- 1Cell biology (CELL) Unit & Platform for Imaging Cells and Tissues (PICT) imaging Platform, de Duve Institute, UCLouvain, Brussels, Belgium
- 2Central Diagnostic Laboratory – Research, University Medical Center Utrecht, Utrecht University, Utrecht, Netherlands
- 3Department of Hematology, University Medical Center Utrecht, Utrecht University, Utrecht, Netherlands
- 4Louvain Institute of Biomolecular Science and Technology, UCLouvain, Louvain-la-Neuve, Belgium
- 5Department of Internal Medicine, Albert Schweitzer Hospital, Dordrecht, Netherlands
- 6Department of Hematology, VieCuri Medical Center, Venlo, Netherlands
- 7Bioanalysis and Pharmacology of Bioactive Lipids Research Group, Louvain Drug Research Institute, UCLouvain, Brussels, Belgium
Sitosterolemia is a metabolic disorder leading to excessive accumulation of phytosterols. Hemolytic stomatocytosis and macrothrombocytopenia are part of the clinical picture. However, the impact of phytosterols on red blood cell (RBC) deformability, membrane lipid composition and distribution and the efficiency of the reference treatment, Ezetimibe, are largely unknown. This study addresses these issues using RBCs from three patients with sitosterolemia and healthy RBCs exposed to β-sitosterol. Patients presented an increased proportion of stomatocytes, decreased RBC deformability and increased RBC hydration and osmotic fragility compared to healthy donors. At the membrane level, patient RBCs showed (i) very high content in β-sitosterols, (ii) increased proportions of saturated fatty acids and polyunsaturated fatty acid species with long and unsaturated carbon chains, and (iii) decreased content in phosphatidylethanolamine species. These lipid changes were accompanied by an almost complete abrogation of cholesterol-enriched domains, which could result from: (i) the reduced phosphatidylethanolamine content which positively correlated with domain abundance; and (ii) the fatty acid modifications and increased phytosterol content, both compatible with higher membrane stiffness. The role of β-sitosterol was supported by comparable changes in RBC morphology and cholesterol-enriched domains upon β-sitosterol integration at the healthy RBC membrane. Finally, Ezetimibe treatment combined with a sterol restricted diet lowered phytosterols and improved anemia and RBC deformability and hydration. However, this treatment had no or limited effect on RBC morphology and cholesterol-enriched domain abundance. This study reveals for the first time that phytosterols affect RBC membrane lipid composition and distribution but also RBC morphology, hydration, deformability and fragility.
Introduction
Sitosterolemia is an autosomal recessive disorder with a very heterogeneous character. Typically associated clinical features consist of hypercholesterolemia, xanthomas usually located at the eyelids but also at tendons of the hand, elbows and knees and a high risk of premature atherosclerosis and cardiac disease (1, 2). Sitosterolemia can also have hematological consequences by causing an abnormal structure and function of red blood cells (RBCs) and platelets, leading to cup-shaped or stomatocytic RBCs, hemolytic anemia, macrocytic thrombocytopenia, splenomegaly and bleeding tendency (1–4).
Sitosterolemia is characterized by the abnormal accumulation of plant sterols, especially sitosterols (1, 2). Plant sterols share high resemblance with cholesterol, an essential membrane lipid component in mammalian cells, but differ by the presence of an ethyl or methyl group, forming sitosterol and campesterol respectively, or a double bond, forming stigmasterol (5, 6). The absorption of sterols in the intestinal lumen is regulated through the sterol influx transporter, Nieman Pick C1 Like 1 (NPC1L1) which has a higher affinity for cholesterol than for plant sterols (2). This implicates that, in a diet equilibrated in both mammalian and vegetable sterols, around 50% of dietary cholesterol is absorbed but plant sterols to a 10-fold lesser extent (7, 8). The absorbed cholesterol is usually esterified in order to be transported by chylomicrons to the liver. In contrast, plant sterols are taken up by ABCG5 (sterolin-1) or ABCG8 (sterolin-2), two sterol efflux transporters that transport plant sterols back into the intestinal lumen. These two sterol efflux transporters are also found at the liver to pump the normally low proportion of sterols co-transported with cholesterol in chylomicrons into the bile (2, 8).
In sitosterolemia, mutations of the genes coding for ABCG5 and ABCG8 cause an abnormally high absorption of plant sterols in the intestinal lumen and decreased elimination of those compounds into the bile (2, 4), causing plasma plant sterol levels to increase 50 to 200-fold (9). As a consequence, plant sterols comprise 15% to 20% of total plasma sterols in patients with sitosterolemia (vs 0.2% in healthy subjects) and are predominantly found in low-density lipoproteins (LDLs) and very low-density lipoproteins (VLDLs) (9, 10). Moreover, cholesterol absorption is also increased in this disorder, usually but not systematically leading to mildly or moderately elevated plasma cholesterol levels (1, 11, 12).
Treatment of patients with sitosterolemia aims in the first place to reduce the plasma levels of both cholesterol and plant sterols by restricting the dietary intake (13). To this end, nutrients highly enriched in plant sterols, notably vegetable oils, wheat germs, nuts, seeds and avocados, should be avoided. The dietary restriction can be combined with a pharmacological approach. While statins are inefficient for treating this disease as the HMG CoA reductase (3-hydroxy-3-methyl-glutaryl-coenzyme A reductase) is already naturally inhibited, the binding to NPC1L1 of the sterol absorption inhibitor Ezetimibe has shown promising results in both adults and pediatric patients (2, 14, 15). Ezetimibe effectively reduces the amount of circulating plant sterols and reduces bleeding tendency, as it increases the number of platelets and restores their size (14). Cholestyramine, a bile acid sequestrant, can be administrated in combination with Ezetimibe.
While it is known that Ezetimibe has a beneficial effect on the platelet alterations (14), the impact of sitosterolemia on RBC functionality and membrane lipid composition and biophysical properties is largely unknown. We here addressed these issues on three patients with sitosterolemia. Patient 1 (P1) was followed before and during the treatment with Ezetimibe and a sterol restricted diet, whereas patients 2 and 3 (P2 & P3) were not treated. We combined a morphological and functional RBC study with a comprehensive lipidomic analysis and imaging of the distribution of cholesterol at the RBC surface. Finally, to see whether the RBC changes observed in sitosterolemia were at least partially dependent on the sitosterol membrane content, RBCs from healthy donors were exposed to high levels of β-sitosterol, one of the most abundant dietary Phytosterols (16).
Materials and methods
Blood collection and preparation
The study was approved by the Medical Ethics Committee of the UCLouvain and the University Medical Center Utrecht (Study 17-450/M). It included 3 patients named respectively patient 1 (P1), 2 (P2) and 3 (P3) based on their order of inclusion into the study. P1 is a 50 year-old man who had a long history of hemolytic anemia and thrombocytopenia, with the latter being previously misdiagnosed as immune thrombocytopenia (ITP). P2 and P3 are 29 and 31 year-old sisters. After informed consent, blood of patients and 9 age- and gender-matched healthy volunteers was collected by venipuncture into K+/EDTA-coated tubes at the University Medical Center Utrecht. Samples from P1 were collected at three time points: (i) before treatment, (ii) after 2 months on a low plant sterol diet and (iii) after 2 years on a sterol poor diet combined with Ezetimibe. Samples from P2 and P3 were collected twice during the study. Blood from healthy controls was obtained by means of the institutional blood donor service. Two additional healthy donors were directly included into the study at UCLouvain for evaluating the effect of β-sitosterol supplementation. Experiments were performed on RBCs separated from other blood components through 10-fold blood dilution in a glucose- and HEPES-containing medium (Dulbecco’s modified eagle medium [DMEM]). Diluted blood was centrifugated at 200g for 2min, the supernatant removed and RBCs suspended in medium. RBCs were washed a second time by centrifugation at 200g for 2min and resuspended in the medium, as in (17).
Routine laboratory measurements
Determinations were carried out at the local hospital and with the hematology analyzer (Cell-Dyn Sapphire, Abbott Diagnostics Division, Santa Clara, CA, USA) located at the University Medical Center Utrecht.
RBC morphology
Blood smears were made with May-Grunwald or Wright-Giemsa staining within 3 hours after blood venipuncture. Washed RBCs were also analyzed either by scanning electron microscopy after glutaraldehyde fixation or by optical microscopy in suspension in µ-dish IBIDI chambers at living state after 24-fold dilution, as in (17) and (18), respectively. The respective abundance of discocytes, stomatocytes and echinocytes/spherocytes was expressed as percentage of the total population.
Osmotic gradient ektacytometry
RBC deformability, expressed as Elongation Index (EI), was measured with the Laser Optical Rotational Red Cell Analyzer (Lorrca, RR Mechatronics, Zwaag, Netherlands) using the osmoscan module (19).
RBC fatty acid composition
Lipids and fatty acids (FAs) were extracted and FAs then measured by gas chromatography exactly as described in (17). All data were expressed in percentage of control (CTL) values.
RBC lipid composition
The cholesterol content was determined with the Amplex Red cholesterol assay kit after RBC lysis and normalized on the hemoglobin (Hb) content (17, 18). RBC membrane phospholipid, sphingolipid, lysophospholipid and β-sitosterol contents were analyzed by lipidomics through HPLC-mass spectrometry measurements (17, 18). Data were expressed in percentage of CTLs.
RBC membrane cholesterol imaging
Cholesterol was visualized on living RBCs spread on poly-L-lysine-coated coverslips by fluorescence microscopy using the mCherry-Theta toxin fragment as in (17, 20). Briefly, RBCs were incubated in suspension with 0.5-1.1µM Theta toxin fragment at 20°C for 20min, pelleted and resuspended in DMEM and immobilized onto poly-L-lysine-coated coverslips. After two rapid washings, coverslips were placed upside-down into Lab-Tek chambers for immediate examination of RBCs in the living state. The RBC spreading on poly-L-lysine coated coverslips is associated to a loss of RBC biconcavity without affecting their viability. Abundance of cholesterol-enriched domains was manually counted, normalized on the RBC hemi-area and expressed as percentage of CTLs.
Exposure of healthy RBCs to β-sitosterol
β-sitosterol solutions were prepared at 1-10µg/ml in DMEM supplemented with 1mg/ml bovine serum albumin. Washed RBCs were incubated with these solutions for 60-105min at 37°C, washed through a centrifugation step at 200g, resuspended in β-sitosterol-free medium and directly analyzed.
Data presentation and statistical analyses
Data are depicted as means ± SD. If several measurements for a specific parameter were performed on the same patient, the mean of all measurements was calculated. Statistical analyses included the comparison of (i) the global patient cohort to the CTL donors, if data for all 3 patients could be collected; (ii) patients individually to the respective CTL donors if at least 3 independent experiments were performed; and (iii) P1 before and under treatment. Statistics covered parametric (t-test with Welch’s correction) and non-parametric tests (Wilcoxon signed rank test, Kruskal-Wallis test with Dunn’s correction) and a paired t-test for the comparison of P1 before and under treatment. ns, not significant; *, p<0.05; **, p<0.01; ***, p<0.001; ****, p<0.0001. For correlations, data from all patients and if available data obtained on healthy RBCs supplemented with β-sitosterol were included and linear Pearson regressions plotted on the graphs.
Results
Mutations and clinical parameters of the three patients
Three patients with sitosterolemia (P1, P2 & P3) were included into the study. They were homozygous (P1) or compound heterozygous (P2 and P3) for pathogenic mutations in the gene encoding for ABCG8 (Table 1). Table 1 shows detailed hematological and clinical parameters, reflecting typical clinical hematological features of sitosterolemia, including hemolytic anemia and macrothrombocytopenia (Table 1). P1 presented a more pronounced hemolytic anemia and a more heterogeneous RBC population which was reflected by the higher percentages of hypochromic and hyperchromic cells. P1 also showed a higher macrothrombocytopenia and increased sitosterol and campesterol levels than the two other patients.
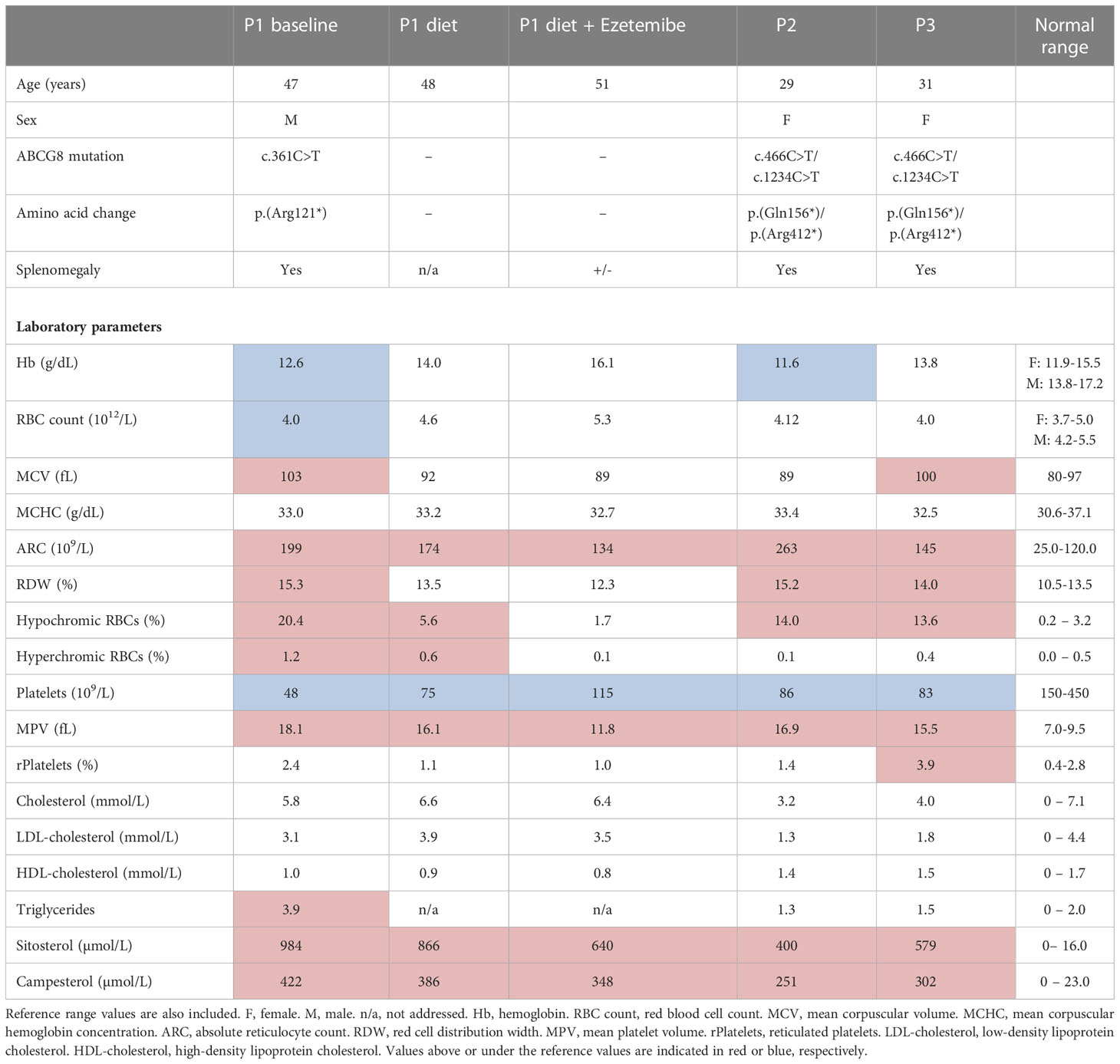
Table 1 Patient characteristics and laboratory parameters. Age, gender, genotypes, amino acid change, splenomegaly and laboratory parameters are shown for three (P1, P2 and P3) patients with mutations in the ABCG8 gene.
Patients exhibit a high proportion of stomatocytes and altered RBC deformability and hydration
In line with previous observations (4), the proportion of discocytes was reduced approximately 2 fold in patient samples whereas the number of stomatocytes was increased 5-8 fold as compared to healthy donors (Figures 1A–C, S1A, B). In contrast, the abundance of echinocytes and spherocytes was not significantly different from healthy donors (Figure 1D). Osmotic gradient ektacytometry revealed a slight decrease in RBC deformability, reflected by a lower Elmax (Figures 1E, G). This decrease was accompanied by a slight increase in Omin, indicating a decrease in the RBC surface-to-volume ratio and an increase in osmotic fragility, and a slight increase in Ohyper, reflecting an increase in RBC hydration (Figures 1F, H). These alterations were the most pronounced for P1, who, as a result, also showed a reduced AUC (Figure 1I).
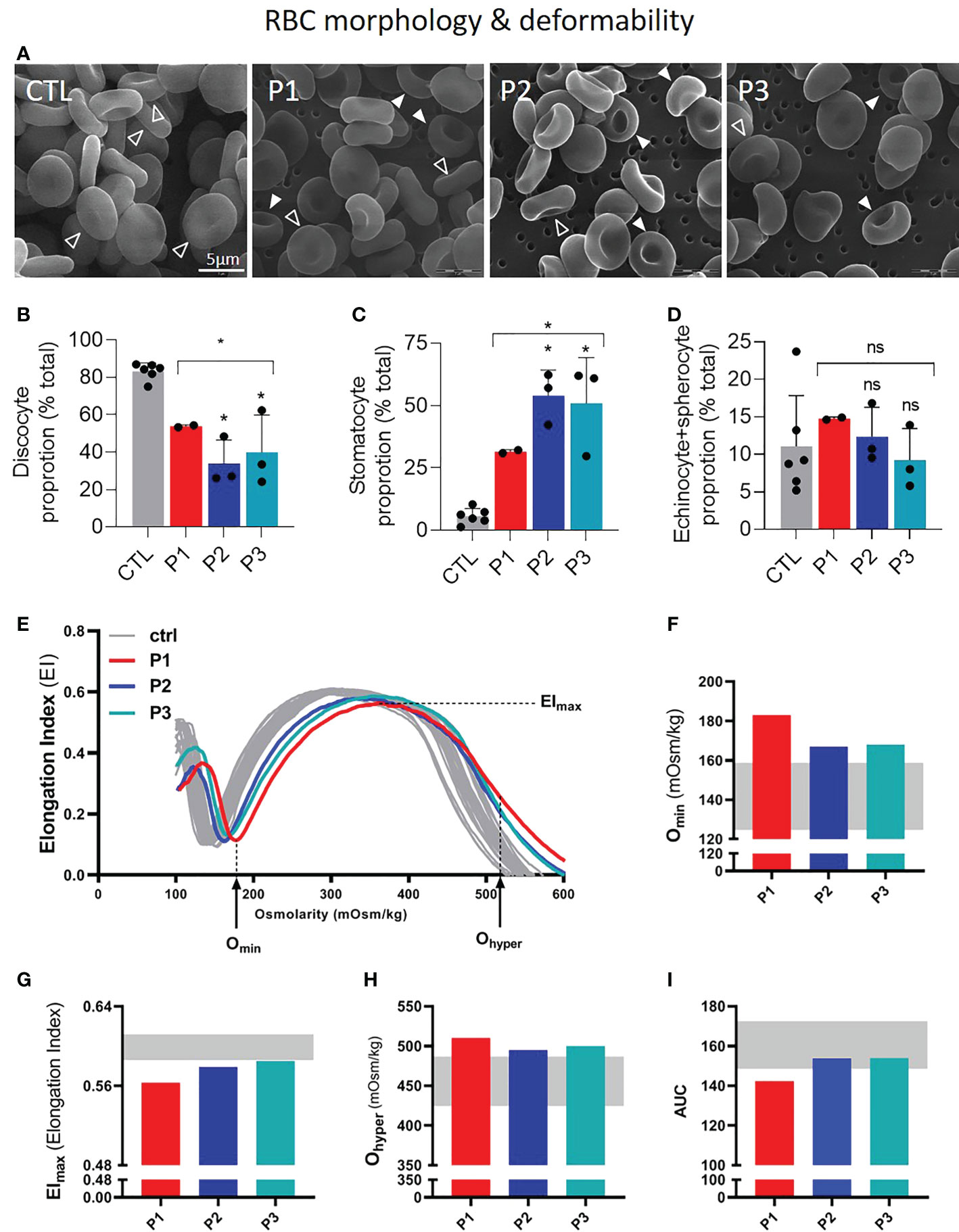
Figure 1 Patients with sitosterolemia show a high proportion of stomatocytes and increased RBC fragility and hydration but decreased deformability. RBCs from patients (P1,2,3; red, dark and light blue columns/curves, respectively) were compared to RBCs from healthy donors (CTL; grey columns/curves/ranges) for morphology (A–D) and deformability by ektacytometry (E–I). Statistical analyses for the global patient cohort are indicated above a bracket including the 3 patient columns and individual statistical analysis above the column of the concerned patient, respectively. (A–D) Scanning electron microscopy of glutaraldehyde-fixed RBCs on filters. (A) Representative images. Open arrowheads, discocytes; filled arrowheads, stomatocytes. (B–D) Quantification of the relative abundance of discocytes (B), stomatocytes (C) and echinocytes and spherocytes (D) expressed as % of the global RBC population. Data are means ± SD of 2-3 independent experiments; unpaired t-tests with Welch’s correction. (E–I) Osmotic gradient ektacytometry curves and derived parameters, i.e. Omin, Elmax, Ohyper (which reflect surface area-to-volume ratio, membrane surface area and cellular hydration, respectively) and AUC (Area Under the Curve, which is a direct reflection of these three parameters). ns, not significant; *p < 0.05.
Patient RBCs show strong increases in β-sitosterol content
To determine if the RBC morphological and functional alterations could be induced by modifications of the RBC lipid composition, we performed a comprehensive lipid analysis. We started with the determination of β-sitosterol levels, most likely to be altered because of their increase in the plasma (4, 9). The increase of β-sitosterol was 100-200 fold in the three patient samples as compared to healthy donors but 2-fold higher in P1 than in the two other patients (Figure 2A). Notably, even if the increase of this phytosterol expressed as percentage of healthy RBCs appeared extreme, the total concentration should be limited as, in contrast to the β-sitosterol levels, the global sterol levels did not seem to be impacted in patient samples (Figure 2B).
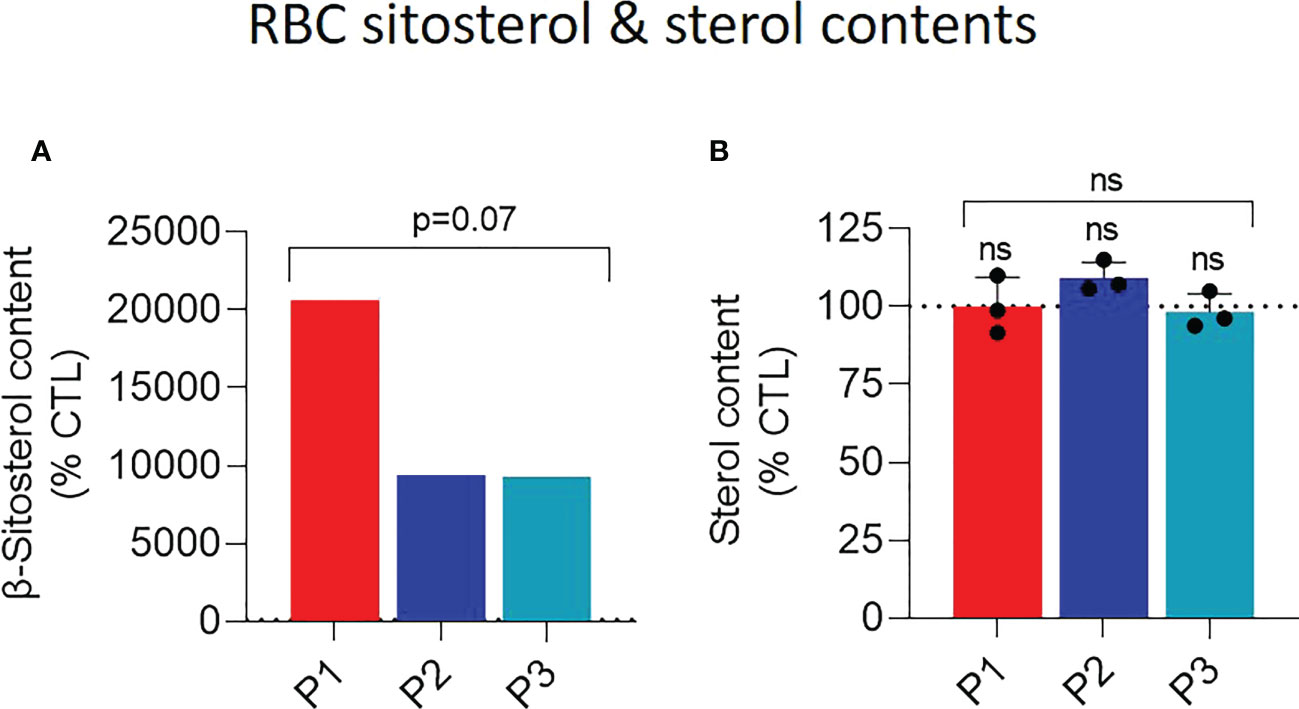
Figure 2 Patient RBCs exhibit heightened abundance of membrane β-sitosterol. RBCs from patients (P1,2,3; red, dark and light blue columns, respectively) and from healthy donors (CTL; dotted black line) were analyzed for their β-sitosterol (A) and sterol (B) contents. Statistical analyses for the global patient cohort are indicated above a bracket including the 3 patient columns and individual statistical analyses above the column of the concerned patient, respectively. (A) RBC β-sitosterol content. Lysed RBCs were submitted to lipid extraction and then assessed for the β-sitosterol content by HPLC-mass spectrometry. Data are expressed as % of CTL RBCs; unpaired t-test with Welch’s correction. (B) RBC sterol content. Lysed RBCs were assessed for their sterol content with a fluorescent assay kit based on a series of enzymatic reactions ending with the production of the fluorescent resorufin. As it cannot be excluded that the kit also detects phytosterols because of the high resemblance between sterol species, we refer to sterol and not cholesterol content. The sterol content was normalized on the hemoglobin (Hb) content and expressed as % of the CTL RBCs. Data are means ± SD of 3 independent experiments; Kruskal-Wallis test with Dunn’s correction.
Patient RBCs exhibit increased proportion of saturated fatty acids and decreased mono- and poly-unsaturated phosphatidylethanolamine species
As the extent of morphological alterations was quite similar despite a 2-fold difference in β-sitosterol levels in the three patient samples, we then wondered whether other membrane lipids could also be altered. We first evaluated the RBC fatty acid (FA) composition since FAs have been reported to impact RBC morphology (21). A slight increase of the proportion of saturated FAs (SFAs) at the expense of monounsaturated (MUFAs) and especially polyunsaturated (PUFAs) was observed in the three patient samples (Figure S2). Further investigation of the FA subgroups showed differential alterations of the ratios of two major SFAs (palmitic acid (C16:0) and stearic acid (C18:0)) and of the two C18:1 MUFAs (oleic acid (cis9) and cis-vaccenic acid (cis11)) in the three patients that were however not significantly different from healthy donor values (Figures S3A–D). In contrast, the ratios of PUFAs with a C18 and C22 chain length and with 2 and 6 unsaturation were all lower than healthy controls, except for the former in P2 (Figures S3E–H).
We next evaluated if and how those FA alterations could specifically modify some sphingolipid and phospholipid species. At the outer plasma membrane leaflet, sphingomyelin (SM) species were only slightly and similarly decreased in the three patient samples (Figure 3A). In contrast, phosphatidylcholine (PC) species were clearly increased for P1 but not for the two sisters (Figures 3B, C, E) while lysoPCs (hydrolyzed forms of PCs) were increased in all patient samples (Figure 3D), leading to reduced lysoPC/PC ratios for P1 and heightened ratios for the two sisters (Figure 3F). At the inner plasma membrane leaflet, mono- and poly-unsaturated phosphatidylethanolamine (PE) species were lowered in all patient samples (Figures 4A, B, D) whereas lysoPE species were not strongly modified (Figure 4C), leading to increased ratios of mono- and poly-unsaturated lysoPE/PE species (Figure 4E).
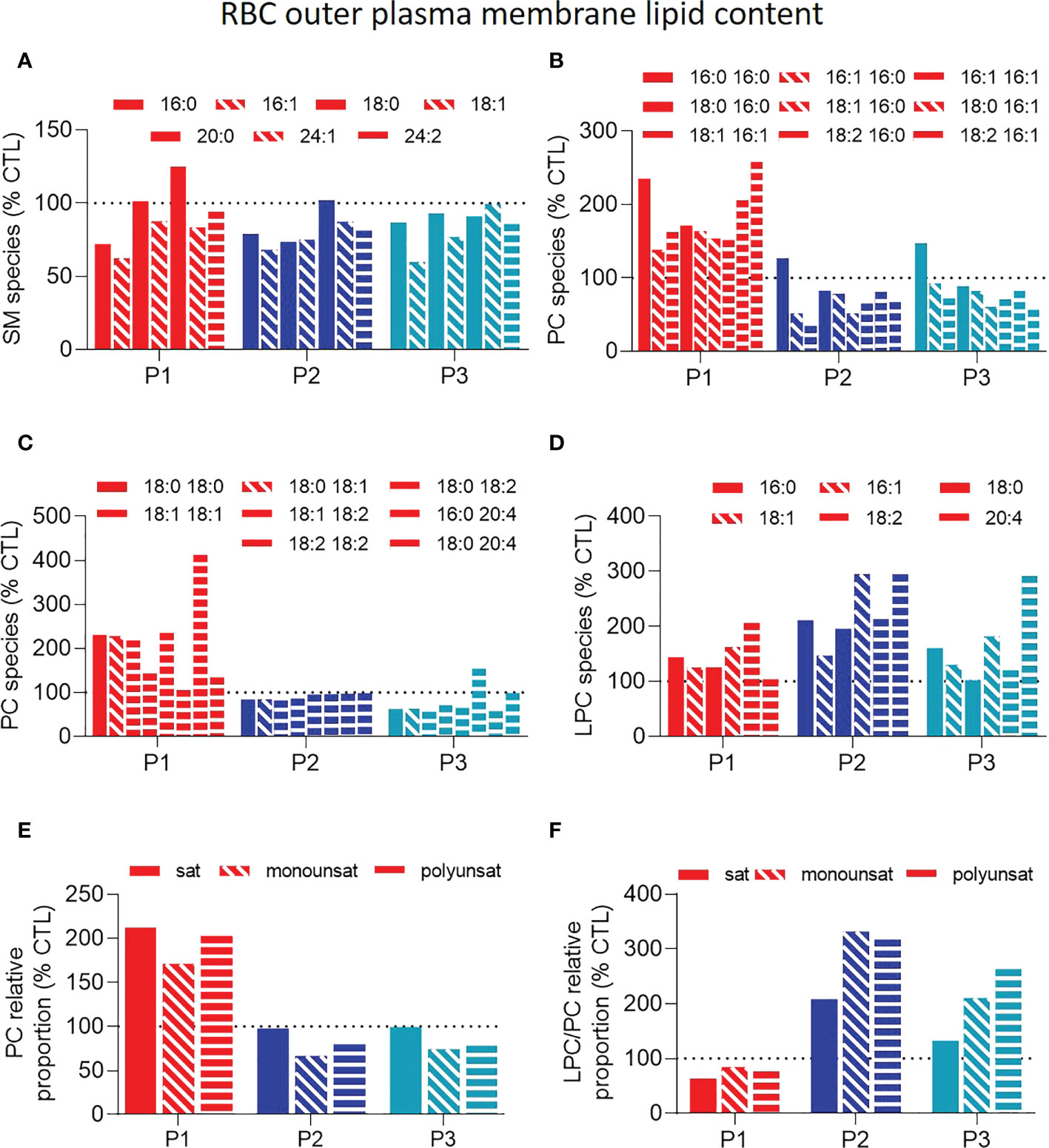
Figure 3 Patient RBCs exhibit similar slightly lower sphingomyelin levels but differential phosphatidylcholine and lysophosphatidylcholine-to-phosphatidylcholine ratios. RBCs from patients (P1,2,3; red, dark and light blue filled columns, respectively) and from healthy donors (CTL; dotted black line) were lysed, lipid extracted and analyzed as in Figure 2A by HPLC-mass spectrometry for composition in sphingomyelin (SM; A) and (lyso)phosphatidylcholine (LPC and PC; B–F) species. (A) SM species classified according to the carbon chain length and the number/position of the double bounds. (B-D) PC (B, C) and LPC (D) species classified according to the carbon chain length and the number/position of the double bounds. (E) Relative abundance of saturated (sat), monounsaturated (monounsat) and polyunsaturated (polyunsat) PC species. (F) Ratios between the relative abundance of sat, monounsat and polyunsat LPC to PC species. All data were expressed as % of the CTL RBCs. Filled, hatched and striped columns represent respectively sat, monounsat and polyunsat lipid species.
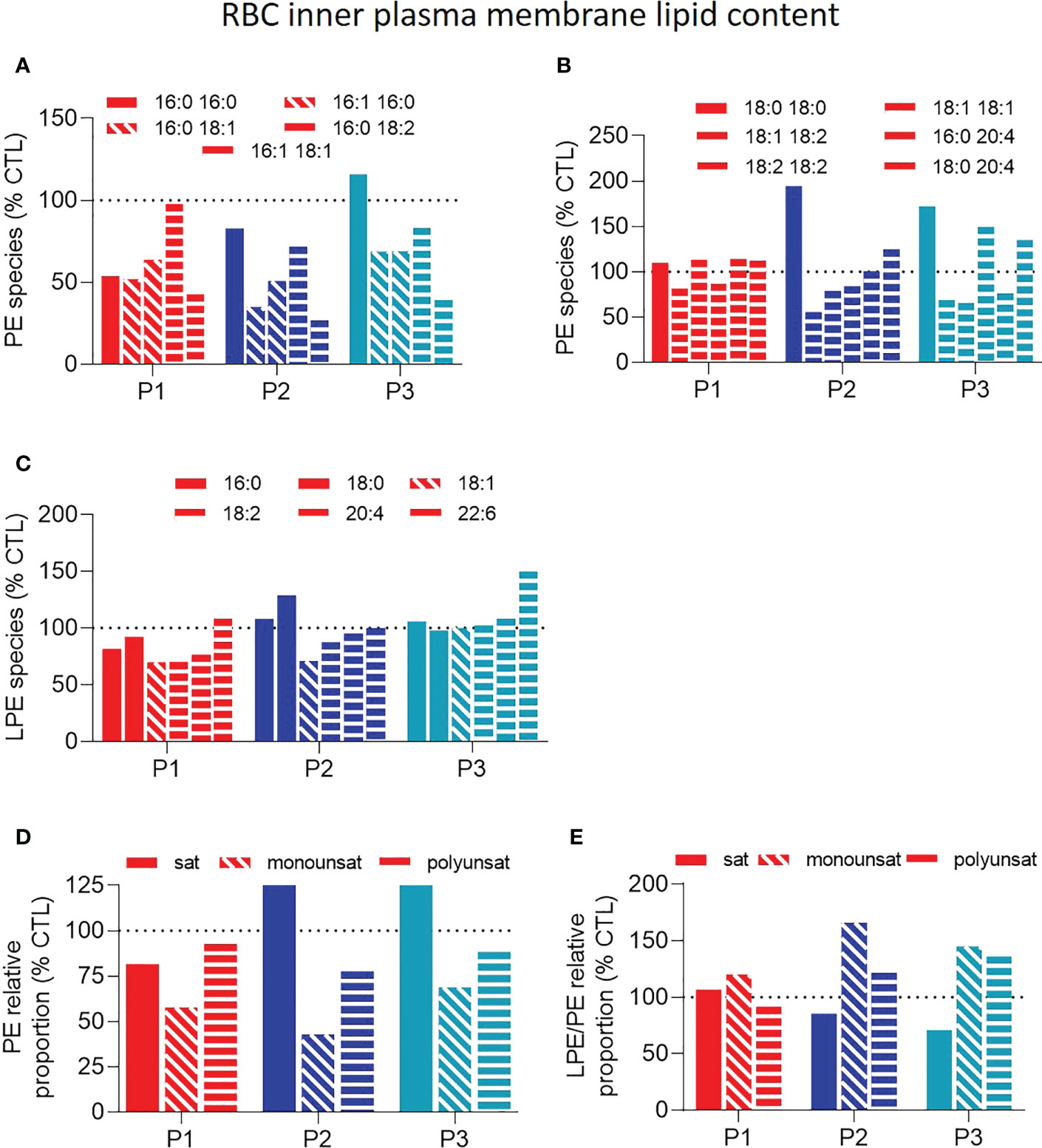
Figure 4 Patient RBCs show decreased unsaturated phosphatidylethanolamine species and increased unsaturated lysophosphatidylethanolamine-to-phosphatidylethanolamine ratios. RBCs from patients (P1,2,3; red, dark and light blue filled columns, respectively) and healthy donors (CTL; dotted black line) were lysed, lipid extracted and analyzed as in Figure 2A, by HPLC-mass spectrometry for composition in (lyso)phosphatidylethanolamine (LPE and PE) species. (A–C) PE and LPE species classified according to the carbon chain length and the number/position of the double bounds. (D) Relative abundance of saturated (sat), monounsaturated (monounsat) and polyunsaturated (polyunsat) PE species. (E) Ratios between the relative abundance of sat, monounsat and polyunsat LPE to PE species. All data were expressed as % of the CTL RBCs. Filled, hatched and striped columns represent respectively sat, monounsat and polyunsat lipid species.
Altogether, the three patients showed (i) higher proportion of SFAs and PUFAs with long carbon chains and a high number of unsaturation, which is compatible with altered membrane stiffness; and (ii) decreased proportion of mono- and poly-unsaturated PE species together with increased unsaturated lyso-to-phospholipid ratios, which are compatible with changes in membrane curvature. Alterations of both membrane stiffness and curvature could explain the observed changes in RBC morphology and deformability.
Patient RBCs present a strong decrease of cholesterol-enriched domains
We next wondered if, and to what extent, lipid content modifications might impact their lateral distribution in submicrometric domains implicated in RBC deformation (20, 22). This analysis was performed on RBCs labeled with a fluorescent Theta toxin fragment specific to endogenous cholesterol, then spread on poly-L-lysine-coated coverslips and directly analyzed by fluorescence vital imaging (23). Despite the loss of RBC biconcavity, this approach allows to evidence similar cholesterol-enriched domains as in RBCs in suspension but in a more reproducible and quantitative way (23). Data revealed that the abundance of cholesterol-enriched domains was heavily reduced in sitosterolemia patients (Figure 5B). This decrease was particularly visible at the RBC periphery, corresponding to RBC high curvature areas (Figure 5A, orange arrowheads).
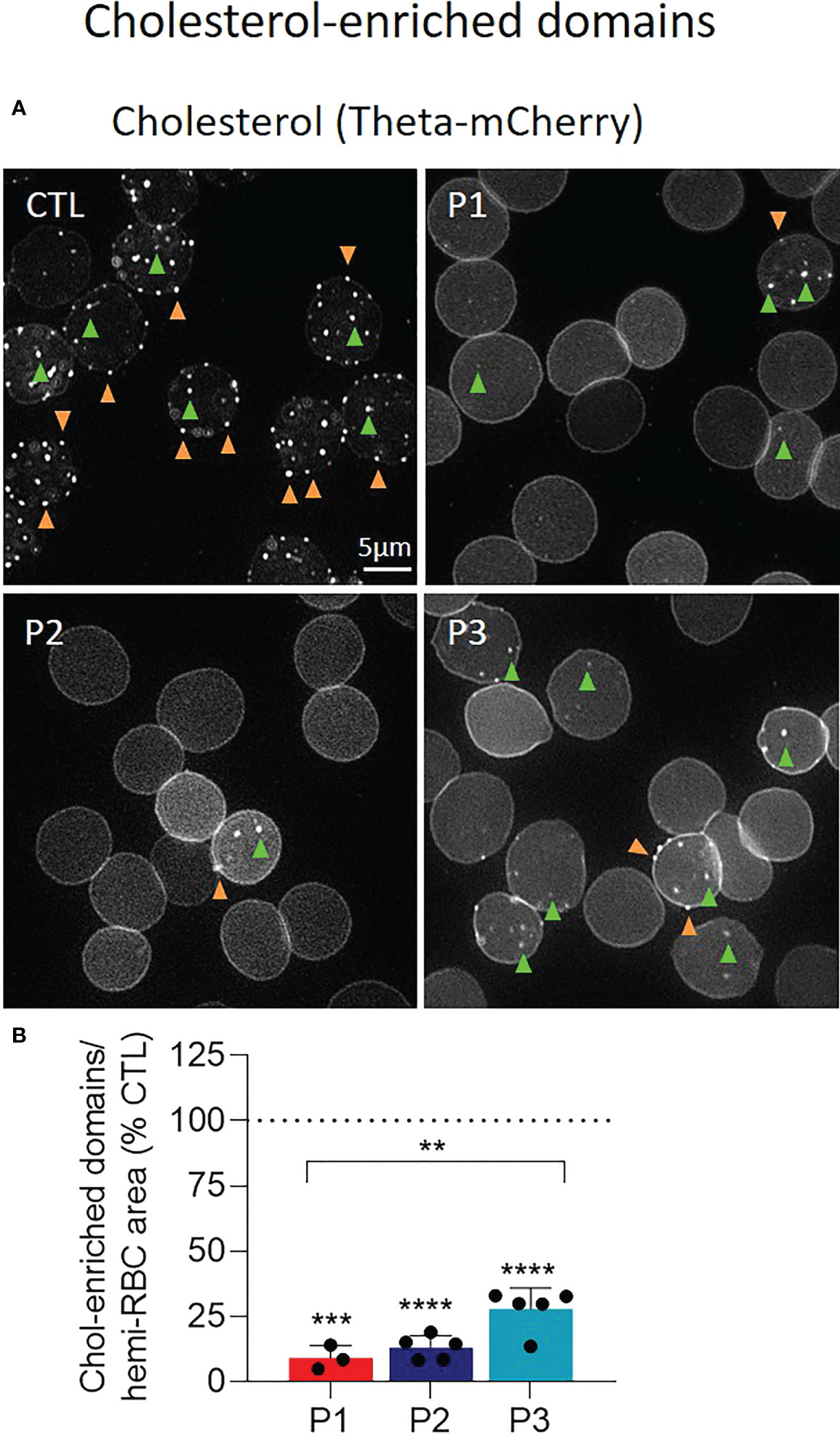
Figure 5 The abundance of cholesterol-enriched domains is dramatically reduced at the surface of patient RBCs. RBCs from patients (P1,2,3; red, dark and light blue filled columns, respectively) and healthy donors (CTL; dotted black line) were labelled with a fluorescent Theta toxin fragment specific to endogenous cholesterol (chol), immobilized on poly-L-lysine (PLL)-coated coverslips and directly observed by vital fluorescence microscopy. (A) Representative images. Green and orange arrowheads, domains in RBC center (low curvature area) and periphery (high curvature area), respectively. (B) Quantification of total chol-enriched domain abundance determined by manual counting, normalized to the RBC hemi-area and expressed as % of CTL RBCs. Data are means ± SD of 3-5 independent experiments and statistical analysis for the global patient cohort are indicated above a bracket including the 3 patient columns and individual statistical analysis above the column of the concerned patient, respectively. Unpaired t-tests with Welch’s correction. **p < 0.01; ***p < 0.001; ****p< 0.0001.
Sterol-low diet combined with Ezetimibe treatment decreases RBC sterol content and improves RBC membrane surface, surface area-to-volume ratio and hydration but only very slightly cholesterol-enriched domain abundance
In order to evaluate the effect of therapy on RBC parameters, we analyzed a blood sample of P1 on low sterol diet and with or without complementary Ezetimibe treatment. The diet alone already improved clinical and hematological parameters, which were restored further by Ezetimibe treatment (Table 1). The combination of diet and Ezetimibe improved the hemolytic anemia (Table 1) and a reduction of spleen size (14.5cm to 13.0cm) was observed (data not shown). The decreased reticulocyte counts and increased hemoglobin levels (Table 1) were surprisingly not accompanied by a beneficial effect on RBC morphology (Figures 6A, B, S1C). Nevertheless, osmotic gradient ektacytometry showed that the EImax, Omin and Ohyper, but not AUC, changed towards normal, indicating an improvement in RBC membrane surface, surface area-to-volume ratio and hydration and subsequently deformability (Figures 6C–G). The decrease in plasma phytosterol levels (Table 1) was accompanied by a slight decrease of RBC sterols and the partial restoration of cholesterol-enriched domain abundance even if it was still reduced by 80% compared to their number detected in healthy donors (vs 90% reduction before treatment; Figures 6H, I). Thus, the applied treatment, despite low beneficial effects on RBC morphology and membrane lipid distribution, showed promising effects on RBC deformability parameters.
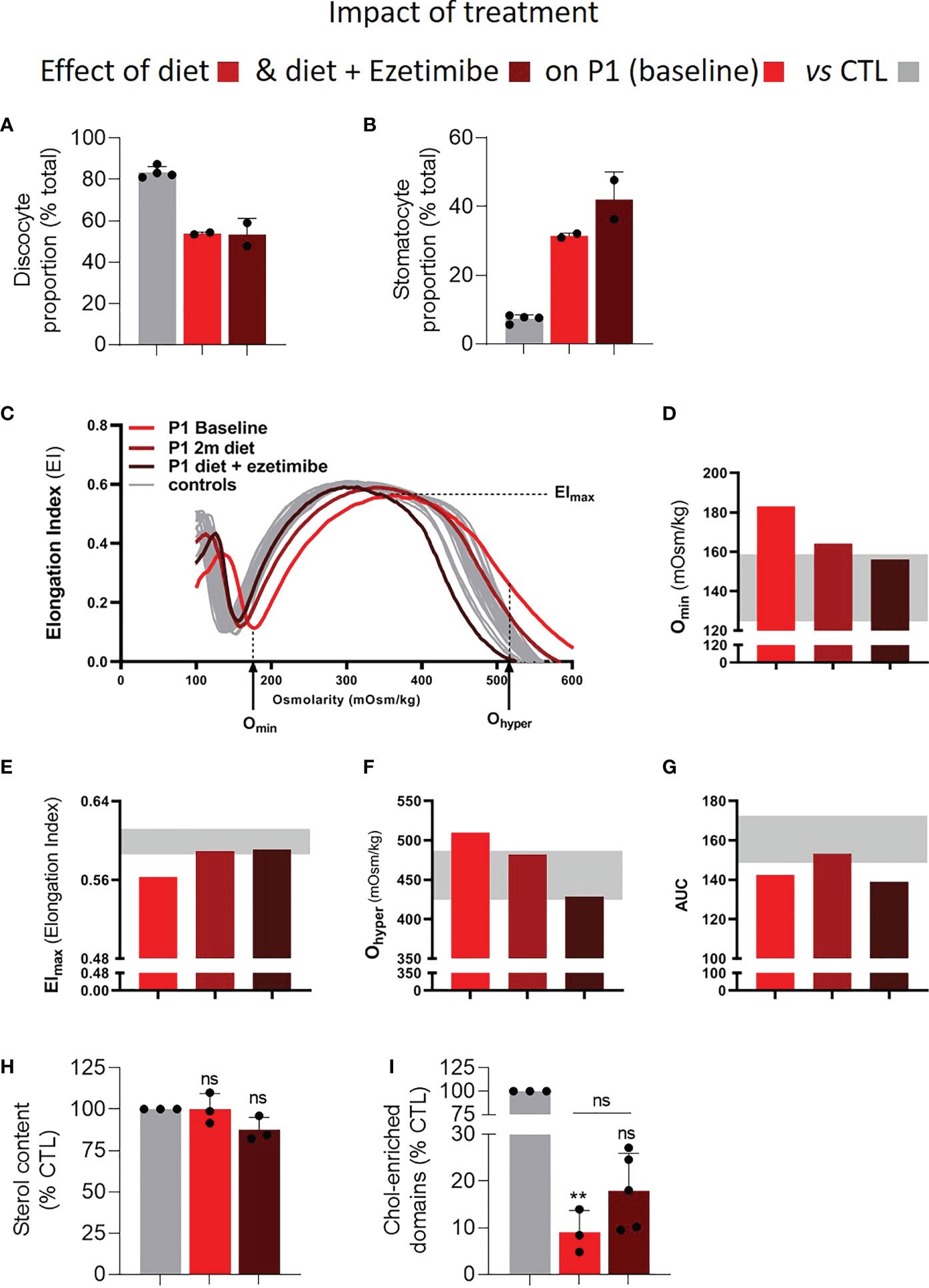
Figure 6 Treatment of P1 with a sterol-poor diet and Ezetimibe largely restores RBC osmotic fragility, area and hydration but not RBC morphology and only slightly improves cholesterol-enriched domain abundance. RBCs from P1 under treatment (diet alone in medium red and diet + Ezetimibe in dark red) or not (light red) were compared to RBCs from healthy control donors (CTL; grey) for morphology (A, B), osmotic gradient ektacytometry (C–G), sterol content (H) and abundance of chol-enriched domains (I). Statistical analyses to compare the patient before and after treatment are indicated above a horizontal black line situated above the patient columns and statistical analysis comparing the patient to the CTL directly above the patient columns. (A, B) RBC morphology determined either by electron microscopy on fixed RBCs as in Figures 1A–C or by light microscopy on living RBCs in IBIDI µ-dish chambers. Data are means ± SD of 2 independent experiments. (C–G) Osmotic gradient ektacytometry curves and derived Omin, Elmax, Ohyper and AUC parameters. For interpretation, see Figures 1E–I. (H) RBC sterol content determined as in Figure 2. Data are means ± SD of 3 independent experiments; Wilcoxon signed rank test. (I) Abundance of chol-enriched domains assessed as in Figure 5. Data are means ± SD of 3-5 independent experiments; Kruskal-Wallis test with Dunn’s correction to compare patient samples to control samples and paired t-test to compare the patient before and after treatment. ns, not significant; **p < 0.01.
RBC supplementation with increasing concentrations of β-sitosterol induces dose-dependent RBC transformation into stomatocytes and cholesterol-enriched domain decline
Intrigued by the observation that stomatocytes were still highly abundant and cholesterol-enriched domains still drastically reduced in P1 upon low sterol dietary input and pharmacological intervention, we determined to which extent the phytosterols were actually responsible for those alterations. For this, RBCs from healthy control donors were incubated with increasing amounts of β-sitosterol. We observed a transformation of discocytes into stomatocytes accompanied by a decrease of cholesterol-enriched domain abundance especially in the high-curvature areas. This effect was dose-dependent at concentrations up to 5µg/ml and was followed by a saturation stage (Figures 7A–D). The abundance of cholesterol-enriched domains positively correlated with the number of discocytes and negatively with the number of stomatocytes (Figures 7E, F).
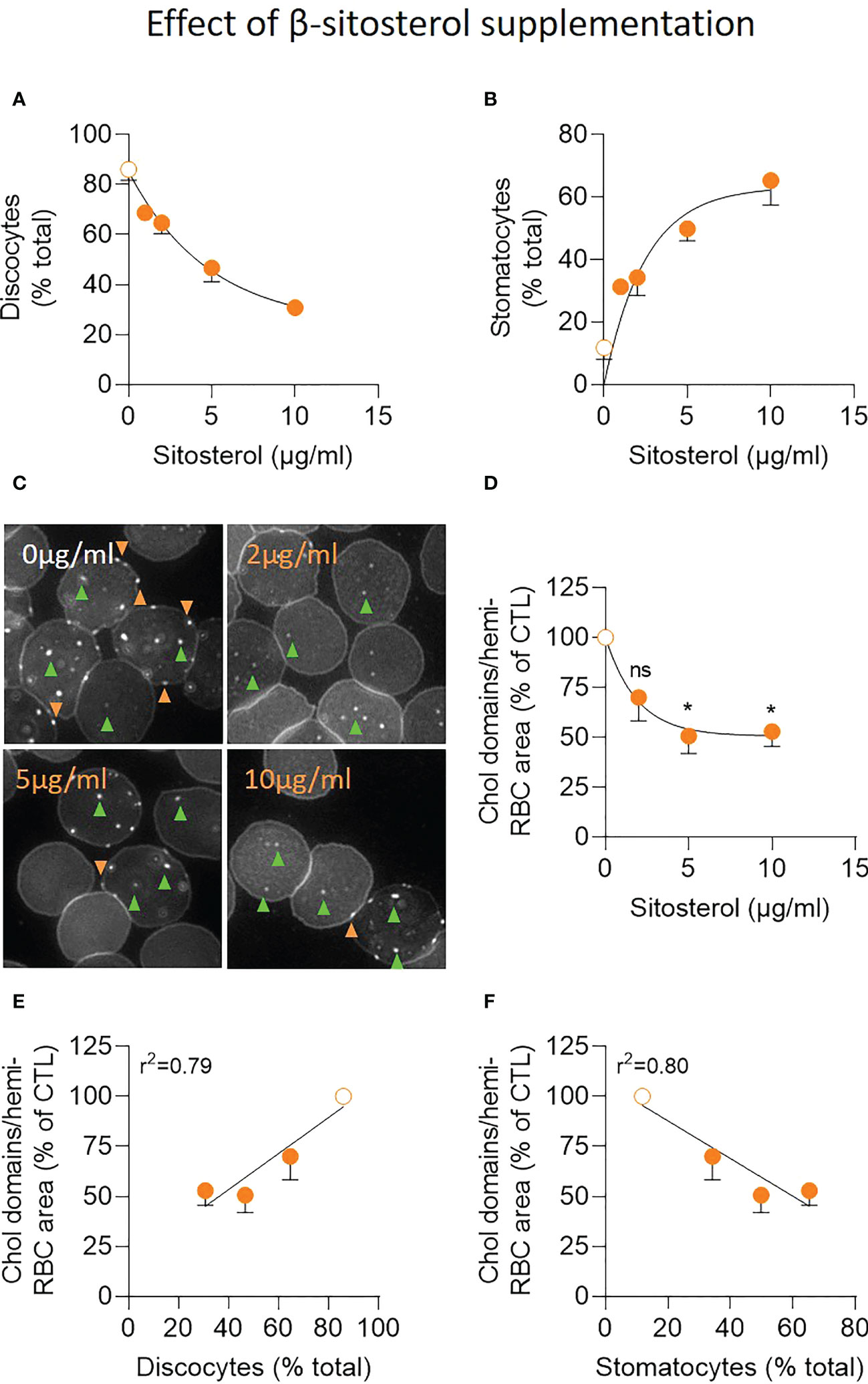
Figure 7 Exposure of healthy RBCs to increasing concentrations of β-sitosterol leads to progressive accumulation of stomatocytes and reduction of cholesterol-enriched domain abundance especially in high curvature areas. RBCs from healthy donors were incubated with increasing concentrations of β-sitosterol (filled orange dots) or not (open orange dots), washed and analyzed for morphology (A, B) and cholesterol (chol)-enriched domain abundance (C, D). (A, B) Morphology of living RBCs assessed as in Figures 6A, B. Data are means ± SD of 2-3 independent experiments. (C, D) Abundance of chol-enriched domains evaluated as in Figure 5. Green and orange arrowheads, domains in RBC center (low curvature areas) and periphery (high curvature areas), respectively. In (C), representative images; in (D), quantification. Data are means ± SD of 3-4 independent experiments; Kruskal-Wallis test with Dunn’s correction to compare treated to untreated RBCs. (E, F) Correlation between the abundance of chol-enriched domains (from D) and the relative proportion of discocytes (from panel 7A) or stomatocytes (from B). ns, not significant; *p < 0.05.
Discussion
We here report a detailed analysis of RBC properties for three cases of hereditary sitosterolemia due to mutations in the ABCG8 gene. We showed that the high proportion of stomatocytes was accompanied by decreased RBC deformability, increased RBC hydration and raised osmotic fragility. By comprehensive lipidomic analysis and live cell imaging, we then revealed profound membrane alterations including extremely high β-sitosterol levels, increased FA saturation, decreased PE content and nearly complete abrogation of cholesterol-enriched domains. The role of sitosterols in these RBC alterations was confirmed by similar results obtained from healthy RBCs exposed to high levels of β-sitosterol. Finally, treating the patient P1 with an adapted diet and Ezetimibe reduced splenomegaly, improved hemolytic anemia, restored RBC deformability and hydration and slightly improved the abundance of cholesterol-enriched domains without, however, normalization of morphological alterations.
RBC morphological and deformability characteristics in sitosterolemia
In agreement with (2, 24–26), the three patients showed a relatively high proportion of stomatocytes, accounting for 30-55% of all RBCs. Su et al. proposed a direct relationship between the plasma phytosterol levels and the RBC morphological alterations, probably resulting from the integration of specific phytosterols in the RBC plasma membrane (27). Accordingly, we show here that exposing RBCs of healthy donors to increasing concentrations of β-sitosterol indeed resulted in a concentration-dependent increase in stomatocytes. However, despite the fact that β-sitosterol levels were 2-fold higher in the RBC membrane of P1 than P2 or 3, the proportion of stomatocytes was lower for P1 than P2 and 3. This might be explained by the integration of phytosterols other than the measured β-sitosterol into the RBC membrane. Alternatively, this could be related to the complex alteration of membrane FA and sphingo-/phospho-lipid composition. This second possibility appeared more likely as the proportion of stomatocytes was not reduced under treatment despite reduced plasma sitosterol levels. All untreated patients showed increased osmotic fragility, as indicated by the increase in Omin. This is in agreement with literature data obtained on RBCs from 13 patients affected by sitosterolemia and on healthy RBCs incubated with sitosterols (1, 4). In addition, the three patients exhibited a slight overhydration likely responsible for the altered RBC fragility and reminiscent to the very high osmotic fragility in overhydrated hereditary stomatocytosis (28).
Lipid content alterations in sitosterolemia
The genetic defects leading to sitosterolemia unavoidably change plasma phytosterol levels. Based on the up to 200-fold plasma phytosterol increases reported (2), the RBC sterol composition might also be affected. We indeed observed a 100 and 200-fold increase of membrane β-sitosterol respectively for P2/P3 and P1 RBCs compared to healthy RBCs, corresponding to 4.1 and 8.2mg of β-sitosterol/dl of blood. Based on the ~5mg of sitosterols/dl and the 8mg of total plant sterols/dl of blood measured by Othman et al. in the RBC membrane of a sitosterolemia patient, we suggest that sitosterols, especially β-sitosterol, could be the major, if not the only, type of plant sterols present in the membrane of P1. The composition in plant lipids might be more complex for P2 and P3. Although this hypothesis needs to be tested, it could explain why all patients showed the same extent of RBC morphological alteration despite differences in β-sitosterol content. In support of this hypothesis, we show that increased levels of β-sitosterol in healthy RBCs resulted in increased amounts of stomatocytes, but that a plateau seemed to be established at 5µg/ml. In contrast to β-sitosterol, the content of global sterols, notably cholesterol, did not appear to be affected in the patient RBCs. This is in agreement with the normal cholesterol plasma levels of sitosterolemia patients found here and previously (1).
Numerous FA alterations were also observed but mainly in a patient-dependent manner, suggesting that they might rather result from specific FA dietary intake than from the underlying genetic alterations (29). Only the limited increase in global SFAs and the decreased ratio of PUFAs with 2 to 6 unsaturation were common to all the patients. The latter might be explained by the protective effect of phytosterols against lipid peroxidation (30) but the origin of the higher proportion of SFAs remained unclear. Likewise, changes in PC amounts were also different in P1 vs the two sisters but in agreement with PC changes observed in RBCs from patients with hereditary stomatocytosis (31). In contrast, all patients had reduced unsaturated PE species contents at the inner plasma membrane leaflet, which very strongly and inversely correlated with the proportion of stomatocytes (Figures S4A, B). Moreover, P2 and P3 showed a specific increase of lysoPE-to-PE ratio. Those PE and lysoPE changes suggested the impact of inner plasma membrane lipids on RBC morphology, in agreement with the fact that inverted cone shape lysophospholipids cause a natural curvature to the membrane (32).
Lipid distribution alterations in sitosterolemia
In addition to the alteration of RBC membrane lipid composition, their distribution in domains was also strongly affected in all patients, as revealed by the almost complete abrogation of cholesterol-enriched domains. This could result from cholesterol restricted lateral mobility at the outer plasma membrane leaflet due to higher membrane stiffness, as expected from the high amount of phytosterols and saturated FAs. Although this remains to be demonstrated, the impact of β-sitosterol on the abundance of cholesterol-enriched domains was shown by exposing healthy RBCs to this phytosterol. Additionally, cholesterol-enriched domain abundance and monounsaturated PE species content strongly and positively correlated (Figure S4C), leading us to propose that PE could represent inner membrane lipid partners to the cholesterol-enriched domains revealed at the outer leaflet. Consequently, its perturbation in sitosterolemia might further affect the assembly of cholesterol-enriched domains. Although we quantified the whole population of cholesterol-enriched domains, careful image examination revealed that both the high- (RBC periphery) and low- (RBC center) curvature associated domains were affected, especially at high sitosterol contents, with three potential consequences. First, independently of their location, reduced cholesterol-enriched domains might be accompanied by a decreased membrane association of the cholesterol binding protein stomatin (33) and by impaired RBC morphology, hydration and fragility, as in some forms of overhydrated stomatocytosis characterized by reduced stomatin membrane content (34). This hypothesis is supported by the strong negative correlations between cholesterol-enriched domain abundance and stomatocyte proportion or Omin levels (Figures S4D, E). Second, affecting the cholesterol-enriched domains in RBC high curvature areas might decrease RBC deformability because high curvature areas are less stabilized during deformation (22), likely explaining why sitosterolemia patients show signs of increased osmotic fragility, hemolysis and sometimes splenomegaly. This hypothesis is supported by the positive correlation between cholesterol-enriched domains and Elmax (Figure S4F). Third, the modification of domains in low-curvature areas proposed to be implicated in calcium exchanges (20) could alter the RBC intracellular calcium content and potentially lead to altered hydration. However, this is expected to lead to dehydration rather than overhydration (34).
Ezetimibe and RBCs in sitosterolemia
As low sterol diet is usually insufficient to reduce the plasmatic phytosterol levels to normal values, Ezetimibe treatment is the main therapy to treat sitosterolemia (2, 24). The latter could even be considered as the gold standard in the treatment of sitosterolemia (24) since perfect control of the plant sterol levels is nearly unachievable (2). Its beneficial effect for the reduction of phytosterols in the plasma has been extensively shown (14, 15, 35) as in our study. In contrast, the effect of Ezetimibe on RBCs of sitosterolemia patients is largely unexplored. In P1, the combination of a sterol poor diet and Ezetimibe led to nearly normal clinical hematological parameters, RBC deformability and hydration whereas cholesterol-enriched domains were only slightly improved and the altered RBC morphology was not. The improvements could be related to reduced phytosterol levels, reaching usually a 30% decrease after 14 weeks of treatment (14). A ~20% reduction of free RBC membrane cholesterol was also described upon Ezetimibe treatment (14) which is in total agreement with our study. This reduced cholesterol content might in turn explain the limited restoration of cholesterol-enriched domain abundance.
Conclusion
Sitosterolemia is associated with important hematological alterations which represent in some cases the only detectable clinical symptoms (2). Subsequently, the presence of stomatocytes and macrothrombocytopenia could assist in avoiding misdiagnosis of sitosterolemia with clinically similar diseases as hypercholesterolemia. Despite the relevance of hematological alterations for this disease, the actual impact on RBCs as well as the efficiency of Ezetimibe to treat eventual RBC alterations are largely overlooked. In this study, we report for the first time alterations of the RBC morphology, deformability, hydration as well as membrane lipid composition and lateral distribution in domains involved in RBC deformation (20, 22). Finally, Ezetimibe nearly improved all routine laboratory parameters and RBC fragility despite of having only a limited or no effect on the RBC morphology and lipid domains.
Data availability statement
The original contributions presented in the study are included in the article/Supplementary Material. Further inquiries can be directed to the corresponding author.
Ethics statement
The study was approved by the Medical Ethics Committee of the UCLouvain and the University Medical Center Utrecht (Study 17-450/M). The patients/participants provided their written informed consent to participate in this study.
Author contributions
A-SC and DT designed the experiments, analyzed and interpreted the data, and wrote the manuscript, the latter with input from MR and RW. JN, KK, AK, MR, BO and RW enrolled the patients and performed laboratory experiments. PVDS did the electron microscopy experiments. EM and YL were responsible for fatty acid analysis while RT and GGM performed lipidomics. All authors reviewed the final version of the manuscript.
Funding
This work was supported by grants from UCLouvain (FSR and Actions de Recherches concertées, ARC) and the F.R.S-FNRS.
Acknowledgments
We thank Drs. A. Miyawaki, M. Abe, T. Kobayashi (Riken Brain Science Institute, Saitama, Japan & University of Strasbourg, France) and H. Mizuno (KU Leuven, Belgium) for the Dronpa-theta-D4 plasmid for cholesterol labelling. Drs. M.G.G. de Sain-Van der Velden and J.J.M. Jans (Department of Metabolic Diagnostics, University Medical Center Utrecht, Utrecht University, Utrecht, The Netherlands) are acknowledged for the plasma sitosterol measurements for P2 and P3.
Conflict of interest
The authors declare that the research was conducted in the absence of any commercial or financial relationships that could be construed as a potential conflict of interest.
Publisher’s note
All claims expressed in this article are solely those of the authors and do not necessarily represent those of their affiliated organizations, or those of the publisher, the editors and the reviewers. Any product that may be evaluated in this article, or claim that may be made by its manufacturer, is not guaranteed or endorsed by the publisher.
Supplementary material
The Supplementary Material for this article can be found online at: https://www.frontiersin.org/articles/10.3389/frhem.2023.1055086/full#supplementary-material
References
1. Wang Z, Cao L, Su Y, Wang G, Wang R, Yu Z, et al. Specific macrothrombocytopenia/hemolytic anemia associated with sitosterolemia. Am. J. Hematol. (2014) 89(3):320–4. doi: 10.1002/ajh.23619
2. Yoo EG. Sitosterolemia: A review and update of pathophysiology, clinical spectrum, diagnosis, and management. Ann. Pediatr. Endocrinol. Metab. (2016) 21(1):7–14. doi: 10.6065/apem.2016.21.1.7
3. Rees DC, Iolascon A, Carella M, O'Marcaigh A S, Kendra JR, Jowitt SN, et al. Stomatocytic haemolysis and macrothrombocytopenia (Mediterranean stomatocytosis/macrothrombocytopenia) is the haematological presentation of phytosterolaemia. Br. J. Haematol. (2005) 130(2):297–309. doi: 10.1111/j.1365-2141.2005.05599.x
4. Wang G, Cao L, Wang Z, Jiang M, Sun X, Bai X, et al. Macrothrombocytopenia/stomatocytosis specially associated with phytosterolemia. Clin. Appl. Thromb. Hemost. (2012) 18(6):582–7. doi: 10.1177/1076029611435090
5. Bhattacharyya AK, Connor WE. Beta-sitosterolemia and xanthomatosis. a newly described lipid storage disease in two sisters. J. Clin. Invest. (1974) 53(4):1033–43. doi: 10.1172/JCI107640
6. Salen G, Shefer S, Nguyen L, Ness GC, Tint GS, Shore V. Sitosterolemia. J. Lipid Res. (1992) 33(7):945–55. doi: 10.1016/S0022-2275(20)41411-7
7. Izar MC, Tegani DM, Kasmas SH, Fonseca FA. Phytosterols and phytosterolemia: Gene-diet interactions. Genes Nutr. (2011) 6(1):17–26. doi: 10.1007/s12263-010-0182-x
8. Othman RA, Myrie SB, Jones PJ. Non-cholesterol sterols and cholesterol metabolism in sitosterolemia. Atherosclerosis (2013) 231(2):291–9. doi: 10.1016/j.atherosclerosis.2013.09.038
9. Berge KE, Tian H, Graf GA, Yu L, Grishin NV, Schultz J, et al. Accumulation of dietary cholesterol in sitosterolemia caused by mutations in adjacent ABC transporters. Science (2000) 290(5497):1771–5. doi: 10.1126/science.290.5497.1771
10. Salen G, Horak I, Rothkopf M, Cohen JL, Speck J, Tint GS, et al. Lethal atherosclerosis associated with abnormal plasma and tissue sterol composition in sitosterolemia with xanthomatosis. J. Lipid Res. (1985) 26(9):1126–33. doi: 10.1016/S0022-2275(20)34286-3
11. Miettinen TA. Phytosterolaemia, xanthomatosis and premature atherosclerotic arterial disease: a case with high plant sterol absorption, impaired sterol elimination and low cholesterol synthesis. Eur. J. Clin. Invest. (1980) 10(1):27–35. doi: 10.1111/j.1365-2362.1980.tb00006.x
12. Salen G, Patel S, Batta AK. Sitosterolemia. Cardiovasc. Drug Rev. (2002) 20(4):255–70. doi: 10.1111/j.1527-3466.2002.tb00096.x
13. Parsons HG, Jamal R, Baylis B, Dias VC, Roncari D. A marked and sustained reduction in LDL sterols by diet and cholestyramine in beta-sitosterolemia. Clin. Invest. Med. (1995) 18(5):389–400.
14. Othman RA, Myrie SB, Mymin D, Merkens LS, Roullet JB, Steiner RD, et al. Ezetimibe reduces plant sterol accumulation and favorably increases platelet count in sitosterolemia. J. Pediatr. (2015) 166(1):125–31. doi: 10.1016/j.jpeds.2014.08.069
15. Salen G, von Bergmann K, Lutjohann D, Kwiterovich P, Kane J, Patel SB, et al. Ezetimibe effectively reduces plasma plant sterols in patients with sitosterolemia. Circulation (2004) 109(8):966–71. doi: 10.1161/01.CIR.0000116766.31036.03
16. Lomenick B, Shi H, Huang J, Chen C. Identification and characterization of beta-sitosterol target proteins. Bioorg. Med. Chem. Lett. (2015) 25(21):4976–9. doi: 10.1016/j.bmcl.2015.03.007
17. Cloos AS, Ghodsi M, Stommen A, Vanderroost J, Dauguet N, Pollet H, et al. Interplay between plasma membrane lipid alteration, oxidative stress and calcium-based mechanism for extracellular vesicle biogenesis from erythrocytes during blood storage. Front. Physiol. (2020) 11:712. doi: 10.3389/fphys.2020.00712
18. Cloos AS, Daenen LGM, Maja M, Stommen A, Vanderroost J, van der Smissen P, et al. Impaired cytoskeletal and membrane biophysical properties of acanthocytes in hypobetalipoproteinemia - a case study. Front. Physiol. (2021) 12:638027. doi: 10.3389/fphys.2021.638027
19. Da Costa L, Suner L, Galimand J, Bonnel A, Pascreau T, Couque N, et al. Diagnostic tool for red blood cell membrane disorders: Assessment of a new generation ektacytometer. Blood Cells Mol. Dis. (2016) 56(1):9–22. doi: 10.1016/j.bcmd.2015.09.001
20. Conrard L, Stommen A, Cloos AS, Steinkuhler J, Dimova R, Pollet H, et al. Spatial relationship and functional relevance of three lipid domain populations at the erythrocyte surface. Cell Physiol. Biochem. (2018) 51(4):1544–65. doi: 10.1159/000495645
21. Zavodnik IB, Zaborowski A, Niekurzak A, Bryszewska M. Effect of free fatty acids on erythrocyte morphology and membrane fluidity. Biochem. Mol. Biol. Int. (1997) 42(1):123–33. doi: 10.1080/15216549700202501
22. Leonard C, Conrard L, Guthmann M, Pollet H, Carquin M, Vermylen C, et al. Contribution of plasma membrane lipid domains to red blood cell (re)shaping. Sci. Rep. (2017) 7(1):4264. doi: 10.1038/s41598-017-04388-z
23. Carquin M, Conrard L, Pollet H, van der Smissen P, Cominelli A, Veiga-da-Cunha M, et al. Cholesterol segregates into submicrometric domains at the living erythrocyte membrane: evidence and regulation. Cell. Mol. Life Sci. (2015) 72(23):4633–51. doi: 10.1007/s00018-015-1951-x
24. Bastida JM, Giros ML, Benito R, Janusz K, Hernandez-Rivas JM, Gonzalez-Porras JR. Sitosterolemia: Diagnosis, metabolic and hematological abnormalities, cardiovascular disease and management. Curr. Med. Chem. (2019) 26(37):6766–75. doi: 10.2174/0929867325666180705145900
25. Cao LJ, Yu ZJ, Jiang M, Bai X, Su J, Dai L, et al. [Clinical features of 20 patients with phytosterolemia causing hematologic abnormalities]. Zhonghua. Yi. Xue. Za. Zhi. (2019) 99(16):1226–31. doi: 10.3760/cma.j.issn.0376-2491.2019.16.007
26. Kaya Z, Niu DM, Yorulmaz A, Tekin A, Gursel T. A novel mutation of ABCG5 gene in a Turkish boy with phytosterolemia presenting with macrotrombocytopenia and stomatocytosis. Pediatr. Blood Cancer (2014) 61(8):1457–9. doi: 10.1002/pbc.24934
27. Su Y, Wang Z, Yang H, Cao L, Liu F, Bai X, et al. Clinical and molecular genetic analysis of a family with sitosterolemia and co-existing erythrocyte and platelet abnormalities. Haematologica (2006) 91(10):1392–5.
28. Andolfo I, Russo R, Gambale A, Iolascon A. Hereditary stomatocytosis: An underdiagnosed condition. Am. J. Hematol. (2018) 93(1):107–21. doi: 10.1002/ajh.24929
29. Clemens MR, Ruess M, Bursa Z, Waller HD. The relationship between lipid composition of red blood cells and their susceptibility to lipid peroxidation. Free Radic. Res. Commun. (1987) 3(1-5):265–71. doi: 10.3109/10715768709069792
30. Yoshida Y, Niki E. Antioxidant effects of phytosterol and its components. J. Nutr. Sci. Vitaminol. (Tokyo). (2003) 49(4):277–80. doi: 10.3177/jnsv.49.277
31. Wiley JS, Ellory JC, Shuman MA, Shaller CC, Cooper RA. Characteristics of the membrane defect in the hereditary stomatocytosis syndrome. Blood (1975) 46(3):337–56. doi: 10.1182/blood.V46.3.337.337
32. Zhang Z, He G, Filipowicz NA, Randall G, Belov GA, Kopek BG, et al. Host lipids in positive-strand RNA virus genome replication. Front. Microbiol. (2019) 10:286. doi: 10.3389/fmicb.2019.00286
33. Rungaldier S, Umlauf E, Mairhofer M, Salzer U, Thiele C, Prohaska R. Structure-function analysis of human stomatin: A mutation study. PloS One (2017) 12(6):e0178646. doi: 10.1371/journal.pone.0178646
34. Albuisson J, Murthy SE, Bandell M, Coste B, Louis-Dit-Picard H, Mathur J, et al. Dehydrated hereditary stomatocytosis linked to gain-of-function mutations in mechanically activated PIEZO1 ion channels. Nat. Commun. (2013) 4:1884. doi: 10.1038/ncomms2899
Keywords: hemolytic anemia, β-sitosterol, phosphatidylethanolamine, cholesterol, lipid domains, stomatocytes, ektacytometry, Ezetimibe
Citation: Cloos A-S, Rab MAE, Van Der Smissen P, van Oirschot BA, Mignolet E, van der Net JB, Koster A, Kleinen K, Larondelle Y, Terrasi R, Muccioli GG, van Wijk R and Tyteca D (2023) Red blood cells from patients with sitosterolemia exhibit impaired membrane lipid composition and distribution and decreased deformability. Front. Hematol. 2:1055086. doi: 10.3389/frhem.2023.1055086
Received: 27 September 2022; Accepted: 16 January 2023;
Published: 07 March 2023.
Edited by:
Lars Kaestner, Saarland University, GermanyReviewed by:
Theodosia A. Kalfa, Cincinnati Children’s Hospital Medical Center, United StatesSyed M Qadri, Ontario Tech University, Canada
Copyright © 2023 Cloos, Rab, Van Der Smissen, van Oirschot, Mignolet, van der Net, Koster, Kleinen, Larondelle, Terrasi, Muccioli, van Wijk and Tyteca. This is an open-access article distributed under the terms of the Creative Commons Attribution License (CC BY). The use, distribution or reproduction in other forums is permitted, provided the original author(s) and the copyright owner(s) are credited and that the original publication in this journal is cited, in accordance with accepted academic practice. No use, distribution or reproduction is permitted which does not comply with these terms.
*Correspondence: Donatienne Tyteca, donatienne.tyteca@uclouvain.be