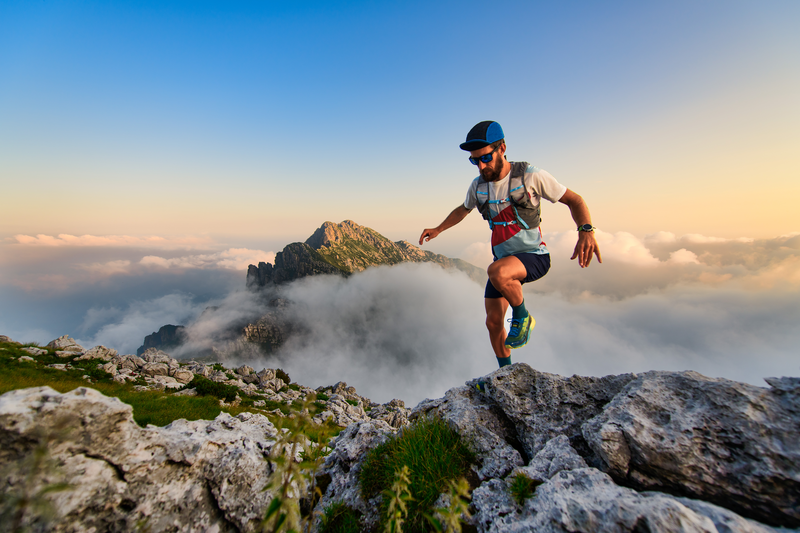
94% of researchers rate our articles as excellent or good
Learn more about the work of our research integrity team to safeguard the quality of each article we publish.
Find out more
SYSTEMATIC REVIEW article
Front. Rehabil. Sci. , 02 April 2024
Sec. Medical and Surgical Rehabilitation
Volume 5 - 2024 | https://doi.org/10.3389/fresc.2024.1336042
This article is part of the Research Topic Advances in Technology-Assisted Rehabilitation View all 17 articles
Introduction: Bone-anchored prostheses (BAP) are an advanced reconstructive surgical approach for individuals who had transfemoral amputation and are unable to use the conventional socket-suspension systems for their prostheses. Access to this technology has been limited in part due to the lag between the start of a new procedure and the availability of evidence that is required before making decisions about widespread provision. This systematic review presents as a single resource up-to-date information on aspects most relevant to decision makers, i.e., clinical efficacy, safety parameters, patient experiences, and health economic outcomes of this technology.
Methods: A systematic search of the literature was conducted by an information specialist in PubMed, MEDLINE, Embase, CINAHL, Cochrane Library, the Core Collection of Web of Science, CADTH's Grey Matters, and Google Scholar up until May 31, 2023. Peer-reviewed original research articles on the outcomes of clinical effectiveness (health-related quality of life, mobility, and prosthesis usage), complications and adverse events, patient experiences, and health economic outcomes were included. The quality of the studies was assessed using the Oxford Centre for Evidence-Based Medicine Levels of Evidence and ROBINS-I, as appropriate.
Results: Fifty studies met the inclusion criteria, of which 12 were excluded. Thirty-eight studies were finally included in this review, of which 21 reported on clinical outcomes and complications, 9 case series and 1 cohort study focused specifically on complications and adverse events, and 2 and 5 qualitative studies reported on patient experience and health economic assessments, respectively. The most common study design is a single-arm trial (pre-/post-intervention design) with varying lengths of follow-up.
Discussion: The clinical efficacy of this technology is evident in selected populations. Overall, patients reported increased health-related quality of life, mobility, and prosthesis usage post-intervention. The most common complication is a superficial or soft-tissue infection, and more serious complications are rare. Patient-reported experiences have generally been positive. Evidence indicates that bone-anchored implants for prosthesis fixation are cost-effective for those individuals who face significant challenges in using socket-suspension systems, although they may offer no additional advantage to those who are functioning well with their socket-suspended prostheses.
Lower-limb amputation severely impacts physical function, psychological well-being, and social participation (1–8). Following a transfemoral (above-knee) amputation, the standard of care for restoring mobility is to fit the individual with a prosthesis that consists of a socket-suspension system to which the prosthetic components (such as the knee and foot) are attached. Approximately 86% of people with major lower-limb amputation are fit with a socket prosthesis (9). A trained prosthetist is required to custom-design the socket for each user according to the condition and shape of their residual limb. Suction to the residual limb or strapping around the pelvis is necessary for a socket to fit properly. Although prosthetic socket-suspension systems have evolved over the past few decades with substantial technological advancements, there are still limitations to their use. The socket must fit firmly to the residual limb to ensure comfort, transmit forces of the skeleton to the ground, and enable the movement of the residual limb to control the position of the prosthetic limb. The interface between the socket and the residual limb is one of the most crucial factors for the success of the prosthesis; however, discomfort and problems related to socket fit are common and have been shown to negatively affect the quality of life and mobility of the user (10–13). The problems that plague many prosthetic users are the lack of comfort, skin ulcers (14), inadequate or fluctuating suspension (15), tissue irritation, excessive heat and perspiration (14), poor control due to the motion of the soft tissue within the socket, and low confidence with mobility (12). Chronic skin problems and pain caused by friction between the residual limb and the prosthesis have been reported in 34%–63% of socket prosthesis users, reducing the use and function of the prosthetic device, quality of life, and body image satisfaction (12, 16–19). In addition, the socket can restrict the range of movement of the hip, leading to difficulties in sitting or participating in the activities of daily living. If the user experiences poor outcomes and problems with the socket, repeat visits to a physician or the prosthetist are required for assessment and adjustment. By some estimates, frequent refitting is typical in up to three-quarters of socket prosthesis users (11). Individuals who are unable to use the socket-suspension systems due to recurrent problems may completely abandon their prostheses (20, 21).
These problems spurred the development of new techniques to attach prosthetic components directly to a titanium implant that is inserted into the bone of the residual limb, obviating the need for a socket interface. Since titanium is naturally biocompatible (non-toxic and non-allergenic), titanium implant integrates with living bone tissue. This process, termed osseointegration (OI), results in a bone-anchored prosthesis in which the implant that extends percutaneously, i.e., through the skin, allows a direct functional and structural connection to the prosthetic components (22, 23). Bone-anchored implants have been used for dental and maxillofacial reconstructions for decades, and since the 1990s, they have been used for prosthetic reconstructions for individuals with transfemoral amputations (24). Bone-anchored prosthesis is a treatment option for various amputation levels in several areas worldwide.
Implants and protocols for bone-anchored prostheses have emerged and evolved over the past several years. Currently, there are two main types of fixations in use, namely, the screw-type (threaded) and press-fit type. Based on these two types of fixations, there are six types of implants for which evidence is available in the peer-reviewed literature (25). The first surgery for a person with transfemoral amputation occurred in 1990 in Sweden with the earliest design [called Osseointegrated Prostheses for the Rehabilitation of Amputees (OPRA)] (26). This is the only type of implant that relies on a screw-type fixation. As the name suggests, the titanium alloy implant is secured to the femur using a threading tool to cut spiral groove threads in the intramedullary cortex of the residual bone and then screwed into the femur. The OPRA technique is characterized by two surgical stages spaced 6 months apart. The success of the osseointegrated prostheses in Sweden spurred the design of implants in Germany in the late 1990s. This implant design diverted from screw-type fixation to intramedullary press-fit alloy devices similar to those used in joint arthroplasty. This led to the design of the Integral Leg Prosthesis (ILP; Orthodynamics, Germany), which was called the Endo-Exo Prosthesis (EEP; ESKA Orthopaedic, Germany) in its earlier iterations. In its latest iteration, the “third generation” of EEP is called Transcutaneous Osseointegrated Prosthetic Systems (TOPS) (27). The EEP and its successor ILP also rely on a two-stage surgical procedure, but the time between surgeries is reduced to 4–6 weeks. The Osseointegrated Prosthetic Limb (OPL; Permedica S.p.A., Italy) evolved from the experience with the ILP and is used in either a two- or single-stage surgery. More recently, the Bone Anchoring Device for Artificial Limbs (BADAL X) attachment using OTN Implants (the Netherlands) has been reported (28). Details on the varying designs and surgical and rehabilitation approaches are published elsewhere (25). Each type of implant has varying levels of evidence in the published literature on clinical efficacy outcomes and complications (25, 29, 30).
Two other types of implants are reported in the literature but are not the focus of this review since they are still in the development stage and/or lack adequate published literature for review. The Compress Device (Zimmer Biomet) was initially designed as a solution for large-gap limb salvage for patients with bone tumors, which is still used for that purpose (25). Since the Compress Device is a newer system, surgical techniques or rehabilitation guidelines have not yet been published. Despite having just finished its clinical trial, the Intraosseous Transcutaneous Amputation Prosthesis (ITAP; Stryker Orthopaedics; ClinicalTrials.gov no. NCT02491424) not be released due to a reported higher risk of infection and implant failure (31, 32).
Since the original surgeries in the 1990s in Sweden, various centers have begun providing BAP and publishing reports on clinical outcomes and complications, including centers in Germany, the Netherlands, Australia, the UK, the USA, and Canada. With the growing body of evidence on the outcomes of BAP from various groups around the world, there is increasing pressure on publicly and privately funded health insurance systems to make this procedure more widely available to all patients who could benefit. Implementation in new centers has been sporadic, typically facility determined, with discrepancies across private and publicly funded health systems. Inequitable access could be partly due to the unavailability of a single resource that brings together information on aspects most relevant for policymakers when making decisions about the provision of this new technology. Systematic reviews have covered outcomes (33–35), complications (36, 37), and implant design (25, 37); however, given the rapidly evolving evidence in this field, this review responds to the need for a single resource that presents an updated systematic review (by type of implant, where reported) of clinical efficacy outcomes, complications, patient preferences, and cost-effectiveness.
This review aims to present a systematic review to answer the four main questions that regulatory bodies and policymakers pose: What are the (a) clinical efficacy, (b) safety, (c) patient experience, and (d) cost-effectiveness of bone-anchored implants that enable attachment of prosthetic devices for persons with transfemoral amputations?
We conducted a systematic review that adhered to the PRISMA 2020 checklists (Supplementary Appendices S1, S2) (38). An a priori protocol was drafted according to the PRISMA-P guidelines (39) and was made available online (40). An experienced medical information specialist developed and tested the search strategies through an iterative process in consultation with the review authors. Using the multifile and deduplication tool options in OVID, we searched Ovid MEDLINE ALL, including ePub Ahead of Print, In-Process & Other Non-indexed Citations, and Embase. In addition, we searched the Cochrane Library (Wiley), CINAHL (Ebsco), Web of Science Core Collection, and PubMed. All searches were performed on 14 March 2021 and updated on 31 May 2023. The strategies utilized a combination of controlled vocabulary (e.g., “bone-anchored prosthesis,” “osseointegration,” “bones of the lower extremity”) and keywords (e.g., “OPRA,” “osseo-anchor,” “femur”). Vocabulary and syntax were adjusted across the databases, and no language or date restrictions were imposed, although animal-only records were removed where possible. The results were downloaded and deduplicated using EndNote version 9.3.3 (Clarivate Analytics) and uploaded to Covidence (41). We performed a gray literature search using CADTH's Grey Matters and Google Scholar to ensure that no primary articles of interest were missed. The reference lists of the articles selected for full-text or included in this review were also searched for additional sources. Specific details regarding the strategies appear in Supplementary Appendix S3. Title and abstract screening and primary exclusion upon full-text review were carried out by two reviewers (MR and TS). Any conflicts at these stages were handled by consensus (between MR and TS), and a third reviewer (JSH) served as an arbiter when needed. Secondary exclusion upon full-text review was conducted by one reviewer (MR) and verified by another (JSH) who is a subject matter expert in prosthesis research.
Population: Individuals with a unilateral or bilateral transfemoral amputation.
Intervention: Percutaneous osseointegrated/bone-anchored implants to which external prosthetic components are attached.
Comparator: Socket-suspension prosthesis systems or no prostheses.
Outcomes: (1) To assess clinical outcomes, health-related quality of life (HRQoL), and functional outcomes, such as mobility, and prosthesis usage, (2) clinical complications and adverse events, (3) patient experiences of benefits and challenges, and (4) any health economic variable.
Time: No restriction.
Studies: Articles published in peer-reviewed journals that were as follows: (1) experimental or observational studies with outcomes data on an intervention group and the comparator and studies with a pre-/post-design, (2) studies reporting specifically on complications and adverse events, (3) studies exploring patient experiences using qualitative methods, and (4) health economic evaluations based on, but not limited to, cost-comparison, cost–benefit analysis, cost-minimization analysis, cost-effectiveness analysis, or cost-utility analysis.
Primary peer-reviewed research reports meeting the PICOTS criteria were included.
Articles not meeting the PICOTS criteria were excluded at this stage. Other health technology assessments, literature reviews, case reports, opinion pieces, and editorials were also excluded.
At this stage, articles that (1) reported on the same patients as those included in other studies, (2) did not report data for the transfemoral level separately, (3) self-identified as interim reports of longer-term follow-up studies, (4) described a bone-anchored prosthesis intervention not of interest, or (5) did not report data on the comparator group were also excluded.
Information was extracted by one reviewer (MR) from included studies only. Essential characteristics of the studies, e.g., implant type, city and country of the center publishing the study, funding source, study type, comparator, length of follow-up, details about external prosthetic components, number of participants, sex ratio, numbers of participants with unilateral or bilateral amputation, age of participants at treatment, time between amputation and surgery, etiology of the patients, and outcomes of interest, were extracted. Quantitative data on outcome measures of clinical efficacy were extracted, collated, and presented in tables.
To assess the quality of the literature, studies on clinical efficacy were reviewed by two reviewers (MR and TS) who assigned the Oxford Centre for Evidence-Based Medicine (OCEBM) Levels of Evidence (42) by consensus and carried out The Risk Of Bias In Non-randomized Studies of Interventions (ROBINS-I) (43). ROBINS-I is a tool designed to evaluate the risk of bias in the estimates of effectiveness or safety in studies that do not randomize the allocation of participants. It is well suited to cohort studies and single-arm trials as it assesses the risks to external validity due to confounding bias, selection bias, information bias, and reporting bias. The severity of these risks of bias is evaluated based on pre-established criteria and rated as “low,” “moderate,” “serious,” “critical,” and “no information,” where applicable.
The quality of literature focusing on complications was assessed by determining the OCEBM Levels of Evidence by one reviewer (MR) and verified by another (TS).
Figure 1 shows the study selection process as a PRISMA diagram. In total, 3,294 references were found in the 8 databases after removing the duplicates. After title and abstract screening by two reviewers, 132 articles were selected for full-text review. Eighty-two articles were excluded based on the primary exclusion criteria; however, an additional 12 were excluded based on the secondary exclusion criteria. Finally, 38 studies were included in this review. Twenty-one were on clinical efficacy outcomes (HRQoL, mobility, or prosthesis usage) with single-arm trial (pre-/post-intervention follow-up) design or cohort studies; nine case series (prospective or retrospective) and one cohort study were specifically reported on infectious or serious complications, two reported on patient experiences based on qualitative research methods, and five reported on health economic evaluations. Table 1 shows the list of studies (by implant type) included for evaluating clinical efficacy outcomes and information on study characteristics. Supplementary Table S1 shows the list of studies excluded at the secondary exclusion stage and the reasons for exclusion.
Commonly reported outcomes of clinical efficacy were HRQoL (as measured by patient-reported outcome measures such as SF-36, EQ-5D, and the Questionnaire for Persons with a Transfemoral Amputation; Q-TFA), mobility (as measured by a trained observer/clinician using instruments such as 2-min Walk Test; 2MWT, 6-min Walk Test; 6MWT, 10-min Walk Test; 10MWT, Timed-Up-and-Go Test; TUG), self-perceived mobility (as measured by PLUS-M), prosthesis usage, and safety parameters (which included the number and types of complications and adverse events). SF-36 was the most commonly reported generic HRQoL outcome measure. Q-TFA is a condition-specific HRQoL outcome measure to assess transfemoral prosthesis users’ quality of life and overall prosthetic situation (64). 2MWT, 6MWT, 10MWT, and TUG are performance-based tests administered and scored by a clinician. They measure the ambulatory potential of persons with lower-limb amputation with and without a prosthesis (65, 66).
Most of the included studies on clinical efficacy reported comparing outcomes before surgery (when patients used the comparator, i.e., socket-suspension systems or no prostheses) and after surgery with varying lengths of follow-up. Please refer to Figure 2 for a Gantt-chart-type depiction of the timeline of surgeries and follow-up in the included literature on the various bone-anchored implant types. Seventeen of the 21 studies were single-arm trials with pre-/post-intervention follow-up designs (where participant served as their own control) and 4 were cohort studies (where the comparisons were made between two distinct groups, the OI intervention group and the socket group). The shortest length of follow-up in the single-arm trials was 1 year postsurgery, and the longest was 15 years postsurgery. Twelve studies were either 1-year or 2-year postsurgery follow-ups. All seventeen single-arm trials of the 21 included studies on clinical efficacy outcomes also reported on complications in varying detail; the other four that did not were cohort studies. The two qualitative research studies were based on phenomenological methods. Two of the five health economic evaluations were based on cost-comparison analysis and three on cost-utility analyses.
Figure 2. Lengths of follow-up of outcomes and complications and years of publication (by implant type).
The quality of the included single-arm trials (pre-/post-intervention follow-up) and cohort studies was assessed using the OCEBM Levels of Evidence and ROBINS-I. The quality of the included case series was assessed using OCEBM Levels of Evidence. Supplementary Table S2 shows the quality assessment results for the studies on clinical outcomes. The general quality of evidence ranged from OCEBM Level 2 to 4, as most studies were single-arm trials or observational case series. The single-arm trials in this review were generally rated at Level 2 as they were deemed similar to well-designed clinical trials with several objective outcome measures and pre-/post-data on patients serving as their own controls. Cohort studies and case series (prospective or retrospective) were rated at Levels 3 and 4, respectively. Current practice patterns preclude study designs of higher methodological quality (such as RCTs) as difficulty with the socket-suspension system is generally considered a requirement for bone-anchored implants. Single-arm trials or cohort studies are therefore considered a methodologically robust and ethical way of comparing socket-suspension to bone-anchored implants.
The risk of bias (assessed using the ROBINS-I) was evaluated over four domains. The risk of confounding bias was generally considered moderate for most studies. In single-arm trials with pre-/post-design, the impact of any baseline confounding is typically minimal. In cohort studies, the risk of baseline confounding exists. One of the potential confounders identified in this review was the reporting of external prosthetic components that are attached to the bone-anchored implant. In studies where information about external prosthetic components was not reported, this risk was unknown and could have contributed to unmeasured confounding, therefore resulting in a moderate rating of confounding bias for several studies. The risk of selection bias was generally low. A lack of consistent reporting of how missing data was handled made the assessment of selection bias due to missing data challenging. Information bias due to the classification of interventions was considered low but was generally considered moderate due to the selection of outcome measures. The risk of reporting bias was generally rated as moderate but serious in two studies. The details on the choice of rating and rationale are presented in Supplementary Table S2.
The literature reported fairly consistent requirements for individuals to be selected for transfemoral OI surgery. Table 2 shows the inclusion and exclusion criteria in each of the included studies. The most common inclusion criteria are recurrent problems or the inability to use socket prostheses (28, 44–52, 54–58, 63), mature skeleton (28, 44, 45, 47–49, 52), or normal residual skeletal anatomy (44, 45, 47), the ability to comply with the treatment and follow-up requirements (45–48, 52, 54, 56, 62), and pre-surgical evaluation by a clinical team (44, 46, 51, 52, 57, 63) along with physical and medical examinations and imaging (44, 45, 47). There also are several contraindications to the OI surgery. The most common exclusion criteria are severe peripheral vascular disease (44–47, 49–52, 54, 56, 57), diabetes mellitus (28, 44–46, 49–52, 54, 56, 57, 62), treatment with chemotherapy (44–46, 49, 50, 52, 54, 56, 57, 63), exposure of the amputated limb to radiation (28, 52, 54, 56–58, 63), current treatment with corticosteroids (44–46) or immunosuppressive drugs (28, 54, 56, 57, 62), and pregnancy (44–46, 54, 56). The most common age range reported for patient selection in the included studies was between 18 and 70 years.
Table 2. Patient selection/inclusion and exclusion criteria in included studies on clinical outcomes.
Table 3 summarizes the results from the included studies on the clinical efficacy outcomes of interest (HRQoL, mobility, and prosthesis usage) for each type of implant. The participants in most included studies underwent OI surgery in their mid-40s, had unilateral transfemoral OI surgery, and underwent primary amputation due to trauma. Time between amputation and the OI surgery varied greatly between studies and ranged from 10 months (44) to 52 years (52). Three studies declared receiving funds, in whole or part, from commercial entities; whereas, 10 studies declared funding, in whole or part, from non-commercial and non-profit sources or government grants. Six declared having received no funding and three did not report having funding sources. The authors of seven studies declared a financial conflict of interest in the companies that supplied the implants. Seven studies were based on implants with screw-type fixation and 14 on those with press-fit fixation. All types of implants (screw-type or press-fit type) showed an improvement to varying degrees in HRQoL, mobility, and prosthesis usage when the pre-surgical condition (with socket prosthesis) of the patients is compared to their postsurgical condition. Most studies were based on the duration of follow-up of 1 year (28, 48, 51, 54–56, 58, 62, 63) or 2 years (44, 45, 57) post-intervention. There were three studies based on a 5-year follow-up (46, 47, 52) and one study each for 10-year (50) and 15-year (49) follow-ups, respectively, post-intervention.
Table 3. Reported clinical outcomes and results of quality of life, mobility outcomes, and prosthetic usage (by implant type).
Compared to baseline, an improvement in SF-36 physical component score (PCS) was reported at 1-year (54, 56), 2-year (44–47, 57), 5-year (46, 47), and 10-year (50) follow-ups. The SF-36 mental component score (MCS), however, was reported to have improved at a 2-year (57) follow-up in one study but did not consistently show improvements in other studies. The condition-specific HRQoL measure, Q-TFA global score, showed an improvement at 1-year (28, 51, 54–56, 58, 62), 2-year (44, 45), 5-year (46, 47, 52), 10-year (50), and 15-year (49) follow-up. Reduction in problems due to the prosthesis, measured by Q-TFA problem score, was reported at 1-year (48, 58, 62), 2-year (44, 45), 5-year (46, 47), 10-year (50), and 15-year (49) follow-up. Two articles (46, 47) presented 5-year follow-up data and reported the interim 2-year follow-up data. They reported that the differences between 2- and 5-year follow-ups for all SF-36 domains and Q-TFA subscales were not statistically significant. Similarly, there were no significant differences in these measures between 5- and 10-year follow-ups (50). A significant reduction in disability (as measured by the WHODAS 2.0) was also reported at 1-year follow-up (63).
Out of the four cohort studies, three reported on the differences in HRQoL outcomes between bone-anchored prosthesis and socket prosthesis users (59–61). Two studies (59, 60) reported that condition-specific HRQoL, as measured by the Q-TFA global score, was significantly higher, and problems related to prosthesis use were also significantly lower in the bone-anchored prosthesis cohort than those in the socket prosthesis cohort. One study (61) found no differences in these variables or the PCS and MCS in SF-36 or the Q-TFA global score. Out of the two cohort studies that also reported EQ-5D results (59, 60), one study (59) reported no significant difference in HRQoL between groups, whereas the other (60) showed a significant increase in the bone-anchored cohort than the socket cohort at 1-year follow-up. This increase could perhaps be due, in part, to a greater sample size (69 patients) in the latter study (60) than in the former (59), which had 39 patients, or because the individuals in the socket group in the former study (59) indicated that they were satisfied with their prostheses, whereas this was not controlled for in the latter study (60).
Improvements in mobility were reported widely, as evident by the significant improvements in the distance walked during the 2MWT at 1-year follow-up (58) and 6MWT at 1-year (51, 54–56, 58, 62) and 2-year (57) follow-ups and improvements in TUG at 1-year follow-up (51, 55, 56). Studies on press-fit implants more commonly used performance-based outcome measures that specifically measured mobility and function. Although observer-based mobility performance measures were not used or reported by the studies on screw-type implants (44–50), information on mobility and prosthesis usage in these studies based on the self-reported Q-TFA did show improvements. Prosthesis use as measured by Q-TFA prosthetic use score was reported to have increased significantly at 1-year (28, 55, 58), 2-year (44, 45), 5-year (46, 47, 52), and 10-year (50), but not at 15-year (49), follow-up. Improved perceived mobility (measured by PLUS-M), balance (measured by ABC), and functional capacity were also reported at 1-year follow-up (63), as were significant reductions in time to don and doff the prosthesis (62).
Out of the four cohort studies, three reported on the differences in mobility between cohorts of bone-anchored prosthesis and socket prosthesis users (53, 60, 61). Gailey et al. (53) and Welke et al. (61) reported no significant differences in mobility between the two groups, as measured by the 10 MWT (53), 6 MWT (61), and TUG (53, 61) or self-perceived mobility, as measured by PLUS-M (53). Gailey et al. (53) reported on a small sample size (22 patients, 11 in each group) and did not report on the mean duration since the OI surgery for those in the bone-anchored prosthesis group. Additionally, the selection of participants is a limitation in Welke et al. (61). Individuals in the socket group in this study reported a high level of functional mobility and are not comparable to those socket users who face significant mobility issues due to their socket and may go on to benefit from bone-anchored prosthesis. Overall, cohort studies that report on comparisons of bone-anchored prosthesis users with socket users should be taken with caution, as individuals who are successful prosthesis users with a socket prosthesis are generally not considered candidates for bone-anchored prostheses.
Four out of seventeen single-arm trials (51, 55, 62, 63) and three out of the four cohort studies (53, 59, 61) reported on external prosthetic components. In the single-arm trials, three (55, 62, 63) reported that participants were fit with the same external components with the bone-anchored implant that they used with their pre-intervention socket system. One single-arm trial (51) did not clearly report this. In the three cohort studies that included details of external prosthetic components, the authors reported that the types of components were similar in both groups (OI and socket).
The evidence suggests that quality of life, mobility, prosthesis use, and satisfaction with the prosthesis improve with bone-anchored implants compared to the patients’ condition as socket prosthesis users. However, socket prosthesis users who do not face significant challenges with their sockets and already have a higher degree of mobility may not benefit as much, even if they opt for bone-anchored implants for prosthesis fixation.
Information on complications and their time frame can be useful in informing clinical decision-making, planning, and informing health economic models. Moreover, 17 out of the 21 articles on outcomes also reported on complications faced by patients. Table 4 summarizes the adverse events and complications reported in these articles. Nine case series and one cohort study reported only on infectious and other serious complications but presented no other outcomes of interest. Table 5 shows the findings of these studies and the odds of complications (where available) by implant type. The most commonly reported complication is superficial (skin/soft tissue) infections that occur in all types of implants from as few as 11% (44) to as much as two-thirds (52) of the patients. These complications are usually managed with oral or intravenous (parenteral) antibiotics or surgical intervention (such as debridement) (36). Soft-tissue refashioning (28, 52, 54, 56–58, 62, 63) and stoma hypergranulation (52, 55, 63) are other complications often reported. Mechanical complications, including the breakage of external parts, also occurred in third (58) to half (49) of the patients treated with BAP and were reported to be managed by exchanging percutaneous implant parts as needed. Such mechanical complications have been reported to increase between 5 and 10 years after implantation (50). More serious complications, such as implant loosening, implant breakage, and implant failure (requiring removal), were reported and were rarer in individuals treated with press-fit implants (ILP or OPL). Implant loosening has been reported to occur more frequently in screw-type implants and in the first 5 years following implantation. Implant removal occurred in 8 out of 51 patients in the OPRA (screw-type) study cohort (50) and as few as 2 out of 39 patients who were fitted with the ILP (press-fit type) implants (52). In the OPRA study cohort, half of the implant removals occurred in the first 5 years following implantation and the other half between 5 and 10 years (50).
Deeper infections affecting the residual femur are rare and appear more prevalent in screw-type implants, although it should be noted that the duration of follow-up is generally longer for these studies than for press-fit implants. The risk of osteomyelitis (deep infection of the bone) was studied in individuals with screw-type OPRA implants, and the 10-year cumulative risk was 20% (67). There was no significant association between osteomyelitis and advanced age, being overweight, patient's sex, or smoking. However, in a retrospective study of complications with the press-fit ILP implant, Al Muderis et al. (69) reported a threefold increase in the risk of mild infections in persons with overweight or obesity, a sixfold increase in the risk of severe infection in female patients, and a sevenfold increase in recurrent infections in patients who were smokers. The increased risk of soft-tissue infections in persons with obesity and in females who were treated with press-fit implants was also reported by another study (74).
Spontaneous periprosthetic fractures were reported to have not occurred with the OPL (71) in a retrospective case series of 347 patients. Periprosthetic fractures due to falls were rare and occurred in as low as 6.3% of patients treated with the OPL implant; however, the follow-up duration of these studies was short (1 or 2 years). The most common cause for a periprosthetic fracture is falling, and the most common location is close to the proximal tip of the implant (71). Females have approximately a fourfold greater increase in the risk of periprosthetic fractures; however, time from amputation to OI surgery, age at OI surgery, or bone density were not reported to be associated with increased risk for fractures (71). Periprosthetic fractures were reported to be managed successfully in most cases by uniting the bone with dynamic hip screws or reconstruction plates (71, 75) and have been reported to not worsen outcomes (75).
One study specifically examined pain and pain management for up to 3 years after OI surgery (72). This was conducted in a group of seven patients with military service experience who experienced severe complex trauma-related injuries and underwent bilateral transfemoral OI surgeries with the press-fit OPL implant. In this group, five patients were required to take analgesic at 6 weeks postsurgery, three needed long-term pain management with opiates (out of which only one continued opiate use at 14 months postsurgery), and one had persistent pain after discharge. A progressive decrease in the use of regular analgesics was reported.
The cumulative survival rate for the ILP implant after 9 years was reported to be 78% in a retrospective case series of 58 patients (70). In this group of 58 patients, approximately 35% experienced implant failures either due to intramedullary stem failure (12%) or mechanical complications, such as dual-cone adapter breakage (23%). Those who experienced stem failure underwent revision surgery to have a larger-diameter OPL stem implanted, and those who experienced mechanical complications were revised in an outpatient setting without anesthesia. It was determined that common factors that lead to implant/stem failure are the initial implantation of a smaller diameter stem or the number of infectious events in a patient. Improvements to the design of the implant have been credited to the reduction in unplanned interventions and structural failure requiring the removal of the implant (68).
A retrospective analysis of mortality in a cohort of 485 patients who received the OPL implant reported that no deaths occurred intraoperatively or during inpatient recuperation or recovery after OI surgery; however, 19 patients in this cohort died within 5 years after the OI surgery (73). Moreover, 17 out of these 19 died due to causes unrelated to OI surgery, and 2 died of infectious complications originating at the stoma site. One of these two deaths occurred between 2 and 5 years following the OI surgery and the other over 5 years after the surgery. Notably, among the seventeen patients in this cohort, three died of suicide, highlighting the need for mental health evaluation and support in persons with amputation. Although not included in the data extraction, there was a case report of a patient who died during the EEP surgical implantation due to a pulmonary embolism, which could have been related to a pre-existing risk of deep vein thrombosis and wheelchair immobilization. This case report recommended that additional preventive measures such as preoperative scoring systems and, in exceptional cases, using an inferior vena cava filter should be considered in patients with a high risk of developing venous thromboembolism.
Two research studies reported on the lived experiences of patients who received screw-type implants. Specific patient quotes from the qualitative studies to elucidate changes and challenges in the lives of the patients due to bone-anchored prostheses are included in Table 6. One study included three persons with upper-limb amputation (two transhumeral and one transradial) and ten persons with transfemoral amputations who used a bone-anchored prosthesis (76). The users described living with a bone-anchored prosthesis as a revolutionary change beyond functional improvements. Some users also described embodying the prosthetic leg as a part of them. The seven transfemoral BAP users in the other study (77) described the feeling of being whole again and described improvements in aspects of social participation, which greatly improved their quality of life. Both studies mentioned some challenges of being a bone-anchored prosthesis user, specifically due to the fear of infections, falling, and breaking the implant.
Five studies addressing the health economic impacts associated with bone-anchored implants for transfemoral prosthetic fixation were included. Table 7 outlines the study characteristics, main findings, and limitations. Two were cost-comparison studies (78, 82) and three were cost-utility studies (79, 81, 83). Two studies were based on the screw -type implant (78, 79), one on the OPL (81), and both did not specify the type of implant (82, 83). Another health economic evaluation was found but excluded as it combined the data for transfemoral and transtibial levels of BAP and separate data for transfemoral users was not available (84). To explore the cost-effectiveness of transfemoral BAP, it is essential that this information be analyzed separately.
Handford et al. (81) reported the cost of bilateral transfemoral OI to be £123,008 and unilateral to be £81,008. Haggstrom et al. (78) reported fewer visits to the prosthetist by those who use BAP vs. socket-suspended prostheses. Despite this, they reported that the costs of prosthetic materials and components were higher for BAP, which made the annual mean costs for bone-anchored and socket prostheses similar. The results of the three cost-utility studies vary greatly due to their methodological approaches. Hansson et al. (79) reported an ICER of €83,374 (in 2009 Euros) per QALY gained by bone-anchored prosthesis users over socket users. Frossard et al. (83) reported an AUD 16,632/QALY gained, and Handford et al. (81) reported £40,040.92/QALY 6 years after the OI surgery due to a steady increase in the patient-reported health utility value (HUV) in the 6 years postsurgery. The cost data in Frossard et al. (83) was based on a 6-year horizon for the press-fit implant, but the utility estimates were based on 2-year follow-up data with screw-type implant (44, 45) and multiplied over the 6 years to obtain differences in QALYs. Handford et al. (81) also presented the results of a subgroup analysis of patients grouped based on preoperative EQ-5D HUV being less or more than 0.60. The mean preoperative HUV of the group that had an HUV of <0.60 was 0.41, which reached 0.77 by 5 years and yielded a cost/QALY of £25,334.87. This, they reported, met the cost-effectiveness threshold of £30,000/QALY advised by NICE (85). The cost continued to fall in this group to neutrality with the comparator at 10.5 years. They concluded that those who perform poorly with socket prosthesis (typically those with an HUV of <0.60) are likely those who face significant challenges in walking or do not walk at all. Osseointegration offers the greatest benefit to these individuals as they continually show improvements in HUV and cost-effectiveness within 5 years. Conversely, in those with a preoperative HUV of >0.60, the gain in HUV and cost-effectiveness is less compelling.
Neither of the two cost-utility studies based on modeling (79, 83) included discounting of costs or outcomes. There is a notable range of prescribed rates for discounting costs and outcomes based on countries or regions (86–88), but these studies did not address discounting. As the failure of bone-anchored implants has been reported to be rare, the implants are estimated to maintain their effectiveness throughout the lifetime of an individual. Contrary to this, the majority of costs occur in the first year postsurgery. Due to this, the absence of discounting could potentially artificially inflate the reported ICER. Hansson et al. (79) presented alternative scenarios based on anticipated declines in utility in users of socket-suspension systems, as worsening of symptoms is expected and could cause a continuing decline in HRQoL in these users. This resulted in the cost per QALY gained of €37,020, €24,662, and €18,952, for a 1%, 2%, and 3% decline, respectively, over 20 years. Lastly, none of these studies take a societal perspective on costs and outcomes, which can be complex to acquire but provide a more holistic picture of the economic impact of bone-anchored prosthesis use.
Overall, bone-anchored implants that enable the direct attachment of prosthetic devices for individuals with transfemoral amputation who have failed conventional socket-suspension systems show promising results. The similarities in the patient selection criteria and the improved outcomes across the included studies add to the credibility of the findings on clinical efficacy. The evidence on clinical efficacy available on different implant types and on shorter (1- or 2-year) and longer (5-, 10-, and 15-year) follow-ups indicates that those who have been fitted with these implants consistently report improvements in quality of life, mobility, satisfaction with the prosthesis, and an overall improvement in situation as a person with an amputation. At 15-year postsurgery follow-up, approximately 64% of patients mentioned that osseointegration improved their overall situation as a person with amputation (49). It is noteworthy that studies that presented interim 2-year and 5-year follow-up data (46, 47) revealed no significant differences in HRQoL (measured by SF-36 domains and Q-TFA subscales) between these two time points. Similarly, there were no significant differences in these measures between 5- and 10-year follow-ups (50). These findings suggest that most advantages of bone-anchored prostheses can be expected within the first 2 years and are maintained beyond that. It is interesting to note that the mobility improvements may contribute to the concomitant improvements in patient-reported health-related quality of life. Mobility has been previously reported to be strongly positively correlated with general satisfaction and HRQoL in individuals with lower-limb prostheses (89). Improvements in mobility are further supported by other studies on BAP users who reported a higher daily step count and daily stepping time when assessing mobility in daily activities, i.e., not in a controlled lab setting (90). In addition, even those with bilateral transfemoral BAP after on average 7 years reported improved mobility (91).
It should be noted that although an improvement in quality of life has been reported, this often does not translate into an improvement in the mental health of BAP users. Only one study (57) reported an improvement in the mental component score of the SF-36, and none employed an instrument specifically designed to address changes in mental health. Mental health is a known challenge within the amputation and prosthesis-user communities (92–94). Depression has been reported to affect as many as one-third of persons with lower-limb amputation (92). More research is required to explore the mental health changes that accompany BAP use, as adequate evaluation and treatment of mental health concerns in this population may improve HRQoL.
Soft tissue infection is the most common complication consistently reported across studies, which is typically managed conservatively. The incidence of hypergranulation and the need for refashioning of the stoma or for soft tissue redundancy suggest that continuous efforts are required to improve and track soft tissue management. Of utmost concern is to continually track serious complications requiring implant removal. Survival rates of implants in the literature ranged from 78% to 99% for studies using press-fit implants (28, 52, 62, 70) and 72% to 92% for studies using screw-fit implants (45–47, 49). There seems to be an equal probability of implant loss for screw-type implants in the first 5 years and the subsequent 5 years in the one study that examined implant loss (50), so a longer-term tracking of these complications is crucial. Mechanical complications are common across implant types but were often reported to be managed by replacing external parts as needed. The incidences of mechanical complications increase between 5 and 10 years after implantation (50), and the cost of replacing external parts may lead to an increase in prosthetic care expenses over time. It has also been reported that at 5- and 10-year follow-ups, mechanical complications tend to be significantly correlated with prosthetic mobility or the occurrence of deep infections. Improved mobility that BAP offers to prosthesis users may therefore inadvertently contribute to mechanical complications (49). The statistics on mechanical complications of external parts need to be considered in the context of the expected longevity of any mechanical prosthesis component, which also needs periodic replacement in active socket prosthesis users.
Patient-reported experiences in the literature are based on screw-type implants and generally positive. In the future, additional qualitative studies on individuals who receive press-fit implants may be beneficial to enable the comparisons of patient perspectives and experiences. In addition, it would be beneficial to explore the changes and challenges that a patient experiences preoperatively and after receiving a BAP. The use of longitudinal qualitative research methods (95, 96) may be well positioned to understand the issues that socket prosthesis users experience and to articulate the changes that they experience when they transition to bone-anchored prosthesis.
The two cost-comparison studies (78, 82) have limited applicability for decision-making on increasing the availability of BAP. Cost-analysis or cost-comparison studies are considered appropriate when the outcomes of the intervention and the comparator are identical (97). It is evident from the information presented here that the outcomes of the socket-suspension and bone-anchored prostheses are not identical. The reports based on pre-/post-study designs illustrate that quality of life or mobility often changes when a previous socket-suspension system user becomes a bone-anchored prosthesis user. However, these two studies present useful information on some of the costs that are considered in the health economic evaluation of this technology.
Economic models/frameworks for evaluating costs and health outcomes differed across studies. The two cost-comparison studies (78, 82) and one of the cost-utility studies (83) did not include the costs of the bone-anchored implant, surgeries, hospital stay, postsurgical follow-up, and rehabilitation or the possible costs of dealing with complications. The results of these studies have limited usefulness and generalizability because of the narrow frame of costs (only prosthetic care costs) included for analysis. Without accounting for the upfront costs associated with bone-anchored implants (such as costs of the surgery, hospital stay, and postsurgical follow-up) and appropriate ongoing costs (such as those related to prosthetic care or dealing with complications), the results from these studies should be interpreted with caution. Not accounting for these costs likely led to an underestimation of the ICER. Hansson et al. (79) included these costs associated with bone-anchored implants, but they did not include the costs of many common complications, and their Markov model did not include many tunnel states in which patients often find themselves during their journey toward becoming BAP users. Handford et al. (81) included a broad list of costs in their analysis. The outcomes measured varied from the number of visits to a prosthetist (78) to utility values based on SF-6D (79) and EQ-5D (81).
Overall, the results from these health economic studies are mixed and complex to interpret. This necessitates future studies in this field to have health economics as a forethought and ideally be based on prospective real-world administrative data over a reasonable time horizon (at least 5 years). This may become increasingly feasible in the future with the growing adoption of electronic medical records. To acquire a more realistic picture of the cost-effectiveness of bone-anchored implants, the costs considered for analysis should include the cost of the implant, surgery, postsurgical care, rehabilitation, regular follow-up, and management of complications and should be compared against the costs borne by the system to service the needs of the socket-suspension system users, including their need for prosthetic services, medical follow-up, complication management, and surgical revisions. The HRQoL outcomes should be collected prospectively and should be generic to allow the calculation of utility values. The comparison of costs and outcomes should ideally be made with the patients’ pre-intervention state of socket prosthesis use, but in the absence of the availability of this information, to a control group matched on several parameters including similar functional mobility restrictions and similar types of prosthetic components. If modeling is deemed a more suitable tool to assist in decision-making, then it should account for many states to more accurately reflect the typical patient trajectory.
Nonetheless, overall, it appears that bone-anchored prostheses involve a higher upfront cost to the healthcare system but yield a longer-term gain, as evident by the improvements in health-related outcomes and reduced problems due to socket systems. Other groups, such as Ontario Health in Canada, concluded in their health technology assessment that bone-anchored implants are a cost-effective intervention (98). However, it is possible that this intervention is mostly cost-effective for those who stand to gain the most out of it, i.e., those who face significant challenges due to socket-suspension systems, and not suitable as primary treatment for prosthetic fixation.
The technology has evolved since the early 1990s with consistent revisions in design and improvements in outcomes for patients. Screw-type implants have longer follow-ups and have been around for a longer time, and the press-fit implants have higher reported case numbers and more comprehensive tracking of outcomes and complications. The press-fit implants have demonstrated a reduced risk of complications with concomitant improvements in quality of life and mobility. The latest iterations of both types of implants can also be used with individuals with a long or short length of the residual femur (28). The surgery can be done as a one-stage procedure that may reduce the burden on the patient and the healthcare system. A recent review presents evidence in favor of the one-stage approach owing to the lower incidence of postsurgical complications with this approach (99). Some work has been done to develop a comprehensive and systematic framework for tracking complications (69, 100) and a systematic outcomes-tracking framework (101, 102); however, not all centers follow the same guidelines for reporting. Future studies in this field can also further improve the quality of evidence by reporting on potential confounders (such as external prosthetic components, pre-existing pain, residual limb length, or bone mineral density) and addressing them by conducting and reporting subgroup analyses or other appropriate statistical tools, if statistical power allows.
As the number of cases increases across centers worldwide, there is also an opportunity to further explore changes in the mental health of prosthesis users and the factors/experiences contributing to changes in the perceptions of patients about their health-related quality of life. Well-designed mixed methods studies (103, 104) could address this need and contextualize the perceived changes in quality of life with patients’ experiences and challenges in their everyday lives. Future research on the lived experiences of patients and their caregivers and the impact of bone-anchored prostheses on productivity and vocational/employment situations will lead to a richer and more wholesome understanding of the change in the lives of patients that bone-anchored prostheses appear to promise.
The studies included in this review present considerable variability in follow-up duration, the type of variables on which data are collected, and the reported outcomes. The resultant inability to do a meta-analysis/synthesis may be perceived as a challenge for policymakers when deciding on the value of providing this technology; however, there is evidence that BAP seems to be a worthwhile alternative for those who are experiencing recurring issues with their socket prosthesis and can have a long-lasting impact on the individual's quality of life, function, and participation in society. As the body of evidence on clinical efficacy and complications evolves in this area, it would be prudent to adopt a standard suite of outcome measures and complication tracking at regular time points and for a longer term and to establish data reporting standards by consensus within the various centers around the globe offering this intervention. This will enable comparisons of outcomes across centers worldwide and across implant types. With such data in the future, a meta-analysis may also become feasible. When policymakers and regulatory bodies approve or implement this technology as a funded alternative intervention to socket prostheses for individuals experiencing recurring issues with their socket prostheses, it is essential that well-designed and planned cost-utility studies be conducted.
There were a few limitations of this review, primarily due to the types of study designs and reporting of information in the included studies. Despite similar measures being reported in studies with a pre-/post-design, a meta-analysis was not feasible due to the varying lengths of follow-up and the variability in how results were reported in the literature. Some articles only reported the statistical significance of the difference between the pre- and post-intervention but not actual values (47, 49, 56, 60), whereas others reported median scores and not mean scores (52, 55, 57). One of the issues that may impact the reported health-related quality of life was the persistence of phantom limb pain. This issue could not be fully explored in this review as this phenomenon is inconsistently reported in the included literature. Other potential confounders, such as residual limb length and type of prosthetic components, are insufficiently reported to allow accurate analysis of their potential impact on outcomes. It should be noted that this review excluded papers on gait parameters as this was recently reviewed (105) and the relationship between gait parameters and clinical outcomes needs to be further examined. Lastly, two of the included studies were reported to have been conducted as a clinical trial (48, 62), and one of these is under regulatory oversight by the FDA (62). These studies may be subject to different obligations to report outcomes and adverse events; however, we assessed their quality and risks of bias using appropriate tools.
Overall, based on the information available presently, the clinical efficacy of bone-anchored prostheses is well established as hundreds of cases have been performed worldwide with beneficial outcomes for patients and complications being managed effectively. Patients also report positive changes in their lived experience. The evidence points to the cost-effectiveness of this technology for those who suffer poor outcomes with standard-of-care socket prostheses, although further work is needed to collect sufficient data for rigorous health economic analysis. Standardizing outcome tracking would help with synthesizing evidence across centers. This paper presents a single resource on data collected in this population that can be used for decision-making on the implementation of BAP for transfemoral amputation.
The original contributions presented in the study are included in the article/Supplementary Material; further inquiries can be directed to the corresponding authors.
MR: conceptualization, funding acquisition, investigation, methodology, project administration, validation, visualization, writing – original draft, writing – review and editing. TS: investigation, methodology, supervision, validation, writing – review and editing. JR: validation, writing – review and editing. AJ: validation, writing – review and editing. JH: conceptualization, funding acquisition, methodology, supervision, validation, writing – review and editing.
The authors declare financial support was received for the research, authorship, and/or publication of this article.
MR is supported by grants from the University Hospital Foundation (RES0046327) and the Glenrose Rehabilitation Hospital Foundation (RES0049057), both of which are based in Edmonton, AB, Canada.
The authors are grateful to Becky Skidmore (information specialist consultant, Ottawa Hospital Research Institute) for carrying out the literature search for this systematic review.
The authors declare that the research was conducted in the absence of any commercial or financial relationships that could be construed as a potential conflict of interest.
All claims expressed in this article are solely those of the authors and do not necessarily represent those of their affiliated organizations, or those of the publisher, the editors and the reviewers. Any product that may be evaluated in this article, or claim that may be made by its manufacturer, is not guaranteed or endorsed by the publisher.
The Supplementary Material for this article can be found online at: https://www.frontiersin.org/articles/10.3389/fresc.2024.1336042/full#supplementary-material
1. Penn-Barwell JG. Outcomes in lower limb amputation following trauma: a systematic review and meta-analysis. Injury. (2011) 42(12):1474–9. doi: 10.1016/j.injury.2011.07.005
2. Ephraim PL, Wegener ST, MacKenzie EJ, Dillingham TR, Pezzin LE. Phantom pain, residual limb pain, and back pain in amputees: results of a national survey. Arch Phys Med Rehabil. (2005) 86(10):1910–9. doi: 10.1016/j.apmr.2005.03.031
3. de Godoy JMP, Braile DM, Buzatto SHG, Longo O, Fontes OA. Quality of life after amputation. Psychol Health Med. (2002) 7(4):397–400. doi: 10.1080/1354850021000015212
4. Sinha R, Van Den Heuvel WJA. A systematic literature review of quality of life in lower limb amputees. Disabil Rehabil. (2011) 33(11):883–99. doi: 10.3109/09638288.2010.514646
5. Horgan O, MacLachlan M. Psychosocial adjustment to lower-limb amputation: a review. Disabil Rehabil. (2004) 26(14–15):837–50. doi: 10.1080/09638280410001708869
6. Senra H, Oliveira RA, Leal I, Vieira C. Beyond the body image: a qualitative study on how adults experience lower limb amputation. Clin Rehabil. (2011) 26(2):180–91. doi: 10.1177/0269215511410731
7. Rybarczyk B, Edwards R, Behel J. Diversity in adjustment to a leg amputation: case illustrations of common themes. Disabil Rehabil. (2004) 26(14–15):944–53. doi: 10.1080/09638280410001708986
8. Singh R, Ripley D, Pentland B, Todd I, Hunter J, Hutton L, et al. Depression and anxiety symptoms after lower limb amputation: the rise and fall. Clin Rehabil. (2009) 23(3):281–6. doi: 10.1177/0269215508094710
9. Rommers GM, Vos LD, Groothoff JW, Eisma WH. Clinical rehabilitation of the amputee: a retrospective study. Prosthet Orthot Int. (1996) 20(2):72–8. doi: 10.3109/03093649609164422
10. Legro MW, Reiber G, del Aguila M, Ajax MJ, Boone DA, Larsen JA, et al. Issues of importance reported by persons with lower limb amputations and prostheses. J Rehabil Res Dev. (1999) 36(3):155–63. PMID: 10659798.10659798
11. Dillingham TR, Pezzin LE, MacKenzie EJ, Burgess AR. Use and satisfaction with prosthetic devices among persons with trauma-related amputations: a long-term outcome study. Am J Phys Med Rehabil. (2001) 80:563–71. doi: 10.1097/00002060-200108000-00003
12. Hagberg K, Brånemark R. Consequences of non-vascular trans-femoral amputation: a survey of quality of life, prosthetic use and problems. Prosthet Orthot Int. (2001) 25:186–94. doi: 10.1080/03093640108726601
13. Pezzin LE, Dillingham TR, MacKenzie EJ, Ephraim P, Rossbach P. Use and satisfaction with prosthetic limb devices and related services. Arch Phys Med Rehabil. (2004) 85:723–9. doi: 10.1016/j.apmr.2003.06.002
14. Koc E, Tunca M, Akar A, Erbil AH, Demiralp B, Arca E. Skin problems in amputees: a descriptive study. Int J Dermatol. (2008) 47(5):463–6. doi: 10.1111/j.1365-4632.2008.03604.x
15. Sanders JE, Fatone S. Residual limb volume change: systematic review of measurement and management. J Rehabil Res Dev. (2011) 48(8):949–86. doi: 10.1682/jrrd.2010.09.0189
16. Butler K, Bowen C, Hughes AM, Torah R, Ayala I, Tudor J, et al. A systematic review of the key factors affecting tissue viability and rehabilitation outcomes of the residual limb in lower extremity traumatic amputees. J Tissue Viability. (2014) 23(3):81–93. doi: 10.1016/j.jtv.2014.08.002
17. Dudek NL, Marks MB, Marshall SC, Chardon JP. Dermatologic conditions associated with use of a lower-extremity prosthesis. Arch Phys Med Rehabil. (2005) 86(4):659–63. doi: 10.1016/j.apmr.2004.09.003
18. Lyon CC, Kulkarni J, Zimerson E, Van Ross E, Beck MH. Skin disorders in amputees. J Am Acad Dermatol. (2000) 42(3):501–7. doi: 10.1016/s0190-9622(00)90227-5
19. Meulenbelt HE, Geertzen JH, Jonkman MF, Dijkstra PU. Determinants of skin problems of the stump in lower-limb amputees. Arch Phys Med Rehabil. (2009) 90(1):74–81. doi: 10.1016/j.apmr.2008.07.015
20. Paternò L, Ibrahimi M, Gruppioni E, Menciassi A, Ricotti L. Sockets for limb prostheses: a review of existing technologies and open challenges. IEEE Trans Biomed Eng. (2018) 65(9):1996–2010. doi: 10.1109/TBME.2017.2775100
21. Knight AD, Dearth CL, Hendershot BD. Deleterious musculoskeletal conditions secondary to lower limb loss: considerations for prosthesis-related factors. Adv Wound Care (New Rochelle). (2021) 10(12):671–84. doi: 10.1089/wound.2019.1079
22. Brånemark PI, Hansson BO, Adell R, Breine U, Lindstrom J, Hallen O, et al. Osseointegrated implants in the treatment of the edentulous jaw. Experience from a 10-year period. Scand J Plast Reconstr Surg Suppl. (1977) 16:1–132. PMID: 356184.
23. Worthington P. History, development, and current status of osseointegration as revealed by experience in craniomaxillofacial surgery. In: Brånemark PI, Rydevik BL, Skalak R, editors. Osseointegration in Skeletal Reconstruction and Joint Replacement. Carol Stream, IL: Quintessence Publishing Co. (1997). 25–44.
24. Hoellwarth JS, Tetsworth K, Akhtar MA, Al Muderis M. The clinical history and basic science origins of transcutaneous osseointegration for amputees. Adv Orthop. (2022) 2022:1–14. doi: 10.1155/2022/7960559
25. Hoellwarth JS, Tetsworth K, Rozbruch SR, Handal MB, Coughlan A, Al Muderis M. Osseointegration for amputees: current implants, techniques, and future directions. JBJS Rev. (2020) 8(3):e0043. doi: 10.2106/JBJS.RVW.19.00043
26. Eriksson E, Brånemark PI. Osseointegration from the perspective of the plastic surgeon. Plast Reconstr Surg. (1994) 93(3):626–37. doi: 10.1097/00006534-199493030-00033
27. Ranker A, Örgel M, Beck JP, Krettek C, Aschoff HH. Transcutaneous osseointegrated prosthetic systems (TOPS) for transfemoral amputees—a six-year retrospective analysis of the latest prosthetic design in Germany. Rehabilitation (Stuttg). (2020) 59(6):357–65. doi: 10.1055/a-1223-3205
28. Atallah R, van de Meent H, Verhamme L, Frölke JP, Leijendekkers RA. Safety, prosthesis wearing time and health-related quality of life of lower extremity bone-anchored prostheses using a press-fit titanium osseointegration implant: a prospective one-year follow-up cohort study. PLoS One. (2020) 15(3):e0230027. doi: 10.1371/journal.pone.0230027
29. Hoyt BW, Walsh SA, Forsberg JA. Osseointegrated prostheses for the rehabilitation of amputees (OPRA): results and clinical perspective. Expert Rev Med Devices. (2020) 17(1):17–25. doi: 10.1080/17434440.2020.1704623
30. Tropf JG, Potter BK. Osseointegration for amputees: current state of direct skeletal attachment of prostheses. Orthoplast Surg. (2023) 12:20–8. doi: 10.1016/j.orthop.2023.05.004
31. ClinicalTrials.gov. Intraosseous Transcutaneous Amputation Prosthesis (ITAP). Identifier: NCT02491424. (2018). Available online at: https://clinicaltrials.gov/study/NCT02491424 (Accessed April 20, 2021).
32. Hoellwarth JS, Tetsworth K, Akhtar MA, Al Muderis M. Transcutaneous osseointegration for amputees. Bone Joint Res. (2021) 10(10):690–2. doi: 10.1302/2046-3758.1010.BJR-2021-0235.R2
33. van Eck CF, McGough RL. Clinical outcome of osseointegrated prostheses for lower extremity amputations: a systematic review of the literature. Curr Orthop Pract. (2015) 26(4):349–57. doi: 10.1097/BCO.0000000000000248
34. Hebert JS, Rehani M, Stiegelmar R. Osseointegration for lower-limb amputation: a systematic review of clinical outcomes. JBJS Rev. (2017) 5(10):e10. doi: 10.2106/JBJS.RVW.17.00037
35. Kunutsor SK, Gillatt D, Blom AW. Systematic review of the safety and efficacy of osseointegration prosthesis after limb amputation. Br J Surg. (2018) 105(13):1731–41. doi: 10.1002/bjs.11005
36. Tillander J, Hagberg K, Hagberg L, Brånemark R. Osseointegrated titanium implants for limb prostheses attachments: infectious complications. Clin Orthop Relat Res. (2010) 468(10):2781–8. doi: 10.1007/s11999-010-1370-0
37. Atallah R, Leijendekkers RA, Hoogeboom TJ, Frölke JP. Complications of bone-anchored prostheses for individuals with an extremity amputation: a systematic review. PLoS One. (2018) 13(8):e0201821. doi: 10.1371/journal.pone.0201821
38. Page MJ, McKenzie JE, Bossuyt PM, Boutron I, Hoffmann TC, Mulrow CD, et al. The PRISMA 2020 statement: an updated guideline for reporting systematic reviews. Br Med J. (2021) 372:n71. doi: 10.1136/bmj.n71
39. Moher D, Shamseer L, Clarke M, Ghersi D, Liberati A, Petticrew M, et al. Preferred reporting items for systematic review and meta-analysis protocols (PRISMA-P) 2015 statement. Syst Rev. (2015) 4(1):1. doi: 10.1186/2046-4053-4-1
40. Rehani M, Stafinski TD, Round J, Jones CA, Hebert JS. Bone-anchored Implants for Direct Attachment of External Prostheses for Persons with Transfemoral Amputation: Protocol for a Systematic Review. (2021). Available online at: https://era.library.ualberta.ca/items/64c34b8d-333f-40d7-9fc2-43da70cea6e5 (Accessed October 15, 2021).
41. Veritas Health Innovation. Covidence Systematic Review Software. Melbourne, Australia. Available online at: www.covidence.org (Accessed March 21, 2021).
42. Howick J, Chalmers I, Glasziou P, Greenhalgh T, Heneghan C, Liberati A, et al. Explanation of the 2011 Oxford Centre for Evidence-Based Medicine (OCEBM) Levels of Evidence (Background Document). (2011). Available online at: https://www.cebm.ox.ac.uk/resources/levels-of-evidence/ocebm-levels-of-evidence (Accessed April 15, 2021).
43. Sterne JA, Hernán MA, Reeves BC, Savović J, Berkman ND, Viswanathan M, et al. ROBINS-I: a tool for assessing risk of bias in non-randomised studies of interventions. Br Med J. (2016) 355:i4919. doi: 10.1136/bmj.i4919
44. Hagberg K, Brånemark R, Gunterberg B, Rydevik B. Osseointegrated trans-femoral amputation prostheses: prospective results of general and condition-specific quality of life at 2-year follow-up. Prosthet Orthot Int. (2008) 32(1):29–41. doi: 10.1080/03093640701553922
45. Brånemark R, Berlin Ö, Hagberg K, Bergh P, Gunterberg B, Rydevik B. A novel osseointegrated percutaneous prosthetic system for the treatment of patients with transfemoral amputation. Bone Joint J. (2014) 96-B:106–13. doi: 10.1302/0301-620X.96B1.31905
46. Brånemark RP, Hagberg K, Kulbacka-Ortiz K, Berlin Ö, Rydevik B. Osseointegrated percutaneous prosthetic system for the treatment of patients with transfemoral amputation: a prospective five-year follow-up of patient-reported outcomes and complications. J Am Acad Orthop Surg. (2019) 27(16):e743–51. doi: 10.5435/JAAOS-D-17-00621
47. Matthews DJ, Arastu M, Uden M, Sullivan JP, Bolsakova K, Robinson K, et al. UK trial of the osseointegrated prosthesis for the rehabilitation for amputees: 1995–2018. Prosthet Orthot Int. (2019) 43(1):112–22. doi: 10.1177/0309364618791616
48. Zaid MB, Wustrack RL, Garibaldi M, Geiger E, Andaya V, O'Donnell RJ, et al. Prospective study of percutaneous bone-anchored implants in transfemoral amputees: brain-machine platform technology for external prosthetic control and feedback. 9th International IEEE/EMBS Conference on Neural Engineering (NER); San Francisco, CA, USA (2019). p. 13–6. doi: 10.1109/NER.2019.8716952
49. Hagberg K, Ghassemi Jahani SA, Kulbacka-Ortiz K, Thomsen P, Malchau H, Reinholdt C. A 15-year follow-up of transfemoral amputees with bone-anchored transcutaneous prostheses. Bone Joint J. (2020) 102-B(1):55–63. doi: 10.1302/0301-620X.102B1.BJJ-2019-0611.R1
50. Hagberg K, Ghasemi Jahani SA, Omar O, Thomsen P. Osseointegrated prostheses for the rehabilitation of patients with transfemoral amputations: a prospective ten-year cohort study of patient-reported outcomes and complications. J Orthop Translat. (2023) 38:56–64. doi: 10.1016/j.jot.2022.09.004
51. Van de Meent H, Hopman MT, Frölke JP. Walking ability and quality of life in subjects with transfemoral amputation: a comparison of osseointegration with socket prostheses. Arch Phys Med Rehabil. (2013) 94(11):2174–8. doi: 10.1016/j.apmr.2013.05.020
52. Reetz D, Atallah R, Mohamed J, van de Meent H, Frolke JPM, Leijendekkers R. Safety and performance of bone-anchored prostheses in persons with a transfemoral amputation: a 5-year follow-up study. J Bone Joint Surg Am. (2020) 102(15):1329–35. doi: 10.2106/JBJS.19.01169
53. Gailey RS, Kristal A, Al Muderis M, Lučarević J, Clemens S, Applegate EB, et al. Comparison of prosthetic mobility and balance in transfemoral amputees with bone-anchored prosthesis vs. Socket prosthesis. Prosthet Orthot Int. (2023) 47(2):130–6. doi: 10.1097/PXR.0000000000000189
54. Al Muderis M, Tetsworth K, Khemka A, Wilmot S, Bosley B, Lord SJ, et al. The osseointegrated group of Australia Accelerated Protocol (OGAAP-1) for two-stage osseointegrated reconstruction of amputated limbs. Bone Joint J. (2016) 98-B(7):952–60. doi: 10.1302/0301-620x.98b7.37547
55. Leijendekkers RA, van Hinte G, Frolke JP, van de Meent H, Atsma F, Nijhuis-van der Sanden MW, et al. Functional performance and safety of bone-anchored prostheses in persons with a transfemoral or transtibial amputation: a prospective one-year follow-up cohort study. Clin Rehabil. (2019) 33(3):450–64. doi: 10.1177/0269215518815215
56. Al Muderis M, Lu W, Li JJ. Osseointegrated prosthetic limb for the treatment of lower limb amputations: experience and outcomes. Unfallchirurg. (2017) 120(4):306–11. doi: 10.1007/s00113-016-0296-8
57. McMenemy L, Ramasamy A, Sherman K, Mistlin A, Phillip R, Evriviades D, et al. Direct skeletal fixation in bilateral above knee amputees following blast: 2 year follow up results from the initial cohort of UK service personnel. Injury. (2020) 51(3):735–43. doi: 10.1016/j.injury.2020.01.006
58. Reif TJ, Khabyeh-Hasbani N, Jaime KM, Sheridan GA, Otterburn DM, Rozbruch SR. Early experience with femoral and tibial bone-anchored osseointegration prostheses. JB JS Open Access. (2021) 6(3):e21.00072. doi: 10.2106/JBJS.OA.21.00072
59. Pospiech PT, Wendlandt R, Aschoff HH, Ziegert S, Schulz AP. Quality of life of persons with transfemoral amputation: comparison of socket prostheses and osseointegrated prostheses. Prosthet Orthot Int. (2021) 45(1):20–5. doi: 10.1177/0309364620948649
60. Örgel M, Schwarze F, Graulich T, Krettek C, Weidemann F, Aschoff HH, et al. Comparison of functional outcome and patient satisfaction between patients with socket prosthesis and patients treated with transcutaneous osseointegrated prosthetic systems (TOPS) after transfemoral amputation. Eur J Trauma Emerg Surg. (2022) 48(6):4867–76. doi: 10.1007/s00068-022-02018-6
61. Welke B, Hurschler C, Schwarze M, Jakubowitz E, Aschoff HH, Örgel M. Comparison of conventional socket attachment and bone-anchored prosthesis for persons living with transfemoral amputation—mobility and quality of life. Clin Biomech (Bristol, Avon). (2023) 105:105954. doi: 10.1016/j.clinbiomech.2023.105954
62. Sinclair S, Beck JP, Webster J, Agarwal J, Gillespie B, Stevens P, et al. The first FDA approved early feasibility study of a novel percutaneous bone anchored prosthesis for transfemoral amputees: a prospective 1-year follow-up cohort study. Arch Phys Med Rehabil. (2022) 103(11):2092–104. doi: 10.1016/j.apmr.2022.06.008
63. Davis-Wilson HC, Christiansen CL, Gaffney BMM, Lev G, Enabulele E, Stoneback JW. Improvements in disability and function in people with lower-limb amputation one year after prosthesis osseointegration. Prosthet Orthot Int. (2023) 47(4):343–9. doi: 10.1097/PXR.0000000000000200
64. Hagberg K, Brånemark R, Hägg O. Questionnaire for persons with a transfemoral amputation (Q-TFA): initial validity and reliability of a new outcome measure. J Rehabil Res Dev. (2004) 41(5):695–706. doi: 10.1682/JRRD.2003.11.0167
65. Gailey RS, Roach KE, Applegate EB, Cho B, Cunniffe B, Licht S, et al. The amputee mobility predictor: an instrument to assess determinants of the lower-limb amputee’s ability to ambulate. Arch Phys Med Rehabil. (2002) 83(5):613–27. doi: 10.1053/apmr.2002.32309
66. Resnik L, Borgia M. Reliability of outcome measures for people with lower-limb amputations: distinguishing true change from statistical error. Phys Ther. (2011) 91(4):555–65. doi: 10.2522/ptj.20100287
67. Tillander J, Hagberg K, Berlin O, Hagberg L, Branemark R. Osteomyelitis risk in patients with transfemoral amputations treated with osseointegration prostheses. Clin Orthop Relat Res. (2017) 475(12):3100–8. doi: 10.1007/s11999-017-5507-2
68. Juhnke DL, Beck JP, Jeyapalina S, Aschoff HH. Fifteen years of experience with integral-leg prosthesis: cohort study of artificial limb attachment system. J Rehabil Res Dev. (2015) 52(4):407–20. doi: 10.1682/JRRD.2014.11.0280
69. Al Muderis M, Khemka A, Lord SJ, Van de Meent H, Frölke JPM. Safety of osseointegrated implants for transfemoral amputees: a two-center prospective cohort study. J Bone Joint Surg Am. (2016) 98(11):900–9. doi: 10.2106/JBJS.15.00808
70. Mohamed J, Reetz D, van de Meent H, Schreuder H, Frölke JP, Leijendekkers R. What are the risk factors for mechanical failure and loosening of a transfemoral osseointegrated implant system in patients with a lower-limb amputation? Clin Orthop Relat Res. (2022) 480(4):722–31. doi: 10.1097/CORR.0000000000002074
71. Hoellwarth JS, Tetsworth K, Kendrew J, Kang NV, van Waes O, Al-Maawi Q, et al. Periprosthetic osseointegration fractures are infrequent and management is familiar. Bone Joint J. (2020) 102-B(2):162–9. doi: 10.1302/0301-620X.102B2.BJJ-2019-0697.R2
72. Wood P, Small C, Mahoney P. Perioperative and early rehabilitation outcomes following osseointegration in UK military amputees. BMJ Mil Health. (2020) 166:294–301. doi: 10.1136/jramc-2019-001185
73. Hoellwarth JS, Tetsworth K, Oomatia A, Akhtar MA, Xu H, Al Muderis M. Association between osseointegration of lower extremity amputation and mortality among adults. JAMA Netw Open. (2022) 5(10):e2235074. doi: 10.1001/jamanetworkopen.2022.35074
74. Black GG, Vaeth AM, Chen Y, Truong AY, Reif TJ, Rozbruch SR, et al. Osseointegration for lower limb amputation: understanding the risk factors and time courses of soft tissue complications. Ann Plast Surg. (2023) 90(6S Suppl 5):S452–6. doi: 10.1097/SAP.0000000000003477
75. Örgel M, Petri M, Ranker A, Wirries N, Graulich T, Krettek C, et al. Management, outcome, and novel classification system of periprosthetic fractures in patients with transcutaneous osseointegrated prosthetic systems (TOPS)–a retrospective cohort analysis. Arch Orthop Trauma Surg. (2022) 142(7):1499–509. doi: 10.1007/s00402-021-03826-y
76. Lundberg M, Hagberg K, Bullington J. My prosthesis as a part of me: a qualitative analysis of living with an osseointegrated prosthetic limb. Prosthet Orthot Int. (2011) 35(2):207–14. doi: 10.1177/0309364611409795
77. Hansen CH, Hansen RL, Jørgensen PH, Petersen KK, Norlyk A. The process of becoming a user of an osseointegrated prosthesis following transfemoral amputation: a qualitative study. Disabil Rehabil. (2019) 41(3):276–83. doi: 10.1080/09638288.2017.1385651
78. Haggstrom EE, Hansson E, Hagberg K. Comparison of prosthetic costs and service between osseointegrated and conventional suspended transfemoral prostheses. Prosthet Orthot Int. (2012) 37(2):152–60. doi: 10.1177/0309364612454160
79. Hansson E, Hagberg K, Cawson M, Brodtkorb TH. Patients with unilateral transfemoral amputation treated with a percutaneous osseointegrated prosthesis: a cost-effectiveness analysis. Bone Joint J. (2018) 100-B(4):527–34. doi: 10.1302/0301-620X.100B4.BJJ-2017-0968.R1
80. Hagberg K, Hansson E, Brånemark R. Outcome of percutaneous osseointegrated prostheses for patients with unilateral transfemoral amputation at two-year follow-up. Arch Phys Med Rehabil. (2014) 95(11):2120–7. doi: 10.1016/j.apmr.2014.07.009
81. Handford C, McMenemy L, Kendrew J, Mistlin A, Akhtar MA, Parry M, et al. Improving outcomes for amputees: the health-related quality of life and cost utility analysis of osseointegration prosthetics in transfemoral amputees. Injury. (2022) 53(12):4114–22. doi: 10.1016/j.injury.2022.10.007
82. Frossard L, Berg D, Merlo G, Quincey T, Burkett B. Cost comparison of socket-suspended and bone-anchored transfemoral prostheses. J Prosthet Orthot. (2017) 29(4):150–60. doi: 10.1097/JPO.0000000000000142
83. Frossard LA, Merlo G, Burkett B, Quincey T, Berg D. Cost-effectiveness of bone-anchored prostheses using osseointegrated fixation: myth or reality? Prosthet Orthot Int. (2018) 42(3):318–27. doi: 10.1177/0309364617740239
84. Black GG, Jung W, Wu X, Rozbruch SR, Otterburn DM. A cost-benefit analysis of osseointegrated prostheses for lower limb amputees in the US health care system. Ann Plast Surg. (2022) 88(3):S224–8. doi: 10.1097/SAP.0000000000003183
85. National Institute for Health and Care Excellence (NICE). Developing NICE Guidelines: the Manual. London. (2014). Available online at: https://www.nice.org.uk/process/pmg20/chapter/introduction (Accessed June 28, 2022).
86. Swedish Council on Health Technology Assessment (SBU). Methods for Health Economic Evaluations–A Guideline Based on Current Practices in Europe. (2015). Available online at: https://www.eunethta.eu/eunethta-methodological-guideline-methods-for-health-economic-evaluations/ (Accessed June 28, 2022).
87. CADTH. Guidelines for the Economic Evaluation of Health Technologies: Canada (4th edition). Ottawa, ON. (2017). Available online at: https://www.cadth.ca/guidelines-economic-evaluation-health-technologies-canada-0 (Accessed June 28, 2022).
88. Haacker M, Hallett TB, Atun R. On discount rates for economic evaluations in global health. Health Policy Plan. (2020) 35(1):107–14. doi: 10.1093/heapol/czz127
89. Wurdeman SR, Stevens PM, Campbell JH. Mobility analysis of amputees (MAAT I): quality of life and satisfaction are strongly related to mobility for patients with a lower limb prosthesis. Prosthet Orthot Int. (2018) 42(5):498–503. doi: 10.1177/0309364617736089
90. Gaffney BMM, Davis-Wilson HC, Awad ME, Tracy J, Melton DH, Lev G, et al. Daily steps and stepping cadence increase one-year following prosthesis osseointegration in people with lower-limb amputation. Disabil Rehabil. (2023):1–6. doi: 10.1080/09638288.2023.2200036
91. Hagberg K. Bone-anchored prostheses in patients with traumatic bilateral transfemoral amputations: rehabilitation description and outcome in 12 cases treated with the OPRA implant system. Disabil Rehabil Assist Technol. (2019) 14(4):346–53. doi: 10.1080/17483107.2018.1449016
92. Williams RM, Ehde DM, Smith DG, Czerniecki JM, Hoffman AJ, Robinson LR. A two-year longitudinal study of social support following amputation. Disabil Rehabil. (2004) 26(14–15):862–74. doi: 10.1080/09638280410001708878
93. Cavanagh SR, Shin LM, Karamouz N, Rauch SL. Psychiatric and emotional sequelae of surgical amputation. Psychosomatics. (2006) 47(6):459–64. doi: 10.1176/appi.psy.47.6.459
94. Sahu A, Sagar R, Sarkar S, Sagar S. Psychological effects of amputation: a review of studies from India. Ind Psychiatry J. (2016) 25(1):4–10. doi: 10.4103/0972-6748.196041
95. Calman L, Brunton L, Molassiotis A. Developing longitudinal qualitative designs: lessons learned and recommendations for health services research. BMC Med Res Methodol. (2013) 13:14. doi: 10.1186/1471-2288-13-14
96. Audulv Å, Hall EOC, Kneck Å, Westergren T, Fegran L, Pedersen MK, et al. Qualitative longitudinal research in health research: a method study. BMC Med Res Methodol. (2022) 22(1):255. doi: 10.1186/s12874-022-01732-4
98. Ontario H. Osseointegrated prosthetic implants for people with lower-limb amputation: a health technology assessment. Ont Health Technol Assess Ser. (2019) 19(7):1–126. PMID: 31911825.
99. Banducci E, Al Muderis M, Lu W, Bested SR. The safety of one-stage versus two-stage approach to osseointegrated prosthesis for limb amputation. Bone Jt Open. (2023) 4(7):539–50. doi: 10.1302/2633-1462.47.BJO-2022-0117.R1
100. Berg D, Frossard L. Health service delivery and economic evaluation of limb lower bone-anchored prostheses: a summary of the Queensland artificial limb service’s experience. Can Prosthet Orthot J. (2021) 4(2):36210. doi: 10.33137/cpoj.v4i2.36210
101. Al Muderis MM, Lu WY, Li JJ, Kaufman K, Orendurff M, Highsmith MJ, et al. Clinically relevant outcome measures following limb osseointegration: systematic review of the literature. J Orthop Trauma. (2018) 32(2):e64–75. doi: 10.1097/BOT.0000000000001031
102. Frossard L, Ferrada L, Quincey T, Burkett B, Berg D. Development of a government continuous quality improvement procedure for assessing the provision of bone anchored limb prosthesis: a process re-design descriptive study. Can Prosthet Orthot J. (2018) 1(2):1–14. doi: 10.33137/cpoj.v1i2.31326
103. Pousett B, Budzinski C, Labbé D, Miller W. A mixed-methods study on prosthesis use among older Canadians with lower-limb amputations. Can Prosthet Orthot J. (2021) 4(1):36833. doi: 10.33137/cpoj.v4i1.36833
104. Tariq S, Woodman J. Using mixed methods in health research. JRSM Short Rep. (2013) 4(6):2042533313479197. doi: 10.1177/2042533313479197
Keywords: bone-anchored prosthesis, osseointegration, lower extremity, transfemoral, treatment outcome, postoperative complications, patient experience, cost-effectiveness analysis
Citation: Rehani M, Stafinski T, Round J, Jones CA and Hebert JS (2024) Bone-anchored prostheses for transfemoral amputation: a systematic review of outcomes, complications, patient experiences, and cost-effectiveness. Front. Rehabil. Sci. 5:1336042. doi: 10.3389/fresc.2024.1336042
Received: 9 November 2023; Accepted: 5 February 2024;
Published: 2 April 2024.
Edited by:
Ruediger Rupp, University Hospital Heidelberg, GermanyReviewed by:
Laurent Frossard, Griffith University, Australia© 2024 Rehani, Stafinski, Round, Jones and Hebert. This is an open-access article distributed under the terms of the Creative Commons Attribution License (CC BY). The use, distribution or reproduction in other forums is permitted, provided the original author(s) and the copyright owner(s) are credited and that the original publication in this journal is cited, in accordance with accepted academic practice. No use, distribution or reproduction is permitted which does not comply with these terms.
Disclaimer: All claims expressed in this article are solely those of the authors and do not necessarily represent those of their affiliated organizations, or those of the publisher, the editors and the reviewers. Any product that may be evaluated in this article or claim that may be made by its manufacturer is not guaranteed or endorsed by the publisher.
Research integrity at Frontiers
Learn more about the work of our research integrity team to safeguard the quality of each article we publish.