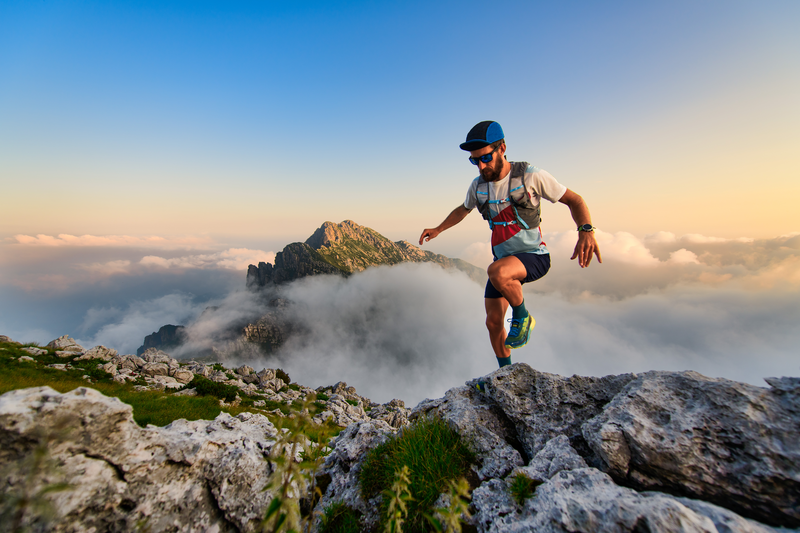
94% of researchers rate our articles as excellent or good
Learn more about the work of our research integrity team to safeguard the quality of each article we publish.
Find out more
ORIGINAL RESEARCH article
Front. Dement. , 13 March 2023
Sec. Aging and Risk Factors for Dementia
Volume 2 - 2023 | https://doi.org/10.3389/frdem.2023.1110553
Background: Brain-derived neurotrophic factor levels are higher in those who are physically active and lower in people with cognitive dysfunction. This study investigated whether brain-derived neurotrophic factor mediated or modified the association of sedentary time to MRI-estimated brain volumes in midlife.
Methods: Baseline (n = 612) and five-year follow-up (n = 418) data were drawn from the multicenter Coronary Artery Risk Development in Young Adults Brain MRI sub-study, including Black and White participants (aged 50.3 years, 51.6% females, 38.6% Black). Sedentary time (hours per day) was categorized into quartiles with low ≤ 4.3 (reference) and high > 8.4. Outcomes of the study were total brain, white matter, gray matter, hippocampal volumes, and white matter fractional anisotropy at baseline and 5-year percent change from baseline. The study used general linear regression models to examine the mediation and moderation effects of brain-derived neurotrophic factor (natural log transformed) on the associations of sedentary time to brain outcomes. The authors adjusted the regression model for age, sex, race, intracranial volume, education, and vascular factors.
Results: Cross-sectionally, baseline participants with the highest sedentary time had a lower total brain (−12.2 cc; 95%CI: −20.7, −3.7), gray matter (−7.8 cc; 95%CI: −14.3, −1.3), and hippocampal volume (−0.2 cc; 95%CI: −0.3, 0.0) compared with populations with the lowest sedentary time. The brain-derived neurotrophic factor levels did not mediate the associations between brain measures and sedentary time. Brain-derived neurotrophic factor was found to moderate associations of sedentary time to total brain and white matter volume such that the brain volume difference between high and low sedentary time decreased as brain-derived neurotrophic factor levels increased. Longitudinally, higher baseline brain-derived neurotrophic factor level was associated with less brain volume decline. The longitudinal associations did not differ by sedentary time, and brain-derived neurotrophic factor did not mediate or moderate the association of sedentary time to brain measure changes.
Conclusions: Higher brain-derived neurotrophic factor levels may buffer the negative effects of sedentary time on the brain.
Several studies suggest that high levels [e.g., >5 h per day or 3.0 metabolic equivalents (MET)] of physical activity (PA) are associated with healthier brain structures and functions in middle-aged (Chang et al., 2010; Hoang et al., 2016; Young et al., 2016) and older persons (Burzynska et al., 2014; Barha et al., 2019). The converse, sedentary behavior, such as television viewing or sitting (e.g., >8 h per day or energy expenditure of 1.5 MET or less), has been shown to be associated with lower brain volumes and poorer cognitive function than an active lifestyle (Launer et al., 2015; Hoang et al., 2016; Young et al., 2016).
Although an association between brain health and levels of PA has been reported, there are few studies identifying intermediaries (mediators) of this association, or factors that may change the direction of the association (moderators) (Kramer, 2021). Brain-Derived Neurotrophic Factor (BDNF) (Bekinschtein et al., 2007; Raz et al., 2009; Brown et al., 2014; Shimada et al., 2014; Weinstein et al., 2014) is a neurotrophic and neuroprotective protein that maintains brain health (Shimada et al., 2014; Weinstein et al., 2014; Li et al., 2020). Is BDNF a mediator or moderator? High levels of PA are associated with relatively higher BDNF plasma levels, which have a positive effect on brain structure and function (Vaynman et al., 2004; Raz et al., 2009; Brown et al., 2014). Likewise experimental studies have shown that blocking BDNF signaling pathways could inhibit the benefits of high activity levels on cognitive and hippocampal function in the memory domain (Vaynman et al., 2004; Bekinschtein et al., 2007; Korol et al., 2013). Additional evidence from genetic studies suggests levels of BDNF secreted from virus-infected neurons are regulated by the BDNF Val66 Met polymorphism and that higher levels of PA were associated with larger regional brain volumes and better cognitive function in Val/Val homozygotes than Met carriers and there were smaller regional volumes in Met carriers than Val/Val homozygotes (Egan et al., 2003; Brown et al., 2014; Takeuchi et al., 2020). Sedentary behavior connotates low levels of activity. There is variability of sedentary time (ST) in the population, and the amount of ST spent by an individual is associated with chronic disease independent of moderate to vigorous PA (Owen et al., 2010). For example, sitting for more than 10 h per day vs. < 5 h per day was associated with an increased risk of CVD among middle-aged women in the Women's Health Initiative population (Young et al., 2016).
Based on our previous finding of a relation between time spent in sedentary behaviors and total brain volume (TBV) (Launer et al., 2015), we investigated whether BDNF levels mediated (in the pathway of ST and brain measures) or moderated (interaction with ST) the ST—brain structure associations (Figure 1) in a cross-sectional and longitudinal analysis.
Figure 1. Hypothesis 1 (mediation) and Hypothesis 2 (moderation/interaction) model, CARDIA Brain-MRI sub-study. (a) BDNF, brain-derived neurotrophic factor; ST, sedentary time.
This study is based on Coronary Artery Risk Development in Young Adults study (CARDIA), a community-based longitudinal study of Black and White men and women aged 18–30 years at baseline, who had been followed up for 30 years by the time of this study and has previously been described in Friedman et al. (1988). The CARDIA brain MRI sub-study was started in the 25th year (Y25) of follow-up and repeated in the 30th year (Y30) of follow-up (Launer et al., 2015). Briefly, the sub-study aimed to characterize the morphology, pathology, physiology, and function of the brain using MRI technology. Participants were enrolled at the Y25 exam with the aim to achieve a balance of ethnicity/race and sex from three of the four CARDIA field centers: Birmingham, AL, Minneapolis, MN, and Oakland, CA. Each center recruited to a target of approximately 200 individuals. Exclusion criteria were contra-indication to MRI or a too large body size for the MRI tube bore. Ethics approval was given by the institutional review boards from each field center and coordinating center (University of Alabama Birmingham, University of Minnesota, and Kaiser Permanente Northern California), the MRI Reading Center (University of Pennsylvania) and the NIH Office of Human Subjects Research Protection for the Intramural Research Program, National Institute on Aging. All participants provided written informed consent at each CARDIA exam, with a separate written consent for participation in the brain MRI sub-study.
Brain MRI acquisition and processing information have been previously reported (Launer et al., 2015). Each CARDIA clinic site acquired brain MRI on 3-T MR scanners and transferred MRI data to a central archive, the MRI Reading Center, located at the University of Pennsylvania. We included the following in our analysis: volumetric (cubic centimeters, cc) estimates of total brain (TBV), gray matter (GMV), white matter (WMV), and the hippocampus (HV). We also explored WM tissue integrity measured by fractional anisotropy (WMFA) from diffusion tensor imaging scans (Haight et al., 2018).
ST was estimated by average hours per day from self-reported time spent (0, 15 mins or less, 30 mins, 1–5 h respectively, and 6 h or more) in the following activities: sitting while watching television, using the computer, doing non-computer paperwork, listening to music, reading, or doing arts, using the phone, and riding transportation. The details of the questionnaire can be found on the CARDIA website (https://www.cardia.dopm.uab.edu/images/more/pdf/Year25/CARDIA/Form91.pdf). We calculated the ST per week by adding up the reported hours in specific activities weighted by weekdays (5/7) and weekends (2/7). Plasma BDNF levels (picograms/ml, pg/ml) from specimens collected at the Y25 exam were assayed using the Quantikine® Human Free BDNF Immunoassay. Free BDNF in plasma, which was not bound to other proteins, receptors, or other factors, was measured by a 3.5-h solid phase ELISA that possesses a detectable range of 125–40,000 pg/ml at a 1:10 dilution.
Based on prior studies, we included in our analysis demographic factors (i.e., age, sex, and race) (Raz et al., 2009; Liu et al., 2016; Brown et al., 2020), and total intracranial volume (ICV), as a measure of head size (Driscoll et al., 2012).
We also included in our models, cardiovascular risk factors, which have been shown to be associated with plasma BDNF levels, and with brain volumes. High levels of vascular risk factors are generally associated with brain atrophy (Launer et al., 2015; Cox et al., 2019), while the relationship between vascular factors and BDNF is inconsistent and may depend on age and sex (Pikula et al., 2013; Jamal et al., 2015).
Other factors included in the models were: Education levels (Chan et al., 2018); BMI (kg/m2) (Ho et al., 2011); tobacco use (ever/never) (Pikula et al., 2013); presence of diabetes (based on fasting glucose, glucose tolerance, hemoglobin A1c, and medications) (American Diabetes Association, 2011), hypertension (based on: ≥ 140/≥90 mmHg or on hypertensive medications) (Chobanian et al., 2003), dyslipidemia (based on: triglycerides ≥ 150 mg/dl, HDL < 35 mg/dl if males; Triglycerides ≥ 150 mg/dl, HDL < 45 mg/dl if females) (Gottesman et al., 2017), and depression symptomology measured by the Center for Epidemiologic Studies Depression Scale (Beekman et al., 1997).
At the Y25 examination, 719 men and women participated in the Brain MRI Sub-study, among whom 625 had plasma BDNF data (Supplementary Figure 1). After excluding 13 persons with missing values of ST data, there were 612 with complete data for these analyses. Secondary longitudinal analyses included 418 (68.3%) participants who attended both the Y25 and Y30 MRI exams and had complete data. There were 194 (31.7%) participants who did not return for the second MRI (Supplementary Table 1). In general, the dropouts had a higher ST and BMI, and more often had diabetes, hypertension, dyslipidemia, hypertension, and smoked. However, both the baseline BDNF and brain measures were similar among the dropouts and attenders.
All brain measures were normally distributed and coded as continuous variables. ST distribution was right skewed. To compare high ST with low ST, ST was categorized into quartiles with the lower 25%ile at ≤ 4.3 h per day as the reference group. We used natural log transformation for the distribution of plasma BDNF levels to normalize the right-skew. Age was a continuous variable. Binary variables included sex (female and male) and race (White and Black) with males and Blacks as the reference groups, respectively.
For descriptive analysis, we used ANCOVA and logistic regression to compare continuous and categorical characteristics at the Y25 exam across the ST quartiles, respectively. For Model 1, we included age, sex, race, and ICV as covariates. Model 2 (supplement) additionally included vascular risk factors, as described above. To test for mediation effects of BDNF (Hypothesis 1), we used general linear regression to examine the associations of ST to MRI outcomes, with and without BDNF in the model. We tested whether levels of BDNF moderated the association of ST to brain measures by entering an interaction term (Hypothesis 2), BDNF by ST. Multicollinearity was checked between all predictor variates with all correlation coefficients < 0.6.
To verify and to expand this basic statistical approach, we also conducted a formal mediation and moderation analyses using the PROCESS model (Hayes, 2018). In these analyses, the direct and indirect (mediation) effects of ST on brain outcomes were estimated, with a test of the significance of BDNF as a mediator. Similarly, the PROCESS analysis tests for moderation effects (Hayes, 2018) by estimating coefficients of the model where brain measures are dependent variables, ST an independent variable, BDNF as the moderating variable, adjusting for covariates. The PROCESS analysis gives values of brain measures by ST quartiles at the 16, 50, and 84%ile of logBDNF.
For the longitudinal analysis, we used the same approach as in the main analysis. Five-year brain measure change was expressed as the percent change in brain measures from Y25 to Y30 exam (Jack et al., 2004).
We conducted all analyses using SAS 9.4® (SAS Institute, Inc., Cary, NC). Significance was based on 95% CI not including 0.
At the Y25 exam, participants were on average 50.3 years old, 51.6% were female, and 38.6% were Black (Table 1). Black participants tended to have higher ST. Vascular risk factors varied differed across ST quartiles. People with higher ST quartiles had higher BMI, and more often had diabetes, hypertension, dyslipidemia, and smoked compared with people in the lowest ST quartile (Table 1, Supplementary Table 3). Smoking (β = 0.23, 95% CI: 0.07, 0.40) and hypertension (β = 0.21, 95% CI: 0.04, 0.39) were significantly associated with one-unit higher logBDNF levels adjusting for age, sex, and race, respectively, compared with never-smoking and non-hypertensive (Table 2).
Adjusting for age, sex, race, and ICV, compared with those in the lowest ST quartile ( ≤ 4.3 h per day), people in the highest ST quartile (>8.4 h per day) had a significantly smaller brain volumes: TBV of −12.2 cc (95%CI: −20.73, −3.69), GMV of −7.8 cc (95%CI: −14.31, −1.28), and HV of −0.2 cc (95%CI: −0.33, −0.03) (Table 3). There were no significant differences in WMV or WMFA across different ST quartiles. Neither ST quartiles nor any of the brain measures were significantly associated with BDNF. Controlling for BDNF, or other covariates, the indirect effects between ST and brain measures were similar to the direct effects (i.e., without BDNF adjustment) suggesting BDNF did not mediate the association between ST and brain structure.
Table 3. Mediation and moderation effects of plasma BDNF levels on sedentary time (ST) and brain measures, model 1, CARDIA brain-MRI sub-study.
In Model 1 (Table 3, Figure 2), plasma BDNF significantly moderated the difference in TBV (95% CIinteraction: 0.28, 16.54) and WMV (95% CIinteraction: 1.08, 10.64) between the highest and lowest ST quartile. In those in the highest quartile of ST, higher BDNF levels were associated with higher TBV and WMV. Therefore, the difference in TBV and WMV between the high and low quartiles of ST was reduced with higher BDNF levels. Controlling for covariates, the difference in TBV between the high and low ST quartiles at the 16th percentile of logBDNF was −21.4 cc (95% CI: −33.60, 9.21), −11.0 cc (95% CI: −19.65, −2.41) at the 50th logBDNF percentile, and a non-significant difference of −4.4 cc (95% CI: −15.89, 7.13) at the 84th percentile. For WMV, the difference between the high and low quartiles was −10.8 cc (95% CI: −18.01, −3.66), −3.6 cc (95% CI: −8.67, 1.47) and 1.0 cc (95% CI: −5.74, 7.80) for the 16th, 50th and 84th logBDNF percentiles respectively. In Model 2, after additionally adjusting for vascular risk factors, plasma BDNF significantly moderated the difference in WMV (95% CIinteraction: 1.14, 11.49) but not the TBV (95% CIinteraction: −0.13, 17.11) between the high and low ST quartiles (Supplementary Table 1).
Figure 2. Moderation effects of BDNF on sedentary time (ST) to brain MRI at the Year 25, CARDIA Brain-MRI sub-study. (a) BDNF, brain-derived neurotrophic factor. (b) Models adjusted for age, sex, race, and intracranial volume (ICV).
Longitudinally, there was no significant difference in percent change in brain measures by ST quartiles adjusting for age, sex, race, and ICV (Table 3). However, the amount of change in brain volumes depended on the BDNF level. For example, with every unit increase in logBDNF, there was a 0.3% (95% CI: 0.14%, 0.45%) less decline in TBV. Table 3 also shows similar associations in GMV, WMV, and HV. There was no evidence that BDNF mediated or moderated the association of ST to change in brain measures.
Based on cross-sectional and longitudinal analyses, we investigated whether level of plasma BDNF influenced the association between ST and brain structure in a community-dwelling, middle-aged cohort of Black and White participants. Plasma BDNF levels modified the association between ST and brain measures. We found the lower the ST, the lower the brain volumes, but the differences between brain volumes in high vs. low ST were smaller as logBDNF increased in TBV and WMV (Figure 2). Our longitudinal analyses showed no association of ST to change in brain volumes but did show less decline in brain volume with higher BDNF levels. We did not find significant mediation or moderation effects in WM integrity in our study population.
BDNF plays a neurotrophic role for neuronal survival and brain plasticity for cognitive function (Weinstein et al., 2014). Experimental and human studies have linked plasma BDNF levels to brain structure and function and have shown exercise increased BDNF levels and brain health. However, prior studies on mediation or moderation of BDNF plasma levels on the PA—brain function are limited, and the findings are inconsistent (Egan et al., 2003; Vaynman et al., 2004; Raz et al., 2009; Brown et al., 2014). A recent review of randomized controlled trials by Gasquoine suggested limited benefits of PA for memory performance through BDNF regulation, suggesting pathways outside the central nervous system regarding the beneficial effects of PA for cognitive function (Gasquoine, 2018).
In general, mechanisms that regulate plasma BDNF levels are not clear. Several studies have shown plasma BDNF levels are under genetic regulation of the BDNF Val66Met polymorphism where Met allele carriers have lower plasma BDNF levels than Val/Val homozygotes (Takeuchi et al., 2020). There are also differences in brain structure or function associated with Met and Val carriership (Brown et al., 2014; Weinstein et al., 2014). Other studies have suggested plasma BDNF levels are influenced by lifestyle factors either directly or indirectly by the BDNF Val66Met polymorphism (Egan et al., 2003). These factors include cognitive stimulation (Adcock et al., 2020), diet (Sánchez-Villegas et al., 2011; Yamada-Goto et al., 2012), stress (Yamada-Goto et al., 2012), sleep (Schmitt et al., 2016), and smoking (Xia et al., 2019). In our study, tobacco use, and hypertension were related to significantly higher plasma BDNF levels (Table 2). This finding is consistent with studies in mid- to late-life populations and in animal models (Kenny et al., 2000; Golden et al., 2010; Amoureux et al., 2012; Weinstein et al., 2014). BDNF activity, the amount of BDNF expressed by the regulating genes, was positively correlated with high blood pressure and negatively associated with smoking (Golden et al., 2010; Weinstein et al., 2014). In animal studies, increased aortic BDNF expression occurs before blood pressure increases (Amoureux et al., 2012). Regional brain BDNF expression increased after chronic nicotine treatment in rats through the BDNF-TrkB pathway (Kenny et al., 2000).
We also found no association of ST to BDNF, suggesting measures of low levels of activity do not capture low levels of exposure to BDNF-associated factors that are specifically stimulated by exercise. These processes may be independent of those that lie along the sedentary—active continuum or are dependent on other lifestyle or disease-related associated processes.
Our findings from the longitudinal analyses suggest that relatively higher BDNF levels are associated with less 5-year decline in TBV, GMV, and HV. Although many studies have focused on maintaining BDNF levels for the benefits of GM structures such as the hippocampus (Erickson et al., 2010; Maioli et al., 2012), there is also evidence that BDNF may play a role in maintaining WM health through expression by glial cells (Sato et al., 2009; Giorgio et al., 2010; Miyamoto et al., 2015). Studies have reported relatively higher BDNF levels were associated with slower change in WMV with age (Driscoll et al., 2012). However, relatively higher BDNF plasma levels are associated with more 5-year decline in WM microstructure measured by WMFA in our study. This relationship could be explained by the upregulation of BDNF levels through oligodendrogenesis by WM glial cells (e.g., astrocytes) to protect and regenerate damaged WM fibers (Sato et al., 2009; Giorgio et al., 2010; Miyamoto et al., 2015).
This study has several strengths. As mentioned in previous publications, the CARDIA study provides data on a large community-based middle-aged cohort that includes Black and White participants. Data on this midlife age group provide important information of early changes in the brain, which may contribute to late-age prediction of cognitive impairment. Further, there are few data on the associations among ST, BDNF and brain volume in a such a cohort, which reflects the wider community. Additionally, we could examine these associations both cross-sectionally and longitudinally. We were also able to systematically study and identify other lifestyle factors that may influence levels of BDNF and should be accounted for in future studies.
Although the people who returned for a second MRI had fewer cardiovascular risk factors, there was no difference in BDNF and brain measures compared to those who did not return. Compared to studies with objective measures of PA, our self-reported ST may be over or underestimated to an unknown degree particularly if there are systematic differences in reporting by a factor of importance to this study, such as smoking or hypertension (Wanner et al., 2017). Furthermore, ST not only includes relatively inactive sitting, but it also includes activities such as computer work, socializing using the phone, or artwork. There are mixed associations between different sedentary activities and the brain, including both positive impact of mentally stimulating activities (e.g., crafts, puzzles, video games, etc.) (Wanders et al., 2021) and a negative impact from TV viewing (Hoang et al., 2016). More complete measures of sedentary behavior which could differentiate time and activities on sitting at work and during leisure time are needed to further explore the effect of cognitive stimulation on BDNF levels and its association with brain outcomes.
Lastly, we were not able to examine the role of the BDNF polymorphism in our study. Additionally, the analysis assumes the BDNF plasma levels reflect its activity in the brain, which is a weakness in all studies of plasma biomarkers of brain health. In humans, peripheral BDNF is often used as a proxy since central BDNF is difficult to measure. In animal studies, the peripheral and central BDNF are associated by BDNF crossing the blood-brain barrier in both directions (Gejl et al., 2019). However, as with all blood-based biomarkers of central processes, there is always a gap in our understanding of how close the blood-based molecules reflect central activity.
Together with other experimental and clinical studies, this study suggests that the negative association of ST with TBV and WMV was reduced in persons with relatively higher plasma BDNF levels in midlife.
The original contributions presented in the study are included in the article/Supplementary material, further inquiries can be directed to the corresponding author.
XZ had full access to all of the data in this study and takes responsibility for the integrity of the data and the accuracy of the data analysis. Concept, design, and drafting of the manuscript: XZ, CQ, and LL. Acquisition, analysis, or interpretation of data: XZ, ZL, CQ, and LL. Critical revision of the manuscript for important intellectual content: XZ, OM, ZL, KY, RB, CQ, and LL. Statistical analysis: XZ, OM, ZL, CQ, and LL. Technical support: ZL. All authors contributed to the article and approved the submitted version.
The Coronary Artery Risk Development in Young Adults Study (CARDIA) is conducted and supported by the National Heart, Lung, and Blood Institute (NHLBI) in collaboration with the University of Alabama at Birmingham (HHSN268201800005I and HHSN268201800007I), Northwestern University (HHSN268201800003I), University of Minnesota (HHSN268201800006I), and Kaiser Foundation Research Institute (HHSN268201800004I). CARDIA was also partially supported by the Intramural Research Program of the National Institute on Aging (NIA) and an intra-agency agreement between NIA and NHLBI (AG0005). CQ received grants from the Swedish Research Council (VR, 2017-05819 and 2020-01574), the Swedish Foundation for International Cooperation in Research and Higher Education (STINT, CH2019-8320), and Karolinska Institutet, Stockholm, Sweden.
This manuscript has been reviewed by CARDIA Publications and Presentations (P&P) committee.
The authors declare that the research was conducted in the absence of any commercial or financial relationships that could be construed as a potential conflict of interest.
All claims expressed in this article are solely those of the authors and do not necessarily represent those of their affiliated organizations, or those of the publisher, the editors and the reviewers. Any product that may be evaluated in this article, or claim that may be made by its manufacturer, is not guaranteed or endorsed by the publisher.
The Supplementary Material for this article can be found online at: https://www.frontiersin.org/articles/10.3389/frdem.2023.1110553/full#supplementary-material
Adcock, M., Fankhauser, M., Post, J., Lutz, K., Zizlsperger, L., Luft, A. R., et al. (2020). Effects of an in-home multicomponent exergame training on physical functions, cognition, and brain volume of older adults: a randomized controlled trial. Font. Med. 6, 321. doi: 10.3389/fmed.2019.00321
American Diabetes Association (2011). Diagnosis and classification of diabetes mellitus. Diab Care. 34(Supplement 1):S62–69. doi: 10.2337/dc11-S062
Amoureux, S., Lorgis, L., Sicard, P., Girard, C., Rochette, L., Vergely, C. (2012). Vascular BDNF expression and oxidative stress during aging and the development of chronic hypertension. Fundam. Clin. Pharmacol. 26, 227–334. doi: 10.1111/j.1472-8206.2010.00912.x
Barha, C. K., Liu-Ambrose, T., Best, J. R., Yaffe, K., Rosano, C., Study, H. A., et al. (2019). Sex-dependent effect of the BDNF Val66Met polymorphism on executive functioning and processing speed in older adults: evidence from the Health ABC study. Neurobiol. Aging. 74, 161–170. doi: 10.1016/j.neurobiolaging.2018.10.021
Beekman, A. T., Deeg, D. J., Van Limbeek, J., Braam, A. W., De Vries, M. Z., Van Tilburg, W. (1997). Criterion validity of the center for epidemiology studies depression scale (CES-D): restuls from a community-based sample of older subjects in the netherlands. Psychol. Med. 27, 231–235. doi: 10.1017/S0033291796003510
Bekinschtein, P., Cammarota, M., Igaz, L. M., Bevilaqua, L. R., Izquierdo, I., Medina, J. H. (2007). Persistence of long-term memory storage requires a late protein synthesis-and BDNF-dependent phase in the hippocampus. Neuron 53, 261–277. doi: 10.1016/j.neuron.2006.11.025
Brown, B. M., Bourgeat, P., Peiffer, J. J. (2014). Influence of BDNF Val66Met on the relationship between physical activity and brain volume. AAN 83, 1345–1352. doi: 10.1212/WNL.0000000000000867
Brown, D. T., Vickers, J. C., Stuart, K. E., Cechova, K., Ward, D. D. (2020). The BDNF Val66Met polymorphism modulates resilience of neurological functioning to brain ageing and dementia: a narrative review. Brain Sci. 10, 195. doi: 10.3390/brainsci10040195
Burzynska, A. Z., Chaddock-Heyman, L., Voss, M. W., Wong, C. N., Gothe, N. P., Olson, E. A., et al. (2014). Physical activity and cardiorespiratory fitness are beneficial for white matter in low-fit older adults. PLoS ONE 9, e107413. doi: 10.1371/journal.pone.0107413
Chan, D., Shafto, M., Kievit, R., Matthews, F., Spink, M., Valenzuela, M., et al. (2018). Lifestyle activities in mid-life contribute to cognitive reserve in late-life, independent of education, occupation, and late-life activities. Neurobiol. Aging. 70, 180–183. doi: 10.1016/j.neurobiolaging.2018.06.012
Chang, M., Jonsson, P. V., Snaedal, J., Bjornsson, S., Saczynski, J. S., Aspelund, T., et al. (2010). The effect of midlife physical activity on cognitive function among older adults: AGES—Reykjavik Study. J. Gerontol. A Biol. Sci. Med. Sci. 65A, 1369–1374. doi: 10.1093/gerona/glq152
Chobanian, A. V., Bakris, G. L., Black, H. R., Cushman, W. C., Green, L. A., Izzo Jr, J. L., et al. (2003). The seventh report of the joint national committee on prevention, detection, evaluation, and treatment of high blood pressure: the JNC 7 report. JAMA 289, 2560–2571. doi: 10.1001/jama.289.19.2560
Cox, S. R., Lyall, D. M., Ritchie, S. J., Bastin, M. E., Harris, M. A., Buchanan, C. R., et al. (2019). Associations between vascular risk factors and brain MRI incides in UK Biobank. Eur. Heart J. 40, 2290–2300. doi: 10.1093/eurheartj/ehz100
Driscoll, I., Martin, B., An, Y., Maudsley, S., Ferrucci, L., Mattson, M. P., et al. (2012). Plasma BDNF is associated with age-related white matter atrophy but not with cognitive function in older, non-demented adults. PLoS ONE 7, e35217. doi: 10.1371/journal.pone.0035217
Egan, M. F., Kojima, M., Callicott, J. H., Goldberg, T. E., Kolachana, B. S., Bertolino, A., et al. (2003). The BDNF val66met polymorphism affects activity-dependent secretion of BDNF and human memory and hippocampal function. Cell 112, 257–269. doi: 10.1016/S0092-8674(03)00035-7
Erickson, K. I., Prakash, R. S., Voss, M. W. (2010). Brain-derived neurotrophic factor is associated with age-related decline in hippocampal volume. J. Neurosci. 30, 5368–5375. doi: 10.1523/JNEUROSCI.6251-09.2010
Friedman, G. D., Cutter, G. R., Donahue, R. P., Hughes, G. H., Hulley, S. B., Jacobs Jr, D. R., et al. (1988). CARDIA: study design, recruitment, and some characteristics of the examined subjects. J. Clin. Epidemiol. 41, 1105–1116. doi: 10.1016/0895-4356(88)90080-7
Gasquoine, P. G. (2018). Effects of physical activity on delayed memory measures in randomized controlled trials with nonclinical older, mild cognitive impairment dementia participants. J. Clin. Exp. Neuropsychol. 40, 874–886. doi: 10.1080/13803395.2018.1442815
Gejl, A. K., Enevold, C., Bugge, A., Andersen, M. S., Nielsen, C. H., Andersen, L. B. (2019). Associations between serum and plasma brain-derived neurotrophic factor and influence of storage time and centrifugation strategy. Sci. Rep. 9, 1–9. doi: 10.1038/s41598-019-45976-5
Giorgio, A., Santelli, L., Tomassini, V. (2010). Age-related changes in gray and white matter structure throughout adulthood. Neuroimage 51, 943–951. doi: 10.1016/j.neuroimage.2010.03.004
Golden, E., Emiliano, A., Maudsley, S., Windham, B. G., Carlson, O. D., Egan, J. M., et al. (2010). Circulating brain-derived neurotrophic factor and indices of metabolic and cardiovascular health: data from the Baltimore Longitudinal Study of Aging. PLoS ONE 5, e10099. doi: 10.1371/journal.pone.0010099
Gottesman, R. F., Schneider, A. L. C., Zhou, Y., Coresh, J., Green, E., Gupta, N., et al. (2017). Association between midlife vascular risk factors and estimated brain amyloid deposition. JAMA 317, 1443–1450. doi: 10.1001/jama.2017.3090
Haight, T., Bryan, R. N., Erus, G., Hsieh, M.-K., Davatzikos, C., Nasrallah, I., et al. (2018). White matter microstructure, white matter lesions, and hypertension: an examination of early surrogate markers of vascular-realted brain change in midlife. NeuroImage Clin. 18, 753–761. doi: 10.1016/j.nicl.2018.02.032
Hayes, A. F. (2018). Introduction to Mediation, Moderation, and Conditional Process Analysis: A Regression-Based Approach. New York, NY: The Guilford Press.
Ho, A. J., Raji, C. A., Becker, J. T., Lopez, O. L., Kuller, L. H., Hua, X., et al. (2011). The effects of physical activity, education, and body mass index on the aging brain. Hum. Brain Mapp. 32, 1371–1382. doi: 10.1002/hbm.21113
Hoang, T. D., Reis, J., Zhu, N., Jacobs, D. R., Launer, L. J., Whitmer, R. A., et al. (2016). Effect of early adult patterns of physical activity and television viewing on midlife cognitive function. JAMA Psychiatry 73, 73–79. doi: 10.1001/jamapsychiatry.2015.2468
Jack, C. R., Shiung, M. M., Gunter, J. L., O'brien, P. C., Weigand, S. D., Knopman, D. S., et al. (2004). Comparison of different MRI brain atrophy rate measures with clinical disease progression in AD. Neurology 62, 591–600. doi: 10.1212/01.WNL.0000110315.26026.EF
Jamal, M., Van der Does, W., Elzinga, B. M., Molendijk, M. L., Penninx, B. W. (2015). Association between smoking, nocotine dependence, and BDNF Val66Met polymorphism with BDNF concentrations in serum. Nicotine Tob. Res. 17, 323–329. doi: 10.1093/ntr/ntu151
Kenny, P. J., File, S. E., Rattray, M. (2000). Acute nicotine decreases, and chronic nicotine increases the expression of brain-derived neurotrophic factor mRNA in rat hippocampus. Mol. Brain Res. 85, 234–238. doi: 10.1016/S0169-328X(00)00246-1
Korol, D. L., Gold, P. E., Scavuzzo, C. J. (2013). Use it and boost it with physical and mental activity. Hippocampus 23, 1125–1135. doi: 10.1002/hipo.22197
Kramer, A. F. (2021). How to better study the associations between physical activity, exercise, and cognitive and brain health. JAMA Netw. Open 4, e215153. doi: 10.1001/jamanetworkopen.2021.5153
Launer, L. J., Lewis, C. E., Schreiner, P. J., Sidney, S., Battapady, H., Jacobs, D. R., et al. (2015). Vascular factors and multiple measures of early brain health: CARDIA brain MRI study. PLoS ONE 10, e0122138. doi: 10.1371/journal.pone.0122138
Li, S., Weinstein, G., Zare, H., Teumer, A., Völker, U., Friedrich, N., et al. (2020). The genetics of circulating BDNF: towards understanding the role of BDNF in brain structure and function in middle and old ages. Brain Commun. 2, fcaa176. doi: 10.1093/braincomms/fcaa176
Liu, H., Wang, L., Geng, Z., Zhu, Q., Song, Z., Chang, R., et al. (2016). A voxel-based morphometric study of age- and sex-related changes in white matter volume in the normal aging brain. Neuropsychiatr. Dis. Treat. 12, 453–465. doi: 10.2147/NDT.S90674
Maioli, S., Puerta, E., Merino-Serrais, P., Fusari, L., Gil-Bea, F., Rimondini, R., et al. (2012). Combination of apolipoprotein E4 and high carbohydrate diet reduces hippocampal BDNF and Arc levels and impairs memory in young mice. J. Alzheimer's Dis. 32, 341–355. doi: 10.3233/JAD-2012-120697
Miyamoto, N., Maki, T., Shindo, A., Liang, A. C., Maeda, M., Egawa, N., et al. (2015). Astrocytes promote oligodendrogenesis after white matter damage via brain-derived neurotrophic factor. J. Neurosci. 35, 14002–14008. doi: 10.1523/JNEUROSCI.1592-15.2015
Owen, N., Healy, G. N., Matthews, C. E., Dunstan, D. W. (2010). Too much sitting: the population-health science of sedentary behavior. Exerc. Sport Sci. Rev. 38, 105–113. doi: 10.1097/JES.0b013e3181e373a2
Pikula, A., Beiser, A. S., Chen, T. C., Preis, S. R., Vorgias, D., DeCarli, C., et al. (2013). Serum brain-derived neurotrophic factor and vascular endothelial growth factor levels are associated with risk of stroke and vascular brain injury: Framingham Study. Stroke 44, 2768–2775. doi: 10.1161/STROKEAHA.113.001447
Raz, N., Rodrigue, K. M., Kenedy, K. M., Land, S. (2009). Genetic and vascular modifiers of age-sensitive cognitive skills: effects of COMT, BDNF, ApoE and hypertension. Neuropsychology 23, 105–116. doi: 10.1037/a0013487
Sánchez-Villegas, A., Galbete, C., Martinez-González, M. A., Martinez, J. A., Razquin, C., Salas-Salvadó, J., et al. (2011). The effect of the Mediterranean diet on plasma brain-derived neurotrophic factor (BDNF) levels: the PREDIMED-NAVARRA randomized trial. Nutr. Neurosci. 14, 195–201. doi: 10.1179/1476830511Y.0000000011
Sato, Y., Chin, Y., Kato, T., Tanaka, Y., Tozuka, Y., Mase, M., et al. (2009). White matter activated glial cells produce BDNF in a stroke model of monkeys. Neurosci. Res. 65, 71–78. doi: 10.1016/j.neures.2009.05.010
Schmitt, K., Holsboer-Trachsler, E., Eckert, A. B. D. N. F. (2016). in sleep, insomnia, and sleep deprivation. Ann. Med. 48, 42–51. doi: 10.3109/07853890.2015.1131327
Shimada, H., Makizako, H., Doi, T., Yoshida, D., Tsutsumimoto, K., Anan, Y., et al. (2014). A large, cross-sectional observational study of serum BDNF, cognitive function, and mild cognitive impairment in the elderly. Front. Aging Neurosci. 6, 69. doi: 10.3389/fnagi.2014.00069
Takeuchi, H., Tomita, H., Taki, Y., Kikuchi, Y., Ono, C., Yu, Z., et al. (2020). Effect of the interaction between BDNF Val66Met polymorphism and daily physical activity on mean diffusivity. Brain Imaging Behav. 14, 806–820. doi: 10.1007/s11682-018-0025-8
Vaynman, S., Ying, Z., Gomez-Pinilla, F. (2004). Hippocampal BDNF mediates the efficacy of exercise on synaptic plasticity and cognition. Eur. J. Neurosci. 20, 2580–2590. doi: 10.1111/j.1460-9568.2004.03720.x
Wanders, L., Bakker, E. A., van Hout, H. P. J., Eijsvogels, T. M. H., Hopman, M. T. E., Visser, L. N. C., et al. (2021). Association between sedentary time and cognitive function: a focus on different domains of sedentary behavior. Prev. Med. 153, 106731. doi: 10.1016/j.ypmed.2021.106731
Wanner, M., Richard, A., Martin, B., Faeh, D., Rohrmann, S. (2017). Associations between self-reported and objectively measured physical activity, sedentary behvaior and overweight/obesity in NHANES 2003–2006. Int. J. Obes. 41, 186–193. doi: 10.1038/ijo.2016.168
Weinstein, G., Beiser, A. S., Choi, S. H., Preis, S. R., Chen, T. C., Vorgas, D., et al. (2014). Serum brain-derived neurotrophic factor and the risk for dementia: the Framingham Heart Study. JAMA Neurol. 71, 55–61. doi: 10.1001/jamaneurol.2013.4781
Xia, H., Du, X., Yin, G., Zhang, Y., Li, X., Cai, J., et al. (2019). Effects of smoking on cognition and BDNF levels in a male Chinese population: relationship with BDNF Val66Met polymorphism. Sci. Rep. 9, 217. doi: 10.1038/s41598-018-36419-8
Yamada-Goto, N., Katsuura, G., Ochi, Y., Ebihara, K., Kusakabe, T., Hosoda, K., et al. (2012). Impairment of fear-conditioning responses and changes of brain neurotrophic factors in diet-induced obese mice. J. Neuroendocrinol. 24, 1120–1125. doi: 10.1111/j.1365-2826.2012.02327.x
Keywords: sedentary time, brain volume, middle-aged, longitudinal, biomarkers
Citation: Zhang X, Meirelles OD, Li Z, Yaffe K, Bryan RN, Qiu C and Launer LJ (2023) Sedentary behavior, brain-derived neurotrophic factor and brain structure in midlife: A longitudinal brain MRI sub-study of the coronary artery risk development in young adults study. Front. Dement. 2:1110553. doi: 10.3389/frdem.2023.1110553
Received: 28 November 2022; Accepted: 24 February 2023;
Published: 13 March 2023.
Edited by:
Zoe Arvanitakis, Rush University, United StatesReviewed by:
Alan W. J. Morris, University of Colorado Anschutz Medical Campus, United StatesCopyright © 2023 Zhang, Meirelles, Li, Yaffe, Bryan, Qiu and Launer. This is an open-access article distributed under the terms of the Creative Commons Attribution License (CC BY). The use, distribution or reproduction in other forums is permitted, provided the original author(s) and the copyright owner(s) are credited and that the original publication in this journal is cited, in accordance with accepted academic practice. No use, distribution or reproduction is permitted which does not comply with these terms.
*Correspondence: Lenore J. Launer, bGF1bmVybEBuaWEubmloLmdvdg==
Disclaimer: All claims expressed in this article are solely those of the authors and do not necessarily represent those of their affiliated organizations, or those of the publisher, the editors and the reviewers. Any product that may be evaluated in this article or claim that may be made by its manufacturer is not guaranteed or endorsed by the publisher.
Research integrity at Frontiers
Learn more about the work of our research integrity team to safeguard the quality of each article we publish.