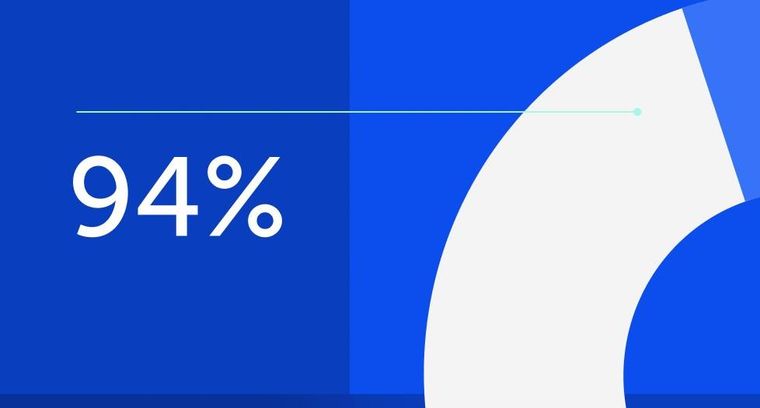
94% of researchers rate our articles as excellent or good
Learn more about the work of our research integrity team to safeguard the quality of each article we publish.
Find out more
MINI REVIEW article
Front. Rehabil. Sci., 24 September 2021
Sec. Translational Research in Rehabilitation
Volume 2 - 2021 | https://doi.org/10.3389/fresc.2021.724003
This article is part of the Research TopicHarnessing Neuroplasticity in the Injured Central Nervous System using Spinal NeuromodulationView all 5 articles
Transcutaneous Spinal Cord Stimulation (TSCS) has been shown to enhance the excitability of spinal neural circuits. This excitation is associated with enhanced voluntary performance in patients with incomplete SCI (iSCI). Though there is much we do not know, combining this altered state of exciability with therapy has the potential to enhance the outcomes associated with activity-based interventions. It is a promising tool to augment the work being done in therapeutic settings with the potential to expedite recovery. There is, however, a lag in assimilating the science for clinical practice. This article will examine current literature related to the application of TSCS in combination with therapeutic interventions for motor recovery and aims to elucidate trends in waveform selection, duration and frequency, and combinatorial therapies that may inform clinical practice. With specific consideration for therapeutic settings, potential benefits, applications, and pitfalls for clinical use are considered. Finally, the next steps in research to move toward wider clinical utility are discussed.
The disruption of transmission of motor and sensory information associated with spinal cord injury (SCI) significantly impacts a person's ability to purposely move. Nearly 70% of new injuries each year are incomplete with incomplete tetraplegia as the fastest growing injury classification (1). Many of these patients will present with motor dysfunction (2). Recovery of hand and walking functions are a high priority among individuals incomplete SCI (iSCI) and a common target of physical therapy (3).
Compensation strategies used early in traditional rehabilitation provide patients with some level of independence, but focus primarily on strong reliable movements (2). These strategies ignore the potential for recovery of function and lack the input to the central nervous system (CNS) required to induce change (4). At best, traditional rehabilitation strategies artificially limit patients' functional status, at worst they lead to progressive loss of latent function and worsening disability over time (5). As patients with SCI are living longer and more active lives, there is a desire to return to more normal function thereby reducing secondary complications and accessibility barriers, which are costly in money, time, and quality of life. Driven by patient demands and emerging evidence on the recovery and repair of CNS damage, there is a rehabilitation paradigm shift toward return to pre-injury function (2, 6, 7). Activity-based therapy (ABT), which provides intensive, high repetition training, is considered the most effective intervention to improve walking function following iSCI (8–11). Providing high-volume, task-specific training to both improve the kinematics of walking and upregulate CNS activity, locomotor training has been shown to yield clinically meaningful improvements in gait speed, endurance, balance, and lower extremity strength (2, 12–14). However, even with these improvements, significant deficits in walking function persist and recovery of independent walking remains elusive for most patients with iSCI. Similarly, upper extremity interventions lack the consistency and durable changes associated with meaningful recovery.
The ABT evidence highlights the need for increased activation of the CNS to induce change in motor function (4, 11). Spinal cord stimulation was offered by researchers as an option to provide neuromodulatory input to potentiate gains achieved through physical rehabilitation interventions alone (15). Implanted lumbosacral epidural spinal cord stimulation (eSCS) (15–18), which acts by directly stimulating the dorsal nerve roots to increase the excitability of interneuronal networks involved in the control of locomotion, was explored first in animals and then human models (16, 18–20). Researchers demonstrated that lumbosacral epidural stimulation alone can facilitate reciprocal, step-like movements, and when used in combination with intensive locomotor training can lead to improved walking abilities (16, 20–23). Researchers have demonstrated that after long-term eSCS with training in chronic, complete SCI, recovery of volitional movement is possible, even in the absence of stimulation (24).
While impact of these improvements on quality of life for patients with SCI cannot be underestimated, the true functional impact and durability of these improvements remains to be seen. eSCS also carries some inherent risk, notably invasive surgical placement of the stimulator (18, 20, 21, 25, 26). Additionally, there is a large variability in the stimulation parameters and rehabilitation protocols reported post-implantation, ranging from 0 to 85 weeks of intensive training (16, 20, 27). Though all participants showed improvement in voluntary motor control, not all recovered durable walking function, nor sustained usage of the implant (16, 20, 24).
Transcutaneous spinal cord stimulation (TSCS) has the potential to impact CNS excitability and, when paired with training, create functional changes in patients with iSCI, that may be comparable to results of eSCS (28). TSCS represents a promising, clinically useful adjunct to existing physical rehabilitation interventions, without the risk and accessibility issues associated with surgical implantation for eSCS.
The purpose of this mini review is to elucidate trends and discuss the clinical relevance of TSCS as an adjunct to physical rehabilitation interventions. A PubMed search was conducted with the following search terms in all fields: transcutaneous spinal cord stimulation AND spinal cord injury AND rehabilitation. Articles were limited to the last 5 years (2016–2021) and English language. Articles that included exclusive examination of animal experiments, other diagnoses (ex: multiple sclerosis and cerebral palsy), and autonomic and non-voluntary functions (ex: bladder) were excluded. The discussion here is limited to studies with clinical relevance, as such, study of healthy persons and description of isolated neurophysiologic charges are also excluded. One hundred fifteen abstracts were screened, 13 articles were reviewed in full and are included here. Table 1 includes key study elements and a brief description of the results of each reference. This mini review aims to build upon the work of previous systematic reviews (41) of the topic by revealing trends meaningful for clinical settings.
Via computational modeling and human EMG studies, non-invasive spinal cord stimulation has been shown to increase excitability of local spinal networks via dorsal root afferents with additional signal enhancement along the full length of the spinal cord (34, 42–45). This change in excitability capitalizes on functionally silent descending pathways to unmask and enhance voluntary movements of involved limbs (34, 44, 45). The priming of the nervous system offered via TSCS could augment existing physical rehabilitation interventions and has shown promise in many common therapeutic targets. TSCS, both in single sessions and repeated applications, is associated with improved standing postural control, gait kinematics, and upper extremity function. TSCS has also been demonstrated to have an impact on autonomic and non-voluntary functions (blood pressure regulation, bladder function, etc.) but those will not be discussed here (28, 34, 36, 46). Therapists should be aware that these may be consequences of interventions targeting motor recovery.
Early reports of TSCS demonstrate involuntary stepping with both single-session and repeated-exposure stimulation (45). Stimulation was delivered via electrodes over the T11/T12 or L1/L2 intervertebral space with anodes over bilateral iliac crest. The waveform consisted of 1 millisecond (msec) pulses with a frequency of 5–30 Hz, filled by a 10 kHz carrier frequency. Participants placed in a side-lying gravity eliminated position and stimulated as described exhibited oscillatory, step-like movements without voluntary effort. Participants demonstrated greater amplitude hip and knee oscillations when stimulation was combined with voluntary effort and with the addition of a second coccygeal stimulation site. Notably, this effect was demonstrated in uninjured, chronic complete and incomplete SCI. This work demonstrates that spinal neural circuits can be altered through spinal stimulation, supraspinal inputs (voluntary effort), and a combination of the two. This allows the field to shift from isolated stimulation to stimulation with training for recovery of voluntary effort.
In 2018, 15 participants with chronic SCI demonstrated recovered ability to maintain upright standing with minimal to no external assistance, following TSCS and training (34). Stimulation paradigms matched those described above (45), notably with use of carrier frequency. Participants showed decreased motor activation threshold, increased lower extremity (LE) muscle activity via surface EMG, and improved weight acceptance during TSCS. Without stimulation, none of the participants were able to support themselves in upright. None of the participants reported pain associated with stimulation or adverse events during the course of the study. Participants reported changes in spasticity, proprioception, and mood, which were not quantified. The authors note the relative speed of skill acquisition, as compared to eSCS studies (18, 20), suggesting that TSCS may have a broader modulatory impact on neural networks and multisegmental projections.
Using the same stimulation parameters with task-specific training, benefits to trunk control (33) and ankle mobility (37) have been reported in participants with both complete and incomplete SCI. Participants improve performance with stimulation only and no maintenance of change without stimulation are reported. Here, again, the speed of skill acquisition is highlighted by the authors.
Exploration of walking functions with TSCS include a wide variety of interventions including over-ground, treadmill-based, and robotic-assisted studies. In a single case report (29), a participant with motor complete SCI underwent training in an exoskeleton with TSCS that included a carrier frequency. In a larger case series (38), participants were provided similar exoskeleton-based training with TSCS without a carrier frequency. The benefits reported were consistent across the two studies including improved voluntary control, coordination, and weight acceptance during stepping. In addition to the differing waveforms, authors of respective studies explored the impact of frequency on spasticity interrupting smooth gait. Higher frequencies were found to have the most benefit on spasticity (38).
Studies of over-ground and treadmill-based walk training with TSCS show similar improvements in gait (35, 36, 40). First, ten participants with iSCI received 23 sessions of TSCS with walking-based physical therapy (36). Here stimulation was delivered with a commercially available, clinically relevant stimulator using a biphasic waveform with 1 ms pulses at 50 Hz. Electrode placement was consistent with previous studies. Authors report that statistically significant improvements in walking speed, endurance, and quality, with changes exceeding individual test minimal clinically important difference (MCID) at or before sessions 18. Again, it is highlighted that TSCS yield functionally important improvements in shorter time frames than traditional models of care or with eSCS. As an adjunct to this work, another group published the results of an experiment comparing TSCS with locomotor training to sham stimulation with locomotor training (40). Biphasic stimulation was again provided with a commercially available stimulator. Participants receiving the experimental stimulation improved their walking speed and distance. No adverse events or protocol deviations due to pain were reported in either paper. Authors of both groups endorse the feasibility of TSCS in clinical settings. In a single case report (35), TSCS including a carrier frequency with the same electrode placement was applied during standing, treadmill walking, and strengthening activities. Authors report recovery of volitional movement and functional skills, which were maintained over 6 weeks without stimulation. The report of more durable changes indicates the addition of TSCS may contribute to recovery of function, rather than a transient state of hyperexcitability.
Improvements in upper extremity (UE) function are also reported in relation to TSCS. In single-case reports (32, 39), case series (31) and prospective cohorts (28, 30) of subjects with motor incomplete SCI, sustained recovery of arm and hand movement is reported. In all of these reports stimulation was delivered in 1 ms pulses at 5–30 Hz, with a carrier frequency of 10 kHz. Electrode placement included one or more cervical intervertebral space, sometimes surrounding the level of injury. Anodes remain over the iliac crest. Interventions varied widely, as is common in UE exploration, with more focused training in grasp and release, as compared to other patterns of prehension. As with lower extremity and walking outcomes, the magnitude and speed of changes associated with TSCS, as compared to traditional rehabilitation is highlighted. Grip strength and functional dexterity show improvement with TSCS and, in several cases, improvements are shown to be durable over time without continued stimulation. TSCS is also reported to have a benefit on spasticity management in the UE (28).
With all the evidence and apparent utility in relation to therapeutic targets, it stands to wonder why TSCS does not have greater clinical deployment. The first obvious issue is lack of consensus on stimulation parameters. Most studies use low frequencies (30–50 Hz) (36, 40) but some add a carrier frequency (34, 45), claiming this makes the stimulation pain free. Proponents claim the carrier frequency selectively blocks transmission of pain information and lowers tissue impedance for deeper penetration of stimulation (47). However, direct comparison of a traditional a symmetrical biphasic waveform and a waveform with carrier frequency found no significant difference in intensity necessary for motor activation and participants' subjective reports of discomfort was equal across the stimulation paradigms (48). Largely the field agrees that electrode placement determines current direction and consequently motor targets (i.e., cervical placements for UE interventions). Without large-scale controlled trials, it is difficult to pick the most efficacious stimulation parameters. As a somewhat related issue, identifying the best responders and the need for individual adjustments in stimulation parameters is a gap in our collective knowledge. Existing evidence suggests that TSCS may be more useful in iSCI, but perhaps it also has a role in screening or conditioning patients with complete injury prior to eSCS. The availability of appropriate stimulation devices may also be an issue for successful deployment into therapeutic settings. Few studies use commercially available, clinically relevant stimulators (36, 40). Additionally, longitudinal study is warranted to more fully glean the impact of TSCS. We don't yet understand if the changes associated with the intervention are long lasting, or if patients will need to use neuromodulatory inputs regularly, like a vitamin or charging a battery, or only as needed, like an orthotic for walking. Finally, there are always the limitations and barriers associated with clinical services, including time, training, and reimbursement.
Even with all the unknowns, the data in support of TSCS is compelling. Though very few studies have demonstrated the use of TSCS in clinical settings, they have made a case for its utility and feasibility, demonstrating it to be a low burden, low risk adjunct to existing interventions (36, 40). When looking at the studies discussed here in aggregate, a few commonalities can be identified. Successful TSCS applications include long pulse duration (0.5 μs to 1 ms) and moderate frequencies (30–50 Hz). Electrode placements targeting upper and lower extremities are well-defined. There is no conclusive evidence that the carrier frequency is necessary. Outcomes between studies with and without carrier frequency are similar and in direct comparison, subject tolerance was not impacted by the carrier frequency. Carrier frequencies are not readily available in clinical stimulators and so, in their absence, clinicians may opt to pursue TSCS with standard biphasic waveforms. For the evidence to be advanced and conclusive decisions on parameters to be made, well-controlled, larger sample studies are needed. Given the heterogeneity and recruitment challenges associated with SCI, this may require multiple centers to come together, agreeing on study design, stimulation parameters, and outcomes.
Finally, there is likely benefit in extending the study of TSCS into other populations. Work has begun in children with iSCI and cerebral palsy (CP) (49, 50). Reports of decreased spasticity lends TSCS to other neurological diagnoses, like stroke (51) and multiple sclerosis (MS) (52), where dysregulation in the CNS leads to dysfunction and atrophy of spinal neural networks. The more populations in which TSCS is demonstrated as useful, the more attention and funding it will draw, ultimately leading to better acceptance in therapeutic settings.
Increasingly, patients are acutely aware of these developing and advancing interventions and seek clinics willing to offer them. Companies are stepping in to fill the technology void and so the responsibility falls to the therapists to decide who and how and when.
RM was the sole author of this article and responsible for all the work herein.
RM receives research support from the Orokawa Foundation and Niche BioMedical.
All claims expressed in this article are solely those of the authors and do not necessarily represent those of their affiliated organizations, or those of the publisher, the editors and the reviewers. Any product that may be evaluated in this article, or claim that may be made by its manufacturer, is not guaranteed or endorsed by the publisher.
I am grateful for the support and collaboration of our research team, notably Liza McHugh, DPT and Ashley Miller, DPT, and the mentorship of Cristina Sadowsky, MD and Amy Bastian, PT, PhD. None of my work is possible without the patients whom I serve and my want to offer them something more.
1. National Spinal Cord Injury Statistical Center Facts and Figures at a Glance. University of Alabama at Birminham. (2021). Available online at: https://www.nscisc.uab.edu/Public/Facts%20and%20Figures%20-%202021.pdf
2. Harkema S, Behrman A, Barbeau H. Chapter 16 - Evidence-based therapy for recovery of function after spinal cord injury. In: Verhaagen J, McDonald JW, editors. Handbook of Clinical Neurology [Internet]. Elsevier (2012).
3. Anderson KD. Targeting recovery: priorities of the spinal cord-injured population. J Neurotrauma. (2004) 21:1371–83. doi: 10.1089/neu.2004.21.1371
4. Lang CE, MacDonald JR, Reisman DS, Boyd L, Kimberley TJ, Schindler-Ivens SM, et al. Observation of amounts of movement practice provided during stroke rehabilitation. Arch Phys Med Rehabil. (2009) 90:1692–8. doi: 10.1016/j.apmr.2009.04.005
5. Allred RP, Maldonado MA, Hsu And JE, Jones TA. Training the “less-affected” forelimb after unilateral cortical infarcts interferes with functional recovery of the impaired forelimb in rats. Restor Neurol Neurosci. (2005) 23:297–302.
6. Behrman AL, Watson E, Fried G, D'Urso K, D'Urso D, Cavadini N, et al. Restorative rehabilitation entails a paradigm shift in pediatric incomplete spinal cord injury in adolescence: an illustrative case series. J Pediatr Rehabil Med. (2012) 5:245–59. doi: 10.3233/PRM-2012-00225
7. McDonald JW, Sadowsky CL, Stampas A. Chapter 20 - The changing field of rehabilitation: optimizing spontaneous regeneration and functional recovery. In: Verhaagen J, McDonald JW, editors. Handbook of Clinical Neurology. Elsevier (2012).
8. Field-Fote EC. Spinal cord control of movement: implications for locomotor rehabilitation following spinal cord injury. Phys Ther. (2000) 80:477–84. doi: 10.1093/ptj/80.5.477
9. Jones ML, Evans N, Tefertiller C, Backus D, Sweatman M, Tansey K, et al. Activity-based therapy for recovery of walking in individuals with chronic spinal cord injury: results from a randomized clinical trial. Arch Phys Med Rehabil. (2014) 95:2239–46.e2. doi: 10.1016/j.apmr.2014.07.400
10. Jones ML, Evans N, Tefertiller C, Backus D, Sweatman M, Tansey K, et al. Activity-based therapy for recovery of walking in chronic spinal cord injury: results from a secondary analysis to determine responsiveness to therapy. Arch Phys Med Rehabil. (2014) 95:2247–52. doi: 10.1016/j.apmr.2014.07.401
11. Dolbow DR, Gorgey AS, Recio AC, Stiens SA, Curry AC, Sadowsky CL, et al. Activity-based restorative therapies after spinal cord injury: inter-institutional conceptions and perceptions. Aging Dis. (2015) 6:254–61. doi: 10.14336/AD.2014.1105
12. Labruyère R, van Hedel HJA. Strength training versus robot-assisted gait training after incomplete spinal cord injury: a randomized pilot study in patients depending on walking assistance. J NeuroEngineering Rehabil. (2014) 11:4. doi: 10.1186/1743-0003-11-4
13. Morrison SA, Lorenz D, Eskay CP, Forrest GF, Basso DM. Longitudinal recovery and reduced costs after 120 sessions of locomotor training for motor incomplete spinal cord injury. Arch Phys Med Rehabil. (2018) 99:555–62. doi: 10.1016/j.apmr.2017.10.003
14. Harkema SJ, Hillyer J, Schmidt-Read M, Ardolino E, Sisto SA, Behrman AL. Locomotor training: as a treatment of spinal cord injury and in the progression of neurologic rehabilitation. Arch Phys Med Rehabil. (2012) 93:1588–97. doi: 10.1016/j.apmr.2012.04.032
15. Gerasimenko Y, Roy RR, Edgerton VR. Epidural stimulation: comparison of the spinal circuits that generate and control locomotion in rats, cats and humans. Exp Neurol. (2008) 209:417–25. doi: 10.1016/j.expneurol.2007.07.015
16. Gill ML, Grahn PJ, Calvert JS, Linde MB, Lavrov IA, Strommen JA, et al. Neuromodulation of lumbosacral spinal networks enables independent stepping after complete paraplegia. Nat Med. (2018) 24:1677–82. doi: 10.1038/s41591-018-0175-7
17. Mayr W, Krenn M, Dimitrijevic MR. Epidural and transcutaneous spinal electrical stimulation for restoration of movement after incomplete and complete spinal cord injury. Curr Opin Neurol. (2016) 29:721–6. doi: 10.1097/WCO.0000000000000382
18. Harkema S, Gerasimenko Y, Hodes J, Burdick J, Angeli C, Chen Y, et al. Effect of epidural stimulation of the lumbosacral spinal cord on voluntary movement, standing, and assisted stepping after motor complete paraplegia: a case study. Lancet. (2011) 377:1938–47. doi: 10.1016/S0140-6736(11)60547-3
19. Rattay F, Minassian K, Dimitrijevic M. Epidural electrical stimulation of posterior structures of the human lumbosacral cord: 2. quantitative analysis by computer modeling. Spinal Cord. (2000) 38:473–89. doi: 10.1038/sj.sc.3101039
20. Angeli CA, Boakye M, Morton RA, Vogt J, Benton K, Chen Y, et al. Recovery of over-ground walking after chronic motor complete spinal cord injury. N Engl J Med. (2018) 379:1244–50. doi: 10.1056/NEJMoa1803588
21. Wagner FB, Mignardot J-B, Le Goff-Mignardot CG, Demesmaeker R, Komi S, Capogrosso M, et al. Targeted neurotechnology restores walking in humans with spinal cord injury. Nature. (2018) 563:65–71. doi: 10.1038/s41586-018-0649-2
22. Minassian K, Jilge B, Rattay F, Pinter MM, Binder H, Gerstenbrand F, et al. Stepping-like movements in humans with complete spinal cord injury induced by epidural stimulation of the lumbar cord: electromyographic study of compound muscle action potentials. Spinal Cord. (2004) 42:401–16. doi: 10.1038/sj.sc.3101615
23. Carhart M, He J, Herman R, D'Luzansky S, Willis W. Epidural spinal-cord stimulation facilitates recovery of functional walking following incomplete spinal-cord injury. IEEE Trans Neural Syst Rehabil Eng. (2004) 12:32–42. doi: 10.1109/TNSRE.2003.822763
24. Peña Pino I, Hoover C, Venkatesh S, Ahmadi A, Sturtevant D, Patrick N, et al. Long-term spinal cord stimulation after chronic complete spinal cord injury enables volitional movement in the absence of stimulation. Front Syst Neurosci. (2020) 14:35. doi: 10.3389/fnsys.2020.00035
25. Herrity AN, Aslan SC, Ugiliweneza B, Mohamed AZ, Hubscher CH, Harkema SJ. Improvements in bladder function following activity-based recovery training with epidural stimulation after chronic spinal cord injury. Front Syst Neurosci. (2021) 14:614691. doi: 10.3389/fnsys.2020.614691
26. Bonizzato M, James ND, Pidpruzhnykova G, Pavlova N, Shkorbatova P, Baud L, et al. Multi-pronged neuromodulation intervention engages the residual motor circuitry to facilitate walking in a rat model of spinal cord injury. Nat Commun. (2021) 12:9. doi: 10.1038/s41467-021-22137-9
27. Darrow D, Balser D, Netoff TI, Krassioukov A, Phillips A, Parr A, et al. Epidural spinal cord stimulation facilitates immediate restoration of dormant motor and autonomic supraspinal pathways after chronic neurologically complete spinal cord injury. J Neurotrauma. (2019) 36:2325–36. doi: 10.1089/neu.2018.6006
28. Inanici F, Brighton LN, Samejima S, Hofstetter CP, Moritz CT. Transcutaneous spinal cord stimulation restores hand and arm function after spinal cord injury. IEEE Trans Neural Syst Rehabil Eng Publ IEEE Eng Med Biol Soc. (2021) 29:310–9. doi: 10.1109/TNSRE.2021.3049133
29. Gad P, Gerasimenko Y, Zdunowski S, Turner A, Sayenko D, Lu DC, et al. Weight bearing over-ground stepping in an exoskeleton with non-invasive spinal cord neuromodulation after motor complete paraplegia. Front Neurosci. (2017) 11:333. doi: 10.3389/fnins.2017.00333
30. Freyvert Y, Yong NA, Morikawa E, Zdunowski S, Sarino ME, Gerasimenko Y, et al. Engaging cervical spinal circuitry with non-invasive spinal stimulation and buspirone to restore hand function in chronic motor complete patients. Sci Rep. (2018) 8:15546. doi: 10.1038/s41598-018-33123-5
31. Gad P, Lee S, Terrafranca N, Zhong H, Turner A, Gerasimenko Y, et al. Non-invasive activation of cervical spinal networks after severe paralysis. J Neurotrauma. (2018) 35:2145–58. doi: 10.1089/neu.2017.5461
32. Inanici F, Samejima S, Gad P, Edgerton VR, Hofstetter CP, Moritz CT. Transcutaneous electrical spinal stimulation promotes long-term recovery of upper extremity function in chronic tetraplegia. IEEE Trans Neural Syst Rehabil Eng Publ IEEE Eng Med Biol Soc. (2018) 26:1272–8. doi: 10.1109/TNSRE.2018.2834339
33. Rath M, Vette AH, Ramasubramaniam S, Li K, Burdick J, Edgerton VR, et al. Trunk stability enabled by noninvasive spinal electrical stimulation after spinal cord injury. J Neurotrauma. (2018) 35:2540–53. doi: 10.1089/neu.2017.5584
34. Sayenko DG, Rath M, Ferguson AR, Burdick JW, Havton LA, Edgerton VR, et al. Self-assisted standing enabled by non-invasive spinal stimulation after spinal cord injury. J Neurotrauma. (2018) 36:1435–50. doi: 10.1089/neu.2018.5956
35. Alam M, Ling YT, Wong AYL, Zhong H, Edgerton VR, Zheng Y-P. Reversing 21 years of chronic paralysis via non-invasive spinal cord neuromodulation: a case study. Ann Clin Transl Neurol. (2020) 7:829–38. doi: 10.1002/acn3.51051
36. McHugh LV, Miller AA, Leech KA, Salorio C, Martin RH. Feasibility and utility of transcutaneous spinal cord stimulation combined with walking-based therapy for people with motor incomplete spinal cord injury. Spinal Cord Ser Cases. (2020) 6:104. doi: 10.1038/s41394-020-00359-1
37. Meyer C, Hofstoetter US, Hubli M, Hassani RH, Rinaldo C, Curt A, et al. Immediate effects of transcutaneous spinal cord stimulation on motor function in chronic, sensorimotor incomplete spinal cord injury. J Clin Med. (2020) 9:E3541. doi: 10.3390/jcm9113541
38. Shapkova EY, Pismennaya EV, Emelyannikov DV, Ivanenko Y. Exoskeleton walk training in paralyzed individuals benefits from transcutaneous lumbar cord tonic electrical stimulation. Front Neurosci. (2020) 14:416. doi: 10.3389/fnins.2020.00416
39. Zhang F, Momeni K, Ramanujam A, Ravi M, Carnahan J, Kirshblum S, et al. Cervical spinal cord transcutaneous stimulation improves upper extremity and hand function in people with complete tetraplegia: a case study. IEEE Trans Neural Syst Rehabil Eng Publ IEEE Eng Med Biol Soc. (2020) 28:3167–74. doi: 10.1109/TNSRE.2020.3048592
40. Estes S, Zarkou A, Hope JM, Suri C, Field-Fote EC. Combined transcutaneous spinal stimulation and locomotor training to improve walking function and reduce spasticity in subacute spinal cord injury: a randomized study of clinical feasibility and efficacy. J Clin Med. (2021) 10:61167. doi: 10.3390/jcm10061167
41. Megía García A, Serrano-Muñoz D, Taylor J, Avendaño-Coy J, Gómez-Soriano J. Transcutaneous spinal cord stimulation and motor rehabilitation in spinal cord injury: a systematic review. Neurorehabil Neural Repair. (2020) 34:3–12. doi: 10.1177/1545968319893298
42. Parazzini M, Fiocchi S, Liorni I, Rossi E, Cogiamanian F, Vergari M, et al. Modeling the current density generated by transcutaneous spinal direct current stimulation (tsDCS). Clin Neurophysiol. (2014) 125:2260–70. doi: 10.1016/j.clinph.2014.02.027
43. Fiocchi S, Ravazzani P, Priori A, Parazzini M. Cerebellar and spinal direct current stimulation in children: computational modeling of the induced electric field. Front Hum Neurosci. (2016) 10:522. doi: 10.3389/fnhum.2016.00522
44. Hofstoetter US, Krenn M, Danner SM, Hofer C, Kern H, McKay WB, et al. Augmentation of voluntary locomotor activity by transcutaneous spinal cord stimulation in motor-incomplete spinal cord-injured individuals: augmentation of locomotion by tSCS in incomplete SCI. Artif Organs. (2015) 39:E176–86. doi: 10.1111/aor.12615
45. Gerasimenko YP, Lu DC, Modaber M, Zdunowski S, Gad P, Sayenko DG, et al. Noninvasive reactivation of motor descending control after paralysis. J Neurotrauma. (2015) 32:1968–80. doi: 10.1089/neu.2015.4008
46. Phillips AA, Squair JW, Sayenko DG, Edgerton VR, Gerasimenko Y, Krassioukov AV. An autonomic neuroprosthesis: noninvasive electrical spinal cord stimulation restores autonomic cardiovascular function in individuals with spinal cord injury. J Neurotrauma. (2018) 35:446–51. doi: 10.1089/neu.2017.5082
47. Ward AR, Robertson VJ. Sensory, motor, and pain thresholds for stimulation with medium frequency alternating current. Arch Phys Med Rehabil. (1998) 79:273–8. doi: 10.1016/S0003-9993(98)90006-5
48. Manson GA, Calvert JS, Ling J, Tychhon B, Ali A, Sayenko DG. The relationship between maximum tolerance and motor activation during transcutaneous spinal stimulation is unaffected by the carrier frequency or vibration. Physiol Rep. (2020) 8:e14397. doi: 10.14814/phy2.14397
49. Nikityuk IE, Moshonkina TR, Gerasimenko YuP, Vissarionov SV, Baindurashvili AG. The regulation of balance in the children presenting with severe cerebral palsy following the treatment with the use of the locomotor training in combination with the electrical stimulation of leg muscles and spinal cord. Vopr Kurortol Fizioter Lech Fiz Kult. (2016) 93:23. doi: 10.17116/kurort2016523-27
50. Solopova IA, Sukhotina IA, Zhvansky DS, Ikoeva GA, Vissarionov SV, Baindurashvili AG, et al. Effects of spinal cord stimulation on motor functions in children with cerebral palsy. Neurosci Lett. (2017) 639:192–8. doi: 10.1016/j.neulet.2017.01.003
51. Moon Y, Zuleger T, Lamberti M, Bansal A, Mummidisetty CK, McKenzie KA, et al. Characterization of motor-evoked responses obtained with transcutaneous electrical spinal stimulation from the lower-limb muscles after stroke. Brain Sci. (2021) 11:289. doi: 10.3390/brainsci11030289
Keywords: spinal cord stimulation, spinal cord injury, rehabilitation, neuromodulation, ambulation
Citation: Martin R (2021) Utility and Feasibility of Transcutaneous Spinal Cord Stimulation for Patients With Incomplete SCI in Therapeutic Settings: A Review of Topic. Front. Rehabilit. Sci. 2:724003. doi: 10.3389/fresc.2021.724003
Received: 11 June 2021; Accepted: 19 August 2021;
Published: 24 September 2021.
Edited by:
Dimitry Sayenko, Houston Methodist Research Institute, United StatesReviewed by:
Darryn Atkinson, University of St. Augustine for Health Sciences, United StatesCopyright © 2021 Martin. This is an open-access article distributed under the terms of the Creative Commons Attribution License (CC BY). The use, distribution or reproduction in other forums is permitted, provided the original author(s) and the copyright owner(s) are credited and that the original publication in this journal is cited, in accordance with accepted academic practice. No use, distribution or reproduction is permitted which does not comply with these terms.
*Correspondence: Rebecca Martin, bWFydGlucmVAa2VubmVkeWtyaWVnZXIub3Jn
Disclaimer: All claims expressed in this article are solely those of the authors and do not necessarily represent those of their affiliated organizations, or those of the publisher, the editors and the reviewers. Any product that may be evaluated in this article or claim that may be made by its manufacturer is not guaranteed or endorsed by the publisher.
Research integrity at Frontiers
Learn more about the work of our research integrity team to safeguard the quality of each article we publish.