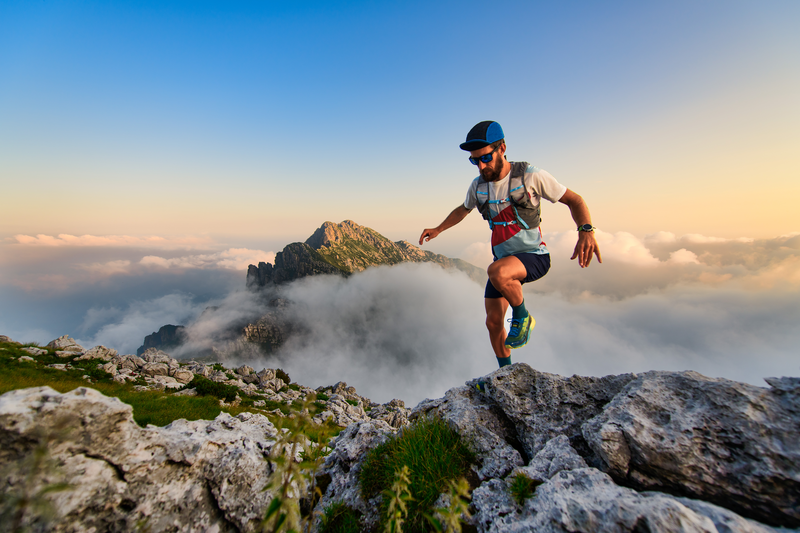
95% of researchers rate our articles as excellent or good
Learn more about the work of our research integrity team to safeguard the quality of each article we publish.
Find out more
EDITORIAL article
Front. Rehabil. Sci. , 13 February 2025
Sec. Rehabilitation in Neurological Conditions
Volume 6 - 2025 | https://doi.org/10.3389/fresc.2025.1563975
This article is part of the Research Topic Rehabilitation to Guide Functional Plasticity and Regeneration with Novel Cellular, Pharmacological and Neuromodulation Therapies View all 6 articles
Editorial on the research topic
Rehabilitation to guide functional plasticity and regeneration with novel cellular, pharmacological and neuromodulation therapies
While we, as a research field, strive to improve outcomes for people with neurological conditions, we understand that no single therapy or intervention can work in isolation. Combining methods represents the future of optimizing outcomes in rehabilitation. Research on combinatorial treatments remains limited. While some studies have explored the combination of exercise- or activity-based therapies with neuromodulation, little has been done to investigate the integration of neuromodulation with cellular or pharmacologic treatments. Given the etablished safety of a broad range of neuromodulation techniques, there is an interesting opportunity to further investigate the potential benefits of combining pharmacologic approaches with neuromodulation.
The quest to restore function following neurological injuries continues to drive innovation in the field of rehabilitation. Despite the complexity of central nervous system injuries and the limited capacity for regeneration, promising avenues are emerging. By integrating rehabilitation with cutting-edge cellular therapies, pharmacological interventions, and neuromodulation strategies, researchers aim to harness the body's inherent plasticity to facilitate recovery and functional regeneration.
Spinal cord injury (SCI) rehabilitation offers a compelling example of these advancements. Cervical SCI disrupts critical neural circuits controlling upper limb function. While endogenous repair mechanisms promote reorganization and adaptive plasticity in sparred circuits, maladaptive rewiring can hinder functional recovery (1–6). Therefore, strategies targeting the functional rewiring of motor pathways are essential to enhance meaningful recovery. Multiple preclinical (7, 8) and clinical (9, 10) studies have demonstrated that rehabilitation improves functional recovery after SCI by training the spared motor networks and providing activity-dependent feedback to locomotor pathways. For instance, Gregoire Courtine's research on neuromodulation for SCI recovery in humans highlights the integration of rehabilitation strategies with epidural (11) or transcutaneous spinal cord stimulation (12), brain–spine interfaces (13, 14), and hypothalamic deep brain stimulation (15). Importantly, the neuroplastic changes induced by rehabilitation training are dependent on the type of adopted training paradigm (16). Strength training primarily modulates motor network excitability and increases number of synapses, whereas skilled motor training elicits broader mechanisms, including synapse formation, enhanced synaptic strength, and network reorganization (16). In stroke, studies on anti-NOGO therapy demonstrate that its efficacy is optimized when combined sequentially with an appropriate rehabilitation regimen (17). These examples underscore the critical need for combined and targeted rehabilitation paradigms.
Building on these concepts, this research topic examines perspectives on combining rehabilitation with advanced therapies, including stem cell applications for SCI (Balbinot), the safety of Hebbian-type stimulation (Haakana et al.), personalized strategies for pediatric cerebral palsy (Behboodi et al., Raess et al.), and the sex-specific effects of acrobatic training on cognitive decline induced by cerebral hypoperfusion (Martini et al.).
Balbinot emphasizes the synergy between targeted rehabilitation and stem cell-based therapies, particularly for improving upper extremity function in cervical SCI. Preclinical studies highlight the necessity of combining regenerative strategies with rehabilitation protocols that mirror clinical practices, notably using neuromodulation to activate neural circuits below the injury level. Techniques such as corticospinal tract and spinal cord stimulation represent a promising frontier to enhance the efficacy of cell-based therapies for severe upper extremity paralysis. The convergence of these approaches holds significant hope for unlocking new treatments in the clinical setting.
Adding further depth, a novel neuromodulation protocol of paired associative stimulation (high PAS), combines high-intensity transcranial magnetic stimulation with high-frequency peripheral nerve stimulation to target corticospinal tract plasticity (Haakana et al., 18, 19). Preliminary findings by Haakana et al. on heart rate variability indicate that this approach is safe, inducing short-term modulation of parasympathetic activity without sustained cardiovascular effects. High PAS has the potential to enhance rehabilitation for neurological conditions, further emphasizing the need for continued exploration of its systemic impacts—especially when combined with other plasticity enhancing approaches.
In parallel, the adaptability and therapeutic potential of neurological interventions extend to pediatric conditions such as cerebral palsy. Functional electrical stimulation has demonstrated mixed results in improving gait kinematics (Behboodi et al.). This highlights the importance of identifying neurotherapeutic responders to optimize individualized protocols tailored to individual needs. Furthermore, combining robotic rehabilitation paired with transcranial direct current stimulation shows promise for enhancing upper extremity motor outcomes. Raess et al. show that, despite logistical challenges and patient-specific barriers, this combination shows feasibility and tolerability, providing a foundation for future research to elucidate optimal protocols for clinical application.
Finally the challenge of treating chronic cerebral hypoperfusion is addressed through innovative strategies such as acrobatic training (Martini et al.). Martini et al. show that this intervention mitigates astrocytic remodeling in hippocampal subfields associated with spatial memory impairments while uncovering sex-specific response. In males, training appears to increase astrocyte populations and improve memory retention, whereas in females, it enhances cell viability, highlighting the nuanced interplay between rehabilitation therapies and sex-specific cellular plasticity.
In conclusion, the integration of advanced cellular, pharmacological, and neuromodulation therapies with comprehensive rehabilitation strategies heralds a new era of possibilities for functional recovery in neurological conditions. While challenges remain, it is imperative to rigorously assess the biological plausibility of these technologies as a cornerstone of their validation. Drawing from the Bradford-Hill criteria (20), this focus on plausibility ensures that the mechanisms driving neural regeneration and plasticity are both scientifically credible and capable of being translated into effective clinical applications. Such a framework is essential for harnessing these interventions to maximize neuroplasticity and advance the field of rehabilitation sciences.
RM: Writing – original draft, Writing – review & editing. SK-R: Writing – original draft, Writing – review & editing. GG: Writing – original draft, Writing – review & editing. GB: Writing – original draft, Writing – review & editing.
The authors declare that the research was conducted in the absence of any commercial or financial relationships that could be construed as a potential conflict of interest.
The author(s) declared that they were an editorial board member of Frontiers, at the time of submission. This had no impact on the peer review process and the final decision.
All claims expressed in this article are solely those of the authors and do not necessarily represent those of their affiliated organizations, or those of the publisher, the editors and the reviewers. Any product that may be evaluated in this article, or claim that may be made by its manufacturer, is not guaranteed or endorsed by the publisher.
1. Bareyre FM, Kerschensteiner M, Raineteau O, Mettenleiter TC, Weinmann O, Schwab ME. The injured spinal cord spontaneously forms a new intraspinal circuit in adult rats. Nat Neurosci. (2004) 7:269–77. doi: 10.1038/nn1195
2. Cohen LG, Bandinelli S, Topka HR, Fuhr P, Roth BJ, Hallett M. Topographic maps of human motor cortex in normal and pathological conditions: mirror movements, amputations and spinal cord injuries. Electroencephalogr Clin Neurophysiol Suppl. (1991) 43:36–50.1773774
3. Green JB, Sora E, Bialy Y, Ricamato A, Thatcher RW. Cortical motor reorganization after paraplegia: an EEG study. Neurology. (1999) 53:736–43. doi: 10.1212/WNL.53.4.736
4. Green JB, Sora E, Bialy Y, Ricamato A, Thatcher RW. Cortical sensorimotor reorganization after spinal cord injury: an electroencephalographic study. Neurology. (1998) 50:1115–21. doi: 10.1212/WNL.50.4.1115
5. von Euler M, Janson AM, Larsen JO, Seiger A, Forno L, Bunge MB, et al. Spontaneous axonal regeneration in rodent spinal cord after ischemic injury. J Neuropathol Exp Neurol. (2002) 61:64–75. doi: 10.1093/jnen/61.1.64
6. Zai LJ, Wrathall JR. Cell proliferation and replacement following contusive spinal cord injury. Glia. (2005) 50:247–57. doi: 10.1002/glia.20176
7. de Leon RD, Hodgson JA, Roy RR, Edgerton VR. Locomotor capacity attributable to step training versus spontaneous recovery after spinalization in adult cats. J Neurophysiol. (1998) 79:1329–40. doi: 10.1152/jn.1998.79.3.1329
8. Leon RDD, Hodgson JA, Roy RR, Edgerton VR. Locomotor capacity attributable to step training versus spontaneous recovery following spinalization in adult cats. J Neurophysiol. (1998) 79(3):1329–40. doi: 10.1152/jn.1998.79.3.1329
9. Behrman AL, Harkema SJ. Physical rehabilitation as an agent for recovery after spinal cord injury. Phys Med Rehabil Clin N Am. (2007) 18:183–202, v. doi: 10.1016/j.pmr.2007.02.002
10. Behrman AL, Harkema SJ. Locomotor training after human spinal cord injury: a series of case studies. Phys Ther. (2000) 80:688–700. doi: 10.1093/ptj/80.7.688
11. Wagner FB, Mignardot JB, Le Goff-Mignardot CG, Demesmaeker R, Komi S, Capogrosso M, et al. Targeted neurotechnology restores walking in humans with spinal cord injury. Nature. (2018) 563:65–71. doi: 10.1038/s41586-018-0649-2
12. Moritz C, Field-Fote EC, Tefertiller C, Van Nes I, Trumbower R, Kalsi-Ryan S, et al. Non-invasive spinal cord electrical stimulation for arm and hand function in chronic tetraplegia: a safety and efficacy trial. Nat Med. (2024) 30:1276–83. doi: 10.1038/s41591-024-02940-9
13. Hachem LD, Balbinot G, Fehlings MG. A digital bridge to reverse paralysis. Cell Res. (2023) 33:892–3. doi: 10.1038/s41422-023-00845-9
14. Lorach H, Galvez A, Spagnolo V, Martel F, Karakas S, Intering N, et al. Walking naturally after spinal cord injury using a brain–spine interface. Nature. (2023) 618:126–33. doi: 10.1038/s41586-023-06094-5
15. Cho N, Squair JW, Aureli V, James ND, Bole-Feysot L, Dewany I, et al. Hypothalamic deep brain stimulation augments walking after spinal cord injury. Nat Med. (2024) 30:3676–86. doi: 10.1038/s41591-024-03306-x
16. Adkins DL, Boychuk J, Remple MS, Kleim JA. Motor training induces experience-specific patterns of plasticity across motor cortex and spinal cord. J Appl Physiol. (2006) 101:1776–82. doi: 10.1152/japplphysiol.00515.2006
17. Wahl AS, Omlor W, Rubio JC, Chen JL, Zheng H, Schröter A, et al. Asynchronous therapy restores motor control by rewiring of the rat corticospinal tract after stroke. Science. (2014) 344(6189):1250–5. doi: 10.1126/science.12530
18. Bunday KL, Perez MA. Motor recovery after spinal cord injury enhanced by strengthening corticospinal synaptic transmission. Curr Biol. (2012) 22:2355–61. doi: 10.1016/j.cub.2012.10.046
19. Jo HJ, Perez MA. Corticospinal-motor neuronal plasticity promotes exercise-mediated recovery in humans with spinal cord injury. Brain. (2020) 143:1368–82. doi: 10.1093/brain/awaa052
Keywords: spinal cord injuries, stroke, cerebral palsy, neuromodulation, rehabilitation
Citation: Mestriner RG, Kalsi-Ryan S, Gholamrezaei G and Balbinot G (2025) Editorial: Rehabilitation to guide functional plasticity and regeneration with novel cellular, pharmacological and neuromodulation therapies. Front. Rehabil. Sci. 6:1563975. doi: 10.3389/fresc.2025.1563975
Received: 20 January 2025; Accepted: 31 January 2025;
Published: 13 February 2025.
Edited and Reviewed by: Tsan-Hon Liou, Taipei Medical University, Taiwan
Copyright: © 2025 Mestriner, Kalsi-Ryan, Gholamrezaei and Balbinot. This is an open-access article distributed under the terms of the Creative Commons Attribution License (CC BY). The use, distribution or reproduction in other forums is permitted, provided the original author(s) and the copyright owner(s) are credited and that the original publication in this journal is cited, in accordance with accepted academic practice. No use, distribution or reproduction is permitted which does not comply with these terms.
*Correspondence: Gustavo Balbinot, Z3VzdGF2b19iYWxiaW5vdEBzZnUuY2E=
Disclaimer: All claims expressed in this article are solely those of the authors and do not necessarily represent those of their affiliated organizations, or those of the publisher, the editors and the reviewers. Any product that may be evaluated in this article or claim that may be made by its manufacturer is not guaranteed or endorsed by the publisher.
Research integrity at Frontiers
Learn more about the work of our research integrity team to safeguard the quality of each article we publish.