- 1Pediatric Hematopoietic Stem Cell Transplantation and Immunodeficiency, Department for Children and Adolescents, Copenhagen University Hospital Rigshospitalet, Copenhagen, Denmark
- 2Department of Clinical Immunology, The Tissue Typing Laboratory, Copenhagen University Hospital Rigshospitalet, Copenhagen, Denmark
- 3University of Helsinki and Division of Pediatric Hematology-Oncology and Stem Cell Transplantation at Hospital for Children and Adolescents, University of Helsinki, Helsinki, Finland
- 4Department of Pediatric Hematology and Oncology, Oslo University Hospital, Oslo, Norway
- 5Department of Pediatric Oncology, Sahlgrenska University Hospital, Gothenburg University, Gothenburg, Sweden
- 6Division of Pediatric Oncology and Hematology, Skåne University Hospital Lund, Lund, Sweden
- 7Section of Biostatistics, Department of Public Health, University of Copenhagen, Copenhagen, Denmark
Analysis of chimerism in blood post‐HCT using STR‐PCR is routinely applied in parallel with quantification of MRD to predict relapse of leukemia. Real time quantitative PCR (RQ-PCR) chimerism is 10‐ to 100‐fold more sensitive, but clinical studies in children are sparse. In a prospective multicenter study, we analyzed increasing mixed chimerism (IMC) in blood samples following transplantation for leukemia in 64 children. IMC was defined as a minimum increase of either 0.1% or 0.05% recipient DNA between two samples or a ≥10-fold increase. Samples closer than 30 days to diagnosis of relapse were omitted. The risk of relapse was higher in children with IMC of both 0.1% and 0.05% compared to children without IMC (27.8 (95% CI 4.4-175.8; P<.001), and 18.4 (95% CI 2.8-120.5; P=0.002), respectively). From the date of IMC, the 3-year CI of relapse or MRD-positivity was 26.7% (CI 9.4-47.0) and 18.5% (6.4-35.3) for IMC ≥ 0.1% (n=27) and ≥ 0.05% (n= 40), respectively. In the subset of children without an IMC ≥ 0.1% or ≥ 0.05%, CI of relapse or molecular relapse were 16.7% (5.0 -34.1) and 10.8% (3.4 -23.3), respectively. In all cases with a relapse undetectable by IMC, MRD remained undetectable prior to relapse and standard chimerism negative. In a landmark analysis, neither an IMC ≥ 0.1% nor ≥ 0.05% prior to 90 days post‐HCT was significantly associated with an increased relapse incidence. These results indicate that the serial monitoring of RQ‐PCR chimerism in peripheral blood post-HCT may be a valuable supplement to the minimal residual disease analysis for an early detection of relapse in acute childhood leukemia.
1 Introduction
Allogeneic hematopoietic stem cell transplantation (HCT) represents a curative option for high-risk childhood leukemia, but relapse remains the major cause of treatment failure (1). The outcome following post-HCT relapse is dismal (2–6). The potential for an early intervention with fast tapering of immunosuppressive treatment, administration of donor lymphocyte infusions (DLI) or targeted therapy with i.e. leukemia specific antibodies (for example blinatumomab), anti-CD19 chimeric antigen receptor (CAR) T-cell therapy or tyrosine kinase inhibitors (TKI) has increased the focus on post-HCT monitoring for impending relapse (7–9).
Screening for relapse post-HCT is currently based on detection of minimal residual disease (MRD) or recipient DNA (chimerism). MRD analyses by flow cytometry or quantitative PCR reach sensitivity levels of 10-4 – 10-5 for more than 90% of all children with acute lymphoblastic leukemia (ALL) (10, 11). In acute myeloid leukemia (AML), MRD is most frequently monitored by flow cytometry with one to two logs lower sensitivities than in ALL (12, 13). More sensitive PCR-based analysis can be used in only 50% of children with AML, and with the risk of loss of markers or persistence of fusion transcripts, the interpretation is complicated (14–16). Furthermore, in general, MRD is in most cases analyzed on bone marrow (BM) samples, which limits the frequency of measurements in children.
In the allogeneic HCT setting, chimerism refers to the presence of hematopoietic cells of donor origin. In malignant disease, the goal is to achieve complete donor chimerism (CDC). PCR based detection of short tandem repeats (STR-PCR) has been the gold standard in the previous decades, with a sensitivity of 1-5% recipient cells (17, 18). Chimerism analysis is simple and applicable regardless of the pre-HCT diagnosis. Increasing or reappearing mixed chimerism (MC) in peripheral blood (PB) by STR-PCR has consistently been associated with an increased incidence of relapse (19–23). A major limitation is the low sensitivity, as reappearance of 1-5% recipient DNA in PB is often past the window of an impending relapse (23). New methods based on real-time quantitative PCR (RQ-PCR) techniques have been developed over the past decades, using amplification of highly polymorphic targets such as single-nucleotide polymorphisms (SNP) or insertion-deletions (In/del) and, more recently, the application of digital PCR and Next Generation Sequencing (NGS) platforms (24–28). Clinical studies have shown that analysis of chimerism by RQ-PCR is a more precise and thus early predictor of relapse compared to standard chimerism analysis (29–38). Studies in adults have reported earlier detection of relapse, and better overall and event free survival if immunomodulation is based on results of chimerism analysis by RQ-PCR (31, 39–41). Currently, published evidence suggests a probable association between RQ-PCR chimerism analysis in PB and risk of relapse related to the dynamics of chimerism, with less evidence towards the importance of achieving or not achieving CDC (30, 35, 42). However, there are only few clinical studies in children and neither scientific nor clinical consensus on the interpretation of results have yet been established. In a previous retrospective study of children with leukemia from our group, we found that IMC ≥ 0.1% and ≥ 0.05% in PB analyzed by high-sensitivity RQ-PCR chimerism was associated with a significantly increased risk of relapse detectable with a median of 208 days prior to relapse. A major limitation to this study was its retrospective design and lack of a pre-defined sample protocol.
In this prospective multicenter study of Nordic children transplanted for leukemia we report results of a high-sensitivity RQ-PCR chimerism analysis in successive samples of PB following a pre-defined sample protocol with frequent samples and compare results to with the MRD and STR-PCR chimerism analyses.
2 Materials and methods
2.1 Patients and samples
This prospective multicenter study was performed in children consecutively transplanted for leukemia between December 2014 and March 2018 at the Department for Children and Adolescents, Rigshospitalet, Copenhagen, Denmark; the Division of Pediatric Hematology-Oncology and Stem Cell Transplantation at Hospital for Children and Adolescents, University of Helsinki, Finland; the Department of Pediatric Hematology and Oncology, Oslo University Hospital, Oslo, Norway; the Department of Pediatric Oncology, Sahlgrenska University Hospital, Gothenburg, Sweden, and the Division of Pediatric Oncology and Hematology, Scania University Hospital Lund, Sweden.
Inclusion criteria were age below 16 years at diagnosis and below 18 years at transplantation for leukemia and for Sweden, Norway and Finland a signed informed consent from a parent/legal guardian.
The PB samples were collected in sodium citrate tubes at study enrolment pre-HCT and bi-weekly from 30 days until 6 months post-HCT and monthly thereafter until relapse, transplant related death or end of follow-up at 18 months post-HCT. Samples were sent by courier to the Department of Clinical Immunology, Copenhagen University Hospital, Rigshospitalet, for RQ-PCR chimerism analysis in batch at end of follow-up. Results were blinded for treating clinicians.
The data collected from each transplant center included data from the initial diagnosis and treatment stratification, HCT procedure, clinical outcome, and results from locally performed STR-PCR chimerism and MRD analyses.
The study was performed in accordance with the Declaration of Helsinki and approved by the Danish Data Protection Agency (ID 30-1319), the Ethical Committees of the Capital Region of Denmark (H-3-2014-FSP21), the Regional Ethical Committee of South East Norway (2015/2421/REK sør-øst), the Central Ethical Review Board of Gothenburg, Sweden (618–15), and the regional ethical committee of Helsinki and Uusimaa Hospital District, Finland (II/15).
2.2 Analysis of STR-PCR chimerism
Analysis of STR-PCR chimerism is recommended by the Eurochimerism consortium as a standardized diagnostic method for detection and monitoring of chimerism after HCT and is performed locally following established guidelines (43, 44). The STR-PCR chimerism analysis was performed in accredited laboratories at all centers. The sample frequency for STR-PCR chimerism analysis was locally defined, and could thus vary between centers.
2.3 Analysis of RQ-PCR chimerism
DNA was extracted from unseparated peripheral blood using a semi-automated platform (Maxwell, Promega, Madison, WI, USA), following manufacturer’s instructions. DNA was frozen and stored at -20°C and samples analyzed in batch at end of follow-up. The analysis of RQ-PCR chimerism was performed using a commercially available kit (KMRtype and KMRtrack, GenDx, Utrecht, Netherlands). This technique is based on allele-specific RQ-PCR of 34 specific insertions/deletions using TaqMan technology. We previously described our initial confirmation of a quantitative sensitivity of 0.1-0.01% in our laboratory and acceptable background at 250 ng input DNA (45).
A screening of 34 polymorphic systems was performed on pre-HCT samples from patient and donor, to obtain a minimum of two patient-specific markers per pair. Subsequently, the post-HCT DNA samples were screened in duplicates for patient DNA using manufacturer’s instructions. DNA negative for the selected markers was used as negative control. Results were calculated using the ΔΔCT method, quantifying DNA relative to a positive control and a reference gene (RNaseP) (44).
2.4 Classification of chimerism results
The detection limit of RQ-PCR chimerism was defined as 0.01% recipient DNA, and values below this level were considered to be complete donor chimerism (CDC).
As previously described (45), RQ-PCR chimerism results were classified according to an increase of recipient DNA from one sample to the next (increasing mixed chimerism (IMC)). Thus, a child with detectable but stable levels of recipient DNA was not categorized as having IMC. We analyzed two different classifications of IMC: a) ≥ 0.1% increase in recipient DNA between two consecutive samples and b) ≥ 0.05% increase in recipient DNA between two consecutive samples (current value ÷ previous value).
As previously described (45), and based on results from a dilution series performed in our laboratory, an increase of 0.05% recipient DNA was considered close to the accuracy of the RQ-PCR chimerism method. Thus, an IMC of 0.05% was only considered significant if the recipient DNA also increased by a minimum of 100% compared to the previous sample (corresponding to 1 CT). Further, an increase by a minimum of 100% compared to the previous sample (corresponding to 1 CT) was also required if the IMC occurred at an absolute level of 5% recipient DNA or above, as at this level the accuracy of the RQ-PCR chimerism is reduced (46). Regardless of the classification, a relative increase ((current value ÷ previous value)/previous value) 10 times (one log, corresponding to 3.3 CT-values) occurring from a level above 0.01% recipient DNA was always considered an IMC. We further classified children as having one or two episodes of IMC.
2.5 Minimal residual disease
MRD was analyzed by RQ-PCR according to the EuroMRD guidelines (47) or by multicolor flow cytometry as described in the NOPHO ALL-2008/iBFM-FORUM and the NOPHO AML 2012 guidelines, in all participating centers. The MRD detection method varied between disease groups, thus for further analysis MRD was categorized as undetected, detectable but unquantifiable, detectable below 10-3, or detectable ≥10-3.
2.6 Statistics
Quantitative variables are described using median (range) unless otherwise stated.
Event free (EFS) and overall survivals (OS) were calculated using the Kaplan–Meier method and comparisons performed with the log-rank test. The cumulative incidence of relapse (CIR) was estimated using the Aalen-Johansen estimator (48) and compared between subgroups by Gray’s test (49). Non-relapse mortality (NRM) was considered a competing event.
Primary clinical endpoint was relapse, defined as ≥ 5% leukemic cells in the BM or extramedullary relapse. A secondary clinical endpoint was a composite endpoint of hematological relapse and detection of MRD above ≥10-3 and less than 5% in AML, ALL and CML if this was interpreted as a molecular relapse by the treating clinician.
In case of hematological relapse, samples taken within 30 days of relapse were not included in the analysis, as these were considered coinciding with relapse. In the analysis of the composite endpoint, samples taken within 30 days of hematological relapse or on the day of MRD-positivity were not included in the analysis, as samples beyond this point could be influenced by clinical intervention. In children with both a hematological and molecular relapse, only the first event was considered for analysis of the composite endpoints.
To evaluate the achievement of CDC in the cohort the cumulative incidence of CDC was estimated using the Aalen-Johansen estimator (48). NRM and relapse were considered competing events.
To evaluate associations between IMC and occurrence of relapse, we used a cause-specific Cox regression analysis including RQ-PCR chimerism as a time-varying variable (50, 51). Children with NRM were censored at time of death. The proportional hazards assumption was assessed by the Kolmogorov-type supremum test (52). For analysis of CIR in the subset of children with an episode of IMC, baseline was date of IMC and time was defined as time from IMC to relapse, NRM or censoring at end of follow up.
To analyze the effect of an early IMC on later relapse we fixed a landmark at 90 days post-HCT and categorized children based on the occurrence or not of an IMC between days 30 and 90 post-HCT. Two children with a relapse prior to 90 days were thus excluded from this analysis. CIR was estimated for children with and without IMC prior to 90 days and the time defined as that from 90 days post-HCT to relapse, NRM or censoring.
Analysis was performed with the statistical software package R (The R Foundation for Statistical Computing, Vienna, Austria) version 3.4.2.
3 Results
3.1 Patients and samples
A total of 65 children received an HCT allogeneic during the study period. Informative markers for RQ-PCR chimerism analysis were identified for 64 of 65 patient/donor pairs (Table 1). For one child, insufficient availability of pre-HCT DNA prevented identification of informative markers. The median follow-up time was 2.4 (1.0-4.4) years. In total, 986 samples of whole peripheral blood DNA were analyzed, with a median of 15 (1–23) samples per child. The percentage of missing samples at each timepoint during follow-up is illustrated in online Supplemental Material Table A.
By RQ-PCR, 658 (67%) of the 986 samples showed MC ≥0.01% recipient DNA; furthermore, all children had at least one sample with MC. A total of 215 (22%) samples had MC ≥0.1% recipient DNA. To illustrate the incidence of achieving CDC, the cumulative incidence of CDC below 0.01% and 0.1% is illustrated as online Supplemental Material Figure 1. In total, 60 samples from 27 children had an IMC ≥ 0.1%, and 107 samples from 40 children had an IMC ≥ 0.05% recipient DNA (Figures 1, 2).
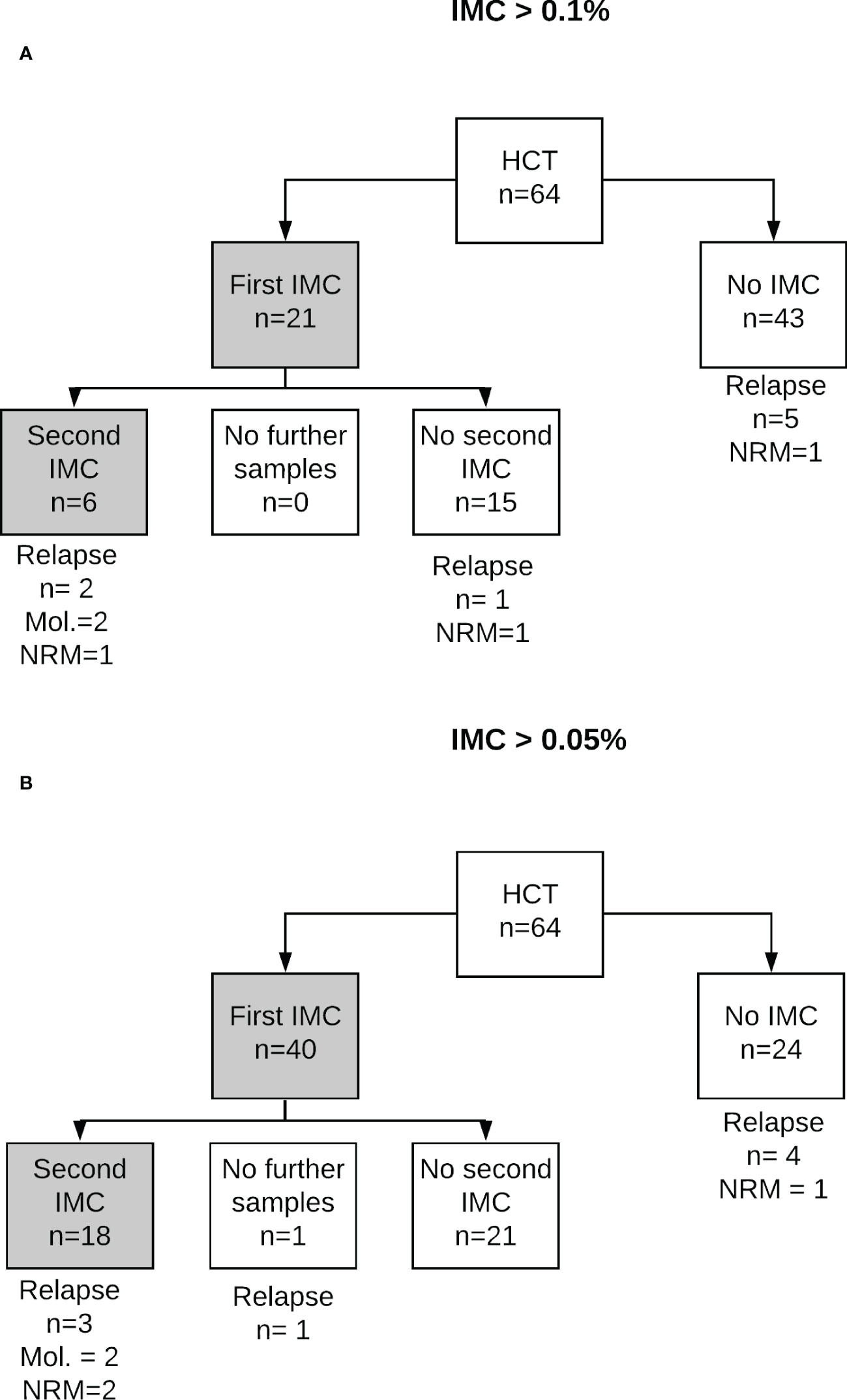
Figure 1 RQ-PCR chimerism results and outcome for two classifications of increasing mixed chimerism. IMC was defined as (A) ≥ 0.1% or (B) ≥ 0.05% recipient DNA. MRD refers to events of detectable MRD above ≥10-3 and less than 5%, interpreted by treated clinician as molecular relapse. IMC, increasing mixed chimerism; NRM, non-relapse mortality; MRD, minimal residual disease.
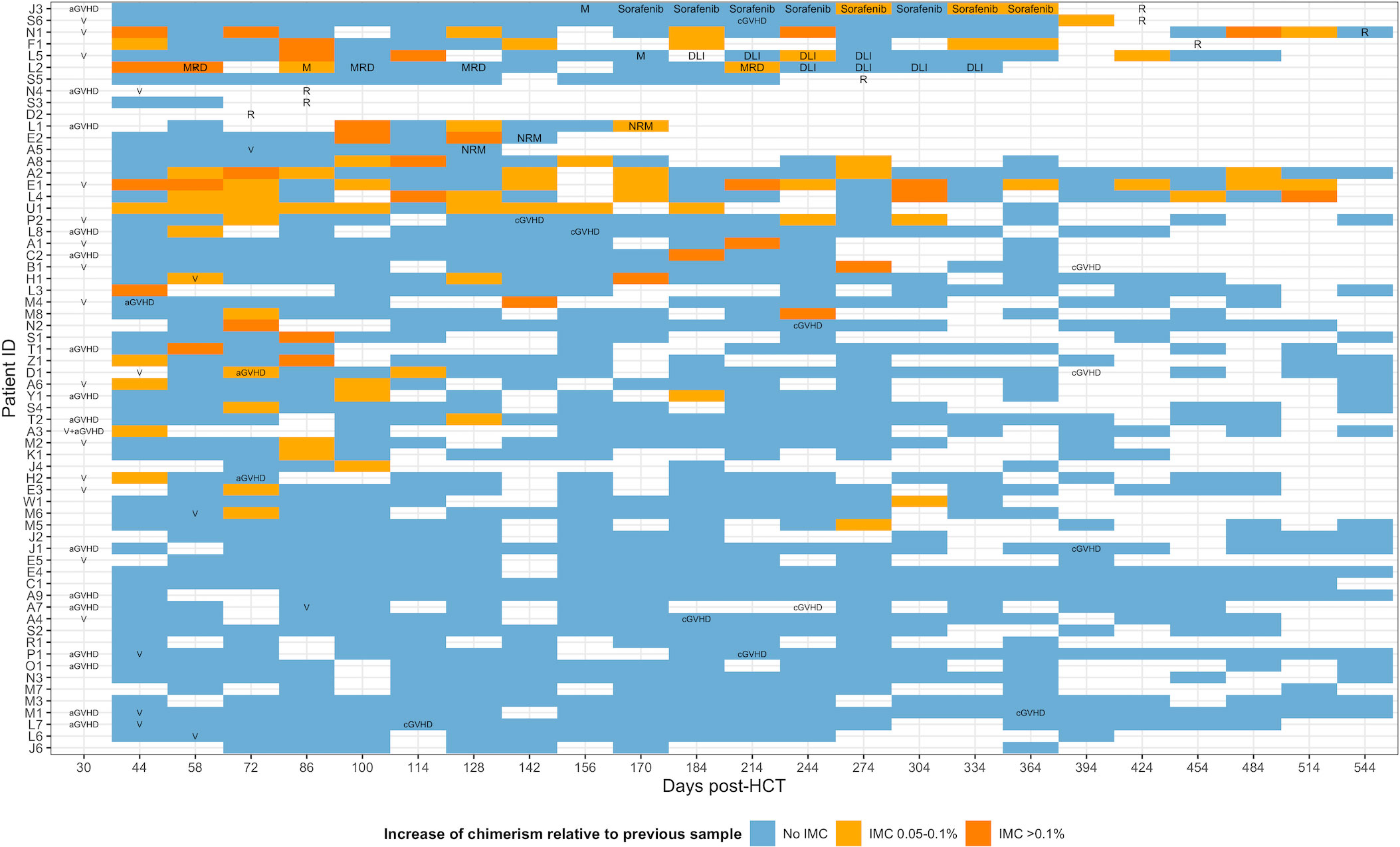
Figure 2 Individual patient profiles illustrating microchimerism, clinical events and transplant outcome. Colors depict level of increasing mixed chimerism as no IMC, IMC < 0.1% and ≥ 0.05% recipient DNA IMC ≥0.1%. Text-labels indicate concurrent diagnosis of relapse, MRD-positivity, acute GVHD grades 2-4, chronic GVHD, identification of viral reactivation (Cytomegalovirus, Adenovirus or Epstein-Barr virus) or detection of MRD in bone marrow. R, relapse; NRM, non-relapse mortality; MRD, detectable minimal residual disease; V, viral reactivation; cGVHD, chronic graft versus host disease; aGVHD, acute graft versus host disease.
3.2 Results by STR-PCR and RQ-PCR chimerism
STR-PCR chimerism was analyzed locally at each center and results were available for all children, with a median of four (1–19) analyses per child and a total of 590 samples with concurrent STR- and RQ-PCR chimerism analysis.
By STR-PCR, MC ≥5% recipient DNA was detected in 20 samples from three children. Of the 590 samples with concurrent STR- and RQ-PCR analysis, 20 (3%) showed MC by both, 179 (30%) were CDC by both and 391 (66%) showed MC by RQ- but CDC by STR-PCR chimerism analysis.
At time of IMC by RQ-PCR, 29 of 33 (88%) samples with concurrent STR-PCR results available showed CDC by STR-PCR, while four of 33 (12%) showed MC.
3.3 Clinical outcome
A total of eight hematological relapses occurred (ALL: n=5, AML: n=3) a median of 11.0 (2.6-31.4) months post-HCT. The 3-year CIR was 14.1% (95% CI 6.2 – 25.3).
Two children received DLI three times, both following MRD-positivity (chronic myeloid leukemia (CML): n=1, AML: n=1). Both were in complete remission at end of follow-up. One child with AML received sorafenib due to detectable MRD prior to progressing to overt relapse. In total, ten children experienced a relapse, MRD-positivity or both.
In total, seven children died, four following relapse and three of NRM (pulmonary hypertension (n=2) and CMV infection (n=1)). The overall survival was 87.3.1% (78.4 – 97.1). Twenty-one children developed acute GVHD (grade II (n=12), grade III (n=6) and grade IV (n=3); three developed limited and ten extensive cGVHD.
There was no association between relapse risk and donor type (MUD/MSD versus other), type of leukemia (acute versus non-acute), disease status at HCT (first complete remission (CR1) versus >CR1) or conditioning regimen (total body irradiation (TBI) versus non-TBI) (data not shown).
3.4 Increasing RQ-PCR chimerism and risk of relapse
The risk of relapse was significantly higher in children with one or more episodes of IMC compared to those without for both classifications of IMC with a hazard ratio (HR) for relapse of 27.8 (95% CI 3.5-135.6; P<.001) and 18.4 (95% CI 2.8-120.5; P=0.002) for children with IMC ≥ 0.1% (n=27) and ≥ 0.05% (n=40), respectively (Table 2). The risk of relapse or MRD-positivity was significantly higher in children with an IMC ≥ 0.1% but not in those with IMC ≥ 0.05%, compared with children without IMC (Table 2).

Table 2 Risk of relapse related to absolute level of RQ-PCR chimerism and two investigated definitions of IMC.
From the first occurrence of an IMC, the 3-year CI of relapse or MRD-positivity was 26.7% (CI 9.4-47.0) and 18.5% (6.4-35.3) for IMC ≥ 0.1% (n=27) and ≥ 0.05% (n= 40), respectively (Figure 3). From the second occurrence of an IMC, the 3-year CI of relapse or MRD-positivity was 63.6% (CI 3.8-93.9) and 38.9% (7.9-70.4) for IMC ≥ 0.1% and ≥ 0.05%, respectively. In the subset of children without IMC ≥ 0.1% and ≥ 0.05%, respectively, the 3 years CIs of relapse or MRD were 16.7% (5.0 -34.1) and 10.8% (3.4 -23.3).
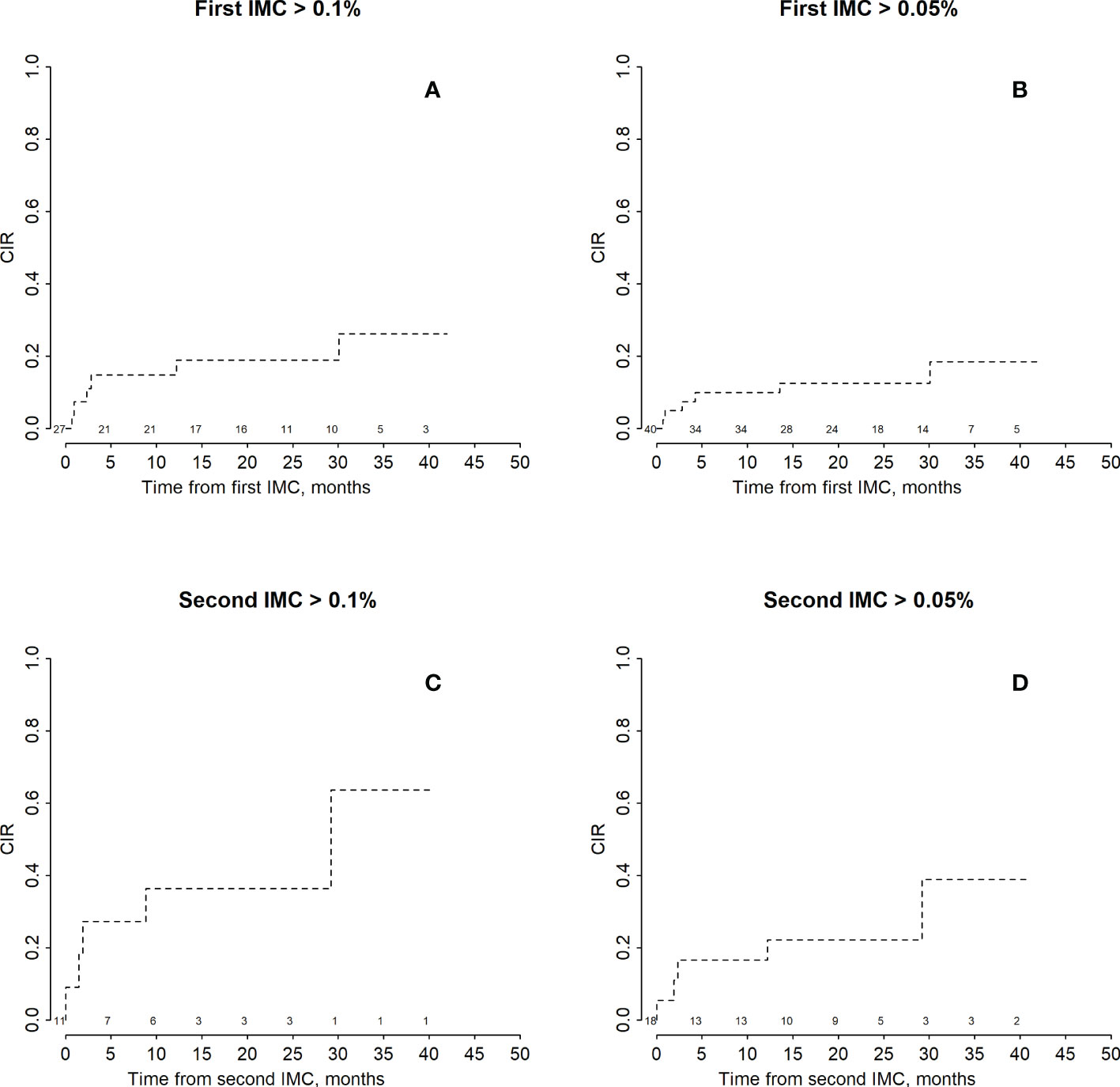
Figure 3 Cumulative incidence of relapse in patients with increasing mixed chimerism. Panels (A, C) show CIR in a subset with recipient chimerism ≥ 0.1% at least once (A) or twice (C) during follow-up. Panels (B, D) show subsets with recipient chimerism ≥ 0.05% recipient DNA at least once (B) or twice (D). Time was defined as months from the first or second episode of IMC, respectively. Numbers at risk are denoted above the x-axis. IMC, increasing mixed chimerism; CIR, cumulative incidence of relapse.
A first IMC ≥ 0.1% and 0.05% was observed between 30 and 916 days prior to relapse, and 86 and 21 days prior to MRD-positivity in two additional children (Table 3).
Four children relapsed without previous IMC, yet of these two had an IMC ≥ 0.1% 9 and 10 days prior to relapse. All four had one or more missing samples prior to the relapse and three of the four children relapsed early (79-91 days post-HCT). The individual profiles of all children with a relapse or MRD-positivity with results of RQ- and STR-PCR chimerism and MRD are provided as online Supplementary Material Figures 2, 3.
In a post-hoc analysis we re-classified IMC ≥ 0.1% and ≥ 0.05% to include only those increases that happened from levels above 0.1 and 0.01% recipient DNA (i.e., not from CDC) to explore if this would provide a better specificity without loss of sensitivity. The association with relapse remained significant but less cases of relapse were detected (data not shown).
3.5 The effect of early mixed chimerism on risk of subsequent relapse
We next estimated the effect of early IMC, defined as prior to day 90, on subsequent risk of relapse or MRD-positivity by creating a landmark at 90 days post-HCT. The children were categorized as with or without an IMC prior to the landmark. Events of IMC after day 90 post-HCT were not considered. By this method, IMC was not associated with an increased CIR (Figure 4). In a post-hoc analysis we analyzed results using an alternative landmark at 120 days post-HCT. This did not affect results (data not shown).
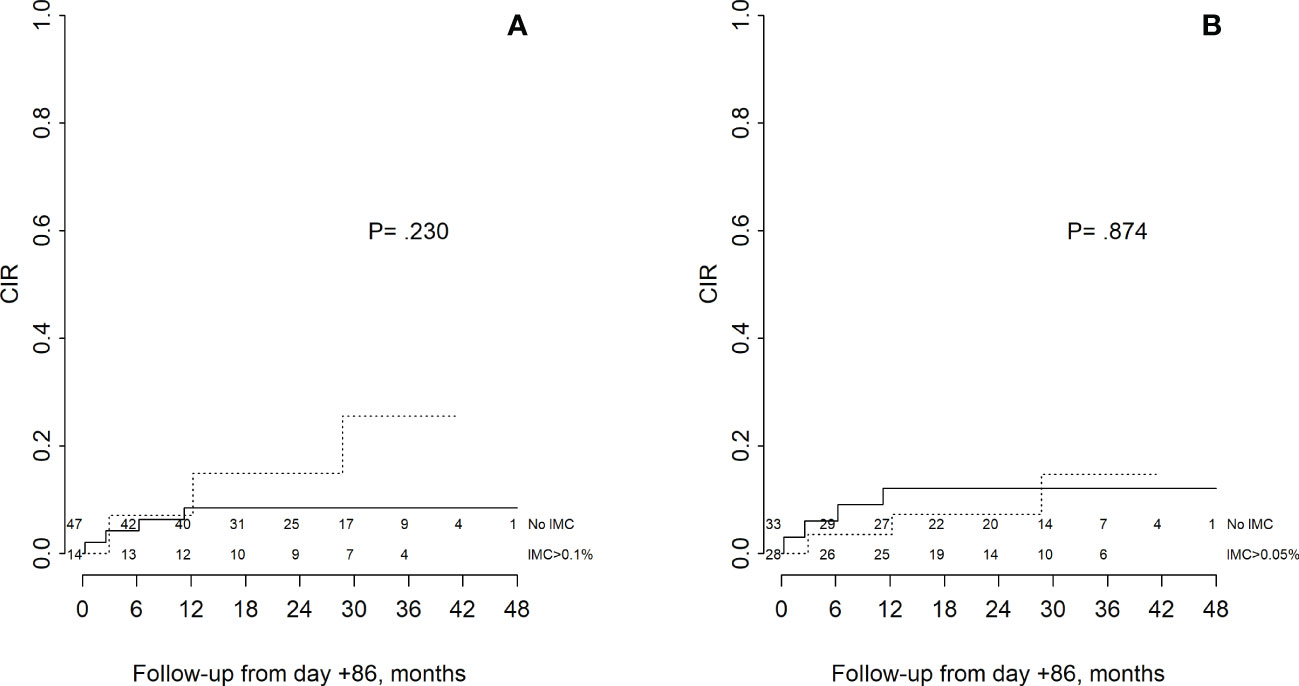
Figure 4 Cumulative incidence of relapse according to IMC prior to 90 days post-HCT. Children were categorized at a landmark of 90 days post-HCT as with or without IMC ≥ 0.1% (A) and ≥ 0.05% (B) prior to the landmark. Time was defined as months from 90 days post-HCT, NRM or censoring and children with relapse or NRM prior to this were excluded. Continuous line depicts children without, dashed line with IMC, respectively. P values derive from comparisons using Gray’s test.
3.6 Increasing STR-PCR chimerism and risk of relapse
Three children had STR-PCR chimerism above 5% in whole blood during follow-up. One child was treated with DLI due to detection of MRD and was in complete remission at end of follow-up, one child died due to NRM and one is in complete remission. STR-PCR chimerism was not associated with risk of relapse but was significantly associated with a composite endpoint of relapse or MRD-positivity (Table 2).
3.7 Minimal residual disease
Results of MRD analysis in BM were available for 61 of 64 children, with a total of 329 samples, 307 of them concurrent with RQ-PCR chimerism in PB and a median of four (1–8) MRD results per child. In total, 19/329 samples from six children had detectable MRD; three samples from two children were positive below quantifiable range (10–4), eight samples from two children were positive below 10-3 and another eight samples from two children were ≥10-3. In 17/19 samples with detectable MRD, RQ-PCR chimerism analysis showed MC 0.01%. Of the six children with detectable MRD, one was treated with sorafenib and subsequently progressed to overt relapse, two received DLI and are in complete remission and three had only a transient MRD below 10-3 and are in complete remission without DLI. Due to the low number of children with detectable MRD and relapse, further analysis was not meaningful to perform.
3.8 Detection of impending relapse by RQ-PCR chimerism, STR-PCR chimerism or minimal residual disease
Among the eight children with a relapse, one was preceded by positive results in the RQ-PCR and MRD analyses and three only by RQ-PCR chimerism analysis. In four children the relapse was not predicted by any method. Among the three children with a molecular relapse, two were preceded by positive results of RQ- and one also by STR-PCR chimerism analysis. Figure 2 depicts the MRD results ≥ 10^-3 in relation to RQ-PCR chimerism. Plots of all cases of relapse and MRD-positivity depicting results of RQ- and STR-PCR chimerism and MRD analysis are available as online Supplemental Material Figure 1.
4 Discussion
Early and precise detection of relapse is essential to decide indications for pre‐emptive treatment or intensified follow-up. RQ‐PCR chimerism is a simple method applicable across all leukemia subtypes. However, the clinical impact of MC and IMC is not yet well‐established, especially among children, and there is no scientific consensus on a clinically relevant cut-off. In general, studies with emphasis on the dynamic changes consistently report an association with relapse (39, 40, 45, 53) whereas some studies of RQ-PCR chimerism at a single or few timepoints do not (37, 42, 54–56). A challenge thus remains in the definition of a positive test that identifies children with a sufficiently high risk of relapse to justify clinical intervention or an intensified follow-up.
In this prospective, multicentre study we tested two classifications of IMC developed in a previous retrospective study (45) and confirm that by RQ-PCR chimerism analysis, an IMC is associated with a higher risk of relapse and two IMCs imply a poor outcome. Importantly, we did not include samples taken within 30 days before relapse, as we would thus be testing the ability of RQ‐PCR chimerism to identify an overt rather than an impending relapse. Using an IMC ≥ 0.05% provided no benefits compared to ≥ 0.1% but entailed more false positive results. Based on this we recommend the use of IMC ≥ 0.1% or a more than one log increase between two samples as a clinical cut-off.
In four of eight children with relapse, relapse was not preceded by IMC. In all four, STR-PCR chimerism and MRD analysis were negative up to the relapse. Importantly, three of the four events occurred early, and two children had only one sample taken prior to relapse, thus excluding the possibility of detecting IMC between two samples. All four had missing samples prior to-relapse. Furthermore, two children had a detectable IMC not included in the analysis because it occurred within 30 days of relapse. Our results thus suggest that causes of false-negative results could be explained by non-adherence to sample protocol and a rapid development of the relapse (57). As illustrated in online Supplemental Material Table A, protocol adherence declined during follow-up and could to some extent explain false negative as well as true negative results. By inclusion of a time-factor in the definition of IMC and calculation the degree of IMC per time unit, the data might be less sensitive to missing samples. One study defined an increment factor based on the course of chimerism kinetics over time, calculating time as days between current sample and last minimum or maximum value preceding relapse (53). While valuable in the attempt to clarify the various causes of IMC, such attempts can be difficult to translate into a clinical setting where future events are unknown. Further, for some subtypes of neoplasms, relapse may develop within the course of weeks, and in these cases frequent samples during follow-up is essential with or without the use of an increment factor. We here describe data from a heterogenous cohort, with insufficient power to adjust for pre-HCT disease. The relative impact of pre-HCT on the interval between IMC and relapse is not known and studies designed to explore this are warranted.
The association between sequential analysis of STR-PCR chimerism and relapse is well documented (7, 54, 58, 59). We found an association only when including detectable MRD in a composite endpoint. Several factors could explain this. STR-PCR chimerism was analysed at fewer time-points than RQ-PCR chimerism. We omitted samples closer than 30 days to relapse, an important difference from most studies of STR-PCR chimerism. Very few samples were positive by STR-PCR and with the low number of events our analysis is likely underpowered. Still, our results are in line with our previous, retrospective study in which RQ- and STR-PCR chimerism were analysed at the same timepoints, suggesting that STR-PCR is not sensitive enough to detect an impending relapse (45).
One clear benefit of STR-PCR chimerism analysis is its precision at levels above 5-10%, at which the RQ-PCR methodology has a low accuracy. In our study, this is reflected by large discrepancies between STR-PCR and RQ-PCR results at levels of recipient DNA above 5%, as shown in Supplemental Material Figure C. Tyler et al. suggested a personalized approach to chimerism analysis using the two methods interchangeably, depending on the level of recipient DNA (46). A more simple approach could be the application of digital PCR or NGS technology for chimerism analysis, as these techniques provide excellent accuracy also at high levels of recipient DNA and with comparable sensitivity to RQ-PCR chimerism analysis (24, 25, 60, 61).
Post-HCT MRD impacts relapse risk and survival among pediatric patients (16, 62–69). In a comprehensive analysis of the prognostic value of peri-HCT MRD in pediatric ALL Bader et al. found detectable MRD at any level post-HCT to be highly predictive of a relapse surpassing the impact of pre-HCT MRD (68). In most of the studies of post-HCT MRD however, a large fraction of relapses remain undetected (16, 65, 68, 70, 71). In the present study, MRD analysis in BM identified two children with a molecular relapse, and one of eight children with a relapse. This could be explained by the limited number of BM analyses during follow-up, and the fact that we did not include samples closer than 30 days to a relapse. Further, in our cohort, the method of MRD analysis varied greatly between the subgroups and our study was not designed to evaluate the prognostic value of MRD. MRD analysis can be performed on PB, allowing for more frequent analyses than in BM. Studies have shown comparable results for BM and PB for T-cell ALL and AML, but not pre-B-ALL (70, 72–74). In a recent study, Jacobsohn et al. found a difference in the OS between children with and without detectable Wilms’ tumour 1 (WT1) gene at day 42 post-HCT in BM, but not in PB (16). A recent study combined NGS-based MRD analysis with RQ-PCR chimerism in PB of adults with AML and with this approach six of eight events of relapse were detected (75).
Importantly, applying RQ-PCR chimerism and MRD analyses to PB reflects two separate approaches that are clearly not interchangeable. It remains unknown to what degree detectable RQ-PCR chimerism post-HCT reflects persistence of the malignant clone or benign haematopoiesis, but most likely it is a combination. The efficacy of HCT relies in large part on the ability of donor T cells to eliminate residual disease present at HCT through the graft versus leukemia (GVL) effect. A prevailing hypothesis is that chimerism analysis is an indirect marker of the alloreactivity of the graft, thus that even if detectable MC is caused by benign recipient haematopoiesis this might be linked to a decline in GVL effect. In a study of lineage-specific STR-PCR chimerism, Bader et al. were able to show that MC prior to relapse mainly debuted in T-cells and/or monocytes, subsequently in granulocytes, independent of the type of leukemia in question (76). Roux et al. found already in 1993 that a relapse of CML was preceded by MC in the mononuclear cell fraction (77). Thus, IMC could reflect an increasing tolerance of the graft eventually paving the way for a relapse (76, 77). A recent study described exhausted, functionally impaired, leukemia specific T-cells present in the BM of patients with AML relapsing after HCT, but not in patients in long-term remission illustrating the importance of durable immunosurveillance for disease-control (78). On the other hand, persistence of chimerism might in turn directly modulate tolerance (79, 80). In contrast, in a recent study of RQ-PCR chimerism in CD3 negative mononuclear cells of PB in adults with AML, IMC and MC were both associated with higher risk of relapse, suggesting a more direct detection of the malignant clone (81). Studies on the dynamics between RQ-PCR chimerism and immune recovery post-HCT are warranted.
We previously found that RQ-PCR chimerism analysis during the first 90 days post-HCT did not identify children with increased relapse risk (45). In the current study, we confirm these results in a setting with predefined sample frequency and a higher number of samples per child (median of 15 vs 10). Previous studies in adult cohorts have found no association between RQ-PCR chimerism early post-HCT and relapse risk (42), and one study linked early events of IMC to viral infections (53). Interestingly, in a pediatric-adult cohort of patients transplanted for AML, Wiedemann et al. found that achieving CDC later than 50 days post-HCT was associated with a lower 2-year relapse-risk (13% vs. 51%; p = 0.002). One could speculate that the velocity of achieving CDC post-HCT reflects graft alloreactivity in a period early post-HCT where events of IMC have multiple causes.
Early detection of impending relapse post-HCT has in large part gained interest due to the rapid increase of possibly curative interventions. Studies on STR-PCR chimerism guided immunomodulatory therapy in children (accelerated tapering of immunosuppressive therapy or DLI) have been able to achieve improve outcomes for children with MC (8, 58, 82–84). Changes in post-HCT immunosuppression guided by RQ-PCR chimerism have led to improved survival rates and/or reversion of MC in single-center studies of adult patients with leukemia (34, 40, 41). The rapid development of novel targeted or immunomodulatory therapies may provide much-needed alternatives, in some cases as a bridge to a second HCT (85). In B-cell precursor ALL (pre B-ALL) this development has been particularly impressive and CD19-targeted strategies including blinatumomab, Fc-optimized CD19 antibodies (86) and most recently CAR-T therapy have been added to the repertoire (87). For most treatment options, an early intervention is critical for outcome (88, 89). Further, treatment efficacy might rely on low tumor burden (88). Using the current strategies for MRD analysis, the interval between detectable MRD and relapse may be too short to allow for pre-emptive strategies due to rapid progression (71). In the current study, we establish that IMC by RQ-PCR chimerism identifies children with a high risk of relapse, with a time-interval allowing for clinical intervention. However, prospective studies of RQ-PCR chimerism guided interventions in children are warranted, as are those on the possible relationship between MC, IMC and GVL effect.
4.1 Limitations
This study is limited mainly by the small size and low number of events. This limited our possibility to adjust for confounders or to perform subgroup analysis. Three children received treatment for imminent relapse post-HCT that might impact our results. Furthermore, there was a discrepancy between the number of samples analyzed by RQ- and STR-PCR chimerism, complicating the comparison between the two methods. Finally, there were missing samples during follow-up, treated as missing-at random.
4.2 Conclusions
In conclusion, we confirmed that IMC by RQ-PCR chimerism analysis in PB appears to be associated with an increased risk of relapse. An IMC of ≥ 0.1% or more than one log increase from the previous sample was detectable at an interval prior to relapse to allow for a clinical intervention and/or intensified follow-up. Ultimately, the effect of chimerism-guided intervention can only be determined in prospective trials with pre-defined classifications and standardized interventions. Based on our results, we suggest an individualized approach to follow-up based on biweekly RQ-PCR chimerism analysis in PB until 6 months post-HCT, then monthly until 18 months post-HCT. If IMC ≥ 0.1% is detected, biweekly RQ-PCR chimerism analysis is performed until CDC. If a second IMC ≥ 0.1% is detected, MRD is analyzed in BM. While biweekly or even monthly MRD analysis in BM is unfeasible in children, increasing the frequency of BM MRD analysis based on the occurrence of IMC could possibly identify children at higher risk of relapse eligible for intensified follow-up.
Data availability statement
The raw data supporting the conclusions of this article will be made available by the authors, without undue reservation.
Ethics statement
The study was performed in accordance with the Declaration of Helsinki and approved by the Danish Data Protection Agency (ID 30-1319), the Ethical Committees of the Capital Region of Denmark (H-3-2014-FSP21), the Regional Ethical Committee of South East Norway (2015/2421/REK sør-øst), the Central Ethical Review Board of Gothenburg, Sweden (618–15), and the regional ethical committee of Helsinki and Uusimaa Hospital District, Finland (II/15). In Denmark, written informed consent from the participants’ legal guardian/next of kin was not required to participate in this study in accordance with the national legislation and the institutional requirements.
Author contributions
AH, HM, TM, KV, JB, KM, DT, SR, HM, CH, KGM, MI conceived of and designed the study. AH, TM, KV, JB, KM, DT, CH, MI collected the data. AH, HM conducted the experiments. AH, SR performed the formal analysis. AH, HM, TM, KV, JB, KM, DT, SR, HM, CH, KGM, MI interpreted the data. AH drafted the manuscript. All authors have critically revised the manuscript. All authors contributed to the article and approved the submitted version.
Funding
This study was supported by the Danish Childhood Cancer Foundation, Swedish Childhood Cancer Fund, Danish Cancer Society, The Norwegian Childhood Cancer Foundation, Dagmar Marshalls Fund and Engineer Otto Christensen’s Fund. These had no role in the study design, data collection or analysis, preparation of the manuscript, or decision to submit.
Acknowledgments
The authors would like to thank the families treated at the Nordic transplant centers participating in this study.
Conflict of interest
The authors declare that the research was conducted in the absence of any commercial or financial relationships that could be construed as a potential conflict of interest.
Publisher’s note
All claims expressed in this article are solely those of the authors and do not necessarily represent those of their affiliated organizations, or those of the publisher, the editors and the reviewers. Any product that may be evaluated in this article, or claim that may be made by its manufacturer, is not guaranteed or endorsed by the publisher.
Supplementary material
The Supplementary Material for this article can be found online at: https://www.frontiersin.org/articles/10.3389/frhem.2023.1055484/full#supplementary-material
Supplementary Figure 1 | Cumulative incidence of complete donor chimerism below 0.1% and 0.01% recipient DNA.
Supplementary Figure 2 | Individual patient profiles of four children with relapse not detected by IMC >0.1 or 0.05% by RQ-PCR chimerism analysis. For clarity, MRD results described as “undetected” are set to 0, regardless of the sensitivity of the applied method.
Supplementary Figure 3 | Individual patient profiles of six children were relapse or molecular relapse was detected by IMC >0.1 or 0.05% by RQ-PCR chimerism analysis. For clarity MRD results described as “undetected” are given the value 0.
References
1. Horowitz M, Schreiber H, Elder A, Heidenreich O, Vormoor J, Toffalori C, et al. Epidemiology and biology of relapse after stem cell transplantation. Bone Marrow Transplant (2018) 53:1379–89. doi: 10.1038/s41409-018-0171-z
2. Lie SO, Abrahamsson J, Clausen N, Forestier E, Hasle H, Hovi L, et al. Long-term results in children with AML: NOPHO-AML study group–report of three consecutive trials. Leukemia (2005) 19:2090–100. doi: 10.1038/sj.leu.2403962
3. Peters C, Schrappe M, von Stackelberg A, Schrauder A, Bader P, Ebell W, et al. Stem-cell transplantation in children with acute lymphoblastic leukemia: A prospective international multicenter trial comparing sibling donors with matched unrelated donors the ALL-SCT-BFM-2003 trial. J Clin Oncol (2015) 33:1265–74. doi: 10.1200/JCO.2014.58.9747
4. Kuhlen M, Willasch AM, Dalle J-H, Wachowiak J, Yaniv I, Ifversen M, et al. Outcome of relapse after allogeneic HSCT in children with ALL enrolled in the ALL-SCT 2003/2007 trial. Br J Haematol (2018) 180:82–9. doi: 10.1111/bjh.14965
5. Weisdorf D. The role of second transplants for leukemia. Best Pract Res Clin Haematol (2016) 29:359–64. doi: 10.1016/j.beha.2016.10.011
6. Lund TC, Ahn KW, Tecca HR, Hilgers MV, Abdel-Azim H, Abraham A, et al. Outcomes after second hematopoietic cell transplantation in children and young adults with relapsed acute leukemia. Biol Blood Marrow Transplant (2019) 25:301–6. doi: 10.1016/j.bbmt.2018.09.016
7. Bader P, Kreyenberg H, Hoelle W, Dueckers G, Handgretinger R, Lang P, et al. Increasing mixed chimerism is an important prognostic factor for unfavorable outcome in children with acute lymphoblastic leukemia after allogeneic stem-cell transplantation: possible role for pre-emptive immunotherapy? J Clin Oncol (2004) 22:1696–705. doi: 10.1200/JCO.2004.05.198
8. Rettinger E, Willasch AM, Kreyenberg H, Borkhardt A, Holter W, Kremens B, et al. Preemptive immunotherapy in childhood acute myeloid leukemia for patients showing evidence of mixed chimerism after allogeneic stem cell transplantation. Blood (2011) 118:5681–8. doi: 10.1182/blood-2011-04-348805
9. Maude SL, Laetsch TW, Buechner J, Rives S, Boyer M, Bittencourt H, et al. Tisagenlecleucel in children and young adults with b-cell lymphoblastic leukemia. N Engl J Med (2018) 378:439–48. doi: 10.1056/NEJMoa1709866
10. Garand R, Beldjord K, Cavé H, Fossat C, Arnoux I, Asnafi V, et al. Flow cytometry and IG/TCR quantitative PCR for minimal residual disease quantitation in acute lymphoblastic leukemia: A French multicenter prospective study on behalf of the FRALLE, EORTC and GRAALL. Leukemia (2013) 27:370–6. doi: 10.1038/leu.2012.234
11. Theunissen P, Mejstrikova E, Sedek L, van der Sluijs-Gelling AJ, Gaipa G, Bartels M, et al. Standardized flow cytometry for highly sensitive MRD measurements in b-cell acute lymphoblastic leukemia. Blood (2017) 129:347–57. doi: 10.1182/blood-2016-07-726307
12. Tierens A, Bjørklund E, Siitonen S, Marquart HV, Wulff-Juergensen G, Pelliniemi T-T, et al. Residual disease detected by flow cytometry is an independent predictor of survival in childhood acute myeloid leukaemia; results of the NOPHO-AML 2004 study. Br J Haematol (2016) 174:600–9. doi: 10.1111/bjh.14093
13. Skou AS, Juul-Dam KL, Ommen HB, Hasle H. Peripheral blood molecular measurable residual disease is sufficient to identify patients with acute myeloid leukaemia with imminent clinical relapse. Br J Haematol (2021) 195:310–27. doi: 10.1111/BJH.17449
14. Buldini B, Rizzati F, Masetti R, Fagioli F, Menna G, Micalizzi C, et al. Prognostic significance of flow-cytometry evaluation of minimal residual disease in children with acute myeloid leukaemia treated according to the AIEOP-AML 2002/01 study protocol. Br J Haematol (2017) 177:116–26. doi: 10.1111/bjh.14523
15. Rubnitz JE, Inaba H, Dahl G, Ribeiro RC, Bowman WP, Taub J, et al. Minimal residual disease-directed therapy for childhood acute myeloid leukaemia: results of the AML02 multicentre trial. Lancet Oncol (2010) 11:543–52. doi: 10.1016/S1470-2045(10)70090-5
16. Jacobsohn DA, Loken MR, Fei M, Adams A, Brodersen LE, Logan BR, et al. Outcomes of measurable residual disease in pediatric acute myeloid leukemia before and after hematopoietic stem cell transplant: Validation of difference from normal flow cytometry with chimerism studies and wilms tumor 1 gene expression. Biol Blood Marrow Transplant (2018) 24:2040–6. doi: 10.1016/j.bbmt.2018.06.010
17. E. S, H. D, F. W. Quantitative analysis of chimerism after allogeneic stem cell transplantation by PCR amplification of microsatellite markers and capillary electrophoresis with flourescence detection: The Vienna experience. Leukemia (2003) 17:224–7. doi: 10.1038/sj.leu.2402756
18. Thiede C, Bornhäuser M, Oelschlägel U, Brendel C, Leo R, Daxberger H, et al. Sequential monitoring of chimerism and detection of minimal residual disease after allogeneic blood stem cell transplantation (BSCT) using multiplex PCR amplification of short tandem repeat-markers. Leukemia (2001) 15:293–302. doi: 10.1038/sj.leu.2401953
19. Baron F, Sandmaier BM FB. Chimerism and outcomes after allogeneic hematopoietic cell transplantation following nonmyeloablative conditioning. Leukemia (2006) 20:1690–700. doi: 10.1038/sj.leu.2404335
20. Bader P, Niethammer D, Willasch A, Kreyenberg H, Klingebiel T. How and when should we monitor chimerism after allogeneic stem cell transplantation? Bone Marrow Transplant (2005) 35:107–19. doi: 10.1038/sj.bmt.1704715
21. Thiede C, Bornhauser M, Ehninger G, C. T, M. B. Strategies and clinical implications of chimerism diagnostics after allogeneic hematopoietic stem cell transplantation. Acta Haematol (2004) 112:16–23. doi: 10.1159/000077555
22. Bader P, Willasch A, Klingebiel T. Monitoring of post-transplant remission of childhood malignancies: Is there a standard? Bone Marrow Transplant (2008) 42 Suppl 2:S31–4. doi: 10.1038/bmt.2008.280
23. Bacher U, Haferlach T, Fehse B, Schnittger S, Kroger N, Kröger N. Minimal residual disease diagnostics and chimerism in the post-transplant period in acute myeloid leukemia. ScientificWorldJournal (2011) 11:310–9. doi: 10.1100/tsw.2011.16
24. Pedini P, Cherouat N, Basire A, Simon S, Budon L, Pourtein M, et al. Evaluation of next-generation sequencing and crystal digital PCR for chimerism monitoring of post-allogeneic hematopoietic stem cell transplantation. Biol Blood Marrow Transplant (2020) 27(1):89.e1–89.e10. doi: 10.1016/j.bbmt.2020.09.023
25. Pettersson L, Vezzi F, Vonlanthen S, Alwegren K, Hedrum A, Hauzenberger D. Development and performance of a next generation sequencing (NGS) assay for monitoring of mixed chimerism. Clin Chim Acta (2021) 512:40–8. doi: 10.1016/j.cca.2020.10.034
26. Alizadeh M. Quantitative assessment of hematopoietic chimerism after bone marrow transplantation by real-time quantitative polymerase chain reaction. Blood (2002) 99:4618–25. doi: 10.1182/blood.V99.12.4618
27. Wu D, Kanaan SB, Penewit K, Waalkes A, Urselli F, Nelson JL, et al. Ultrasensitive quantitation of genomic chimerism by single-molecule molecular inversion probe capture and high throughput sequencing of copy number deletion polymorphisms. J Mol Diagn (2021) 24(2):167–76. doi: 10.1016/J.JMOLDX.2021.10.005
28. Blouin AG, Askar M. Chimerism analysis for clinicians: a review of the literature and worldwide practices. Bone Marrow Transplant (2022) 57:347–59. doi: 10.1038/S41409-022-01579-9
29. Haugaard AK, Kofoed J, Masmas TN, Madsen HO, Marquart HV, Heilmann C, et al. Is microchimerism a sign of imminent disease recurrence after allogeneic hematopoietic stem cell transplantation? A systematic review of the literature. Blood Rev (2020) 44. doi: 10.1016/j.blre.2020.100673
30. Wiedemann B, Klyuchnikov E, Kröger N, Zabelina T, Stahl T, Zeschke S, et al. Chimerism studies with quantitative real-time PCR in stem cell recipients with acute myeloid leukemia. Exp. Hematol (2010) 38:1261–71. doi: 10.1016/j.exphem.2010.08.006
31. Itälä-Remes M, Leino J, Kairisto V. Comparison of microsatellite-PCR and quantitative alleleSEQR PCR for chimerism detection in patients with high relapse risk AML/MDS treated with pre-emptive post-transplant immunomodulation. London, UK: Poster Present 39 th Annu Meet Eur Gr Blood Marrow Transplantation (2013).
32. Vago L, Mazzi B, Chiesa L, Greco R, Crucitt L, Tettamanzi V, et al. High-sensitivity haematopoietic chimerism by qPCR for relapse prediction and specifi c identifi cation of HLA loss leukaemic variants. London, UK: Oral Present Present 39 th EBMT Annu Meet p. 7–10 (2013).
33. Kletzel M, Huang W, Olszewski M, Khan S. Validation of chimerism in pediatric recipients of allogeneic hematopoietic stem cell transplantation (HSCT) a comparison between two methods: real-time PCR (qPCR) vs. variable number tandem repeats PCR (VNTR PCR). Chimerism (2013) 4:1–8. doi: 10.4161/chim.23158
34. Jacque N, NGuyen S, Golmard J, Garnier A, Uzunov M, Vernant J, et al. Prediction of relapse and impact of immunomodulation based on quantitative RT-PCR of insertion/deletion polymorphism for chimerism analysis in acute leukaemia. London, UK: Oral Present Present 39 th EBMT Annu Meet 2013 p. 7–10 (2013).
35. Horky O, Mayer J, Kablaskova L, Razga F, Krejci M, Kissova J, et al. Increasing hematopoietic microchimerism is a reliable indicator of incipient AML relapse. Int J Lab. Hematol (2011) 33:57–66. doi: 10.1111/j.1751-553X.2010.01249.x
36. Willasch AM, Rettinger E, Zabel M, Kreyenberg, Hermann Meyer V, Borkhardt A, et al. Clinical impact of post-transplant chimerism monitoring in CD33/34 bone marrow subpopulations and whole blood in pediatric AML: Prospective comparison of highly sensitive real time sequence polymorphism PCR versus gold-standard conventional STR-PCR. Blood (2013) 122; 21:411. doi: 10.1182/blood.V122.21.411.411
37. Willasch AM, Kreyenberg H, Shayegi N, Rettinger E, Meyer V, Zabel M, et al. Monitoring of hematopoietic chimerism after transplantation for pediatric myelodysplastic syndrome: Real-time or conventional short tandem repeat PCR in peripheral blood or bone marrow? Biol. Blood Marrow Transplant (2014) 20:1918–25. doi: 10.1016/j.bbmt.2014.07.030
38. Willasch A, Schneider G, Reincke BS, Shayegi N, Kreyenberg H, Kuci S, et al. Sequence polymorphism systems for quantitative real-time polymerase chain reaction to characterize hematopoietic chimerism-high informativity and sensitivity as well as excellent reproducibility and precision of measurement. Lab Hematol (2007) 13:73–84. doi: 10.1532/LH96.07004
39. Jacque N, Nguyen S, Golmard J, Uzunov M, Garnier A, Leblond V, et al. Chimerism analysis in peripheral blood using indel quantitative real-time PCR is a useful tool to predict post-transplant relapse in acute leukemia. Bone Marrow Transplant (2014) 50:1–7. doi: 10.1038/bmt.2014.254
40. Qin X-Y, Li G-X, Qin Y-Z, Wang Y, Wang F-R, Liu D-H, et al. Quantitative chimerism: an independent acute leukemia prognosis indicator following allogeneic hematopoietic SCT. Bone Marrow Transplant (2014) 49:1269–77. doi: 10.1038/bmt.2014.158
41. Ahci M, Stempelmann K, Buttkereit U, Crivello P, Trilling M, Heinold A, et al. Clinical utility of quantitative PCR for chimerism and engraftment monitoring after allogeneic stem cell transplantation for hematologic malignancies. Biol Blood Marrow Transplant (2017) 23:1658–68. doi: 10.1016/j.bbmt.2017.05.031
42. Elkaim E, Picard C, Galambrun C, Barlogis V, Loundou A, Curtillet C, et al. Peripheral blood cells chimerism after unrelated cord blood transplantation in children: Kinetics, predictive factors and impact on post-transplant outcome. Br J Haematol (2014) 166:557–65. doi: 10.1111/bjh.12918
43. Kreyenberg H, Hölle W, Möhrle S, Niethammer D, Bader P. Quantitative analysis of chimerism after allogeneic stem cell transplantation by PCR amplification of microsatellite markers and capillary electrophoresis with fluorescence detection: the tuebingen experience. Leukemia (2003) 17:237–40. doi: 10.1038/sj.leu.2402761
44. van der Velden VHJ, Hochhaus A, Cazzaniga G, Szczepanski T, Gabert J, van Dongen JJM. Detection of minimal residual disease in hematologic malignancies by real-time quantitative PCR: Principles, approaches, and laboratory aspects. Leukemia (2003) 17:1013–34. doi: 10.1038/sj.leu.2402922
45. Haugaard AK, Madsen HO, Marquart HV, Rosthøj S, Masmas TN, Heilmann C, et al. Highly sensitive chimerism detection in blood is associated with increased risk of relapse after allogeneic hematopoietic cell transplantation in childhood leukemia. Pediatr. Transplant (2019):e13549. doi: 10.1111/petr.13549
46. Tyler J, Kumer L, Fisher C, Casey H, Shike H. Personalized chimerism test that uses selection of short tandem repeat or quantitative PCR depending on patient’s chimerism status. J Mol Diagnostics (2019) 21:483–90. doi: 10.1016/j.jmoldx.2019.01.007
47. van der Velden VHJ, Cazzaniga G, Schrauder A, Hancock J, Bader P, Panzer-Grumayer ER, et al. Analysis of minimal residual disease by Ig/TCR gene rearrangements: guidelines for interpretation of real-time quantitative PCR data. Leukemia (2007) 21:604–11. doi: 10.1038/sj.leu.2404586
48. Aalen OO, Johansen S. An empirical transition matrix for non-homogeneous Markov chains based on censored observations. Scand J Stat (1978) 5:141–50. doi: 10.2307/4615704
49. Gray RJ. A class of K-sample tests for comparing the cumulative incidence of a competing risk. Ann Stat (1988) 16:1141–54. doi: 10.2307/2241622
50. Iacobelli S, Statistical Committee EBMT. Suggestions on the use of statistical methodologies in studies of the European group for blood and marrow transplantation. Bone Marrow Transplant. (2013) 48:S1–S37. doi: 10.1038/bmt.2012.282
51. Cortese G, Andersen PK. Competing risks and time-dependent covariates. Biometrical J (2009) 52:NA–A. doi: 10.1002/bimj.200900076
52. Lin DY, Wei LJ, Ying Z. Checking the cox model with cumulative sums of martingale-based residuals. Biometrika (1993) 80:557. doi: 10.2307/2337177
53. Sellmann L, Rabe K, Bünting I, Dammann E, Göhring G, Ganser A, et al. Diagnostic value of highly-sensitive chimerism analysis after allogeneic stem cell transplantation. Bone Marrow Transplant (2018) 53(11)1457–65. doi: 10.1038/s41409-018-0176-7
54. Bader P, Beck J, Frey A, Schlegel PG, Hebarth H, Handgretinger R, et al. Serial and quantitative analysis of mixed hematopoietic chimerism by PCR in patients with acute leukemias allows the prediction of relapse after allogeneic BMT. Bone Marrow Transplant (1998) 21:487–95. doi: 10.1038/sj.bmt.1701119
55. Schaap N, Schattenberg A, Bär B, Mensink E, de Man A, Geurts van Kessel A, et al. Red blood cell phenotyping is a sensitive technique for monitoring chronic myeloid leukaemia patients after T-cell-depleted bone marrow transplantation and after donor leucocyte infusion. Br J Haematol (2000) 108:116–25. doi: 10.1046/j.1365-2141.2000.01803.x
56. Schattenberg A, De Witte T, Salden M, Vet J, Van Dijk B, Smeets D, et al. Mixed hematopoietic chimerism after allogeneic transplantation with lymphocyte-depleted bone marrow is not associated with a higher incidence of relapse. Blood (1989) 73:1367–72.
57. Lindahl H, Vonlanthen S, Valentini D, Björklund AT, Sundin M, Mielke S, et al. Lineage-specific early complete donor chimerism and risk of relapse after allogeneic hematopoietic stem cell transplantation for acute myeloid leukemia. Bone Marrow Transplant (2022) 57:753–9. doi: 10.1038/S41409-022-01615-8
58. Bader P, Kreyenberg H, Hoelle W, Dueckers G, Kremens B, Dilloo D, et al. Increasing mixed chimerism defines a high-risk group of childhood acute myelogenous leukemia patients after allogeneic stem cell transplantation where pre-emptive immunotherapy may be effective. Bone Marrow Transplant (2004) 33:815–21. doi: 10.1038/sj.bmt.1704444
59. Preuner S, Peters C, Pötschger U, Daxberger H, Fritsch G, Geyeregger R, et al. Risk assessment of relapse by lineage-specific monitoring of chimerism in children undergoing allogeneic stem cell transplantation for acute lymphoblastic leukemia. Haematologica (2016) 101:741–6. doi: 10.3324/haematol.2015.135137
60. Waterhouse M, Pfeifer D, Follo M, Duyster J, Schäfer H, Bertz H, et al. Early mixed hematopoietic chimerism detection by digital droplet PCR in patients undergoing gender-mismatched hematopoietic stem cell transplantation. Clin Chem Lab Med (2017) 55:1115–21. doi: 10.1515/cclm-2016-0900
61. Fortschegger M, Preuner S, Printz D, Poetsch AR, Geyeregger R, Pichler H, et al. Detection and monitoring of lineage-specific chimerism by digital droplet PCR-based testing of Deletion/Insertion polymorphisms. Biol Blood Marrow Transplant (2020) 26:1218–24. doi: 10.1016/j.bbmt.2020.02.016
62. Lamble A, Phelan R, Burke M. When less is good, is none better? the prognostic and therapeutic significance of peri-transplant minimal residual disease assessment in pediatric acute lymphoblastic leukemia. J Clin Med (2017) 6:66. doi: 10.3390/jcm6070066
63. Bader P, Kreyenberg H, von Stackelberg A, Eckert C, Salzmann-Manrique E, Meisel R, et al. Monitoring of minimal residual disease after allogeneic stem-cell transplantation in relapsed childhood acute lymphoblastic leukemia allows for the identification of impending relapse: results of the ALL-BFM-SCT 2003 trial. J Clin Oncol (2015) 33:1275–84. doi: 10.1200/JCO.2014.58.4631
64. Lavrinenko VA, Meleshko AN, Lutskovich DV, Mareiko YE, Prudnikov DV, Aleynikova OV, et al. Minimal residual disease monitoring by RQPCR of Ig/TCR rearrangements: an effective method to predict relapse in children with acute lymphoblastic leukemia after allogeneic hematopoietic stem cell transplantation. Cell Ther Transplant (2018) 7:47–60. doi: 10.18620/ctt-1866-8836-2018-7-4-47-60
65. Lovisa F, Zecca M, Rossi B, Campeggio M, Magrin E, Giarin E, et al. Pre- and post-transplant minimal residual disease predicts relapse occurrence in children with acute lymphoblastic leukaemia. Br J Haematol (2018) 180:680–93. doi: 10.1111/bjh.15086
66. Umeda K, Iwai A, Kawaguchi K, Mikami M, Nodomi S, Saida S, et al. Impact of post-transplant minimal residual disease on the clinical outcome of pediatric acute leukemia. Pediatr Transplant (2017) 21:e12926. doi: 10.1111/petr.12926
67. Ladetto M, Böttcher S, Kröger N, Pulsipher MA, Bader P. Methods and role of minimal residual disease after stem cell transplantation. Bone Marrow Transplant (2019) 54:681–90. doi: 10.1038/s41409-018-0307-1
68. Bader P, Salzmann E, Balduzzi A, Dalle J-H, Woolfrey A, Bar M, et al. More precisely defining risk peri-HCT in pediatric ALL: Pre- vs post MRD measures, serial positivity, and risk modeling. Blood Adv (2019) 3(21):3393–405. doi: 10.1182/bloodadvances.2019000449
69. Mo XD, Lv M, Huang XJ. Preventing relapse after haematopoietic stem cell transplantation for acute leukaemia: The role of post-transplantation minimal residual disease (MRD) monitoring and MRD-directed intervention. Br J Haematol (2017) 179:184–97. doi: 10.1111/bjh.14778
70. Balsat M, Renneville A, Thomas X, de Botton S, Caillot D, Marceau A, et al. Postinduction minimal residual disease predicts outcome and benefit from allogeneic stem cell transplantation in acute myeloid leukemia with NPM1 mutation: A study by the acute leukemia French association group. J Clin Oncol (2017) 35:185–93. doi: 10.1200/JCO.2016.67.1875
71. Shah NN, Borowitz MJ, Robey NC, Gamper CJ, Symons HJ, Loeb DM, et al. Feasibility of treating post-transplantation minimal residual disease in children with acute leukemia. Biol Blood Marrow Transplant (2014) 20:1000–7. doi: 10.1016/j.bbmt.2014.03.021
72. Zeijlemaker W, Kelder A, Oussoren-Brockhoff YJM, Scholten WJ, Snel AN, Veldhuizen D, et al. Peripheral blood minimal residual disease may replace bone marrow minimal residual disease as an immunophenotypic biomarker for impending relapse in acute myeloid leukemia. Leukemia (2016) 30:708–15. doi: 10.1038/leu.2015.255
73. Coustan-Smith E, Sancho J, Hancock ML, Razzouk BI, Ribeiro RC, Rivera GK, et al. Use of peripheral blood instead of bone marrow to monitor residual disease in children with acute lymphoblastic leukemia. Blood (2002) 100:2399–402. doi: 10.1182/blood-2002-04-1130
74. Brisco MJ, Sykes PJ, Hughes E, Dolman G, Neoh SH, Peng LM, et al. Monitoring minimal residual disease in peripheral blood in b-lineage acute lymphoblastic leukaemia. Br J Haematol (1997) 99:314–9. doi: 10.1046/j.1365-2141.1997.3723186.x
75. Aguirre-Ruiz P, Ariceta B, Viguria MC, Zudaire MT, Blasco-Iturri Z, Arnedo P, et al. Assessment of minimal residual disease by next generation sequencing in peripheral blood as a complementary tool for personalized transplant monitoring in myeloid neoplasms. J Clin Med (2020) 9:3818. doi: 10.3390/jcm9123818
76. Bader P, Stoll K, Huber S, Geiselhart A, Handgretinger R, Niemeyer C, et al. Characterization of lineage-specific chimaerism in patients with acute leukaemia and myelodysplastic syndrome after allogeneic stem cell transplantation before and after relapse. Br J Haematol (2000) 108:761–8. doi: 10.1046/j.1365-2141.2000.01927.x
77. Roux E, Abdi K, Speiser D, Helg C, Chapuis B, Jeannet M, et al. Characterization of mixed chimerism in patients with chronic myeloid leukemia transplanted with T-cell-depleted bone marrow: Involvement of different hematologic lineages before and after relapse. Blood (1993) 81:243–8. doi: 10.1182/blood.V81.1.243.243
78. Noviello M, Manfredi F, Ruggiero E, Perini T, Oliveira G, Cortesi F, et al. Bone marrow central memory and memory stem T-cell exhaustion in AML patients relapsing after HSCT. Nat Commun (2019) 10:1065. doi: 10.1038/s41467-019-08871-1
79. Dickinson AM, Norden J, Li S, Hromadnikova I, Schmid C, Schmetzer H, et al. Graft-versus-leukemia effect following hematopoietic stem cell transplantation for leukemia. Front Immunol (2017) 8:496. doi: 10.3389/fimmu.2017.00496
80. Zuber J, Sykes M. Mechanisms of mixed chimerism-based transplant tolerance. Trends Immunol (2017) 38:829–43. doi: 10.1016/j.it.2017.07.008
81. Bouvier A, Riou J, Thépot S, Sutra Del Galy A, François S, Schmidt A, et al. Quantitative chimerism in CD3-negative mononuclear cells predicts prognosis in acute myeloid leukemia patients after hematopoietic stem cell transplantation. Leukemia (2020) 34(5):1342–53. doi: 10.1038/s41375-019-0624-4
82. Horn B, Wahlstrom JT, Melton A, Liou A, Ouachee-Chardin M, Sunkersett G, et al. Early mixed chimerism-based preemptive immunotherapy in children undergoing allogeneic hematopoietic stem cell transplantation for acute leukemia. Pediatr Blood Cancer (2017) 64:e26464. doi: 10.1002/pbc.26464
83. Rettinger E, Merker M, Salzmann-Manrique E, Kreyenberg H, Krenn T, Dürken M, et al. Pre-emptive immunotherapy for clearance of molecular disease in childhood acute lymphoblastic leukemia after transplantation. Biol Blood Marrow Transplant (2017) 23:87–95. doi: 10.1016/j.bbmt.2016.10.006
84. Rujkijyanont P, Morris C, Kang G, Gan K, Hartford C, Triplett B, et al. Risk-adapted donor lymphocyte infusion based on chimerism and donor source in pediatric leukemia. Blood Cancer J (2013) 3:e137. doi: 10.1038/bcj.2013.39
85. Schlegel P, Jung G, Lang A-M, Döring M, Schulte JH, Ebinger M, et al. ADCC can improve graft vs leukemia effect after T- and b-cell depleted haploidentical stem cell transplantation in pediatric b-lineage ALL. Bone Marrow Transplant (2019) 54:689–93. doi: 10.1038/s41409-019-0606-1
86. Seidel UJE, Schlegel P, Grosse-Hovest L, Hofmann M, Aulwurm S, Pyz E, et al. Reduction of minimal residual disease in pediatric b-lineage acute lymphoblastic leukemia by an fc-optimized CD19 antibody. Mol Ther (2016) 24:1634–43. doi: 10.1038/mt.2016.141
87. Brudno JN, Somerville RPT, Shi V, Rose JJ, Halverson DC, Fowler DH, et al. Allogeneic T cells that express an anti-CD19 chimeric antigen receptor induce remissions of b-cell malignancies that progress after allogeneic hematopoietic stem-cell transplantation without causing graft-Versus-Host disease. J Clin Oncol (2016) 34:1112–21. doi: 10.1200/JCO.2015.64.5929
88. Pulsipher MA, Bader P, Klingebiel T, Cooper LJNN. Allogeneic transplantation for pediatric acute lymphoblastic leukemia: The emerging role of peritransplantation minimal residual disease/chimerism monitoring and novel chemotherapeutic, molecular, and immune approaches aimed at preventing relapse. Biol. Blood Marrow Transplant (2009) 15:62–71. doi: 10.1016/j.bbmt.2008.11.009
Keywords: chimerism, acute leukemia, allogeneic hematopoietic stem cell transplantation (AlloHCT), relapse, pediatric leukemia, real-time quantitative PCR
Citation: Haugaard AK, Madsen HO, Masmas TN, Vettenranta K, Buechner J, Mellgren K, Turkiewicz D, Rosthøj S, Marquart HV, Heilmann C, Müller KG and Ifversen M (2023) Highly-sensitive chimerism analysis in blood after allogeneic hematopoietic cell transplantation in childhood leukemia: Results from the Nordic Microchimerism Study. Front. Hematol. 2:1055484. doi: 10.3389/frhem.2023.1055484
Received: 27 September 2022; Accepted: 18 January 2023;
Published: 06 February 2023.
Edited by:
Paolo Strati, University of Texas MD Anderson Cancer Center, United StatesReviewed by:
Eva Rettinger, University Hospital Frankfurt, GermanyXiao-Dong Mo, Peking University People’s Hospital, China
Fabio Guolo, San Martino Hospital (IRCCS), Italy
Copyright © 2023 Haugaard, Madsen, Masmas, Vettenranta, Buechner, Mellgren, Turkiewicz, Rosthøj, Marquart, Heilmann, Müller and Ifversen. This is an open-access article distributed under the terms of the Creative Commons Attribution License (CC BY). The use, distribution or reproduction in other forums is permitted, provided the original author(s) and the copyright owner(s) are credited and that the original publication in this journal is cited, in accordance with accepted academic practice. No use, distribution or reproduction is permitted which does not comply with these terms.
*Correspondence: Marianne Ifversen, marianne.ifversen@regionh.dk