- 1Department of Pediatrics, Boston Children’s Hospital, Boston, MA, United States
- 2Department of Pediatrics, University of Florida, Gainesville, FL, United States
- 3Department of Physical Therapy, University of Florida, Gainesville, FL, United States
- 4Breathing Research and Therapeutics (BREATHE) Center, University of Florida, Gainesville, FL, United States
- 5Department of Surgery, University of Florida, Gainesville, FL, United States
- 6Department of Speech, Language and Hearing Sciences, University of Missouri, Columbia, MO, United States
- 7Dalton Cardiovascular Center Investigator, University of Missouri, Columbia, MO, United States
Introduction: Pompe disease is an inherited disease characterized by a deficit in acid-α-glucosidase (GAA), an enzyme which degrades lysosomal glycogen. The phrenic-diaphragm motor system is affected preferentially, and respiratory failure often occurs despite GAA enzyme replacement therapy. We hypothesized that the continued use of diaphragm pacing (DP) might improve ventilator-dependent subjects' respiratory outcomes and increase ventilator-free time tolerance.
Methods: Six patients (3 pediatric) underwent clinical DP implantation and started diaphragm conditioning, which involved progressively longer periods of daily, low intensity stimulation. Longitudinal respiratory breathing pattern, diaphragm electromyography, and pulmonary function tests were completed when possible, to assess feasibility of use, as well as diaphragm and ventilatory responses to conditioning.
Results: All subjects were eventually able to undergo full-time conditioning via DP and increase their maximal tolerated time off-ventilator, when compared to pre-implant function. Over time, 3 of 6 subjects also demonstrated increased or stable minute ventilation throughout the day, without positive-pressure ventilation assistance.
Discussion: Respiratory insufficiency is one of the main causes of death in patients with Pompe disease. Our results indicate that DP in Pompe disease was feasible, led to few adverse events and stabilized breathing for up to 7 years.
1. Introduction
Pompe disease is a rare autosomal recessive disorder caused by a mutation in the gene that encodes lysosomal enzyme acid-α-glucosidase (GAA). A deficiency or absence of GAA leads to glycogen accumulation in multiple organ systems, including cardiac, striated, and smooth muscles, visceral organs, and neuronal tissue (1–4). The accumulation of glycogen triggers a chain of biochemical and metabolic events that result in diffuse cell death in the involved tissues (1, 5, 6). The consequent impairment of the neurologic, neuromuscular and cardiovascular system leads to the typical features of the disease: developmental delay, loss of balance, progressive general hypotonia and muscular weakness, respiratory insufficiency, cardiomegaly, cardiorespiratory failure and, eventually, death (7–10).
Based on age and severity of onset, the patients can be classified as early-onset (infantile, EOPD) or later-onset (juvenile or adult, LOPD) types. The early-onset form is a severe life-threatening condition characterized by profound hypotonia and weakness that, when untreated, inevitably leads to cardiorespiratory failure and death of affected patients (11). In contrast, the later-onset forms are notable for a slow, progressive deterioration of strength and ventilatory function, without significant cardiac involvement. Ventilatory muscle weakness and hypoventilation are predominant and common features with disease progression of both early- and later-onset Pompe disease (12–16). Commercially-available enzyme replacement therapy (ERT) infusions supplement innate GAA production, to preserve walking and decelerate respiratory declines (17–20). Respiratory failure and ventilator dependence are inevitable outcomes. Diaphragm functional impairment is well documented in Pompe disease (15, 16), but an accumulation of evidence indicates that declines in phrenic motoneuron function are probably inevitable as the disease progresses (3, 4, 6, 13). Thus, respiratory failure will ultimately reflect impairments of the entire phrenic-diaphragm motor unit (i.e., motoneuron and myofibers).
The diaphragm is the primary breathing muscle and contraction enables tidal volume during resting breathing (21). When diaphragm integrity is critically compromised, life-saving correction is required to prevent death. Mechanical ventilation (MV) sustains ventilation in subjects with hypoventilation and respiratory failure and in many cases can stabilize patient-reported outcomes and maintain survival (22, 23). However, its use can also be associated with impaired quality of life (24, 25) and potential severe noninfectious (such as cardiovascular repercussion or barotrauma associated with high ventilator settings) and infectious (e.g., tracheitis, pneumonia) complications (26, 27). Additionally, prolonged use of MV and extended diaphragmatic inactivity may independently lead to severe diaphragm-muscle atrophy and dysfunction (ventilator-induced diaphragmatic dysfunction - VIDD), which may impede weaning from MV after an acute illness (28–30).
Diaphragm pacing (DP) is a form of respiratory support that generates ventilation via direct stimulation of the phrenic nerves to evoke diaphragm contraction. When used for patients with spinal cord injury (SCI), DP has been associated with reduced reliance on positive pressure MV (31–33). We previously reported an initial response to 6 months of DP, in three subjects with severe Pompe disease (34). This work demonstrated the feasibility of DP in this population, and provided initial evidence of a possible rehabilitative impact of DP. Further, the success of DP in that small sample of Pompe patients suggested that the diaphragm muscle was functional, but may not have been receiving optimal neural (phrenic) input during spontaneous breathing. The aim of this observational study was to build upon the prior report by comprehensively evaluating longer-term (e.g., up to 7 years post-DP implant) changes in respiratory function in adult and pediatric subjects with Pompe disease who underwent DP to treat ventilatory failure. We hypothesized that use of DP would preserve or improve the diaphragm activity and independent breathing ability of implanted patients.
2. Methods
2.1. Study design
This was a longitudinal observational study of changes in respiratory function and diaphragm activity following clinical implantation of DP. Study procedures were approved by the University of Florida Institutional Review Board, and the study was registered at www.clinicaltrials.gov (NCT02354651). The active study period ended in January 2019.
2.2. Participants
Subjects were eligible to participate if they were between ages 2 and 65 years; diagnosed with Pompe disease via mutational analysis or GAA enzyme activity assay in blood spot and/or fibroblast culture less than 40% of control values; exhibited chronic ventilatory insufficiency and functional quadriparesis; and were eligible to receive a diaphragmatic pacemaker as part of their clinical care. Subjects who required six or more daily hours of invasive (MV) or non-invasive ventilatory (NIV) support while awake and upright, for at least 21 days in duration, were considered to require prolonged mechanical ventilation (35).
Subjects, or their healthcare surrogate reviewed the informed consent form, received an opportunity to ask questions regarding the risks and benefits of their participation, and provided their consent to participate.
2.3. Diaphragm pacemaker
Local surgeons evaluated patients for eligibility for diaphragm pacing and implanted the NeuRx Diaphragm Pacing Stimulation system (DPS® NeuRx RA/4 system, Synapse Biomedical) as part of clinical management of chronic ventilatory insufficiency. Briefly, implantation occurred through laparoscopic mapping of diaphragm contractility to identify the motor point of each hemidiaphragm, followed by implantation of two bipolar stimulating electrodes in each hemidiaphragm motor point, plus a subcutaneous reference electrode (36). Lead wires were then externalized to a pacing socket and connected to a removable battery-operated external pulse generator (EPG). Figure 1 illustrates the components of the DP system. While the patient remained under anesthesia the surgical team determined the maximal stimulation amplitude and frequency that could be used. However, since sensory function remains intact with Pompe disease, the initial DP settings were determined only after the patient awakened. The stimulus parameters for each electrode were titrated to elicit a comfortable level of stimulation, and the respiratory rate was matched to the patient's spontaneous rate. The EPG was programmed to deliver a stimulation amplitude, frequency, and pulse width at a fixed respiratory rate, with the goal to deliver tidal volume and minute ventilation through the stimulated diaphragm contractions (37).
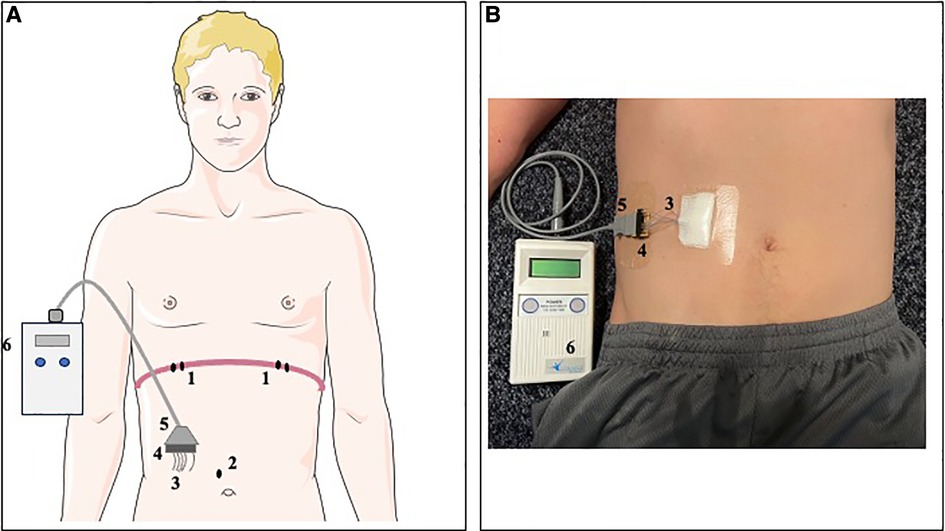
Figure 1. Representation of DP system in a schematic model (A) and simulated participant (B). Two bipolar electrodes (1) were laparoscopically implanted adjacent to the motor point of each hemidiaphragm. Additionally, a subcutaneous reference electrode (2) was implanted in the abdominal fascia. The four stimulation leads and reference electrode were externalized (3) in the right upper quadrant of the abdomen and mounted to an external connector block (4). An external connector cord (5) could be connected to the DP external pulse generator (6) to deliver stimulations at the programmed stimulation settings.
2.4. Diaphragm conditioning
Subjects began post-operative diaphragm conditioning as soon as possible (usually 3–5 days post-operatively) with an initial goal to tolerate 30 min of stimulation at the highest comfortable amplitude. The goal of diaphragm conditioning was to deliver a consistent stimulation that preserved inspiratory volume during reduced or removed positive pressure ventilation. Conditioning typically progressed by small increases in pacing duration every 2–5 days as tolerated, toward a goal of 24-hour pacing by day 30 post-surgery. The amplitude or pulse width of the external stimulator was increased on follow-up clinical visits, if the increase led to pain-free improvements in breath volume. In addition, subjects were encouraged to pace for short periods daily with reduced or no MV support. If subjects could not tolerate breathing without MV, they used the lowest MV support that yielded stable breathing for at least 20 min at a time.
2.5. Study measurements
Feasibility was measured through patient or parent reports of pacemaker use and tracking of adverse events. Response to pacing was evaluated by changes in positive pressure breathing support, breathing pattern without ventilator assistance, pulmonary functional tests, and diaphragm EMG. When possible, the respiratory and neuromuscular responses to diaphragm conditioning were measured within the first week, 1-month, and 6-month postoperative follow-ups, with longer-term repeated measurements every 1–2 years as able, when subjects were in their usual state of health. Test sessions occurred while patients were seated as upright as possible. Pulse oximetry, heart rate, respiratory rate, and end-tidal CO2 were monitored during test sessions.
2.5.1. Breathing pattern
During each study visit, the tidal volume (VT), respiratory rate (RR) and minute ventilation (VE) were collected when possible. Airflow and pressure were sampled directly from the airway opening at 100 Hz frequency, using a pressure transducer and pneumotachograph connected to a clinical respiratory monitor (NM3, Philips-Respironics). Up to 20 min of ventilation were recorded both with and without the use of the diaphragm pacer, during the lowest tolerated support.
2.5.2. Pulmonary functional tests
Pulmonary functional testing included peak cough flow and the pressure-generating capacity of the inspiratory muscles. Tests were administered in accordance with the American Thoracic Society and the European Respiratory Society guidelines (38).
Voluntary peak cough flow (PCF) represents the largest expiratory airflow recorded during a maximal volitional cough effort (39). PCF data were collected with a heated pneumotachograph (HR 3500B, 3700B, or 3813 Hans Rudolph, Shawnee, KS) connected to the tracheostomy or facemask. Since PCF can be influenced by inspiratory operating volume (40), patients above 4 years of age were instructed to take a deep breath and cough as forcefully as possible. Spontaneous coughs were recorded in preschool-aged children. Airflow from the pneumotach was recorded by a data acquisition system (S30-16 ADInstruments, Boston, MA) and laptop computer.
Maximal inspiratory pressure (MIP) represents the greatest pressure generated during a maximal voluntary inspiratory effort (41). A pressure transducer connected to a one-way valve was directly attached to the subject's tracheostomy (n = 5), or placed over the nose and mouth with a face mask (n = 1). A minimum of 3 trials was obtained; additional trials were administered if pressure efforts showed >20% variation. Participants were provided with as much rest as needed between efforts, and the average of the efforts was tracked over time.
2.5.3. Diaphragm unstimulated EMG
Diaphragm activity was recorded during assessments of spontaneous (unpaced), off-ventilator minute ventilation and maximal inspiratory pressure. The external pacer leads were connected to a digital analog converter (PowerLab 16/30, ADInstruments) via a customized adaptor. Signals were sampled at 20 kHz, band-pass filtered (1–5,000 kHz).
2.5.4. Data analysis
One-minute periods of breathing were selected for analysis. Selections were within the middle of the acquired breathing pattern sample and represented stable periods free from speaking, moving, or coughing. To evaluate longitudinal changes in the non-ventilated breathing pattern following DP, a mixed effects model with repeated measures was used. The independent variables were the time post-DP implant (4–6 weeks, 3–6 months, and 24 ± 3 months) and support (with or without DP). The time ranges were selected to maximize the number of available participants during each interval. For significant main effects, multiple comparisons were corrected using a Tukey test.
To prepare the diaphragm EMG signal for further analysis, ECG artifact was removed from the EMG signal, using an off-line gating approach. The EMG signal was rectified, and then high pass filtered (cutoff: 10 Hz) to remove T-wave offset. Then, the R wave was removed from the ECG channel by creating a short window (60–100 msec, depending on underlying heart rate), smoothing with a triangular window, and using the threshold feature to remove the portion of signal occurring during the QRS complex. To replace signal in areas where the QRS was removed, the EMG signal within the immediately preceding window was copied and inserted. The peaks of the root mean square of resting inspirations were averaged across one-minute intervals and normalized to the amplitude achieved during maximal inspiratory pressure. Using mixed effects models, two separate analyses evaluated changes in unpaced, off-ventilator spontaneous diaphragm activity over time; one analysis focused on changes in MIP-normalized EMG activity during resting breathing, and the second approach evaluated changes in EMG activity during resting breathing, using the coefficient of variation averaged across both hemidiaphragms. Sphericity was assessed with Geisser-Greenhouse's epsilon. For all statistical analyses, significance was p < 0.05.
3. Results
3.1. Participants
During the study period, nine subjects were enrolled. Two eligible patients elected not to undergo surgical placement, while a third participant was determined to be clinically ineligible for a DP implant due to coexisting medical conditions. Table 1 shows clinical characteristics of the six subjects who underwent DP. All required chronic, full-time MV at the time of implant (tracheostomy: n = 4, NIV: n = 2). Three subjects (one male, two females) were children diagnosed with EOPD in infancy who also required invasive feeding support, and the remaining three were adults with LOPD (two males, one female) who fed independently. Four subjects enrolled in the study upon DP implantation, while the other 2 subjects (subjects 4, 6) received DP at outside institutions and enrolled within 3 months following implantation. All subjects were evaluated at least 3 times, over a span ranging from early recovery up to 7 years post-DP.
3.2. Feasibility measurements
All subjects initiated clinical diaphragm conditioning within 5 days of implantation. Five subjects (83%) achieved 24-hours of DP use within the first month and thus commenced DP assisted breathing for short periods with reduced or no MV. Subject 1 was able to independently attach and detach the external pulse generator, but the remaining subjects required caregiver support to maintain a wearing schedule. Subject 6 tolerated pacing, but required physical assistance from outside caregivers to attach the external pulse generator. This subject commenced diaphragm conditioning with the pacemaker only when healthcare assistants visited the home (∼1–2 h/day) and did not consistently increase her DP use at long-term follow-up.
The use of the DP stimulator was periodically restricted by breaks in one or more external pacemaker leads. Five subjects (83%) experienced at least one lead break. However, 4 of the 9 lead breaks occurred in a single subject (subject 2). To reduce the likelihood of external lead damage, subject 2's diaphragm conditioning regimen was modified. The subject continued to breathe without support during waking hours, and DP stimulation was limited to periods when the subject was stationary in a wheelchair during school. Leads repairs were influenced by subjects' access to a trained DPS surgeon in their local area and occurred within a few days, up to several weeks following lead disruption. Following lead repairs, the ability to pace with reduced support resumed unimpeded. A lead break was considered rare for most individuals. On average, a lead break occurred every 3.7 person-years of DP during a cumulative 34 person-years of the study.
3.3. Adverse events
Every subject experienced one or more adverse events during the observational period, and two adult subjects were hospitalized for serious adverse events. Nearly all adverse events were medical complications of subjects' underlying severe Pompe disease and chronic ventilatory insufficiency. The only adverse events determined to be related to DP were the presence of skin irritation or scabbing of the external leads in 3 subjects (Table 2).
3.4. Unassisted breathing
Prior to DP implantation, all subjects required full-time positive pressure invasive or non-invasive ventilation (summarized in Table 1, individual case descriptions in Appendix). The daily assisted ventilation requirement generally decreased after diaphragm pacing implantation and conditioning (Figure 2). The individual subject responses to DP varied from a small improvement to a substantial reduction in the amount of daytime during which MV was required. Three subjects (subjects 1, 2 and 5) were fully weaned from daytime MV within 6 months of DP implantation. Longer-term follow-up revealed a largely preserved ventilator-free breathing while awake for up to 7 years post-implant.
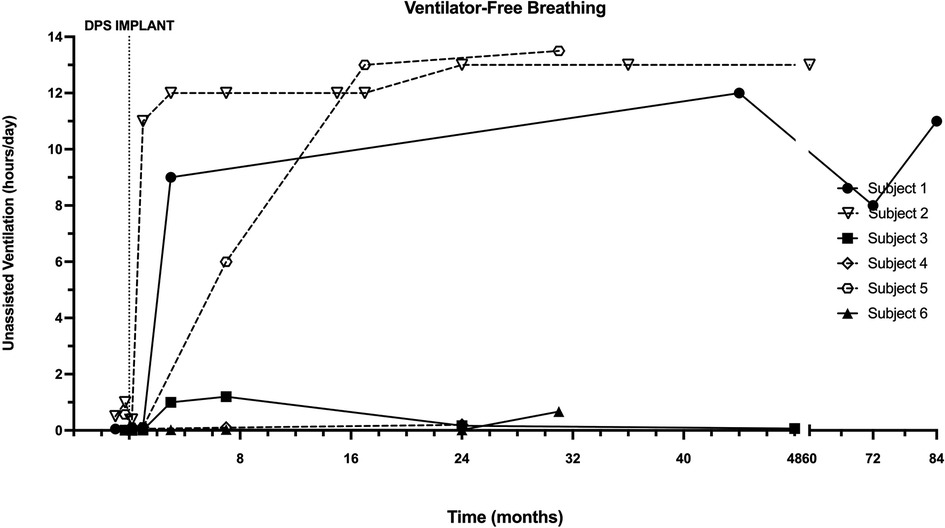
Figure 2. Longitudinal changes in ventilator-free breathing with DP. Ventilator-free breathing in each patient was recorded through interrogation of ventilator data chips during assessments following DP. One adult and two pediatric subjects achieved sustained weaning during waking hours, which typically occurred within the first 6 months.
3.4.1. Effect of DP on unassisted breathing pattern
The timeframes for testing subjects varied due to their timing of enrollment, distance from our test center, ability to travel, and state of health. Thus, subjects were tested within the first 4–6 weeks (n = 5), 3–6 months (n = 4), and 24 ± 3 months (n = 5) following DP implant. Figure 3 illustrates differences in tidal volume, respiratory rate, and minute ventilation over the first two years post-DP. Values are compared for periods of no DP breathing vs. DP breathing. A significant time effect was detected for tidal volume (p = 0.031), with breath volume at 2 years significantly larger than 4–6 weeks. With No DP, tidal volume averaged: 4 wk: 3.7 ± 2.3 ml/kg, 6mo: 4.9 ± 1.8 ml/kg, 2y: 4.8 ± 1.8 ml/kg. Average VT values with DP were: 4 wk: 3.8 ± 2.4 ml/kg, 6mo: 5.6 ± 2.0 ml/kg, 2y: 5.6 ± 2.2 ml/kg. While DP appeared to increase average tidal volume at 6 and 24 months, these differences were not significant (p = 0.183). Significant differences in respiratory rate were not observed between on and off DP conditions (p = 0.657). This may be due to the fact that the rate of the DP stimulation was manually set to coincide with each subject's typical spontaneous breathing rate. Respiratory rate trended downward over time (p = 0.191). On average, the respiratory rate data for the No DP condition were: 4 wk: 35 ± 14 bpm, 6mo: 35 ± 15 bpm, and 2y: 27 ± 7 bpm. With DP, the values were: 4 wk: 34 ± 15 bpm, 6mo: 33 ± 10 bpm, 2y: 28 ± 5 bpm. Owing to the increased tidal volume, values for minute ventilation were elevated during DP, although this did not reach statistical significance due to extensive variability across subjects (p = 0.450). With No DP, minute ventilation values were: 4 wk: 4.2 ± 1.8 L/min, 6mo: 5.1 ± 1.7 L/min, 2y: 3.6 ± 0.8 L/min. With DP, minute ventilation values were 4 wk: 4.6 ± 2.2 L/min, 6mo: 5.4 ± 3.5 L/min, 2y: 3.8 ± 1.0 L/min.
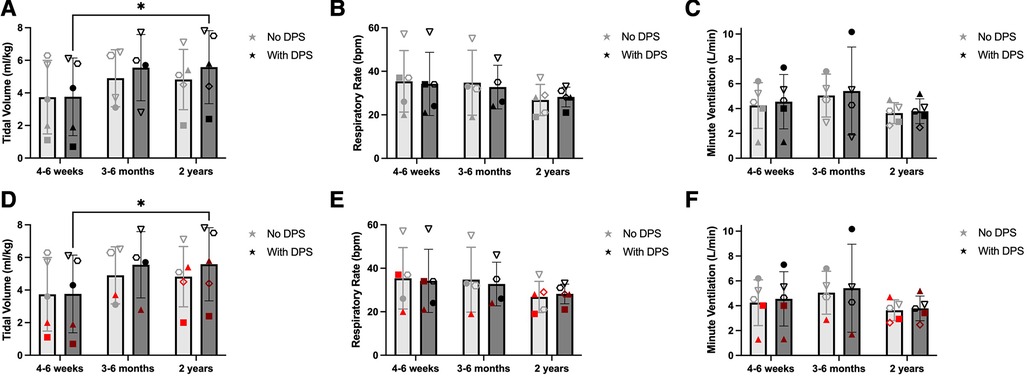
Figure 3. Effect of DP on unassisted breathing pattern. One-minute periods of off-ventilator breathing were with and without DP, and changes in ventilation were assessed between the first 4–6 weeks (n = 5), 3–6 months (n = 4), and 2 years (±3 months, n = 5) after DP. Paced tidal volume increased significantly between baseline and 2 years (A). Changes in respiratory rate (B) and minute ventilation (C) were more variable. Those who weaned during daytime hours (black) were observed to have greater tidal volume early after pacing (D) than those who continued full-time MV (red). Weaning-based differences in respiratory rate (E) or minute ventilation (F) were less discernable.
Figure 4 depicts longer-term changes in the individual breathing pattern of each subject, without ventilator or pacer support. Most participants demonstrated a rapid and shallow breathing pattern immediately following DP implant, that stabilized within the first 3–6 postoperative months. While within-subject variability of breath volume and minute ventilation was noted, particularly for subjects 1, 2, and 3, most subjects' daily tolerance for off-ventilator breathing remained consistent over the duration of this observational study.
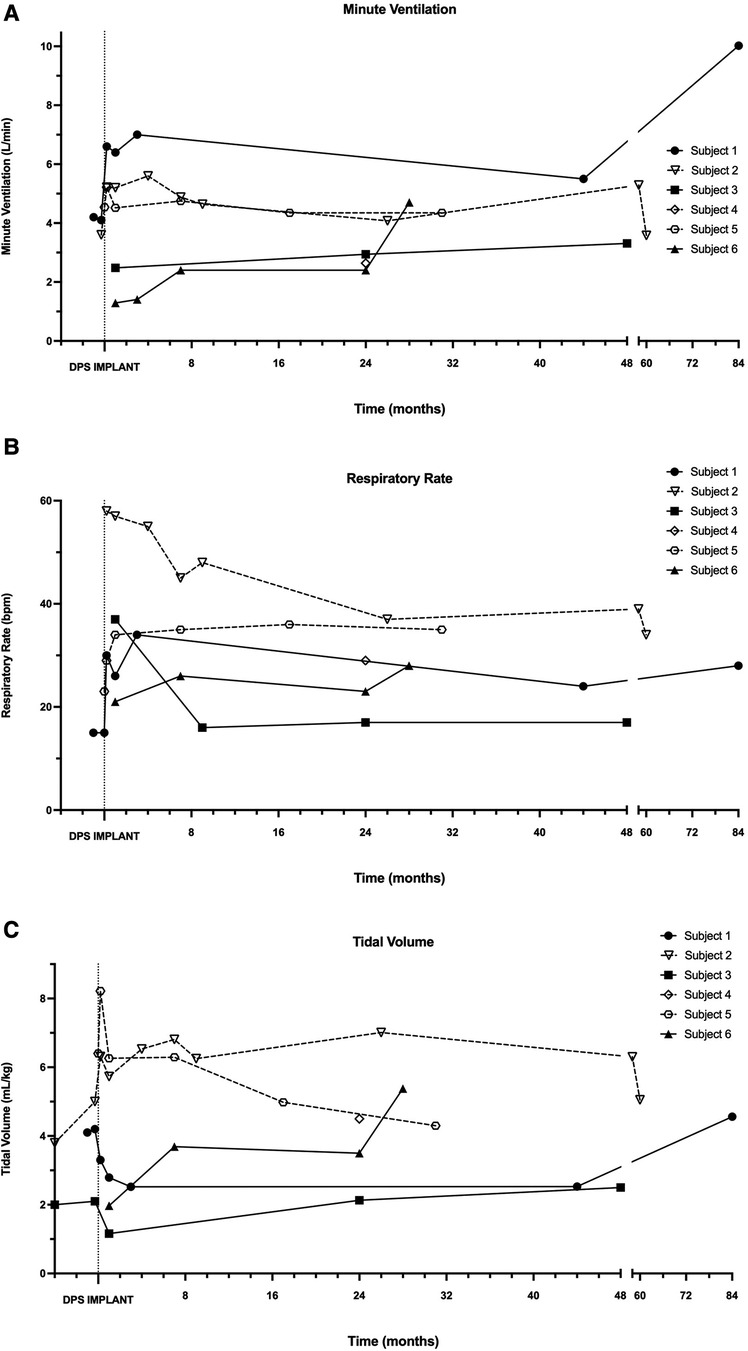
Figure 4. Longitudinal changes in independent minute ventilation, tidal volume and respiratory rate. During in-person assessments following DP implantation, minute ventilation (A), respiratory rate (B), and tidal volume (C) were recorded in each patient without the use of mechanical ventilation or DP.
3.5. Pulmonary function
Baseline pre-operative MIP and PCF were obtained from four subjects (subjects 1–3, 5). In each instance, MIP was profoundly impaired, and PCF values indicated an ineffective cough (Figure 5). Post-operatively, many subjects had modest decreases in MIP with a stabilization in PCF. One participant (subject 2) demonstrated large and sustained gains in both MIP and PCF post-implant.
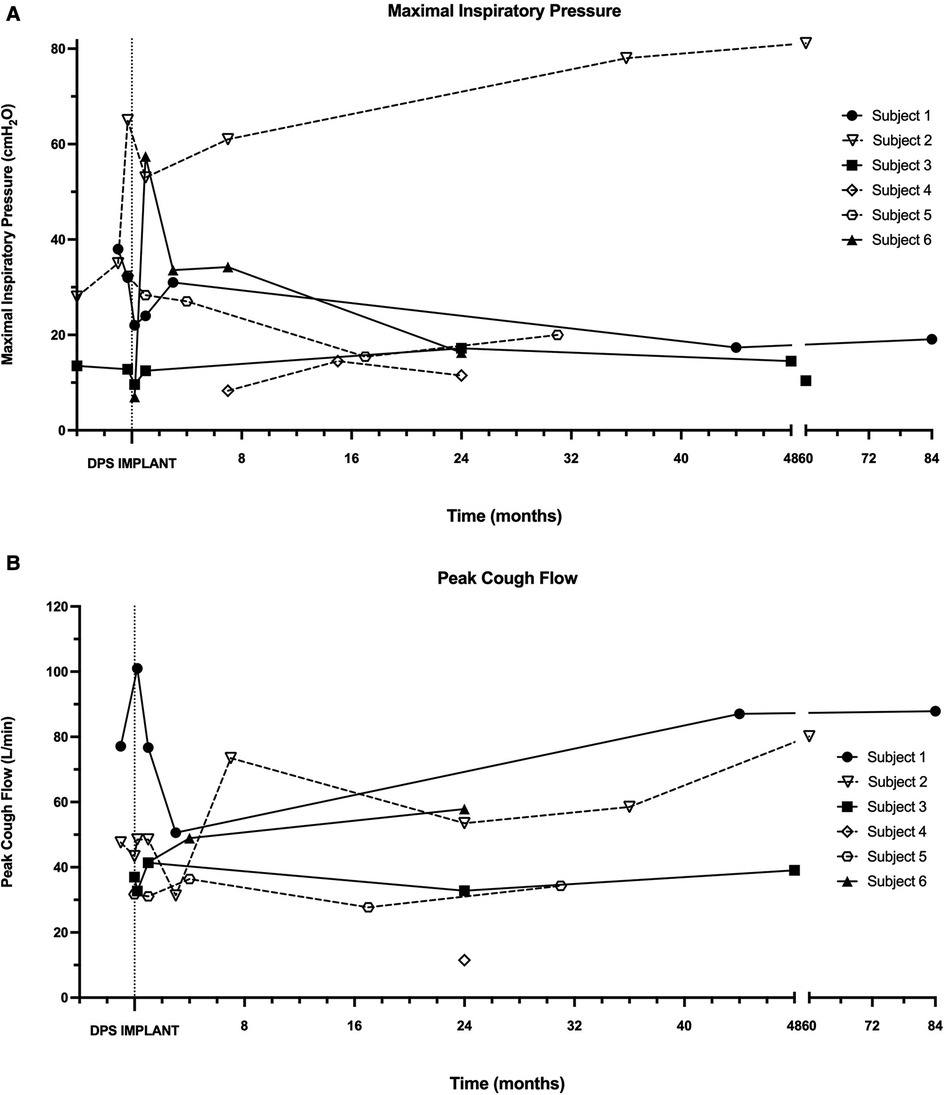
Figure 5. Maximal inspiratory pressure and peak cough flow. During in-person assessments following DP implantation, maximal inspiratory pressure (A) and peak cough flow (B) were recorded in each patient without the use of mechanical ventilation or DP.
3.6. Diaphragm electromyography
Inspiratory phasic bursting of the diaphragm was observed for all subjects while spontaneously breathing without MV or DP. Figure 6 depicts changes in the diaphragm peak integrated EMG (iEMG) amplitude and coefficient of variation of the iEMG, during unpaced, off-ventilator breathing, between 4 and 6 weeks (n = 5), 3–6 months (n = 4), and 2 years (±3 months, n = 5) following DP implant. The diaphragm EMG peak burst activity was normalized to the activation during the MIP maneuver (essentially, “% maximum”). Thus, a decline in normalized EMG output over time would indicate that spontaneous breathing was being achieved without needing to activate the diaphragm as intensely. This analyses suggested that diaphragm EMG activity trended downward over the duration of the study. However, between-subject variability was high, and no statistically significant changes were detected over time (p = 0.251). Variation in the subjects' breath-to-breath activation of the diaphragm was measured using the coefficient of variation of the iEMG signal and did not differ significantly (p = 0.185). While those who weaned from daytime MV tended to have greater iEMG coefficient of variation at 2 years than those who continued support, the low sample size did not permit us to test differences in activity between those who weaned from daytime MV and those who continued support. Notably, recordings from the indwelling electrodes yielded excellent EMG signal quality and enabled quantitative assessment of diaphragm activity of the subjects, for up to seven years following DP implant (Figure 7).
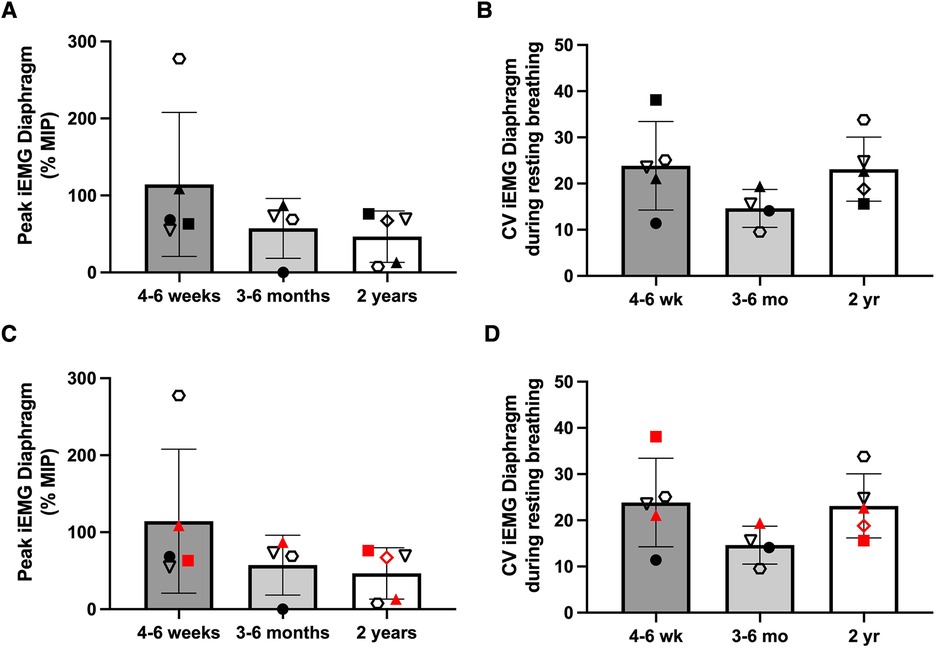
Figure 6. Longitudinal changes in diaphragm EMG during unassisted breathing. The peak integrated EMG of the right and left hemidiaphragms was recorded and averaged during off-ventilator, unpaced ventilation, and changes in activity were assessed between the first 4–6 weeks (n = 5), 3–6 months (n = 4), and 2 years (±3 months, n = 5) after DP. Individual results were variable, and no changes in peak iEMG (A) or coefficient of variation of the EMG (B) were detected. Further, no clear differences in peak iEMG (C) or coefficient of variation (D) were noted between those who weaned during daytime hours (black) and those who continued full-time MV (red).
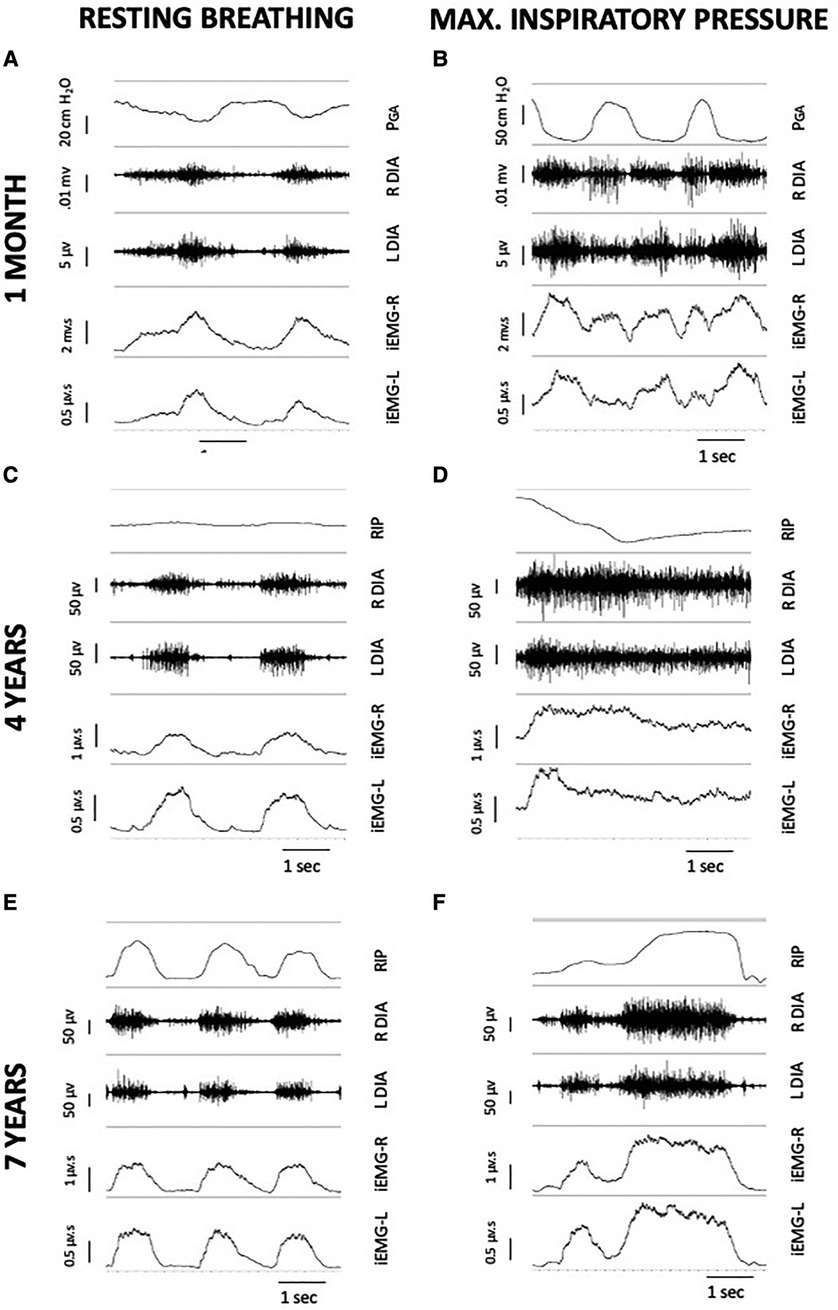
Figure 7. Serial, 5-second EMG recordings from indwelling right and left diaphragm electrodes of subject 1, during unpaced breathing without MV support (left column) and during MIP (right column). Each individual image includes a breath marker (either PGA or an abdominal RIP band), raw EMG of the right and left hemi-diaphragm, and a tracing of the integrated signal after cardiac filtering. At one month post-DP (A,B), PGA deflections remained negative during inspiratory efforts, indicating presence of a paradoxical breathing pattern and trunk rocking movements by the patient during off-ventilator breathing. At four years post-DP (C,D), the subject had repairs of DP leads and a ground wire replacement. Small, positive deflections of an abdominal RIP band during resting breathing (C) suggested emergence of diaphragm descent, with paradoxical movements during MIP due to predominant accessory muscle recruitment. At seven years post-DP (E,F), the ability to record inspiratory bursting from the diaphragm electrodes was retained in the subject, despite additional repairs of individual channel leads. DP: diaphragm pacing; EMG, electromyogram; MIP, maximal inspiratory pressure; MV, mechanical ventilation; PGA, gastric pressure; RIP, respiratory inductance plethysmography.
4. Discussion
This report outlines safety, feasibility, and longitudinal changes in breathing and diaphragm function following DP, in a small cohort of people with respiratory failure due to severe Pompe disease. It was noteworthy that following 2 years of DP use, an increase in unassisted tidal volume was detected in some subjects, accompanied by an increase in routine off-ventilator breathing time. This would not be expected to occur in the natural course of the disease. While the patient population remained prone to complications relating to their underlying neuromuscular disease, DP was well-tolerated. The few adverse events were related only to skin irritation and scabbing at points of wire externalization. Moreover, quantitative assessments of diaphragm activity during resting breathing and maximal voluntary efforts were a novel aspect of this study and possible for up to seven years, via recordings from indwelling electroides.
When unassisted breathing becomes impossible, one primary reason to consider the use of DP is that prolonged use of MV induces significant diaphragm atrophy and contractile dysfunction (28–30). The presence of ventilator-induced diaphragm dysfunction, or VIDD, is less understood in conditions where pre-existing neuromuscular dysfunction coincides with a new MV requirement (42). However, in a rat model, the presence of spinal cord injury greatly exacerbates VIDD (43). In the current cohort of Pompe patients, severe dysfunction of the phrenic motor system led to a chronic reliance on positive pressure ventilation. In some cases, the reliance of ventilator support was reduced for extended periods of the day following conditioning with DP. Thus, DP may be a supplemental approach that, when used in conjunction with other available strategies to preserve respiratory function, including enzyme replacement therapy, respiratory strength training, pulmonary hygiene, and respiratory support, may assist with stabilizing function. While many in the sample improved their ability to increase diaphragm activity and generate tidal volume over time, our methods do not permit us to directly attribute changes in independent breathing to an improved function of phrenic motor units.
The therapeutic benefits of phrenic stimulation to replace ventilation have been well-documented in those with SCI (44, 45). Use of DP early after SCI appears to increase the ability to voluntarily activate the diaphragm and generate tidal volume. Several groups have reported the use of DP to augment or completely replace positive pressure ventilation (33, 37, 46). Additionally, DP for SCI is associated with a reduced risk of respiratory infections (47) and improved quality of life (32). Beyond SCI, reports in congenital central hypoventilation syndrome (48), acute flaccid myelitis (49), and other neurological conditions (50) indicate off-label clinical use of DP can be implemented safely and associated with relatively few adverse effects (31).
Although cervical SCI disrupts the supraspinal control of the phrenic motor system, biology of the diaphragm remains relatively preserved. Thus, with sufficient phrenic stimulation, normal diaphragm contractility is the goal for DP in SCI (37, 44). When sensory loss accompanies SCI, patients can frequently tolerate high enough initial DP settings to enable a tidal volume sufficient for periods of off-ventilator, paced breathing (33, 51). It has been suggested that the DP settings can be increased upon implant in SCI, to the lowest settings necessary to achieve a functional stimulated breath volume (37, 51). Despite this guidance, the optimal DP “dose” and conditioning regimen for eliciting phrenic motor plasticity and ventilator weaning have not been evaluated in a controlled study.
In comparison to SCI, the pathophysiology of Pompe disease warrants a different approach – and expected clinical benefit – with DP. Sensory feedback remains intact in Pompe disease, and initial settings of the DP device were typically limited by participants' sensory tolerance. When subjects initiated diaphragm conditioning at our institution, we used a respiratory monitor to confirm a stable tidal volume and end-tidal CO2 during DP and recommended this approach whenever possible to avoid the presence of fatigue. Studies in an amyotrophic lateral sclerosis rodent model suggest that excessively high stimulation amplitude and frequency may contribute to motoneuron degeneration (52); thus, careful titration of stimulator amplitude and frequency is warranted. This initial descriptive study does not provide sufficient information to recommend an optimal “DP dose” to augment breathing ability in Pompe disease, but it does indicate that ongoing, Pompe related diaphragm or phrenic neuromuscular changes do not prevent DP from being effective.
Chronic ventilatory failure from Pompe disease accompanies fiber glycogenosis, muscle atrophy, and phrenic neuromuscular impairment (3, 4, 8). While DP could potentially counteract inactivity-induced weakness and atrophy of the diaphragm from prolonged MV in Pompe disease (4, 34), there is no evidence that DP would mitigate existing diaphragm pathology or reverse existing denervation of phrenic motor units. Moreover, coordination is required between the diaphragm and synergistic or accessory muscles of breathing and swallowing, to carry out complex respiratory-related behaviors and protective reflexes (53). Thus, normal minute ventilation and pulmonary function would not be a reasonable goal for DP in severe Pompe disease. On average, off-ventilator breath volumes of the sample (paced and unpaced) at 2 years post-DP increased by more than 20% over values early after implant. These relative gains in breath volume enabled daytime independence from MV in some subjects; those with low ventilation at the outset at implant remained largely MV-dependent. The small sample does not permit a detailed analysis of factors associated with daytime ventilator weaning. Moreover, since DP is not expected to alter the primary pathophysiology and clinical progression of Pompe disease, its therapeutic durability remains unknown.
Three subjects (subjects 1, 2, 5) achieved sustained daytime independence from MV with DP, which led to multiple quality of life enhancements. One subject was able to be discharged from a long-term facility to home, and another was able to be enrolled into regular classes at public school. Additionally, all three of these subjects were able to substantially reduce their requirements for home nursing and respiratory therapies. Overnight weaning from MV was not considered, due to the potential risk for central sleep apnea in Pompe disease (54). When DP was included in the acute care following spinal cord injury, it was associated with an average $144,444 in cost savings (55). The cost savings associated with use of DP to support daytime weaning are not understood for Pompe disease and outside the scope of this study.
The remaining subjects experienced an incomplete recovery of independent ventilatory function. Subject 3 was diagnosed with severe tracheomalacia and left mainstem bronchomalacia that required tracheostomy placement day 8 post-implant. Nevertheless, he was able to breathe up to 60 min without ventilator support at day 90, which had not been possible for at least 3 years prior to DP implant. The high-grade malacia necessitated some long-term positive pressure to prevent airway collapse, but over the following 3 years, his daytime inspiratory pressure requirement on the ventilator decreased from 29 cm H2O to <18 cm H2O. After 5 years of DP, the subject stopped DP and withdrew from participation, as his priorities shifted away from pacing. He passed away one year later. The major limitation for subject 4 was the rapid emergence of copious secretions without positive pressure ventilation. The patient did not have a functional swallow and required mechanical insufflation-exsufflation for airway clearance due to chronic aspiration. Despite the difficulty with secretion management, the subject was able to reduce MV inspiratory pressure from 26 cm H2O pre-implant to 22 cm H2O post-implant, and he improved from <1 min to 10 consecutive minutes of breathing using only DP. In the first post-operative year, subject 6 used DP for <2 h daily due to lack of available support at home. At 15 months post-implant, the subject was able to commit to 8 h of daily pacing, and daily off-ventilator time increased to 20 min. By the final visit, DP use increased to 24 h per day, and she reported 50 consecutive minutes of off-ventilator pacing. While these subjects did not experience sustained ventilator weaning, reported benefits of even short periods included an improved ability to smell, more natural speech, and greater ease with dependent transfers.
While DP appeared to stabilize or improve unassisted breathing over time, MIP continued to decline in most subjects. The diaphragm is a mixed muscle with approximately 50%–55% slow-twitch, fatigue resistance fibers, with the remaining fast-twitch fibers divided equally between fatigue-resistant and fatigable fibers (56). In healthy individuals, only a small percentage of the diaphragm's force generating capacity is needed for a tidal breath, presumably through contractions of slower, fatigue-resistant fibers. In contrast, robust diaphragm and inspiratory muscle recruitment occurs during MIP. Continuous, low-frequency chronic DP furthered fatigue resistance of diaphragm muscle fibers in human SCI (57). If similar fiber adaptations occurred with DP in Pompe disease, these fiber properties may have enabled longer independent ventilation at the expense of maximal foce generation during MIP or expulsive reflexes (58). Additionally, loss of pulmonary function is significantly associated with vastus lateralis muscle fiber damage in both infantile and older-onset Pompe phenotypes (59). In previous studies, vacuolated fibers and autophagic vacuoles were particularly observed in fast-twitch fibers of patients with Pompe disease and often preceded severe disease, along with a high occurrence of structural alterations in those with severe clinical disease (60). Observations from the diaphragm of one subject (subject 1) suggest that fiber degeneration observed in other patients with severe Pompe disease was not reversed by DP (4). The impacts of DP on an atrophic diaphragm require further clarification.
4.1. Limitations
This project studied an unusual clinical management approach for a rare disease. Thus, the small sample and heterogeneity of the participants limited our ability to perform detailed statistical analyses. While all participants exhibited functional dependence and chronic ventilatory failure, many had other medical comorbidities or underlying complications from long-standing Pompe disease (e.g., airway malacia, finger/hand contractures) that influenced their ability to utilize DP without interruption. Participants and their families shared anecdotal reports that some daily functional activities improved during DP, including improved trunk control and sitting endurance, breath and speech support, and airway clearance. However, we did not systematically administer a patient-reported outcome. A larger sample could help delineate the gains of DP as perceived by patients and their caregivers, and perhaps better distinguish the characteristics of DP responders vs. non-responders.
Indwelling EMG revealed robust inspiratory bursting during maximal inspiratory efforts and a potential trend toward lower relative recruitment of the diaphragm during tidal breathing. While this observation could be due to conditioning of the diaphragm, it is also possible that compensatory recruitment of the accessory inspiratory muscles contributed to a lower diaphragmatic recruitment during off-ventilator breathing. Moreover, compensatory abdominal muscle recruitment during expiration can also offset diaphragmatic weakness (61). Although the study design did not include diaphragmatic ultrasound, this approach could help distinguish contributions of the respiratory muscles during tidal breathing and maximal efforts. Our methods did not permit us to make discrete physiological comparisons between the diaphragm and accessory muscle activity, but the issue warrants further investigation.
While longitudinal assessments were conducted in the research lab whenever possible, most participants lacked the physical capacity to travel extended distances for follow-up. Moreover, some did not enroll into the study until after the DP implantation or were unavailable for regular home visits. These complications led to the inability to collect all measurements at least annually in every participant.
5. Conclusions
In conclusion, the results are consistent with our hypothesis that DP has the potential to augment ventilation in those with chronic ventilator failure due to Pompe disease. Recordings from long-term indwelling pacing electrodes provided further physiologic insights into diaphragm activity, for up to 7 years post-DP. In a multisystem, progressive condition such as Pompe disease, a multi-faceted approach is required to optimize function and improve the lived experiences of patients. DP may be an additional option beyond routine clinical care, particularly for those who are ineligible for other emerging treatments, such as next-generation enzyme replacement therapies or gene therapy. It is recommended that assessments of unassisted tidal volumes be included in preoperative evaluations of those under consideration for DP. While no firm conclusions can be drawn, diaphragm pacing may be considered as an adjunct in the treatment of this rare, debilitating condition.
Data availability statement
The raw data supporting the conclusions of this article will be made available by the authors, without undue reservation.
Ethics statement
The studies involving human participants were reviewed and approved by University of Florida IRB-01. Written informed consent to participate in this study was provided by the participants’ legal guardian/next of kin. Written informed consent was obtained from the individual(s), and minor(s)' legal guardian/next of kin, for the publication of any potentially identifiable images or data included in this article.
Author contributions
BS, BB, DF, CC, and TP: contributed to conception and design of the study. JE: organized the database. CL, JE, and BS: performed data collection and analysis. CL: wrote the first draft of the manuscript. CL, JE, CL-A, and BS: wrote sections of the manuscript. CC, CL-A, and BS: synthesized the case summaries in the supplement. All authors contributed to the article and approved the submitted version.
Funding
The project was funded by the Eunice Kennedy Shriver National Institute of Child Health and Human Development (Grant no. R21HD090752). Early pilot work was supported by a grant from the Acid Maltase Deficiency Association.
Conflict of interest
The authors declare that the research was conducted in the absence of any commercial or financial relationships that could be construed as a potential conflict of interest.
Publisher's note
All claims expressed in this article are solely those of the authors and do not necessarily represent those of their affiliated organizations, or those of the publisher, the editors and the reviewers. Any product that may be evaluated in this article, or claim that may be made by its manufacturer, is not guaranteed or endorsed by the publisher.
Supplementary material
The Supplementary Material for this article can be found online at: https://www.frontiersin.org/articles/10.3389/fresc.2023.1184031/full#supplementary-material
References
1. Raben N, Plotz P, Byrne BJ. Acid alpha-glucosidase deficiency (glycogenosis type II, pompe disease). Curr Mol Med. (2002) 2(2):145–66. doi: 10.2174/1566524024605789
2. Hers HG. α-Glucosidase deficiency in generalized glycogenstorage disease (pompe’s disease). Biochem J. (1963) 86(1):11–6. doi: 10.1042/bj0860011
3. DeRuisseau LR, Fuller DD, Qiu K, DeRuisseau KC, Donnelly WH Jr, Mah C, et al. Neural deficits contribute to respiratory insufficiency in pompe disease. Proc Natl Acad Sci U S A. (2009) 106(23):9419–24. doi: 10.1073/pnas.0902534106
4. Fuller DD, Trejo-Lopez JA, Yachnis AT, Sunshine MD, Rana S, Bindi VE, et al. Case studies in neuroscience: neuropathology and diaphragm dysfunction in ventilatory failure from late-onset pompe disease. J Neurophysiol. (2021) 126(2):351–60. doi: 10.1152/jn.00190.2021
5. Shea L, Raben N. Autophagy in skeletal muscle: implications for pompe disease. Int J Clin Pharmacol Ther. (2009) 47(Suppl 1):S42–7. doi: 10.5414/cpp47042
6. Falk DJ, Todd AG, Lee S, Soustek MS, ElMallah MK, Fuller DD, et al. Peripheral nerve and neuromuscular junction pathology in pompe disease. Hum Mol Genet. (2015) 24(3):625–36. doi: 10.1093/hmg/ddu476
7. Pellegrini N, Laforet P, Orlikowski D, Pellegrini M, Caillaud C, Eymard B, et al. Respiratory insufficiency and limb muscle weakness in adults with pompe’s disease. Eur Respir J. (2005) 26(6):1024–31. doi: 10.1183/09031936.05.00020005
8. Kobayashi H, Shimada Y, Ikegami M, Kawai T, Sakurai K, Urashima T, et al. Prognostic factors for the late onset pompe disease with enzyme replacement therapy: from our experience of 4 cases including an autopsy case. Mol Genet Metab. (2010) 100(1):14–9. doi: 10.1016/j.ymgme.2010.01.015
9. van Gelder CM, van Capelle CI, Ebbink BJ, Moor-van Nugteren I, van den Hout JM, Hakkesteegt MM, et al. Facial-muscle weakness, speech disorders and dysphagia are common in patients with classic infantile pompe disease treated with enzyme therapy. J Inherit Metab Dis. (2012) 35(3):505–11. doi: 10.1007/s10545-011-9404-7
10. Van der Beek NA, Hagemans ML, Reuser AJ, Hop WC, Van der Ploeg AT, Van Doorn PA, et al. Rate of disease progression during long-term follow-up of patients with late-onset pompe disease. Neuromuscul Disord. (2009) 19(2):113–7. doi: 10.1016/j.nmd.2008.11.007
11. Kishnani PS, Hwu WL, Mandel H, Nicolino M, Yong F, Corzo D. A retrospective, multinational, multicenter study on the natural history of infantile-onset pompe disease. J Pediatr. (2006) 148(5):671–6. doi: 10.1016/j.jpeds.2005.11.033
12. Berger KI, Chan Y, Rom WN, Oppenheimer BW, Goldring RM. Progression from respiratory dysfunction to failure in late-onset pompe disease. Neuromuscul Disord. (2016) 26(8):481–9. doi: 10.1016/j.nmd.2016.05.018
13. De Vito EL, Monteiro SG, Aruj PK. Blunted hypercapnic respiratory drive response in subjects with late-onset pompe disease. Respir Care. (2016) 61(7):930–5. doi: 10.4187/respcare.03940
14. Gaeta M, Musumeci O, Mondello S, Ruggeri P, Montagnese F, Cucinotta M, et al. Clinical and pathophysiological clues of respiratory dysfunction in late-onset pompe disease: new insights from a comparative study by MRI and respiratory function assessment. Neuromuscul Disord. (2015) 25(11):852–8. doi: 10.1016/j.nmd.2015.09.003
15. Prigent H, Orlikowski D, Laforet P, Letilly N, Falaize L, Pellegrini N, et al. Supine volume drop and diaphragmatic function in adults with pompe disease. Eur Respir J. (2012) 39(6):1545–6. doi: 10.1183/09031936.00169011
16. Spiesshoefer J, Henke C, Kabitz HJ, Brix T, Gorlich D, Herkenrath S, et al. The nature of respiratory muscle weakness in patients with late-onset pompe disease. Neuromuscul Disord. (2019) 29(8):618–27. doi: 10.1016/j.nmd.2019.06.011
17. van der Ploeg AT, Barohn R, Carlson L, Charrow J, Clemens PR, Hopkin RJ, et al. Open-label extension study following the late-onset treatment study (LOTS) of alglucosidase alfa. Mol Genet Metab. (2012) 107(3):456–61. doi: 10.1016/j.ymgme.2012.09.015
18. Ditters IAM, Huidekoper HH, Kruijshaar ME, Rizopoulos D, Hahn A, Mongini TE, et al. Effect of alglucosidase alfa dosage on survival and walking ability in patients with classic infantile pompe disease: a multicentre observational cohort study from the European pompe consortium. Lancet Child Adolesc Health. (2022) 6(1):28–37. doi: 10.1016/S2352-4642(21)00308-4
19. Sarah B, Giovanna B, Emanuela K, Nadi N, Jose V, Alberto P. Clinical efficacy of the enzyme replacement therapy in patients with late-onset pompe disease: a systematic review and a meta-analysis. J Neurol. (2022) 269(2):733–41. doi: 10.1007/s00415-021-10526-5
20. Diaz-Manera J, Kishnani PS, Kushlaf H, Ladha S, Mozaffar T, Straub V, et al. Safety and efficacy of avalglucosidase alfa versus alglucosidase alfa in patients with late-onset pompe disease (COMET): a phase 3, randomised, multicentre trial. The Lancet Neurology. (2021) 20(12):1012–26. doi: 10.1016/S1474-4422(21)00241-6
21. Mead J, Loring SH. Analysis of volume displacement and length changes of the diaphragm during breathing. J Appl Physiol Respir Environ Exerc Physiol. (1982) 53(3):750–5. doi: 10.1152/jappl.1982.53.3.750
22. Boentert M, Drager B, Glatz C, Young P. Sleep-disordered breathing and effects of noninvasive ventilation in patients with late-onset pompe disease. J Clin Sleep Med. (2016) 12(12):1623–32. doi: 10.5664/jcsm.6346
23. Boentert M, Karabul N, Wenninger S, Stubbe-Drager B, Mengel E, Schoser B, et al. Sleep-related symptoms and sleep-disordered breathing in adult pompe disease. Eur J Neurol. (2015) 22(2):369–76, e27. doi: 10.1111/ene.12582
24. Gonzalez R, Bustinza A, Fernandez SN, Garcia M, Rodriguez S, Garcia-Teresa MA, et al. Quality of life in home-ventilated children and their families. Eur J Pediatr. (2017) 176(10):1307–17. doi: 10.1007/s00431-017-2983-z
25. Huttmann SE, Magnet FS, Karagiannidis C, Storre JH, Windisch W. Quality of life and life satisfaction are severely impaired in patients with long-term invasive ventilation following ICU treatment and unsuccessful weaning. Ann Intensive Care. (2018) 8(1):38. doi: 10.1186/s13613-018-0384-8
26. Diaz R, Heller D. Barotrauma and mechanical ventilation. In: Statpearls. Treasure Island, FL: StatPearls Publishing (2022).
27. Busl KM, Ouyang B, Boland TA, Pollandt S, Temes RE. Prolonged mechanical ventilation is associated with pulmonary complications, increased length of stay, and unfavorable discharge destination among patients with subdural hematoma. J Neurosurg Anesthesiol. (2015) 27(1):31–6. doi: 10.1097/ANA.0000000000000085
28. Goligher EC, Dres M, Fan E, Rubenfeld GD, Scales DC, Herridge MS, et al. Mechanical ventilation-induced diaphragm atrophy strongly impacts clinical outcomes. Am J Respir Crit Care Med. (2018) 197(2):204–13. doi: 10.1164/rccm.201703-0536OC
29. Hussain SN, Cornachione AS, Guichon C, Al Khunaizi A, Leite Fde S, Petrof BJ, et al. Prolonged controlled mechanical ventilation in humans triggers myofibrillar contractile dysfunction and myofilament protein loss in the diaphragm. Thorax. (2016) 71(5):436–45. doi: 10.1136/thoraxjnl-2015-207559
30. van den Berg M, Hooijman PE, Beishuizen A, de Waard MC, Paul MA, Hartemink KJ, et al. Diaphragm atrophy and weakness in the absence of mitochondrial dysfunction in the critically ill. Am J Respir Crit Care Med. (2017) 196(12):1544–58. doi: 10.1164/rccm.201703-0501OC
31. Onders RP, Elmo M, Kaplan C, Katirji B, Schilz R. Extended use of diaphragm pacing in patients with unilateral or bilateral diaphragm dysfunction: a new therapeutic option. Surgery. (2014) 156(4):776–84. doi: 10.1016/j.surg.2014.07.021
32. Romero FJ, Gambarrutta C, Garcia-Forcada A, Marin MA, Diaz de la Lastra E, Paz F, et al. Long-term evaluation of phrenic nerve pacing for respiratory failure due to high cervical spinal cord injury. Spinal Cord. (2012) 50(12):895–8. doi: 10.1038/sc.2012.74
33. Wijkstra PJ, van der Aa H, Hofker HS, Curto F, Giacomini M, Stagni G, et al. Diaphragm pacing in patients with spinal cord injury: a European experience. Respiration. (2022) 101(1):18–24. doi: 10.1159/000517401
34. Smith BK, Fuller DD, Martin AD, Lottenberg L, Islam S, Lawson LA, et al. Diaphragm pacing as a rehabilitative tool for ventilator-dependent pompe disease: case series. Phys Ther. (2016) 96(5):696–703. doi: 10.2522/ptj.20150122
35. MacIntyre NR, Epstein SK, Carson S, Scheinhorn D, Christopher K, Muldoon S. Management of patients requiring prolonged mechanical ventilation: report of a NAMDRC consensus conference. Chest. (2005) 128(6):3937–54. doi: 10.1378/chest.128.6.3937
36. Onders RP, Dimarco AF, Ignagni AR, Aiyar H, Mortimer JT. Mapping the phrenic nerve motor point: the key to a successful laparoscopic diaphragm pacing system in the first human series. Surgery. (2004) 136(4):819–26. doi: 10.1016/j.surg.2004.06.030
37. Kerwin AJ, Zuniga YD, Yorkgitis BK, Mull J, Hsu AT, Madbak FG, et al. Diaphragm pacing improves respiratory mechanics in acute cervical spinal cord injury. J Trauma Acute Care Surg. (2020) 89(3):423–8. doi: 10.1097/TA.0000000000002809
38. ATS/ERS statement on respiratory muscle testing. Am J Respir Crit Care Med. (2002) 166(4):518–624. doi: 10.1164/rccm.166.4.518
39. Brennan M, McDonnell MJ, Duignan N, Gargoum F, Rutherford RM. The use of cough peak flow in the assessment of respiratory function in clinical practice- A narrative literature review. Respir Med. (2022) 193:106740. doi: 10.1016/j.rmed.2022.106740
40. LoMauro A, Aliverti A. Respiratory muscle activation and action during voluntary cough in healthy humans. J Electromyogr Kinesiol. (2019) 49:102359. doi: 10.1016/j.jelekin.2019.102359
41. Truwit JD, Marini JJ. Validation of a technique to assess maximal inspiratory pressure in poorly cooperative patients. Chest. (1992) 102(4):1216–9. doi: 10.1378/chest.102.4.1216
42. Liang F, Li T, Azuelos I, Giordano C, Liang H, Hussain SN, et al. Ventilator-induced diaphragmatic dysfunction in mdx mice. Muscle Nerve. (2017) 57(3):442–8. doi: 10.1002/mus.25760
43. Smuder AJ, Gonzalez-Rothi EJ, Kwon OS, Morton AB, Sollanek KJ, Powers SK, et al. Cervical spinal cord injury exacerbates ventilator-induced diaphragm dysfunction. J Appl Physiol. (2016) 120(2):166–77. doi: 10.1152/japplphysiol.00488.2015
44. Onders RP, Elmo M, Kaplan C, Schilz R, Katirji B, Tinkoff G. Long-term experience with diaphragm pacing for traumatic spinal cord injury: early implantation should be considered. Surgery. (2018) 164(4):705–11. doi: 10.1016/j.surg.2018.06.050
45. Le Pimpec-Barthes F, Gonzalez-Bermejo J, Hubsch JP, Duguet A, Morelot-Panzini C, Riquet M, et al. Intrathoracic phrenic pacing: a 10-year experience in France. J Thorac Cardiovasc Surg. (2011) 142(2):378–83. doi: 10.1016/j.jtcvs.2011.04.033
46. Posluszny JA Jr, Onders R, Kerwin AJ, Weinstein MS, Stein DM, Knight J, et al. Multicenter review of diaphragm pacing in spinal cord injury: successful not only in weaning from ventilators but also in bridging to independent respiration. J Trauma Acute Care Surg. (2014) 76(2):303–9; discussion 9–10. doi: 10.1097/TA.0000000000000112
47. Hirschfeld S, Exner G, Luukkaala T, Baer GA. Mechanical ventilation or phrenic nerve stimulation for treatment of spinal cord injury-induced respiratory insufficiency. Spinal Cord. (2008) 46(11):738–42. doi: 10.1038/sc.2008.43
48. Chang GY, Salazar T, Karnwal A, Kun SS, Ellashek J, Shin CE, et al. Perioperative outcomes and the effects of anesthesia in congenital central hypoventilation patients. Sleep Breath. (2022) 27(2):505–10. doi: 10.1007/s11325-022-02632-z
49. Edmiston TL, Elrick MJ, Kovler ML, Jelin EB, Onders RP, Sadowsky CL. Early use of an implantable diaphragm pacing stimulator for a child with severe acute flaccid myelitis-a case report. Spinal Cord Ser Cases. (2019) 5:67. doi: 10.1038/s41394-019-0207-7
50. Gonzalez-Bermejo J, LLontop C, Similowski T, Morelot-Panzini C. Respiratory neuromodulation in patients with neurological pathologies: for whom and how? Ann Phys Rehabil Med. (2015) 58(4):238–44. doi: 10.1016/j.rehab.2015.07.001
51. Onders RP, Khansarinia S, Ingvarsson PE, Road J, Yee J, Dunkin B, et al. Diaphragm pacing in spinal cord injury can significantly decrease mechanical ventilation in multicenter prospective evaluation. Artif Organs. (2022) 46(10):1980–7. doi: 10.1111/aor.14221
52. Lepore AC, Tolmie C, O'Donnell J, Wright MC, Dejea C, Rauck B, et al. Peripheral hyperstimulation alters site of disease onset and course in SOD1 rats. Neurobiol Dis. (2010) 39(3):252–64. doi: 10.1016/j.nbd.2010.03.021
53. Huff A, Reed MD, Smith BK, Brown EH Jr, Ovechkin AV, Pitts T. Strategies for the integration of cough and swallow to maintain airway protection in humans. Lung. (2018) 196(5):601–8. doi: 10.1007/s00408-018-0133-7
54. Kansagra S, Austin S, DeArmey S, Kazi Z, Kravitz RM, Kishnani PS. Longitudinal polysomnographic findings in infantile pompe disease. Am J Med Genet A. (2015) 167A(4):858–61. doi: 10.1002/ajmg.a.37007
55. Kerwin AJ, Diaz Zuniga Y, Yorkgitis BK, Mull J, Hsu AT, Madbak FG, et al. Diaphragm pacing decreases hospital charges for patients with acute cervical spinal cord injury. Trauma Surg Acute Care Open. (2020) 5(1):e000528. doi: 10.1136/tsaco-2020-000528
56. Mizuno M, Secher NH. Histochemical characteristics of human expiratory and inspiratory intercostal muscles. J Appl Physiol. (1989) 67(2):592–8. doi: 10.1152/jappl.1989.67.2.592
57. Glenn WW, Hogan JF, Loke JS, Ciesielski TE, Phelps ML, Rowedder R. Ventilatory support by pacing of the conditioned diaphragm in quadriplegia. N Engl J Med. (1984) 310(18):1150–5. doi: 10.1056/NEJM198405033101804
58. Mantilla CB, Seven YB, Sieck GC. Convergence of pattern generator outputs on a common mechanism of diaphragm motor unit recruitment. Prog Brain Res. (2014) 209:309–29. doi: 10.1016/B978-0-444-63274-6.00016-3
59. van den Berg LE, Drost MR, Schaart G, de Laat J, van Doorn PA, van der Ploeg AT, et al. Muscle fiber-type distribution, fiber-type-specific damage, and the pompe disease phenotype. J Inherit Metab Dis. (2013) 36(5):787–94. doi: 10.1007/s10545-012-9541-7
60. Schoser BG, Muller-Hocker J, Horvath R, Gempel K, Pongratz D, Lochmuller H, et al. Adult-onset glycogen storage disease type 2: clinico-pathological phenotype revisited. Neuropathol Appl Neurobiol. (2007) 33(5):544–59. doi: 10.1111/j.1365-2990.2007.00839.x
Keywords: Pompe disease, diaphragm, mechanical ventilation, breathing, phrenic stimulation
Citation: Liberati C, Byrne BJ, Fuller DD, Croft C, Pitts T, Ehrbar J, Leon-Astudillo C and Smith BK (2023) Diaphragm pacing and independent breathing in individuals with severe Pompe disease. Front. Rehabil. Sci. 4:1184031. doi: 10.3389/fresc.2023.1184031
Received: 10 March 2023; Accepted: 20 July 2023;
Published: 31 July 2023.
Edited by:
Rich S. Severin, University of Illinois at Chicago, United StatesReviewed by:
Abdallah Fayssoil, Hôpital Raymond-Poincaré, FranceMirco Lusuardi, IRCCS Local Health Authority of Reggio Emilia, Italy
Antonio Toscano, University of Messina, Italy
© 2023 Liberati, Byrne, Fuller, Croft, Pitts, Ehrbar, Leon-Astudillo and Smith. This is an open-access article distributed under the terms of the Creative Commons Attribution License (CC BY). The use, distribution or reproduction in other forums is permitted, provided the original author(s) and the copyright owner(s) are credited and that the original publication in this journal is cited, in accordance with accepted academic practice. No use, distribution or reproduction is permitted which does not comply with these terms.
*Correspondence: Barbara K. Smith bksmith@ufl.edu