from this, platelet-reactive B cells (red) are also present in blood, which enhances platelet clearance. Endothelial cells are shown on the sides of central column in the figure. (4) Cytotoxic T cells and plasma cells in bone marrow inhibit platelet production.
In recent years, there has been an increasing focus on investigating the cytokine profile in ITP and its potential impact on disease pathogenesis and management. This review seeks to address unresolved issues regarding the involvement of cytokines in the progression of ITP. The aim is to contribute to the decision-making process of clinicians regarding the appropriate form of treatment.
2 Normal immune system physiology
B and T lymphocytes play a critical role in the adaptive immune response. Through recognition of specific antigens, B lymphocytes undergo differentiation into plasma cells and memory B cells, generating a humoral response (21). Plasma cells produce IgG antibodies that neutralize bacterial toxins and viruses. Regulatory B lymphocytes, known as Bregs, secrete anti-inflammatory cytokines (IL-10) to curb T-cell and monocyte activation and other autoimmune responses (22).
T lymphocytes undergo thymic maturation and generate cellular responses by recognizing antigens on major histocompatibility complex (MHC) molecules on the surface of antigen-presenting cells (APCs). Furthermore, T-cell precursors differentiate into effector T cells and memory T cells, which can be CTL CD8+, Th1 CD4+, or Th2 CD4+ (23). The effector response of Th1 and Th2 involves the secretion of various growth factors and interleukins, with Th1 mainly associated with inflammation and Th2 with the allergic response (24). Regulatory T cells (Tregs) maintain immune homeostasis by suppressing autoreactivity in the peripheral blood. Tregs exert their suppressive effects on cellular and humoral immune responses by directly inhibiting the function of CTLs and Th cells. This is achieved through direct interaction with B cells or by the secretion of specific cytokines (IL-10, TGF-β) (25). Tregs express CD4 and CD25, and they can inhibit CD4+CD25- and CD8+ lymphocytes, thereby contributing to immune tolerance (25). While effector T cells can also express CD25, Foxp3 is a specific marker for Tregs.
The humoral and cellular immune responses are interconnected as T-helper (Th) cells stimulate both T and B cells. While Th cells can activate T and B cells, T-cell-independent responses are also prevalent in B cells. To prevent the recognition of self-antigens, the immune system employs various mechanisms (24, 25). One such mechanism occurs in the thymus and bone marrow, where immature lymphocytes encounter self-antigens displayed by APCs (26). T cells bearing TCRs with low or intermediate affinity for MHC molecules undergo positive selection and mature. In contrast, those with high affinity undergo negative selection and apoptosis. The peripheral mechanism entails Treg cells that inactivate circulating autoreactive lymphocytes (27). Furthermore, B cells can remove T cells with TCRs for the same antigens, thus preventing the generation of autoantibodies. Additionally, Treg and Breg (regulatory B cells) lymphocytes employ bidirectional control mechanisms between humoral and cellular responses (28).
3 Dysregulation of the immune system observed in immune thrombocytopenia
In ITP, autoantibodies are undetectable in approximately 25% of patients (29). In addition to the presence of autoantibodies that target platelets, immune system alterations involving T cells have been observed in individuals with ITP. Specifically, platelet destruction is mediated by cytotoxic T lymphocytes and Th1 cells, while the functionality of Th1 and regulatory T cells is altered. These changes are linked to the expansion of autoreactive B cell clones (20). Autoreactive B cells produce IgG autoantibodies directed against the glycoproteins (GPs) of platelet membranes, which facilitate the binding of phagocytes that destroy platelets. These GPs are also present in megakaryocytes (30). Genetic susceptibility may also be involved in ITP development. For instance, multiple polymorphisms like MHC HLAB8DR3 and the IL-1 receptor antagonist have been associated with the onset of ITP (31, 32).
Recent research has demonstrated that platelets exhibit the expression of toll-like receptors (TLRs) and are capable of presenting antigens via MHC-II (33). These TLRs serve as pattern recognition receptors and have the ability to recognize various pathogen-associated molecular patterns (PAMPs). TLR recognition of molecules from injured tissues may contribute to the pathogenesis of ITP. Several mechanisms have been proposed to explain the spontaneous resolution of ITP in some patients after treatment with antibacterial agents. One such mechanism involves the inactivation of TLR-mediated destruction, which is implicated in the pathogenesis of ITP (34).
Abnormalities in B lymphocytes have also been observed in patients with ITP. Increases in splenic B lymphocytes have been noted, while decreases in Bregs have been observed in untreated patients with ITP (35). Bregs play an important role in modulating the immune response; hence, alterations in their function may contribute to the disruption of Tregs observed in ITP (35). Figure 2 summarizes the multiple cellular mechanisms of ITP pathogenesis.
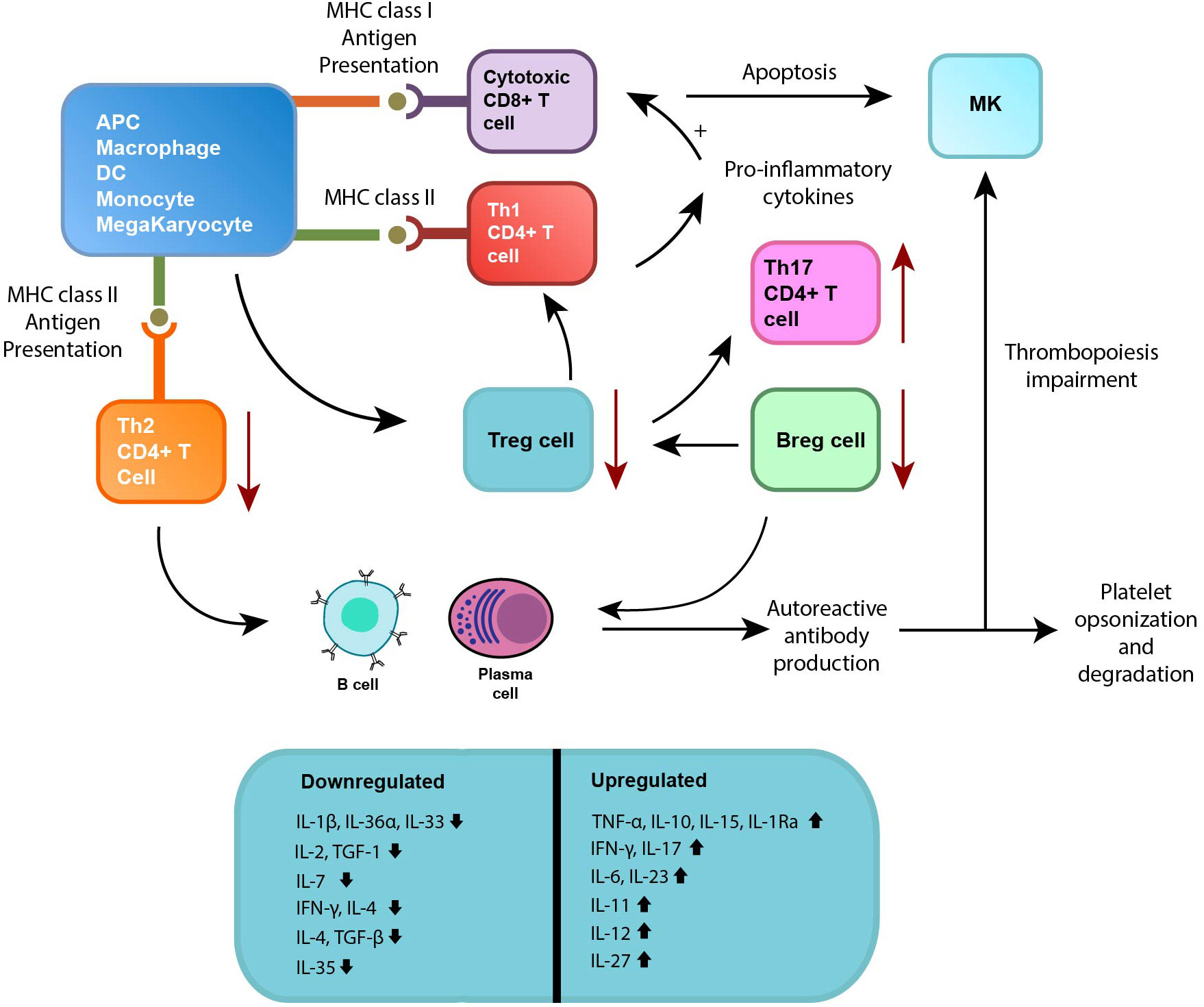
Figure 2 Several cellular pathogenic mechanisms and cytokines involved in ITP. Abnormal regulation of B cells and plasma cells (displayed at the bottom) plays a significant role, as they produce autoantibodies that bind to platelets and megakaryocytes (MKs). This interaction leads to impaired function and degradation of platelets and MKs. The cellular immune response in ITP is also affected, resulting in a decrease in regulatory T cells (Tregs) and regulatory B cells (Bregs) (green box). This reduction further promotes the survival of autoreactive plasma cells, which, in turn, enhances autoantibody production. Additionally, there is an imbalance observed in the subsets of CD4+ T cells, specifically the helper T cells (Th cells). Furthermore, cytotoxic CD8+ T cells become activated in ITP, causing the apoptosis (cell death) of platelets and MKs. This activation also leads to dysregulation of the bone marrow (BM) niche homeostasis, disrupting the process of megakaryopoiesis (formation of MKs) and thrombopoiesis (production of platelets). Interleukins such as IL-4, IL-7, TGF- β are downregulated whereas interleukins such as IL-6, IL-11, and IL-22 are upregulated.
4 Changes in T lymphocytes response
ITP results in changes in T-cell populations, such as an increase in Th1 cells relative to Th2 cells, increased Th17 cells, and a decrease in Treg cells. In patients with active ITP, there is an imbalance in the Th1/Th2 response, with an increase in pro-inflammatory CD16+ monocytes that release TNF, promoting Th1 development and IL-17 release, and suppressing Tregs (36). Th1 cells produce cytokines that initiate the cellular response, including IL-2, IFN-γ, and TNF-β. Furthermore, higher levels of IL-23 and TLR4 have been reported in monocytes (37).
Cytotoxic T lymphocytes (CTLs) are crucial in the immune response against tumors, bacteria, and viruses, inducing apoptosis in targeted cells (38, 39). Dendritic cells stimulated by tumor cell-derived exosomes enhance CD8+ CTL responses, leading to improved anti-tumor immunity. CD4+ T cells indirectly aid CTL activation by modifying antigen-presenting cells, playing a significant role in the initiation and persistence of CD8+ T-cell responses. CD4+ helper T cells play a direct role in stimulating CD8+ CTL responses. These CTLs also contribute to the process of platelet lysis, which is a key factor in the development of ITP (40).
Tregs are critical in maintaining peripheral immune tolerance (41). However, patients with ITP may have decreased Tregs or defects in their suppressive functions (42). Research findings demonstrate the generation of platelet glycoprotein-specific Tregs from non-regulatory T cells in patients with ITP. These cells possess the ability to induce antigen-specific immune suppression (43).
5 The role of the Th1/Th2 imbalance in immune thrombocytopenia
The balance between Th1 and Th2 cells is crucial for normal human immunity, with Th1 cells being responsible for inflammatory responses and cellular immunity while Th2 cells stimulate B cell proliferation (44). Aberrant activation of Th1 lymphocytes may contribute to the development of ITP. Although the underlying cause of Th1 dominance in ITP is not fully understood, uncontrolled Th1 lymphocyte activation is believed to be a crucial mechanism (45). The imbalance between Th1/Th2 in ITP yields conflicting evidence in various studies, with some studies reporting more involvement of Th1 and its related cytokines whereas other lean toward Th2. The Th1 response is characterized by the secretion of cytokines such as IL-2, INF-γ, and TNF-α, while the Th2 response produces cytokines such as IL-4, IL-5, IL-6, IL-10, and IL-13. Although the primary distinction between human Th1 and Th2 subsets is based on IFN-γ and IL-4 production, recent research on gene polymorphisms suggests their contribution to the pathogenesis of ITP (20, 46). Studies have demonstrated that the Th1/Th2 ratio is substantially higher in ITP patients than in healthy individuals, indicating a potential role for Th1/Th2 polarization in the pathogenesis of ITP (37). However, some studies suggest that Th2 polarization may be more relevant since the Th1/Th2 ratio is directly associated with platelet counts (47). Treatment with dexamethasone, a steroid hormone, can rebalance the Th1/Th2 axis by increasing the levels of anti-inflammatory cytokines such as IL-4, IL-10, and TGF-β (48). Additionally, IL-18, a cytokine that promotes Th1 responses, is elevated in ITP patients, along with IFN-γ, a cytokine produced by T lymphocytes and natural killer cells that are stimulated by IL-18. The balance between IL-18 and its inhibitor, IL-18BP, is involved in the progression of ITP, with high levels of IL-18 and low levels of IL-18BP positively correlated with Th1 bias (49). The imbalance of Th1/Th2 observed in ITP varies based on the stage of the disease. In the acute phase, there is an upregulation of Th1 immune responses. Th1 cells secrete pro-inflammatory cytokines such as IFN-γ and TNF-α. These cytokines contribute to the activation of macrophages and cytotoxic T cells, promoting platelet destruction. Furthermore, there is decrease in Th2 cytokines (20). In the persistent stage of ITP, there is a shift toward a mixed Th1/Th2 immune response. While Th1 responses remain active, there is also an increase in Th2 cytokine production (50). In some cases, as ITP progresses to the chronic or persistent stage, there can be a rebalancing of Th1 and Th2 responses. Th2 cytokines, particularly IL-4 and IL-10, may become more prominent in the chronic stage. IL-4 can inhibit Th1 responses and promote B cell differentiation, leading to the production of anti-inflammatory antibodies (47) (Table 1).
6 Role of cytokines in immune thrombocytopenia
T helper cells are a subset of immune cells that can be categorized based on their production of cytokines, with Th1, Th2, and Th17 cells being the most commonly studied. Th1 cells are responsible for cellular immunity and produce cytokines such as interferon and interleukin-2, while Th2 cells promote antibody production by producing cytokines such as IL-4, IL-5, and IL-13 (69). Although ITP was previously thought to be primarily associated with a Th1 immune response, recent research has suggested that a less pronounced Th1 response may be involved, and, in some cases, a Th2 response may be present.
6.1 Interleukin-17
Although IL-17 does not directly influence the differentiation of Th1 cells, its involvement is crucial for the development of a Th1-type immune response (70). IL-17 induces the expression of several cytokines and chemokines, including IL-6, TGF-b, matrix metalloproteinase, and intercellular adhesion molecule-1 in various cell types such as BM stromal cells. These cells, in turn, maintain an inflammatory cytokine milieu (71). A study by Okamoto et al. reported that individuals with ITP had a higher abundance of cells expressing specific markers, namely CD68, CD163, and IL-17, when compared with healthy control subjects (72). Furthermore, the expression levels of CD68 and CD163 exhibited a positive correlation with IL-17 expression in these patients (72).
Similarly, another study by Stimpson et al. reported that the ratio of IL-10 to IL-17 expression in CD4+ T-cell culture showed discriminatory potential between ITP patients who had complete, partial, or non-response to corticosteroid treatment (P=0.002) (64). Zhou et al. showed that Th17-associated pro-inflammatory cytokines such as IL-17A/F, IL-6, and IL-23 were elevated in ITP patients, whereas the levels of inflammatory inhibitory factors, including IL-10 and transforming growth factor-b, were decreased (73)
In a study conducted by Ye et al., it was observed that patients with ITP exhibit increased mRNA expression levels of several cytokines, including IL-23p19, IL-12p40, IL-23R, IL-12Rβ1, IL-17A, IL-17F, and RORC (62). Additionally, these patients displayed elevated levels of Th17 cells and plasma IL-17 and IL-23 and a negative correlation with platelet count (62).
6.2 Interleukin-6
IL-6 plays a crucial role in the regulation of megakaryocytopoiesis and platelet activity. During thrombocytopenia, an elevated IL-6 concentration may contribute to compensatory megakaryocytopoiesis and increased residual platelet function in patients with ITP (74). A cross-sectional case–control study by Fatma et al. showed that IL-6 levels were significantly higher in acute ITP patients than in chronic ITP and control patients (56). There were also significant positive correlations observed between IgM, IgG, and platelet counts, as well as a significant negative correlation between both TNF-α and IL-6 levels and platelet counts (56). Li et al. found that the percentages of CD8(+) T lymphocytes and CD19(+) B lymphocytes were significantly higher in ITP patients than in healthy controls. The serum levels of IL-4, IL-6, IL-11, IL-17, and TPO were significantly higher in ITP patients than in healthy controls, while TGFβ level was significantly decreased (59).
6.3 Interleukin-7
IL-7 is a cytokine belonging to the IL-2 family that is synthesized by bone marrow stromal and epithelial cells (75). Its receptor is composed of two chains: IL-7R alpha (CD127) and gamma chain (CD132), which are expressed at high levels on quiescent T cells, except for CD4+CD25+ Tregs (76). IL-7 has a crucial role in T-cell development and survival by promoting the survival of early thymocytes and expanding T-cell precursors, modulating T-cell development and selection in the thymus. Additionally, IL-7 can stimulate T-cell immune functions by promoting the production of Th1 cytokines such as IL-2, interferon-γ (IFN-γ), and tumor necrosis factor-α (TNF-α), while inducing Th2 cytokines IL-4 and IL-5 to a lesser extent (76).
Li et al. reported that the levels of plasma IL-7 were lower in patients with ITP than in healthy individuals (57). The levels of IL-7 in bone marrow, however, did not differ between ITP patients and healthy controls. The study found a positive correlation between plasma IL-7 levels and platelet counts (57).
Based on the known role of IL-7 in apoptosis resistance and the stimulation of pro-inflammatory cytokines, it can be speculated that the observed decrease in plasma IL-7 levels in ITP patients may be a result of negative feedback on the pro-inflammatory function in these individuals (57).
6.4 Interleukin-9
Recently, Th9 cells have been identified as a distinct subset of T helper cells that possess the unique ability to selectively produce IL-9. The pleiotropic effects of IL-9 suggest that Th9 cells may play a critical role in the pathogenesis of various autoimmune diseases (77).
Qiao et al. conducted a research study to explore the potential role of Th9 and Th17 cells in individuals with ITP (58). The study cohort included 49 ITP patients with active symptoms, 39 ITP patients in remission, and 20 healthy individuals as a control group. The findings of the research revealed significantly elevated levels of Th9 cells and the cytokine IL-9 in active ITP patients. Of note, a positive correlation was observed between Th9 and Th17 cells, as well as between the plasma levels of IL-9 and IL-17 in ITP patients (58).
Similarly, in a study conducted by Goelz et al., it was observed that individuals with acute ITP exhibited significantly increased plasma levels of both pro-inflammatory (such as tumor necrosis factor-α (TNF-α) and IL-15) and anti-inflammatory (such as IL-1 receptor antagonist (Ra), IL-10, and interferon γ-induced protein (IP-10)) cytokines when compared with healthy controls (53).
6.5 Interleukin-10
IL-10 is a vital cytokine involved in the regulation of immune responses by acting as an anti-inflammatory agent (78). Its primary source is a specific subset of CD4 lymphocytes (79). The IL-10 gene is situated on chromosome 1 and harbors promoter point mutations that may have an impact on IL-10 expression levels. This, in turn, has the potential to influence the development and progression of autoimmune diseases (80). Inflammatory conditions require regulation by IL-10-producing Tregs to effectively control several autoimmune diseases (81).
Li et al. conducted a study on newly diagnosed ITP patients and healthy individuals to investigate the peripheral Th cell subsets and Tregs (82). The findings indicate that patients with ITP exhibit heightened activation of effector T cells (Teffs), coupled with a reduction in the quantity and functionality of Tregs. The levels of IL-10 in the cultured supernatant were lower in ITP patients but increased in patients who experienced remission (82).
Soliman et al. found that individuals with specific genotypes of the IL-10 promoter (1082 AA and 592 AA) have lower levels of IL-10 and altered immune cell profiles (lower CD4, T-reg, CD4/CD8). Importantly, IL-10 serum levels and genotype frequencies were not different between controls and individuals with ITP. Additionally, lower levels of T-reg cells were associated with a higher likelihood of developing chronic ITP (83).
According to the research conducted by Goelz et al., their findings demonstrate a significant increase in the cytokines TNF-α, IFN-γ, IL-6, IL-10, and IL-13 among patients with immune thrombocytopenia (ITP) during their initial diagnosis (63).
Goelz et al. reported that in acute ITP patients, both pro-inflammatory (TNF-α, IL-15) and anti-inflammatory (IL-1 receptor antagonist (Ra), IL-10, and interferon γ-induced protein (IP-10)) cytokines were found to be significantly elevated (53). Ma et al. conducted a study that revealed that the levels of Th2 cytokines (IL-4 and IL-10) were considerably higher in patients with ITP than in patients in the control group (P<0.05) (54). In contrast, the levels of Th1 cytokines (IFN-γ, IL-2), Treg cytokine (TGF-β1), and Th17 cytokine (IL-17) were lower in ITP patients (P<0.05) (54).
Zhang et al. reported that the levels of IFN-γ, TGF-β1, IL-4, and IL-10 cytokines were significantly reduced in mice with ITP (55). However, there was no significant increase in the secretion of pro-inflammatory cytokines IL-2 and IL-17A or in the proportion of Th17 cells. The frequency of Treg cells and the mRNA expression of Foxp3 were significantly decreased in splenocytes of ITP mice (55).
6.6 Interleukin-12
IL-12 is a Th1 cytokine that is primarily produced by cells of the innate immune system. It plays a critical role in the differentiation of naive T cells into Th1 cells. A study by Li et al. investigated the role of imbalanced plasmacytoid dendritic cells (pDCs) and myeloid dendritic cells (mDCs) in regulating the balance of CD4+ T-cell subsets in ITP (47). The study results showed that ITP patients had higher levels of IL-6, IL-12, and IL-23 than those of healthy controls. Additionally, the levels of Th1 and Th17 cells in ITP patients increased, along with increased levels of IFN-γ and IL-17, while the proportion of Th2 and Treg cells decreased, along with decreased levels of IL-4 and transforming growth factor beta (TGF-β). Furthermore, Pearson correlation analysis showed a positive correlation between the proportion of total DCs and IL-12/IL-23 in ITP patients. The study also found that the Th1/Th2 ratio and IFN-γ and IL-12 levels were negatively correlated with platelet counts, whereas IL-23 was positively correlated with IL-17 and negatively correlated with platelet count (47). Another study examined the expression of cytokines belonging to the IL-12 family, including IL-12, IL-23, IL-27, and IL-35, as well as their relevant cytokines such as IFN-γ, IL-4, IL-17A, and IL-10, in patients with chronic ITP (84). The study found significantly higher levels of IL-12p70, IL-23, IL-27, IFN-γ, and IL-17A in the plasma of cITP patients than in that of control subjects (p<0.01) (84).
Li et al. conducted a study to investigate the role of Th1 polarization and upregulation of Th17 cells in patients with ITP (66). The study involved 46 patients with ITP and 22 healthy individuals as controls. The results showed that the expression of mRNA for IL-12 p40, IL-12 p35, and IL-23 p19 was significantly higher in bone marrow mononuclear cells than in controls. Additionally, the levels of IL-12 and IL-23 were higher in the bone marrow plasma and peripheral blood plasma of ITP patients than in controls (66).
6.7 Interleukin-21
IL-21 is a pleiotropic cytokine that exerts its effects on a diverse array of lymphoid, myeloid, and epithelial cell populations. One of the significant actions of IL-21 is its ability to impede the maturation and functionality of dendritic cells derived from the bone marrow (BMDCs) (85). Zhang et al. found that individuals with ITP have significantly higher expressions of IL-21 on mononuclear cells than healthy individuals (65). Specifically, the ratio of IL-21 to GAPDH mRNA expression on mononuclear cells was significantly higher in ITP patients (9.524 ± 0.97) than in healthy controls (3.701 ± 0.60, P<0.01) (65).
6.8 Interleukin-22
IL-22 has both pro-inflammatory and anti-inflammatory properties and is primarily synthesized by T cells and natural killer (NK) cells (84). In a study conducted by Liu and Liu, a cohort of 110 patients with ITP was recruited, consisting of 55 individuals with active ITP, 55 in remission from ITP, and 55 healthy controls (66). The study revealed that IL-22 serum levels were significantly higher in patients with active ITP than in those in both the remission group and the control group (p < 0.05) (66). Similar findings were reported in a review by Azizi et al. who found that Th22 and IL-22 were increased in ITP (84).
6.9 Interleukin-27
Recent scientific investigations have demonstrated that IL-27 displays immunosuppressive properties through the modulation of T-cell differentiation and the dampening of inflammatory responses in autoimmune disorders, such as rheumatoid arthritis (86) and systemic lupus erythematosus (87). Reduced expression of IL-27 has been found to be associated with increased cytotoxic T lymphocyte-mediated platelet destruction in patients with ITP (88). IL-27 has been discovered to play a regulatory role in the suppression of acquired immunity by stimulating the development of T helper cells and inducing the expansion of inducible regulatory T cells that produce IL-10 (66).
In the study conducted by Hassan et al., it was found that patients with ITP had significantly higher levels of IL-27 than healthy controls (67). Among the patient groups, those with acute ITP had the highest levels of IL-27, while those in remission had the lowest levels. Furthermore, patients who received intravenous immunoglobulin (IVIG) or combined steroid and IVIG therapy had significantly higher levels of IL-27 than other treatment groups (67). Similar findings were shared by Gad Allah et al., who reported higher levels of IL-27 in ITP patients than the controls (p <0.001) (89).
6.10 Interleukin-33
IL-33, classified as a Th2-oriented cytokine, exhibits the ability to augment the production of Th2 cytokines such as IL-5 and IL-13. Furthermore, it can function as both a conventional cytokine and a nuclear factor involved in regulating gene transcription (90). Li et al. conducted a study to investigate the levels of IL-33 and sST2 in patients with active ITP compared with those with ITP in remission and normal controls. The study found a significant decrease in IL-33 levels in the plasma of patients with active ITP, indicating the downregulation of IL-33 in this patient group. Additionally, the sST2 level was significantly upregulated in patients with active ITP compared with those in remission and normal controls (52). A study by Zhan et al. demonstrated that the levels of certain interleukins (IL-1β, IL-36α, IL-36γ, and IL-33) were downregulated in patients with ITP compared with healthy individuals (51). The study also showed a positive correlation between the platelet count and the level of IL-37 in ITP patients (51). In another study by Li et al., it was observed that individuals with active ITP had a significant decrease in plasma IL-33 levels (p < 0.01) (52). Additionally, soluble ST2 (sST2) levels were upregulated (p < 0.01) in individuals with active ITP when compared with normal controls (52).
6.11 Interleukin-35
IL-35 is a recently identified cytokine that is classified as a member of the IL-12 family. It is mainly produced by Bregs, DCs, NK cells, and tumor-associated macrophages (TAMs) (91). Sun et al. reported that individuals with active ITP had significantly lower levels of IL-35 in their plasma (10.27 ± 0.527 pg/mL) than in individuals in remission (26.00 ± 3.372 pg/mL) and healthy controls (48.96 ± 3.615 pg/mL). The researchers also observed that individuals in remission had lower plasma levels of IL-35 than healthy controls (p < 0.05) (68).
7 Expression of human leukocyte antigen in immune thrombocytopenia and their association with cytokines
Aberrant expression of self-molecules, such as HLA-DR, on immune-targeted tissues can lead to the development of autoimmune diseases by activating autoreactive T lymphocytes and producing autoantibodies (92). For instance, abnormal HLA-DR expression on pancreatic islet cells and myelin has been associated with type I diabetes (93) and multiple sclerosis (94), respectively. The induction of transient HLA-DR expression by cytokines or inflammatory mechanisms can enhance the immune response or direct a tissue-specific immune response. In the case of ITP, HLA-DR expression is observed in various GPIb+ cell populations expressing different molecules, including CD41, CD45, CD14, CD80, and glycophorin (95). Patients with chronic ITP display high HLA-DR expressions on platelet-isolated spleen cells, and splenic T cells show a high level of in vitro platelet-stimulated IL-2 secretion (95). Semple et al. found that compared with controls, children with chronic ITP exhibit increased serum levels of IL-2, IFN-γ, and IL-10, while none of the patients had detectable serum levels of IL-4 or IL-6 (96). The expression of HLA-DR on platelets shows an inverse correlation with platelet count, but not with serum cytokines, suggesting that platelet HLA-DR expression is a common phenomenon in patients with ITP (96).
HLA-G is a non-classical major histocompatibility complex class I antigen that exhibits potent immune-inhibitory functions. It has been demonstrated to benefit patients with allotransplantation or autoimmune diseases by engaging inhibitory receptors, such as ILT-2/LILRB1/CD85j, ILT-4/LILRB2/CD85d, and KIR2DL4, on immune cells, thereby inducing apoptosis of cytotoxic T cells, immobilization of NK cells, and inhibiting mononuclear cells (97, 98). Recent studies have shown that HLA-G is not limited to placental cells but is also expressed in human peripheral blood mononuclear cells (PBMCs) and plasma (99). Furthermore, in addition to its immune-inhibitory properties, HLA-G interacts with ILT2/ILT4 to suppress lymphocyte proliferation and antibody secretion by activated B cells, thereby facilitating allotransplantation by preventing immune rejection through its actions on immune cells (100).
In a recent study conducted by Li et al., the potential role of HLA-G in the pathogenesis of ITP was investigated (101). The researchers observed a significant decrease in the levels of HLA-G in the plasma of ITP patients who tested positive for antiplatelet autoantibodies compared with healthy controls. Furthermore, they observed reduced expressions of membrane-bound HLA-G and immunoglobulin-like transcripts on CD4+ and CD14+ cells in ITP patients. Moreover, the study found that HLA-G upregulated the secretion of IL-4 and IL-10 while downregulating the secretion of TNF-α, IL-12, and IL-17 by PBMCs of ITP patients. These results suggest that HLA-G may promote Th2 differentiation and suppress Th1 and Th17 immune responses (101).
8 Role of cytokines in the treatment of immune thrombocytopenia
Human IL-11 has been demonstrated to have anti-inflammatory properties by decreasing the expression of proinflammatory cytokines such as IL-1 and TNF-α. Furthermore, IL-11 reduces the expression of chemokine receptors, resulting in the inhibition of phagocytic cell migration to inflamed areas and decreased phagocytosis of immune-complexed platelets (102). In a study, Lin et al. investigated the therapeutic efficacy of recombinant human interleukin-11 (rhIL-11) in patients with ITP (103). The results of the study showed that rhIL-11 treatment led to a response rate of 67.7% in ITP patients. Patients who responded positively to treatment exhibited a significant decrease in Th1 and Tbet levels and an increase in Th2 and GATA-3 levels. Furthermore, rhIL-11 treatment led to the normalization of Th1/Th2 and Tbet/GATA-3 ratios in these patients, which were similar to those observed in healthy controls (103).
Zheng et al. utilized a mouse model of CD41-induced ITP to investigate the underlying mechanisms of IL-9 treatment (104). The researchers observed that IL-9 treatment increased the numbers of mature megakaryocytes, which are progenitor cells that differentiate into platelets, and CD41+Sca-1+ cells, which are stem cells that differentiate into various cell types, in the bone marrow of the model mice. Additionally, the researchers discovered that IL-9 treatment boosted the activation of downstream signaling molecules, such as phosphorylated signal transducer and activator of transcription 5 (STAT5) (104).
In a study conducted by Zhan et al., it was observed that the serum levels of various interleukins (IL-4, IL-5, IL-6, IL-10, IL-12p70, and IL-13), growth-related oncogene (GRO), interferon (IFN)-γ, and TNF-α were significantly lower in pre-treatment patients than in healthy controls (p < 0.05). However, in patients who achieved remission after receiving high-dose dexamethasone (HD-DXM) treatment, the levels of these cytokines were significantly higher (p < 0.05) than their pre-treatment levels. Except for TNF-α, there was no significant difference (p > 0.05) in cytokine levels between remission patients and healthy controls (105).
In a small cohort of patients with treatment-resistant ITP, IFN-α (IFN-α) has been utilized with some success by Pfueller et al. (106). These patients exhibited heightened levels of the cytokines IL-4 and IL-10, but low levels of IL-2 and IFN-γ. After IFN-α treatment, there was a notable increase in IL-2, IFN-γ, and NK cell activity, coupled with a decrease in IL-4 and IL-10. Furthermore, there was a decrease in autoantibodies targeting GPIIb/IIIa (106). An alternative treatment for chronic ITP could be the induction of immunological tolerance against the platelet-derived GPIIb/IIIa complex through oral immunization with a solubilized form of this glycoprotein receptor.
9 Conclusion
ITP is a disease characterized by immune system dysregulation, leading to the destruction of platelets and a decrease in platelet count. ITP pathogenesis is multifaceted and involves changes in T lymphocyte responses, alterations in the Th1/Th2 balance, and cytokine production. In ITP, a shift toward a Th1 cytokine profile is observed, resulting in the increased production of cytokines such as IFN-γ, IL-2, and TNF-α. This shift is associated with the activation of cytotoxic T cells and macrophages, which destroy platelets. Conversely, Th2 cytokines such as IL-4 and IL-10 are downregulated in ITP, leading to the decreased production of anti-inflammatory cytokines and a further pro-inflammatory state. The therapeutic role of cytokines in ITP is complicated since both pro- and anti-inflammatory cytokines have potential therapeutic applications. For instance, high-dose dexamethasone administration can stimulate the production of anti-inflammatory cytokines such as IL-10, promoting platelet production and reducing platelet destruction. However, further research is necessary to gain a deeper understanding of cytokine function in ITP and identify new therapeutic targets for this challenging disease.
Author contributions
Conceptualization, MA; methodology, MA; validation, MA; formal analysis, MA; resources, MA; data curation, MA; writing—original draft preparation, MA; writing—review and editing, MA; visualization, MA.
Conflict of interest
The author declares that the research was conducted in the absence of any commercial or financial relationships that could be construed as a potential conflict of interest.
Publisher’s note
All claims expressed in this article are solely those of the authors and do not necessarily represent those of their affiliated organizations, or those of the publisher, the editors and the reviewers. Any product that may be evaluated in this article, or claim that may be made by its manufacturer, is not guaranteed or endorsed by the publisher.
References
1. Zufferey A, Kapur R, Semple JW. Pathogenesis and therapeutic mechanisms in immune thrombocytopenia (ITP). J Clin Med (2017) 6:16. doi: 10.3390/jcm6020016
2. LeVine DN, Brooks MB. Immune thrombocytopenia (ITP): Pathophysiology update and diagnostic dilemmas. Veterinary Clin Pathol (2019) 48:17–28. doi: 10.1111/vcp.12774
3. Zainal A, Salama A, Alweis R. Immune thrombocytopenic purpura. J Community Hosp Internal Med Perspectives (2019) 9:59–61. doi: 10.1080/20009666.2019.1565884
4. Pietras NM, Pearson-Shaver AL. Immune thrombocytopenic purpura. In: StatPearls [Internet] Treasure Island (FL): StatPearls Publishing (2022).
5. Frederiksen H, Maegbaek ML, Nørgaard M. Twenty-year mortality of adult patients with primary immune thrombocytopenia: a Danish population-based cohort study. Br J Haematol (2014) 166:260–7. doi: 10.1111/bjh.12869
6. Perera M, Garrido T. Advances in the pathophysiology of primary immune thrombocytopenia. Hematology (2017) 22:41–53. doi: 10.1080/10245332.2016.1219497
7. Weycker D, Hanau A, Hatfield M, Wu H, Sharma A, Bensink ME, et al. Primary immune thrombocytopenia in US clinical practice: incidence and healthcare burden in first 12 months following diagnosis. J Med Economics (2020) 23:184–92. doi: 10.1080/13696998.2019.1669329
8. Kewan T, Gunaratne TN, Mushtaq K, Alayan D, Daw H, Haddad A. Outcomes and management of immune thrombocytopenia secondary to COVID-19: Cleveland clinic experience. Transfusion (2021) 61:2014–8. doi: 10.1111/trf.16368
9. Stasi R. Pathophysiology and therapeutic options in primary immune thrombocytopenia. Blood Transfusion (2011) 9:262. doi: 10.2450/2010.0080-10
10. Shulman NR, Weinrach RS, Libre EP, Andrews HL. The role of the reticuloendothelial system in the pathogenesis of idiopathic thrombocytopenic purpura. Trans Assoc Am Physicians (1965) 78:374–90.
11. Zheng SS, Ahmadi Z, Leung HHL, Wong R, Yan F, Perdomo JS, et al. Antiplatelet antibody predicts platelet desialylation and apoptosis in immune thrombocytopenia. Haematologica (2022) 107:2195–205. doi: 10.3324/haematol.2021.279751
12. Iraqi M, Perdomo J, Yan F, Choi PY, Chong BH. Immune thrombocytopenia: antiplatelet autoantibodies inhibit proplatelet formation by megakaryocytes and impair platelet production in vitro. Haematologica (2015) 100:623–32. doi: 10.3324/haematol.2014.115634
13. Bartley TD, Bogenberger J, Hunt P, Li YS, Lu HS, Martin F, et al. Identification and cloning of a megakaryocyte growth and development factor that is a ligand for the cytokine receptor Mpl. Cell (1994) 77:1117–24. doi: 10.1016/0092-8674(94)90450-214
14. Gilbert MM, Grimes AB, Kim TO, Despotovic JM. Romiplostim for the treatment of immune thrombocytopenia: spotlight on patient acceptability and ease of use. Patient Preference Adherence (2020), 1237–50. doi: 10.2147/PPA.S192481
15. Wei Y, Hou M. T cells in the pathogenesis of immune thrombocytopenia. In: Seminars in Hematology, vol. 53. Elsevier (2016). p. S13–5. doi: 10.1053/j.seminhematol.2016.04.005
16. Ware RE, Howard TA. Elevated numbers of gamma-delta (γδ+) T lymphocytes in children with immune thrombocytopenic purpura. J Clin Immunol (1994) 14:237–47. doi: 10.1007/BF01552310
17. Yoshimura C, Nomura S, Nagahama M, Ozaki Y, Kagawa H, Fukuhara S. Plasma-soluble Fas (APO-1, CD95) and soluble Fas ligand in immune thrombocytopenic purpura. Eur J Haematol (2000) 64:219–24. doi: 10.1034/j.1600-0609.2000.9o096.x
18. Talaat R, Elmaghraby A, Barakat S, El-Shahat M. Alterations in immune cell subsets and their cytokine secretion profile in childhood idiopathic thrombocytopenic purpura (ITP). Clin Exp Immunol (2014) 176:291–300. doi: 10.1111/cei.12279
19. Guo N-H, Fu X, Zi F-M, Song Y, Wang S, Cheng J. The potential therapeutic benefit of resveratrol on Th17/Treg imbalance in immune thrombocytopenic purpura. Int Immunopharmacol (2019) 73:181–92. doi: 10.1016/j.intimp.2019.04.061
20. Kostic M, Zivkovic N, Cvetanovic A, Marjanović G. CD4+ T cell phenotypes in the pathogenesis of immune thrombocytopenia. Cell Immunol (2020) 351:104096. doi: 10.1016/j.cellimm.2020.104096
21. Cancro MP, Tomayko MM. Memory B cells and plasma cells: The differentiative continuum of humoral immunity. Immunol Rev (2021) 303:72–82. doi: 10.1111/imr.13016
22. Abebe EC, Dejenie TA, Ayele TM, Baye ND, Teshome AA, Muche ZT. The role of regulatory B cells in health and diseases: a systemic review. J Inflammation Res (2021) 14:75. doi: 10.2147/JIR.S286426
23. Oh DY, Fong L. Cytotoxic CD4+ T cells in cancer: Expanding the immune effector toolbox. Immunity (2021) 54:2701–11. doi: 10.1016/j.immuni.2021.11.015
27. Theofilopoulos AN, Kono DH, Baccala R. The multiple pathways to autoimmunity. Nat Immunol (2017) 18:716–24. doi: 10.1038/ni.3731
28. Salinas GF, Braza F, Brouard S, Tak P-P, Baeten D. The role of B lymphocytes in the progression from autoimmunity to autoimmune disease. Clin Immunol (2013) 146:34–45. doi: 10.1016/j.clim.2012.10.005
29. Zhang HY, Hou M, Zhang XH, Guan XH, Sun GZ. The diagnostic value of platelet glycoprotein-specific autoantibody detection in idiopathic thrombocytopenic purpura. Zhongguo Shi Yan Xue Ye Xue Za Zhi (2004) 12:204–6.
30. Marini I, Bakchoul T. Pathophysiology of autoimmune thrombocytopenia: current insight with a focus on thrombopoiesis. Hämostaseologie (2019) 39:227–37. doi: 10.1055/s-0039-1678732
31. Wienzek-Lischka S, König IR, Papenkort EM, Hackstein H, Santoso S, Sachs UJ, et al. HLA-DRB3* 01: 01 is a predictor of immunization against human platelet antigen-1a but not of the severity of fetal and neonatal alloimmune thrombocytopenia. Transfusion (2017) 57:533–40. doi: 10.1111/trf.13950
32. Pesmatzoglou M, Lourou M, Goulielmos GN, Stiakaki E. DNA methyltransferase 3B gene promoter and interleukin-1 receptor antagonist polymorphisms in childhood immune thrombocytopenia. Clin Dev Immunol (2012) 2012:352059. doi: 10.1155/2012/352059
33. Maouia A, Rebetz J, Kapur R, Semple JW. The immune nature of platelets revisited. Transfusion Med Rev (2020) 34:209–20. doi: 10.1016/j.tmrv.2020.09.005
34. Norris PA, Segel GB, Burack WR, Sachs UJ, Lissenberg-Thunnissen SN, Vidarsson G, et al. FcγRI and FcγRIII on splenic macrophages mediate phagocytosis of anti-glycoprotein IIb/IIIa autoantibody-opsonized platelets in immune thrombocytopenia. Haematologica (2021) 106:250. doi: 10.3324/haematol.2020.248385
35. Li X, Zhong H, Bao W, Boulad N, Evangelista J, Haider MA, et al. Defective regulatory B-cell compartment in patients with immune thrombocytopenia. Blood J Am Soc Hematol (2012) 120:3318–25. doi: 10.1182/blood-2012-05-432575
36. Wang Q, Li J, Yu TS, Liu Y, Li K, Liu S, et al. Disrupted balance of CD4+ T-cell subsets in bone marrow of patients with primary immune thrombocytopenia. Int J Biol Sci (2019) 15:2798. doi: 10.7150/ijbs.33779
37. Takahashi N, Saitoh T, Gotoh N, Nitta Y, Alkebsi L, Kasamatsu T, et al. The cytokine polymorphisms affecting Th1/Th2 increase the susceptibility to, and severity of, chronic ITP. BMC Immunol (2017) 18:1–11. doi: 10.1186/s12865-017-0210-3
38. Ni X, Zhao Y, Yu T, Wang H, Wang L, Xu P, et al. Rapamycin inhibits cytotoxic T lymphocytes-mediated platelet destruction in immune thrombocytopenia. Blood (2022) 140:8404–5. doi: 10.1182/blood-2022-166345
39. Wonderlich J, Shearer G, Livingstone A, Brooks A, Soloski MJ, Presby MM. Induction and measurement of cytotoxic T lymphocyte activity. Curr Protoc Immunol (2018) 120:3.11. 11–13.11. 29. doi: 10.1002/cpim.38
40. Wen R, Wang Y, Hong Y, Yang Z. Cellular immune dysregulation in the pathogenesis of immune thrombocytopenia. Blood Coagulation Fibrinolysis (2020) 31:113–20. doi: 10.1097/MBC.0000000000000891
41. Dominguez-Villar M, Hafler DA. Regulatory T cells in autoimmune disease. Nat Immunol (2018) 19:665–73. doi: 10.1038/s41590-018-0120-4
42. Wang SC, Yang KD, Lin CY, Huang AY, Hsiao CC, Lin MT, et al. Intravenous immunoglobulin therapy enhances suppressive regulatory T cells and decreases innate lymphoid cells in children with immune thrombocytopenia. Pediatr Blood Cancer (2020) 67:e28075. doi: 10.1002/pbc.28075
43. Aslam R, Hu Y, Gebremeskel S, Segel GB, Speck ER, Guo L, et al. Thymic retention of CD4+CD25+FoxP3+ T regulatory cells is associated with their peripheral deficiency and thrombocytopenia in a murine model of immune thrombocytopenia. Blood (2012) 120:2127–32. doi: 10.1182/blood-2012-02-413526
44. Ruterbusch M, Pruner KB, Shehata L, Pepper M. In vivo CD4+ T cell differentiation and function: revisiting the Th1/Th2 paradigm. Annu Rev Immunol (2020) 38:705–25. doi: 10.1146/annurev-immunol-103019-085803
45. Ji X, Zhang L, Peng J, Hou M. T cell immune abnorMalities in immune thrombocytopenia. J Hematol Oncol (2014) 7:1–6. doi: 10.1186/s13045-014-0072-6
46. Lin X, Xu A, Zhou L, et al. Imbalance of T lymphocyte subsets in adult immune thrombocytopenia. Int J Gen Med (2021), 937–47. doi: 10.2147/IJGM.S298888
47. Li Q, Liu Y, Wang X, Sun M, Wang L, Wang X, et al. Regulation of Th1/Th2 and Th17/Treg by pDC/mDC imbalance in primary immune thrombocytopenia. Exp Biol Med (2021) 246:1688–97. doi: 10.1177/15353702211009787
48. Guo C, Chu X, Shi Y, He W, Li L, Wang L, et al. Correction of Th1-dominant cytokine profiles by high-dose dexamethasone in patients with chronic idiopathic thrombocytopenic purpura. J Clin Immunol (2007) 27:557–62. doi: 10.1007/s10875-007-9111-1
49. Shan NN, Zhu XJ, Peng J, Qin P, Zhuang XW, Wang HC, et al. Interleukin 18 and interleukin 18 binding protein in patients with idiopathic thrombocytopenic purpura. Br J Haematol (2009) 144:755–61. doi: 10.1111/j.1365-2141.2008.07520.x
50. Schifferli A, Cavalli F, Godeau B, Liebman HA, Recher M, Imbach P, et al. Understanding immune thrombocytopenia: looking out of the box. Front Med (2021) 8:613192. doi: 10.3389/fmed.2021.613192
51. Zhan Y, Cheng L, Wu B, Ji L, Chen P, Li F, et al. Interleukin (IL)-1 family cytokines could differentiate primary immune thrombocytopenia from systemic lupus erythematosus-associated thrombocytopenia. Ann Transl. Med (2021) 9:222. doi: 10.21037/atm-20-4729
52. Li P-P, Zhang X-M, Yuan D, Liu X, Li Y, Shan N-N. Decreased expression of IL-33 in immune thrombocytopenia. Int Immunopharmacol (2015) 28:420–4. doi: 10.1016/j.intimp.2015.06.035
53. Goelz N, Bosch AMS, Rand ML, Eekels JJM, Franzoso FD, Schmugge M. Increased levels of IL-10 and IL-1Ra counterbalance the proinflammatory cytokine pattern in acute pediatric immune thrombocytopenia. Cytokine (2020) 130:155078. doi: 10.1016/j.cyto.2020.155078
54. Ma L, Liang Y, Fang M, Guan Y, Si Y, Jiang F, et al. The cytokines (IFN-γ, IL-2, IL-4, IL-10, IL-17) and Treg cytokine (TGF-β1) levels in adults with immune thrombocytopenia. Die Pharmazie-An Int J Pharm. Sci (2014) 69:694–7.
55. Zhang G, Zhang P, Liu H, Liu X, Xie S, Wang X, et al. Assessment of Th17/Treg cells and Th cytokines in an improved immune thrombocytopenia mouse model. Hematology (2017) 22:493–500. doi: 10.1080/10245332.2017.1301040
56. Fatma E, Ahmed K, Nihal E-K, Salwa H. Cytokines and immunoglobulin derangement in Egyptian children with primary immune thrombocytopenic purpura. Egyptian J Haematol (2018) 43:1–4. doi: 10.4103/ejh.ejh_37_17
57. Li H-Y, Zhang D-L, Zhang X, Liu X-F, Xue F, Yang R-C. Interleukin-7 is decreased and maybe plays a pro-inflammatory function in primary immune thrombocytopenia. Platelets (2015) 26:243–9. doi: 10.3109/09537104.2014.903392
58. Qiao J, Li X, Wu Y, Wu X, Zhu F, Liu N, et al. An increased expression profile of Th9/IL-9 correlated with Th17/IL-17 in patients with immune thrombocytopenia. Platelets (2017) 28:287–94. doi: 10.1080/09537104.2016.1218454
59. Li W, Wang X, Li J, Liu M, Feng J. A study of immunocyte subsets and serum cytokine profiles before and after immunal suppression treatment in patients with immune thrombocytopenia. Zhonghua Nei Ke Za Zhi (2016) 55:111–5. doi: 10.3760/cma.j.issn.0578-1426.2016.02.009
60. Li Q, Zhang L, Xia R, Zeng Q, Wang Y, Xia L, et al. Plasma Levels of Interleukin 12 Family Cytokines and Their Relevant Cytokines in Adult Patients with Chronic Immune Thrombocytopenia before and after High-Dose Dexamethasome Treatment. Med Principles Practice (2015) 24:458–64. doi: 10.1159/000433472
61. Li Q, Yang M, Xia R, Xia L, Zhang L. Elevated expression of IL-12 and IL-23 in patients with primary immune thrombocytopenia. Platelets (2015) 26:453–8. doi: 10.3109/09537104.2014.934217
62. Ye X, Zhang L, Wang H, Chen Y, Zhang W, Zhu R, et al. The role of IL-23/Th17 pathway in patients with primary immune thrombocytopenia. PloS One (2015) 10:e0117704. doi: 10.1371/journal.pone.0117704
63. Goelz N, Winkler J, Eekels JJM, Rand ML, Speer O, Schmugge M. Cytokine and chemokine levels correlate with apoptosis markers in platelets of pediatric patients with immune thrombocytopenia. Blood (2016) 128:1371. doi: 10.1182/blood.V128.22.1371.1371
64. Stimpson ML, Lait PJP, Schewitz-Bowers LP, Williams EL, Thirlwall KF, Lee RWJ, et al. IL-10 and IL-17 expression by CD4+ T cells is altered in corticosteroid refractory immune thrombocytopenia (ITP). J Thromb Haemostasis (2020) 18:2712–20. doi: 10.1111/jth.14970
65. Zhang Q, Bai H, Wang M-L, Ma H, Zhang XL, Wang CB, et al. Effects of the interleukin-21 expression in patients with immune thrombocytopenia and its regulation by high-dose dexamethasone. Zhongguo Shi Yan Xue Ye Xue Za Zhi (2015) 23:465–70. doi: 10.7534/j.issn.1009-2137.2015.02.033
66. Liu Q, Liu Y. Role of IL-10 and IL-22 cytokines in patients with primary immune thrombocytopenia and their clinical significance. J Clin Lab Analysis (2022) 36:e24573. doi: 10.1002/jcla.24573
67. Hassan T, Abdel Rahman D, Raafat N, Fathy M, Shehab M, Hosny A, et al. Contribution of interleukin 27 serum level to pathogenesis and prognosis in children with immune thrombocytopenia. Med (Baltimore) (2022) 101:e29504. doi: 10.1097/md.0000000000029504
68. Sun T, Zhang D, Yang Y, Zhang X, Lv C, Fu R, et al. Interleukin 35 may contribute to the loss of immunological self-tolerance in patients with primary immune thrombocytopenia. Br J Haematol (2015) 169:278–85. doi: 10.1111/bjh.13292
69. Butcher MJ, Zhu J. Recent advances in understanding the Th1/Th2 effector choice. Faculty Rev (2021) 10. doi: 10.12703/r/10-30
70. Yasuda K, Takeuchi Y, Hirota K. The pathogenicity of Th17 cells in autoimmune diseases. In: Seminars in Immunopathology, vol. 41. Springer (2019). p. 283–97.
72. Okamoto N, Homma M, Kawaguchi Y, Kabasawa N, Uto Y, Hattori N, et al. Increased expression of interleukin-17 is associated with macrophages in chronic immune thrombocytopenia. Int J Clin Exp Pathol (2018) 11:2419–29.
73. Zhou L, Xu F, Chang C, Tao Y, Song L, Li X. Interleukin-17-producing CD4+ T lymphocytes are increased in patients with primary immune thrombocytopenia. Blood Coagulation Fibrinolysis (2016) 27:301–7. doi: 10.1097/MBC.0000000000000423
74. Haznedaroglu IC, Büyükaşik Y, Koşar A, Ozcebe OI, Kirazli S, Dündar S. Selectins and IL-6 during the clinical course of idiopathic thrombocytopenic purpura. Acta Haematol (1999) 101:16–20. doi: 10.1159/000040915
75. Nguyen V, Mendelsohn A, Larrick JW. Interleukin-7 and immunosenescence. J Immunol Res (2017) 2017. doi: 10.1155/2017/4807853
76. Winer H, Rodrigues GO, Hixon JA, Aiello FB, Hsu TC, Wachter BT, et al. IL-7: comprehensive review. Cytokine (2022) 160:156049. doi: 10.1016/j.cyto.2022.156049
77. Deng Y, Wang Z, Chang C, Lu L, Lau CS, Lu Q. Th9 cells and IL-9 in autoimmune disorders: Pathogenesis and therapeutic potentials. Hum Immunol (2017) 78:120–8. doi: 10.1016/j.humimm.2016.12.010
78. Saraiva M, Vieira P, O’garra A. Biology and therapeutic potential of interleukin-10. J Exp Med (2019) 217:e20190418. doi: 10.1084/jem.20190418
79. Neumann C, Scheffold A, Rutz S. Functions and regulation of T cell-derived interleukin-10. In: Seminars in Immunology, vol. 44. Elsevier (2019). p. 101344.
80. Toker H, Gorgun EP, Korkmaz EM, Yüce HB, Poyraz O. The effects of IL-10 gene polymorphism on serum, and gingival crevicular fluid levels of IL-6 and IL-10 in chronic periodontitis. J Appl Oral Sci (2018) 26. doi: 10.1590/1678-7757-2017-0232
81. Johnsen J. Pathogenesis in immune thrombocytopenia: new insights. In: Hematology 2010, the American Society of Hematology Education Program Book, vol. 2012. (2012). p. 306–12.
82. Li F, Ji L, Wang W, Hua F, Zhan Y, Zou S, et al. Insufficient secretion of IL-10 by Tregs compromised its control on over-activated CD4+ T effector cells in newly diagnosed adult immune thrombocytopenia patients. Immunol Res (2015) 61:269–80. doi: 10.1007/s12026-014-8620-2
83. Soliman MA, Helwa MA, Fath-Allah SK, El-Hawy MA, Badr HS, Barseem NF. IL-10 polymorphisms and T-cell subsets could affect the clinical presentation and outcome of childhood immune thrombocytopenia in Egyptian population. APMIS (2018) 126:380–8. doi: 10.1111/apm.12823
84. Azizi G, Yazdani R, Hamid KM, Razavi A, Mirshafiey A. IL-22 Produced by T helper Cell 22 as a New player in the Pathogenesis of Immune Thrombocytopenia. Endocr Metab Immune Disord Drug Targets (2015) 15:242–50. doi: 10.2174/1871530315666150331154733
85. Leonard WJ, Wan CK. IL-21 signaling in immunity. F1000Res (2016) 5. doi: 10.12688/f1000research.7634.1
86. Han L, Chen Z, Yu K, Yan J, Li T, Ba X, et al. Interleukin 27 signaling in rheumatoid arthritis patients: good or evil? Front Immunol (2022) 12:5534. doi: 10.3389/fimmu.2021.787252
87. Ali YB, El-Akhras BA, El-Shazly R, Bassyouni IH. Genetic polymorphisms of IL-27 and risk of systemic lupus erythematosus disease in the Egyptian population. Clin Rheumatol (2021) 40:4899–907. doi: 10.1007/s10067-021-05858-6
88. Abdel-Fattah NR. Interleukin 27 serum level and its prognostic significance in children with immune thrombocytopenia. Zagazig Univ. Med J (2020) 26:132–9. doi: 10.21608/ZUMJ.2019.11145.1156
89. Gad Allah H, Moussa M, El-Ghammaz A, Ali B. Role of interleukin-27 in immune thrombocytopenic purpura and its impact on disease response. Egyptian J Haematol (2016) 41:116–20. doi: 10.4103/1110-1067.196177
90. Chan BCL, Lam CWK, Tam LS, Wong CK. IL33: roles in allergic inflammation and therapeutic perspectives. Front Immunol (2019) 10:364. doi: 10.3389/fimmu.2019.00364
91. Ye C, Yano H, Workman CJ, Vignali DAA. Interleukin-35: structure, function and its impact on immune-related diseases. J Interferon Cytokine Res (2021) 41:391–406. doi: 10.1089/jir.2021.0147
92. Zhao Z, Ren J, Dai C, Kannapell CC, Wang H, Gaskin F, et al. Nature of T cell epitopes in lupus antigens and HLA-DR determines autoantibody initiation and diversification. Ann Rheumatic Dis (2019) 78:380–90. doi: 10.1136/annrheumdis-2018-214125
93. Li CW, Osman R, Menconi F, Concepcion ES, Tomer Y. Flexible peptide recognition by HLA-DR triggers specific autoimmune T-cell responses in autoimmune thyroiditis and diabetes. J Autoimmun (2017) 76:1–9. doi: 10.1016/j.jaut.2016.09.007
94. Wang J, Jelcic I, Mühlenbruch L, Haunerdinger V, Toussaint NC, Zhao Y, et al. HLA-DR15 molecules jointly shape an autoreactive T cell repertoire in multiple sclerosis. Cell (2020) 183:1264–1281.e1220. doi: 10.1016/j.cell.2020.09.054
95. Audia S, Mahévas M, Samson M, Godeau B, Bonnotte B. Pathogenesis of immune thrombocytopenia. Autoimmun Rev (2017) 16:620–32. doi: 10.1016/j.autrev.2017.04.012
96. Semple J, Milev Y, Cosgrave D, Mody M, Hornstein A, Blanchette V, et al. Differences in serum cytokine levels in acute and chronic autoimmune thrombocytopenic purpura: relationship to platelet phenotype and antiplatelet T-cell reactivity. Blood (1996) 87:4245–54. doi: 10.1182/blood.V87.10.4245.bloodjournal87104245
97. Zhuang B, Shang J, Yao Y. HLA-G: An important mediator of maternal-fetal immune-tolerance. Front Immunol (2021) 12:744324. doi: 10.3389/fimmu.2021.744324
98. Ferreira LM, Meissner TB, Tilburgs T, Strominger JL. HLA-G: at the interface of maternal–fetal tolerance. Trends Immunol (2017) 38:272–86. doi: 10.1016/j.it.2017.01.009
99. Contini P, Murdaca G, Puppo F, Negrini S. HLA-G expressing immune cells in immune mediated diseases. Front Immunol (2020) 11:1613. doi: 10.3389/fimmu.2020.01613
100. Naji A, Menier C, Morandi F, Agaugu� S, Maki G, Ferretti E, et al. Binding of HLA-G to ITIM-bearing Ig-like transcript 2 receptor suppresses B cell responses. J Immunol (2014) 192:1536–46. doi: 10.4049/jImmunol1300438
101. Li X, Sheng Z, Sun Y, Wang Y, Xu M, Zhang Z, et al. Human leukocyte antigen-G upregulates immunoglobulin-like transcripts and corrects dysfunction of immune cells in immune thrombocytopenia. Haematologica (2021) 106:770. doi: 10.3324/haematol.2018.204040
102. Andersson J. Cytokines in idiopathic thrombocytopenic purpura (ITP). Acta Paediatrica (1998) 87:61–4. doi: 10.1111/j.1651-2227.1998.tb01237.x
103. Lin Y, Zhou X, Guo W, Li Q, Pan X, Bao Y, et al. RhIL-11 treatment norMalized Th1/Th2 and T-bet/GATA-3 imbalance in in human immune thrombocytopenic purpura (ITP). Int Immunopharmacol (2016) 38:40–4. doi: 10.1016/j.intimp.2016.05.002
104. Zheng Y, He Y, Xiao M, Zhang W, Xia W, Hu H, et al. Interleukin 9 prevents immune thrombocytopenia in mice via JAK/STAT5 signaling. Exp Cell Res (2020) 388:111801. doi: 10.1016/j.yexcr.2019.111801
105. Zhan Y, Zou S, Hua F, Li F, Ji L, Wang W, et al. High-dose dexamethasone modulates serum cytokine profile in patients with primary immune thrombocytopenia. Immunol Lett (2014) 160:33–8. doi: 10.1016/j.imlet.2014.03.002
106. Pfueller SL, Weber S, Lüscher EF. Studies of the mechanism of the human platelet release reaction induced by immunologic stimuli. III. Relationship between the binding of soluble IgG aggregates to the Fc receptor and cell response in the presence and absence of plasma. J Immunol (1977) 118:514–24. doi: 10.1172/JCI109559
Keywords: idiopathic, platelets, interleukin, autoantibodies, lymphocytes
Citation: Andreescu M (2023) The link between immune thrombocytopenia and the cytokine profile: a bridge to new therapeutical targets. Front. Hematol. 2:1191178. doi: 10.3389/frhem.2023.1191178
Received: 21 March 2023; Accepted: 25 July 2023;
Published: 10 August 2023.
Edited by:
Suparno Chakrabarti, Narayana Health, IndiaReviewed by:
Dipendra Kumar Mitra, All India Institute of Medical Sciences, IndiaPankaj Malhotra, Post Graduate Institute of Medical Education and Research (PGIMER), India
Copyright © 2023 Andreescu. This is an open-access article distributed under the terms of the Creative Commons Attribution License (CC BY). The use, distribution or reproduction in other forums is permitted, provided the original author(s) and the copyright owner(s) are credited and that the original publication in this journal is cited, in accordance with accepted academic practice. No use, distribution or reproduction is permitted which does not comply with these terms.
*Correspondence: Mihaela Andreescu, dGV2ZXRtaWhhZWxhQGdtYWlsLmNvbQ==