- 1Brazilian Nanotechnology National Laboratory (LNNano), Brazilian Center for Research in Energy and Materials (CNPEM), São Paulo, Brazil
- 2Center for Nuclear Energy in Agriculture (CENA), University of São Paulo (USP), São Paulo, Brazil
- 3School of Technology, University of Campinas (UNICAMP), Sao Paulo, Brazil
- 4Grupo de Pesquisa em Bioquímica e Toxicologia em Caenorhabditis elegans (GBToxCe), Universidade Federal do Pampa (UNIPAMPA), Uruguaiana, Rio Grande do Sul, Brazil
- 5Departamento de Física, Universidade Federal do Ceará (UFC), Ceará, Brazil
Carbon nanomaterials are promising advanced materials for novel technologies. Therefore, biosafety studies are mandatory to support their safe development, uses, and disposal in sustainable innovation. Traditional toxicological assays are typically expensive, time-consuming, and have low throughput; they have been replaced by new approach methodologies (NAMs) focused on in vitro, in chemico and in silico approaches, along with alternative models. Caenorhabditis elegans has emerged as a complete model organism for predictive toxicology due to its transparent body, short reproductive and life cycles, and fully sequenced genome with high homology with the human genome. In this review, we discuss the current status, state-of-the-art characterization techniques, and scientific gaps in nanotoxicity studies involving the carbon nanomaterials and the C. elegans model considering the last two decades of research. Moreover, we show the existing supportive tools to evaluate the internalization and biodistribution of carbon nanomaterials in C. elegans and discuss their advantages and limitations. Methodological and experimental gaps must still be discussed with the scientific community; hence, we bring this discussion to light and point out future orientations and perspectives. This review will contribute for guiding the research with C. elegans and harmonization of assays/protocols linked to computational tools and nanoinformatics approaches during the development of carbon nanomaterials.
1 Introduction
Carbon nanomaterials (CNMs) are allotropes of carbon that have received significant attention because their unique properties enable novel products and technologies. They have been exploited in the biomedical field for drug delivery, imaging, tissue engineering, and target photothermal therapy (Maiti et al., 2019; Madima et al., 2020). CNMs have also been applied in composites, catalysis, electronic devices, food industries, environmental remediation, and agricultural technologies (Jangid and Prabhu Inbaraj, 2021; Singh et al., 2021; Cui et al., 2022; Hashim et al., 2022). However, this growing interest is approaching a relevant impasse as they can be released into the environment, thus reaching organisms and humans (Ding et al., 2022). Therefore, nanotechnology risks must be well investigated toward the safe, sustainable, and responsible application of CNMs (Caldeira et al., 2022).
Over the last decades, in vitro and in vivo models have been applied to provide pivotal insights into our understanding of nanomaterial-mediated toxicity mechanisms. The ethical ambition to conduct experiments without using animals has resulted in intense efforts over the past few decades from industry, academia, and regulatory bodies to develop and implement new approach methodologies (NAMs). The expansion of in silico (structure-based/toxicokinetic models) and in vitro methods (e.g., 3D cells culture, organs-on-chips, and organoids), together with the establishment of alternative model organisms, compose substantial new approach methodologies for analyzing the harmful effects of nanomaterials, supporting the next-generation risk assessment strategy (Pimtong et al., 2021; Magurany et al., 2023).
Caenorhabditis elegans is one of the biological models that has been exploited to study the biological effects of nanomaterials (NMs), considering the One-Health perspective for protecting animal, human, and environmental health (Gao, 2021). It was the first organism sequenced at the multicellular level, and it has complete and well-described digestive, reproductive, endocrine, sensory, and neuromuscular systems with signaling pathways quite similar to humans (Cook et al., 2019; Hastings et al., 2019).
The adoption of C. elegans in biological assays is considered to be advantageous because its culture in laboratory is inexpensive, and it has a high reproductive capacity (∼300 progeny per hermaphrodite adult) and a short life cycle, which are valuable relative to other in vivo models that have longer life cycles and complex manipulation. Due to its abundance in ecosystems and its key role in soil nutrient decomposition and cycling, C. elegans is a valuable marker of environmental changes. Unlike cell and tissues cultures, C. elegans responds to a stimulus by metabolically activating its neuronal, motor, digestive, and reproductive systems, besides endocrine signaling and behavioral responses. It is also highly sensitive to both environmental pollutants and drugs, which permits rapid toxicological screening by studying several complex and functional endpoints, such as lifespan and survival, growth rate, locomotory ability, reproduction, fertility, oxidative stress, intestinal damage, and alterations in the metabolic profiles (Tejeda-Benitez and Olivero-Verbel, 2016). Due to its transparent cuticle, optical, and fluorescence microscopy techniques can be used to visualize the uptake, translocation, and excretion of pollutants in vivo as well as structure-specific gene expression in transgenic strains (e.g., ROS induction marked by fluorescent proteins as GFP) (Batasheva et al., 2019).
Several regions of C. elegans genes are similar to human genes, and it exhibits highly conserved levels compared to other vertebrates in terms of gene functions and metabolic pathways (e.g., insulin/IGF-1 signaling (IIS) pathway) (Hastings et al., 2019). Most human neurotransmitter systems are present in this nematode, such as acetylcholine (ACh), glutamate, γ-aminobutyric acid (GABA), serotonin, and dopamine, making C. elegans an interesting animal to study neurotoxicology. A complete diagram of the chemical and electrical connections present in C. elegans neurons is available (Hall et al., 2005). Connectomes that encompass the C. elegans body were recently entirely characterized (Cook et al., 2019). Its alimentary system presents many characteristics that are similar to that of mammals, such as an acidified lumen, secretion of digestive enzymes, and peristalsis (Chauhan et al., 2013). Due to these features, C. elegans can be applied as a platform for understanding CNM toxicity at the organism, tissue, and molecular levels and extrapolating these effects to other complex organisms. However, nematodes have no blood–brain barrier; thus, pollutants directly interact with their nervous system (Hunt, 2017). Furthermore, the lack of a respiratory and circulatory system, as well as specific organs (e.g., brain, heart, lungs, eyes, and kidney) is a challenge to full elucidation of the potential toxicity of pollutants to humans (Gonzalez-Moragas et al., 2015).
Over the last 20 years, several relevant toxicity studies have been performed with C. elegans and CNMs. However, this knowledge has not been summarized yet. In addition, from our perspective, some scientific challenges and methodological particularities are mentioned but not thoroughly discussed in several studies. In this context, this review intends to sum up the current knowledge about the effects of carbon nanomaterials against the C. elegans model, as well as address the main techniques to support this evaluation while pointing out their advantages and limitations. Finally, we also raised the scientific and experimental gaps in nanotoxicity studies by offering some recommendations to advance this research field.
2 Toxicity of carbon nanomaterials on Caenorhabditis elegans: the state of the art
Current literature demonstrates that biological effects of carbon nanomaterials are strongly dependent on the physicochemical properties of the NM in question (e.g., morphology, size, surface charge, and chemistry) as well as its dispersion state, concentration, and conditions of exposure (e.g., period of duration, life stage of nematode, strain, and presence/absence of bacteria). Supplementary Table S1 considers these aspects, summarizes the published studies with C. elegans and these materials, and details the experimental conditions used.
Studies have reported accumulation of graphene oxide (GO) in the nematode gut and its translocation to reproductive organs, neurons, and even to eggs. A significant reduction in C. elegans reproductive capacity along with induction of germline apoptosis has been observed, besides the interruption of its cell cycle (Zhao et al., 2016; Chatterjee et al., 2017). GO exposure also reduces the nematode lifespan (Wu et al., 2013b), impairs its locomotion (Wu et al., 2014a), defecation behavior, and immune response (Wu et al., 2013a; Wu et al., 2014b). A potential neurotoxic effect of GO was also noticed because it accumulates in C. elegans head and decreases the dopaminergic and glutamatergic neurotransmitter contents (Kim et al., 2020).
Several biological mechanisms related to GO toxicity have been studied with C. elegans, such as apoptosis, RNA interference, and miRNA function. Overexpression of reproductive-related genes, such as egl-1 and ced-13, and even apoptosis-correspondent genes, such as ced-3, ced-4, and ced-9, due to multigenerational GO exposure was found (Jin et al., 2022). The expression of oxidative stress-related genes (sod-1, sod-3, and clt-2) was also reported (Tsai et al., 2021), as well as genes important for the function of AFD neurons (ceh-14 and ttx-1). GO also acted by dysregulating the expression of some proteins, such as NLP30, CNC-2, ISP-1, SOD-3, LYS-1, LYS-8, SSP-1, DOD-6, and F55G11.4 (Ding et al., 2018; Jin et al., 2022). Some strategies have been reported to reduce the toxicity of GO to C. elegans, such as chemically degrading it by sodium hypochlorite (Bortolozzo et al., 2021) or modifying its surface with bovine serum albumin (Sivaselvam et al., 2020; Côa et al., 2022).
The induction of oxidative stress and the reduction of nematode lifespan, growth, fertility, and reproduction have been observed in nematodes exposed to multi-walled carbon nanotubes (MWCNTs) (Sinha et al., 2016; Côa et al., 2022). The translocation of carbon nanotubes (CNTs) from pharynx to the intestine and gonads has been also reported (Wu et al., 2013a), although the pharynx has been pointed out as a key barrier in preventing this translocation to secondary organs (Zhuang et al., 2016). MWCNTs also affected the nematode behavior and brood size in a transgenerational study with activation of germline long non-coding RNA linc-7 (Zhao et al., 2022). The expression patterns of some genes required for intestinal development and the defecation cycle have been affected by exposure to MWNCTs (Shu et al., 2015). CNTs also dysregulated the expression of tbh-1 associated with the octopamine signal, or the expression of IncRNAs, such as linc-2, -7, -9, -32, and -50 (Zhao et al., 2022). miRNAs can be also dysregulated in the nematodes after prolonged exposure to MWCNT (0.1–10 mg L–1); it was observed that the expression levels of some miRNAs increased (e.g., mir-77, mir-52, mir-36, and mir-40) and others decreased (e.g., mir-57, mir-355, mir-249, and mir-1) in response to MWCNTs (Zhao et al., 2014).
Carbon quantum dots (C-QDs) can also be taken up by C. elegans via oral ingestion; they accumulate in gonad and germ cells without representing potential toxicity to C. elegans (Singh et al., 2018; Atchudan et al., 2019; Han et al., 2019). Hence, these carbon nanomaterials could be safely applied in bioimaging due to their fluorescent characteristics and apparent biocompatibility (Li et al., 2020).
Up to now, key studies have provided valuable insights into CNM toxicity to C. elegans. The surface chemistry of carbon nanomaterials has been discussed as a precursor to alterations in the toxicological profile and translocation of these materials in the C. elegans body (Nouara et al., 2013; Chatterjee et al., 2014; Yang et al., 2015; Chatterjee et al., 2017; Rive et al., 2019). Nevertheless, some aspects remain to be clarified, such as: 1) how the shape of carbon nanomaterials impacts biological effects; 2) whether the CNM colloidal behavior influences the obtained results in terms of toxicity; 3) how specifically CNMs are translocated from the primary to secondary target organs of C. elegans (Yao et al., 2022); 4) whether CNMs can be transferred to the next generations and what are the impacts of that; 5) whether the characteristics of CNMs can change over time in a toxicity assay with C. elegans, mainly in the presence of food (bacteria). In addition, toxicokinetic studies could be performed with C. elegans to predict the fate and behavior of CNMs in an entire animal with metabolically active systems toward obtaining valuable data for human health protection and explaining the fate and dynamics of NMs in the ecosystem.
The implementation of C. elegans in microcosm systems could be an interesting method of evaluating the behavior of CNMs in environmentally relevant scenarios and assessing the transport of CNMs in the food chain. This nematode could also be advantageous to further study of the toxicity of mixtures of CNMs with pre-existing environmental pollutants, as well as the impacts of physical, chemical, and biological transformations of CNMs in the environment to the toxicological response (e.g., photodegradation, homo or heteroaggregation, or coating with biomolecules).
3 Tools for evaluating nanomaterial internalization and biodistribution in Caenorhabditis elegans
Assessing the uptake and biodistribution of nanomaterials in organisms is a basic aspect of understanding and interpreting their potential effects, besides to identifying their persistence and behavior in the environment (Petersen et al., 2023). There are several methods to evaluate the biodistribution of organic and inorganic substances from biological matrices. However, identifying and quantifying nanomaterials is challenging, especially those that are carbon-based, which need to be distinguished from the carbon background from the organisms.
The uptake of nanomaterials by C. elegans occurs mainly through the mouth, which size is approximately 1 μm at the adult stage (Leung et al., 2008; Hunt, 2017). When internalized, CNMs can be translocated from the gastrointestinal region to proximities of the nervous system (Wang et al., 2017). Reproductive organs such as gonads and spermatheca are other targeted tissues. Some robust techniques are presented in Table 1, and their advantages and limitations are examined. Although imaging techniques are strategic instruments, it is notable that other tools are better exploited to understand the biodistribution of carbon nanomaterials in C. elegans. Adopting a multi-analytical approach from the materials and biological sciences seems to be the most promising alternative because no one technique is sufficient to fully access and understand NM fate and behavior.
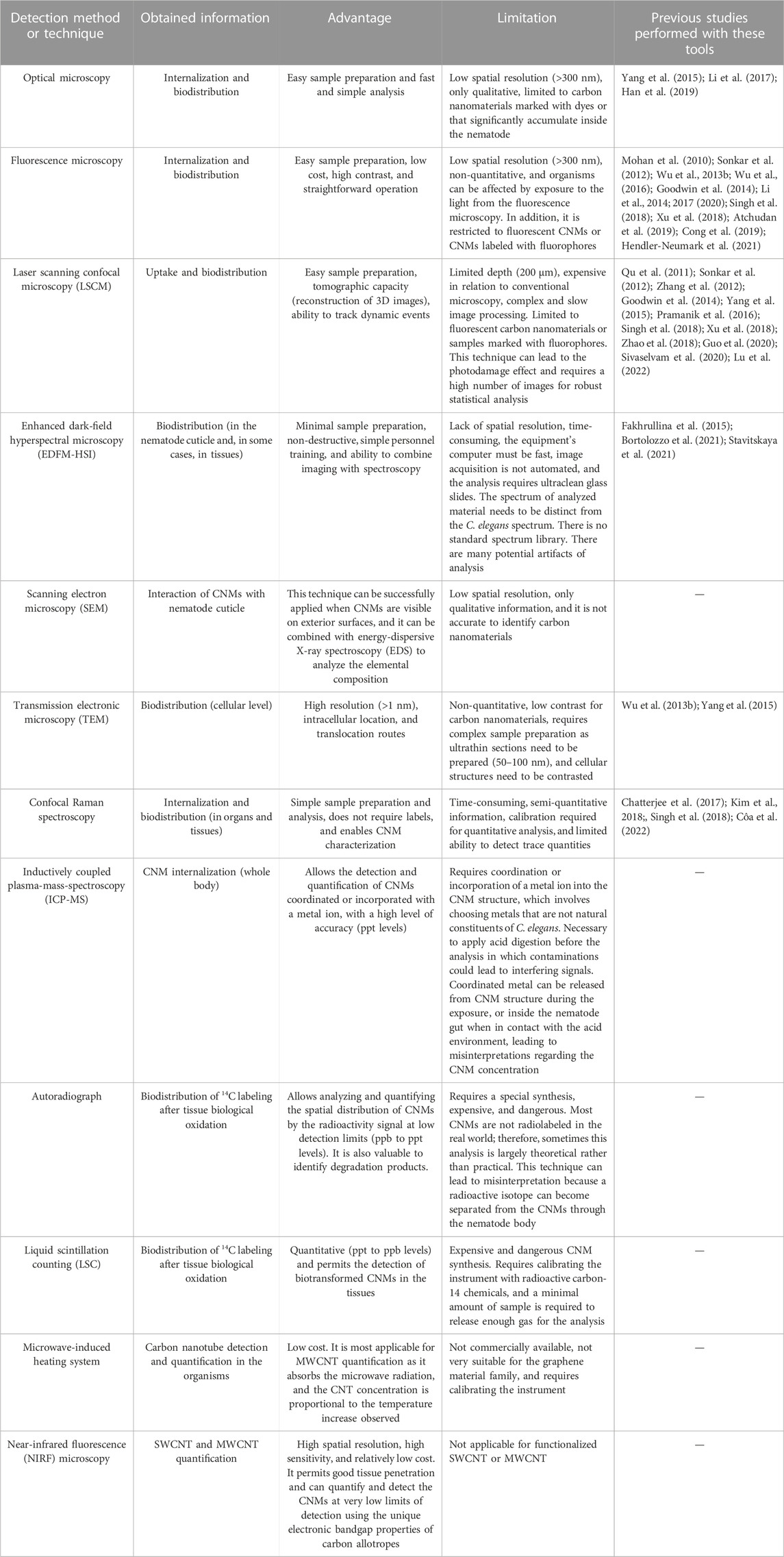
TABLE 1. Summary of promising tools to study carbon nanomaterial fate, uptake, and biodistribution in Caenorhabditis elegans.
Moreover, some instruments do not detect carbon nanomaterials with high precision, and the signal-to-noise ratio of these materials in the tissues is a challenge, especially when the purpose is to evaluate environmentally relevant concentrations (range at ng L−1). Labeling carbon nanomaterials with metals, fluorescent dyes (e.g., rhodamine B), or 14C could be a robust method of improving the ability to quantify these materials, especially in in situ conditions (Goodwin et al., 2018). However, these labels must be toxically tested and must remain attached to the carbon nanostrcuture during their internalization and analysis (Petersen et al., 2023).
The influence of organic molecules adsorbed on the CNM surfaces during the experiments (e.g., biocorona formation) is also an open and core question because it may impact detection and quantification methods. This impact has not been discussed in the literature; thus, the available techniques must be revisited.
Applying orthogonal methods would improve the discussions and reliability of results, thus reducing interferences and misinterpretations because the results would be based on measuring the same parameter using different physical principles (Simon et al., 2023). Radio-labeling CNMs is an option to help the development of new methods (Petersen et al., 2023). As important as choosing the right technique, the accuracy of sample preparation is a key element of avoiding bias and artifacts of analysis that can compromise the biological outcomes (Johnson et al., 2017a). In this direction, there is a field to be exploited because there are no standardized procedures to separate or extract CNMs from the biological matrices, especially from the nematode cuticle. However, one protocol was developed to remove non-ingested gold nanoparticles from the C. elegans cuticle and medium surrounding before bioaccumulation studies (Johnson et al., 2017b), this approach could be the basis for this additional research.
4 Scientific and methodological challenges in assays
4.1 Factors influencing the nano–bio interactions of Caenorhabditis elegans and carbon nanomaterials
Relevant efforts have been made to study carbon nanomaterial toxicity with C. elegans. However, difficulties inherent to nanotoxicity assays, the lack of details of the adopted methodologies, and the absence of standardized protocols may lead to inconsistent, hardly comparable, and irreproducible results (Selck et al., 2016). Moreover, specific factors may affect the interface between nematodes and nanomaterials in biological assays with C. elegans. All these key factors are summarized in Figure 1 and discussed in this topic.
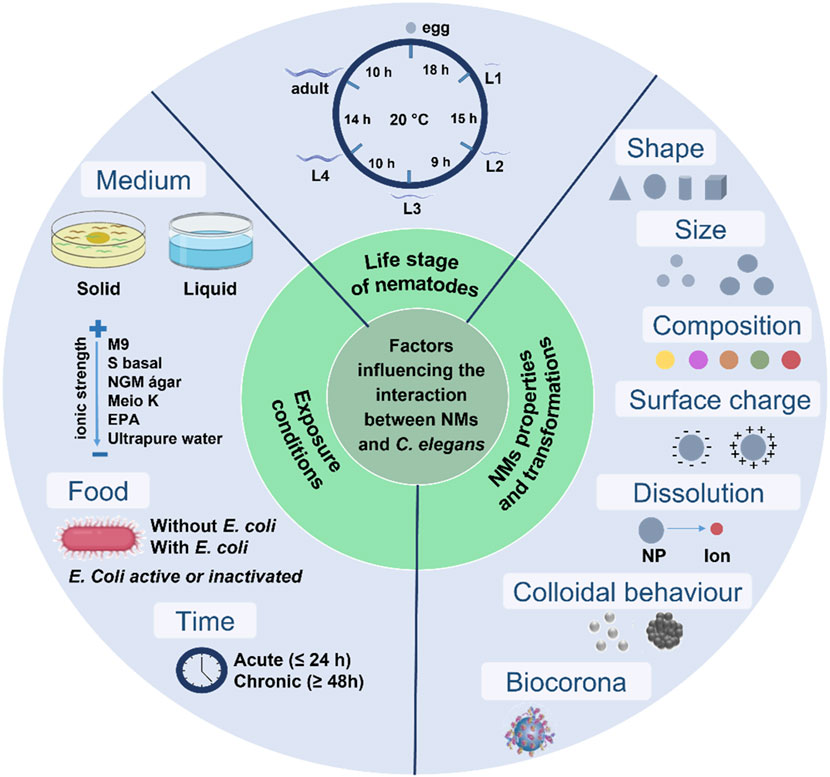
FIGURE 1. Factors that may influence the nano–bio interactions in assays with a Caenorhabditis elegans biological model.
Different from conventional chemicals (i.e., organic and inorganic molecules or bulk materials), the intrinsic physicochemical properties of nanomaterials (e.g., size, morphology, surface chemical composition, and charge) play a substantial role in their toxicity as they govern their contact and interface with organisms (Johnston et al., 2010; Ou et al., 2016). The NM surface charge (i.e., positive or negative), for instance, may interfere in the attractive force between the NMs and the C. elegans cuticle (Gonzalez-Moragas et al., 2017a). Moreover, differences in the synthesis method for the same nanomaterial, variations between batches and purification protocols, and impurities from synthesis are also critical issues for toxicity assessment (Liu et al., 2022). In view of this, it is key to characterize the physico-chemical properties of nanomaterials to understand their biological effects (ISO, 2012). A multi-technique approach and the use of orthogonal techniques are of fundamental importance and has been extensively documented in nanosafety guidelines and described in previous reviews (Gao and Lowry, 2018; Simon et al., 2023).
Another key element is the proper preparation of stock dispersions for biological experiments. They must present good colloidal stability to guarantee accurate dilutions as the low stability of NMs stock dispersions leads to low reproducibility. Ultrasounds or surfactant strategies have been used for this purpose but each one has drawbacks. The use of surfactants (e.g., tetrahydrofuran—THF) should be avoided because they may be toxic to the organisms (Zhu et al., 2006; Petersen and Henry, 2012). Probe-type ultrasounds are not suitable to prepare dispersions of carbon nanomaterials because high energies may modify the CNM properties, such as decreasing their size and/or increasing their structural defects (Mullick Chowdhury et al., 2014; Le et al., 2019). Ultrasonic baths are more appropriate for dispersing carbon nanomaterials because water temperature does not exceed 20 ± 2°C; high temperatures lead to strong CNM agglomeration that cannot be reversed by applying more energy as we empirically observed in the routine of our research group. Moreover, it is recommended that the period of sonication be studied toward protecting the CNM structure (OECD, 2017).
The carbon nanomaterial properties and exposure conditions (i.e., media composition and food availability) are key for toxicity because they can modify the NM behavior and their interactions with organisms. In addition, the life stage of nematodes and the exposure period are essential criteria to be considered (Liu et al., 2020).
The assessment of NM toxicity is typically carried out in either solid (nematode growth media (NGM)) or liquid (K-medium, S basal, M9) media with different chemical compositions. However, it is worth mentioning that they were developed to assess the toxicity of conventional chemicals. Therefore, they cannot be used for nanotoxicity studies without modification. In solid media, nematodes may be exposed to different concentrations of NMs due to the non-homogeneous mixture of these materials over the agar. Nanomaterials are also subject to interact with solid media components (e.g., phosphate) or bacteria, which can change the NM properties affecting the biological outcomes (Gonzalez-Moragas et al., 2017b). C. elegans may respond with an aversive behavior in the solid medium by avoiding exposure to carbon nanomaterials (Xiao et al., 2018).
In liquid media, multiple factors are associated with the biological effects of nanomaterials, such as aggregation/agglomeration, sedimentation, and transformations (i.e., dissolution or interaction with the food source and bacteria). These phenomena are strongly influenced by the medium’s characteristics, such as pH, ionic strength, and temperature. High ionic strength, for example, can reduce the electrostatic or steric stabilization of CNMs, thereby promoting the formation of aggregates/agglomerates and, subsequently, sedimentation (Yang et al., 2016).
Aggregation and sedimentation are phenomena that affect the conditions in which the nematodes are exposed to CNMs. The formation of aggregates/agglomerates and the settling of CNMs to the bottom of the exposure wells, where nematodes are located, may primarily influence the amount of material internalized by nematodes because the C. elegans buccal cavity has a size-selective filtering mechanism that captures the food and excludes large particles (>1 μm at l stage and >3 µm when adults) (Fang-Yen et al., 2009). As the toxic effect is associated with the amount of internalized substances, understanding the aggregation and agglomeration behavior is decisive for making assumptions about the toxicity of the tested material.
Furthermore, the settling of CNMs may also lead to differences between the nominal dose (i.e., what was administered in the test) versus “the delivered dose” that reaches the nematode at the bottom of well in the assays, resulting in levels of exposure higher than was calculated by initial dispersion measurements (Wang et al., 2018).
It is expected that the toxicological effect and the dose that affects 50% of organisms (LC50 or EC50) may vary for the same studied material in different exposure conditions (i.e., different medium or in presence/absence of bacteria), which makes the risk assessment process a massive challenge as it is based on LC50 values. This challenge should be solved by developing standardized dosimetry methodologies that would allow discerning the administered dose of the delivered/bioavailable dose. Even though no standardized protocol has been designed yet to study the sedimentation behavior of CNMs, significant advances have been made with cells that could guide further studies with C. elegans (DeLoid et al., 2017).
Moreover, the aggregation/agglomeration behavior assessment of carbon nanomaterials could be supported by the OECD guidelines no 318 (OECD, 2017) and no 317 (OECD, 2022), which are not specific to the CNM case but can be useful to initiating these discussions. In addition, dynamic light scattering could be a useful technique to assess the CNM aggregation, similar to what was adopted in our recent publication to study the stability of GO and MWCNT (Côa et al., 2022).
Another variable is related to the fact that NMs can undergo surface modifications when they encounter biomolecules in biological media. At this point, adding a food source (bacteria, commonly Escherichia coli) is an overlooked step that may result in misinterpretations because bacteria may interact with nanomaterials, leading to unknown and different effects.
The importance of assessing the influence of bacteria in studies with C. elegans was pointed out in several studies for conventional chemicals (Donkin and Williams, 1995; Ke and Aschner, 2019) and different NMs (Starnes et al., 2015; Luo et al., 2016; Hanna et al., 2018), but it is still far from being understood for carbon nanomaterials. A report has described, for example, that positively charged NMs interacted with bacteria, making the food unavailable to the nematodes (Hanna et al., 2018). As its developmental physiology is sensitive to nutrient depletion (Kaplan et al., 2018), a decrease in nematode growth, reproduction, and fertility was observed. Other researchers noticed a possible dietary transfer of NMs to the food chain when C. elegans was fed with E. coli after its interaction with the tested NMs (Priyam et al., 2021; How and Huang, 2023).
Indeed, the interaction of NMs with bacteria can affect the bacteria’s integrity, causing its lysis and release of its biomolecules, such as proteins, nucleic acids, lipids, and carbohydrates, in biological medium (Schaechter and Neidhardt, 1987). Consequently, these molecules can be adsorbed onto the NM surface, forming a coating known as biocorona, which will strongly affect the NM biological response and toxicity because the entity that cells “see” is not the naked nanomaterial but a hybrid nanomaterial–biomolecule instead (Markiewicz et al., 2018). The same effect can occur when NMs attach to the bacteria’s surface and are taken up by the nematodes. Inside the nematode pharynx, the biomolecules that composed the bacteria will be released by the physical degradation induced by the pharyngeal grinder; thus, these biomolecules will interface with the reactive surface of NMs. These nano–bio interactions may result in the biocorona formation as demonstrated in Figure 2, which will change particle surface properties and their agglomeration and settling behavior, altering the way by which the nematodes will be exposed to CNMs (Lynch et al., 2014).
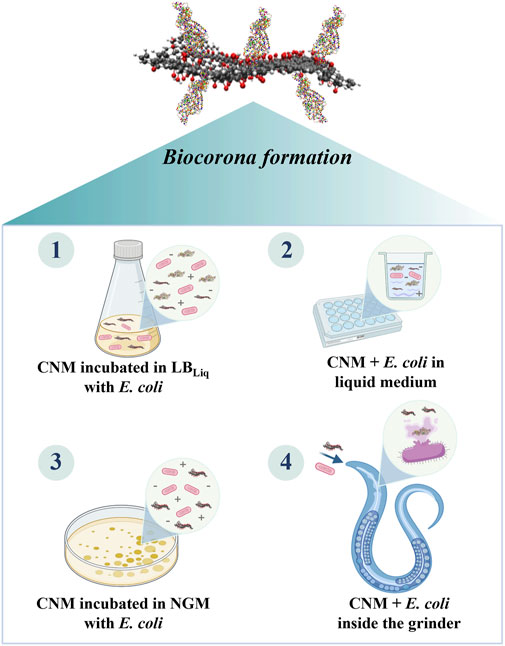
FIGURE 2. Scenarios in which the surface of CNMs can be coated by biomolecules during the biological assays with Caenorhabditis elegans leading to the biocorona formation.
Although the biocorona concept has been extensively discussed in the nanotoxicology field over the last 20 years (Mahmoudi et al., 2023), the occurrence of the biocorona formation at the interface of bacteria–NM interactions during the toxicological assays remains unknown for the C. elegans scientific community. Therefore, it must be incorporated into the scope of the studies because many were performed in the presence of bacteria, as was pointed out in Supplementary Table S1.
Moreover, these discussions indicate that exposure conditions must be standardized to allow the comparability of the data that are fundamental to predicting the potential risks of nanomaterials. One possible alternative is to review and adapt one guideline developed by the International Standard Organization (ISO) for studying the toxicity of environmental samples on the growth, reproduction, and fertility of C. elegans. ISO 10872:2020 is not focused on nanomaterials, but it establishes qualitative and quantitative parameters that must be reached to confirm the viability of C. elegans in the assays. The exact quantity of bacteria to be added to the tests was prescribed in this guideline, and a reference substance was indicated to verify the sensitivity of the C. elegans culture. Both elements are a starting point for the discussions because the current methodologies found in the literature do not incorporate a reference substance or even report the bacteria quantity applied. Obviously, adapting this protocol to different types of nanomaterials will require much work; hence, it will be discussed in the next topic.
In addition to the harmonization, one of the challenges to understanding CNM toxicity is interpreting and comparing the large volume of published data collected under a wide range of exposure conditions. The generation of data and no compilation of that with the physicochemical characterization of carbon nanomaterials do not contribute to generating mathematical models that can predict the toxicological effects (Lynch et al., 2021). Scientific publications in this field need to improve this aspect. The publication of raw data and data sharing needs to be encouraged to translate the collected data into knowledge (Harper et al., 2013).
4.2 Experimental gaps in toxicity tests with Caenorhabditis elegans and carbon nanomaterials
Although C. elegans has been successfully used as a model in nanotoxicology, some methodological barriers must be debated, including the lack of a standardized protocol to perform the toxicity assays and the investigation of the mechanisms of toxicity.
First, the laboratory practices and conditions must be carefully controlled. Different types of agars, the quality of reagents and water, and the variability in nutrient and vitamin content of media are factors that can alter the nematode gene expression, fertility, growth rate, resistance to pathogens, and lifespan. Furthermore, laboratory conditions, such as temperature and humidity, are critical to the C. elegans culture, influencing, for example, the prevalence of males in the culture. Finally, mutations in the bacterial strains need to be avoided, as well as contaminations with other bacteria or pathogens that can reduce the viability of C. elegans (Höss et al., 2012; Pho and MacNeil, 2019).
Developing a standardized medium for NM testing must be a priority in the C. elegans community as it is a central point to achieving comparable data. During the NANoREG project (Kleiven and Oughton, 2015), the guideline ISO 10872 was revised toward harmonizing the medium for nanotoxicological studies because most media have high chloride content and high ion strength. Two media were proposed: i) the EPA moderately hard reconstituted water, which has a low ionic strength (4 mM), and ii) simulated soil pore water (SSPW), which is an ecologically relevant medium for soil matrices, named ISO, 2010. However, until this date, few studies have been carried out with those media, thus making difficult to attest whether they are more suitable for nanotoxicity studies. It is important to emphasize that each NM has a distinct colloidal behavior; therefore, characterizing the NMs in the medium should be a key step to be incorporated in a standardized protocol.
E. coli concentration and viability are criteria that must be fully described and harmonized. By using a reference substance (BAC-C16) and applying the ISO 10872:2017, Hanna et al. (2016) observed that a minimum bacteria density (i.e., 500 FAU) is required for nematode development in chronic assays. Furthermore, they also noticed that a variation in bacterial density in 50 FAU occasioned inhibition of nematode growth by 19%, demonstrating that applying a standardized bacterial density is fundamental to robust experiments. Hanna et al. (2016) also tested the feasibility of this protocol for testing positive polystyrene nanoparticles. They reported that feeding the nematodes with UV-killed E. coli decreased the polystyrene NPs toxicity, suggesting that bacterial viability is a critical parameter. Moreover, they also observed that positive polystyrene NPs agglomerated with E. coli, making the food unavailable to C. elegans and causing inhibition of nematode growth. These findings confirm that the viability and density of E. coli are major parameters to be considered in the adoption of ISO 10872:2020 for nanotoxicity studies. At this point, it is important to highlight that some NMs can affect bacterial integrity or be taken up together with bacteria, leading to the biocorona formation, as previously mentioned. All these aspects depend on the NM type and biological medium; therefore, they need to be investigated.
Special attention should be given to techniques that allow characterizing CNMs in experimental media (in situ), such as the cryo-TEM technique. However, because these techniques are time-consuming, expensive, difficult to implement, and require specialized equipment that sometimes is not available for complex matrices, an alternative is applying strategic experimental controls. The National Institute of Standards and Technology (NIST) has published a list of experimental controls to be included in assays to improve their reliability (Petersen, 2015). Other experimental controls could be added to a standardized protocol toward distinguishing the artifacts from the biological outcomes in the CNM case.
Another difficulty is related to accurately counting organisms during the experiments to produce the dose–response curves. Although it appears to be simple, it is a time-consuming and error-prone process when researchers have many samples. The adopted methodologies are usually approximations that are not properly reported in publications (Scanlan et al., 2018). At the beginning of the research, high doses of carbon nanomaterials (>10 mg L–1) were tested with C. elegans. In addition to not being environmentally relevant, they lead to artifacts of analysis because carbon nanomaterials can aggregate in liquid media and settle at the bottom of the well, not allowing the nematodes to be accurately counted as we have been observed in our assays. Adopting automatic measurements, such as those performed by the Copas Biosort equipment, can be problematic because it is difficult to identify and separate the nematodes from aggregates. On the other hand, these automatic measurements are advantageous alternatives when environmentally relevant concentrations are studied.
There is a barrier in the studies conducted to investigate the trophic transfer of nanomaterials using E. coli and C. elegans. In these methodologies, authors interacted the NMs and E. coli in a Luria–Bertani (LB) broth. Afterward, nematodes were exposed to this mixture. However, this procedure may not be suitable because LB is a highly nutrient-rich microbial medium composed of peptone, yeast extract, and NaCl that will interact with the NM surface. In this way, a biocorona could be formed on NMs (as illustrated in Figure 2), and the toxicity outcomes will be related to this biocorona, not only to the effects of NMs. Therefore, it is important that we thoroughly examine the current exposure protocols to ensure they align with the main purpose.
The use of fluorescent probes (e.g., CM-H2DCFDA, Nile red, or acridine orange) for labeling damage on nematode tissues is also a topic of concern. As carbon nanomaterials are highly adsorbent and, typically, are taken up and accumulate in the nematode intestine, they can hinder the permeability of these reagents to the analyzed tissue. This aspect may generate uncertain results, mainly if high concentrations of CNMs are used. Thus, it may be more suitable to replace the fluorescent probes for transgenic strains marked to reduce or increase the damaged tissue’s fluorescence.
Developing harmonized protocols is a major issue toward producing comparable experimental data and minimizing the generation of artifacts. A rigorous posture is expected from nanotoxicology scientists to investigate both toxicity and the possibility of interfering/bias during the tests. Implementing good laboratory practices (GLP) to maintain the C. elegans culture and carry out assays is imperative to ensure the generated data’s consistency, reliability, reproducibility, quality, and integrity (Pho and MacNeil, 2019). Finally, interlaboratory comparisons need to be encouraged in the C. elegans community because they offer valuable insights about factors that need to be controlled in the protocols to enhance the accuracy and reliability of results (Höss et al., 2012).
5 Conclusion and future perspectives
Significant advances have been made toward understanding carbon nanomaterial toxicity. Overall, graphene-based materials, carbon nanotubes, and fullerene may potentially be toxic to C. elegans as they may affect its survival, reproduction, growth, fertility, germline cells, the functionality of the intestinal barrier and neurons, as well as deregulate the gene expression and important signaling pathways. However, most studies have focused on the biological effect. They overlooked the importance of exploring materials science, especially to clarify what are the properties of carbon nanomaterials that influence their toxicological profile to C. elegans. There is a knowledge gap in relation to the colloidal behavior of CNMs in the performed assays. Therefore, future studies should focus on correlating the biological results with the physicochemical properties and colloidal stability of CNMs. There is also a lack of multigenerational and transgenerational studies explaining how the observed effects can be transferred to the next generations, especially focused on epigenetic modifications.
Some experimental issues require investigation to guarantee the reliability of existing results, such as i) the influence of E. coli on CNM behavior, fate, and toxicity during the exposure, ii) transformations that these materials may suffer inside the nematode with emphasis on chemical and physical analyses and toxicological response, and iii) the products excreted after CNM ingestion. Some tools (e.g., microwave-induced heating system or autoradiograph) could be better exploited to measure the amount of CNM ingested and accumulated by C. elegans and to understand how CNMs are translocated to secondary target organs of this nematode.
As several factors influence the nano–bio interactions in the C. elegans experiments, many methodological gaps need to be revisited by considering the new facets described herein. These issues need to be standardized in protocols that include strategic experimental controls, reference substances, and guidance on the best practices. Cause-and-effects diagrams should be employed to gather information about possible interferences in the tests. The application of the FAIR (Findability, Accessibility, Interoperability, and Reuse of digital assets) data principle is also imperative within the C. elegans scientific community.
We hope these outstanding questions can encourage scientists to take advantage of the possibilities offered by C. elegans to continue elevating the studies to a higher level of knowledge in the coming decades. Furthermore, the perspectives raised herein open the door for new studies that would help toxicologists to validate C. elegans as a model organism to be implemented worldwide within the perspective of new and reliable approach methodologies for regulatory purposes.
Author contributions
FC contributed to the conception, structure of the paper, analysis, writing, and interpretation of available literature. LB contributed to the development of the initial draft and interpretation of available literature. DSA and AGSF reviewed and improved the output for important intellectual content. DSTM supervised, reviewed, and was responsible for project administration and funding acquisition. All authors contributed to the article and approved the submitted version.
Funding
This work was supported by Coordenação de Aperfeiçoamento Pessoal de Nível Superior (CAPES)—Finance Code 001, the National Institute for Research, Development, and Innovation in Functional Complex Materials (INCT-Inomat), the National System of Laboratories in Nanotechnologies (SisNANO/MCTI), the Sao Paulo Research Foundation (FAPESP), the Brazil-China Center for Research and Innovation in Nanotechnology (CBCIN/MCTI), and the National Council for Scientific and Technological Development (CNPq). DSA acknowledges FAPERGS (Grant 21/2551-0001963-8), AGSF acknowledges funding from CNPq (Grant 305355/2022-8), and DSTM thanks the CNPq for the Research Productivity Fellowship grant (315575/2020-4).
Conflict of interest
The authors declare that the research was conducted in the absence of any commercial or financial relationships that could be construed as a potential conflict of interest.
The authors AGSF and DSTM declared that they were an editorial board member of Frontiers, at the time of submission. This had no impact on the peer review process and the final decision.
Publisher’s note
All claims expressed in this article are solely those of the authors and do not necessarily represent those of their affiliated organizations, or those of the publisher, the editors, and the reviewers. Any product that may be evaluated in this article, or claim that may be made by its manufacturer, is not guaranteed or endorsed by the publisher.
Supplementary material
The Supplementary Material for this article can be found online at: https://www.frontiersin.org/articles/10.3389/frcrb.2023.1241637/full#supplementary-material
References
Atchudan, R., Edison, T. N. J. I., Perumal, S., Clament Sagaya Selvam, N., and Lee, Y. R. (2019). Green synthesized multiple fluorescent nitrogen-doped carbon quantum dots as an efficient label-free optical nanoprobe for in vivo live-cell imaging. J. Photochem. Photobiol. A Chem. 372, 99–107. doi:10.1016/j.jphotochem.2018.12.011
Batasheva, S., Fakhrullina, G., Akhatova, F., and Fakhrullin, R. (2019). “Caenorhabditis elegans nematode: A versatile model to evaluate the toxicity of nanomaterials in vivo,” in Nanotechnology characterization tools for environment, health, and safety (Berlin, Heidelberg: Springer Berlin Heidelberg), 323–345. doi:10.1007/978-3-662-59600-5_11
Bortolozzo, L. S., Côa, F., Khan, L. U., Medeiros, A. M. Z., Da Silva, G. H., Delite, F. S., et al. (2021). Mitigation of graphene oxide toxicity in C. elegans after chemical degradation with sodium hypochlorite. Chemosphere 278, 130421. doi:10.1016/j.chemosphere.2021.130421
Cong, S., Liu, K., Qiao, F., Song, Y., and Tan, M. (2019). Biocompatible fluorescent carbon dots derived from roast duck for in vitro cellular and in vivo C. elegans bio-imaging. Methods 168, 76–83. doi:10.1016/j.ymeth.2019.07.007
Caldeira, C., Farcal, R., Garmendia Aguirre, I., Mancini, L., Tosches, D., Amelio, A., et al. (2022). Safe and sustainable by design chemicals and materials - framework for the definition of criteria and evaluation procedure for chemicals and materials. Luxembourg: JRC Publications Repository. doi:10.2760/487955
Chatterjee, N., Kim, Y., Yang, J., Roca, C. P., Joo, S.-W., and Choi, J. (2017). A systems toxicology approach reveals the Wnt-MAPK crosstalk pathway mediated reproductive failure in Caenorhabditis elegans exposed to graphene oxide (GO) but not to reduced graphene oxide (rGO). Nanotoxicology 11, 76–86. doi:10.1080/17435390.2016.1267273
Chatterjee, N., Yang, J., Kim, H. M., Jo, E., Kim, P. J., Choi, K., et al. (2014). Potential toxicity of differential functionalized multiwalled carbon nanotubes (MWCNT) in human cell line (BEAS2B) and caenorhabditis elegans. J. Toxicol. Environ. Health, Part A 77, 1399–1408. doi:10.1080/15287394.2014.951756
Chauhan, V. M., Orsi, G., Brown, A., Pritchard, D. I., and Aylott, J. W. (2013). Mapping the pharyngeal and intestinal pH of Caenorhabditis elegans and real-time luminal pH oscillations using extended dynamic range pH-sensitive nanosensors. ACS Nano 7, 5577–5587. doi:10.1021/nn401856u
Chen, H., Li, H., and Wang, D. (2017). Graphene oxide dysregulates neuroligin/NLG-1-mediated molecular signaling in interneurons in Caenorhabditis elegans. New York Plaza, New York: Nature Publishing Group, 1–14. doi:10.1038/srep41655
Chen, P. H., Hsiao, K. M., and Chou, C. C. (2013). Molecular characterization of toxicity mechanism of single-walled carbon nanotubes. Biomaterials 34, 5661–5669. doi:10.1016/j.biomaterials.2013.03.093
Côa, F., Delite, F. d. S., Strauss, M., and Martinez, D. S. T. (2022). Toxicity mitigation and biodistribution of albumin corona coated graphene oxide and carbon nanotubes in Caenorhabditis elegans. NanoImpact 27, 100413. doi:10.1016/j.impact.2022.100413
Cook, S. J., Jarrell, T. A., Brittin, C. A., Wang, Y., Bloniarz, A. E., Yakovlev, M. A., et al. (2019). Whole-animal connectomes of both Caenorhabditis elegans sexes. Nature 571, 63–71. doi:10.1038/s41586-019-1352-7
Cui, K., Chang, J., Feo, L., Chow, C. L., and Lau, D. (2022). Developments and applications of carbon nanotube reinforced cement-based composites as functional building materials. Front. Mater 9. doi:10.3389/fmats.2022.861646
DeLoid, G. M., Cohen, J. M., Pyrgiotakis, G., and Demokritou, P. (2017). Preparation, characterization, and in vitro dosimetry of dispersed, engineered nanomaterials. Nat. Protoc. 12, 355–371. doi:10.1038/nprot.2016.172
Ding, X., Pu, Y., Tang, M., and Zhang, T. (2022). Environmental and health effects of graphene-family nanomaterials: potential release pathways, transformation, environmental fate and health risks. Nano Today 42, 101379. doi:10.1016/j.nantod.2022.101379
Ding, X., Rui, Q., and Wang, D. (2018). Functional disruption in epidermal barrier enhances toxicity and accumulation of graphene oxide. Ecotoxicol. Environ. Saf. 163, 456–464. doi:10.1016/j.ecoenv.2018.07.102
Donkin, S. G., and Williams, P. L. (1995). Influence of developmental stage, salts and food presence on various end points using Caenorhabditis Elegans for aquatic toxicity testing. Environ. Toxicol. Chem. 14, 2139–2147. doi:10.1002/etc.5620141218
Fakhrullina, G. I., Akhatova, F. S., Lvov, Y. M., and Fakhrullin, R. F. (2015). Toxicity of halloysite clay nanotubes in vivo: A Caenorhabditis elegans study. Environ. Sci. Nano 2, 54–59. doi:10.1039/C4EN00135D
Fang-Yen, C., Avery, L., and Samuel, A. D. T. (2009). Two size-selective mechanisms specifically trap bacteria-sized food particles in Caenorhabditis elegans. Proc. Natl. Acad. Sci. 106, 20093–20096. doi:10.1073/pnas.0904036106
Gao, P. (2021). The exposome in the era of one health. Environ. Sci. Technol. 55, 2790–2799. doi:10.1021/acs.est.0c07033
Gao, X., and Lowry, G. V. (2018). Progress towards standardized and validated characterizations for measuring physicochemical properties of manufactured nanomaterials relevant to nano health and safety risks. NanoImpact 9, 14–30. doi:10.1016/j.impact.2017.09.002
Gonzalez-Moragas, L., Berto, P., Vilches, C., Quidant, R., Kolovou, A., Santarella-Mellwig, R., et al. (2017a). In vivo testing of gold nanoparticles using the Caenorhabditis elegans model organism. Acta Biomater. 53, 598–609. doi:10.1016/j.actbio.2017.01.080
Gonzalez-Moragas, L., Maurer, L. L., Harms, V. M., Meyer, J. N., Laromaine, A., and Roig, A. (2017b). Materials and toxicological approaches to study metal and metal-oxide nanoparticles in the model organism: Caenorhabditis elegans. Mater Horiz. 4, 719–746. doi:10.1039/c7mh00166e
Gonzalez-Moragas, L., Roig, A., and Laromaine, A. (2015). C. elegans as a tool for in vivo nanoparticle assessment. Adv. Colloid Interface Sci. 219, 10–26. doi:10.1016/j.cis.2015.02.001
Goodwin, C. M., Lewis, G. G., Fiorella, A., Ellison, M. D., and Kohn, R. (2014). Synthesis and toxicity testing of cysteine-functionalized single-walled carbon nanotubes with Caenorhabditis elegans. RSC Adv. 4, 5893. doi:10.1039/c3ra44888f
Goodwin, D. G., Adeleye, A. S., Sung, L., Ho, K. T., Burgess, R. M., and Petersen, E. J. (2018). Detection and quantification of graphene-family nanomaterials in the environment. Environ. Sci. Technol. 52, 4491–4513. doi:10.1021/acs.est.7b04938
Guo, T., Cheng, L., Zhao, H., Liu, Y., Yang, Y., Liu, J., et al. (2020). The C. elegans miR-235 regulates the toxicity of graphene oxide via targeting the nuclear hormone receptor DAF-12 in the intestine. Sci. Rep. 10, 16933. doi:10.1038/s41598-020-73712-x
Hall, D. H., Lints, R., and Altun, Z. (2006). Nematode neurons: anatomy and anatomical methods in Caenorhabditis elegans. Int. Rev. Neurobiol. 69, 1–35. doi:10.1016/S0074-7742(05)69001-0
Han, G., Zhao, J., Zhang, R., Tian, X., Liu, Z., Wang, A., et al. (2019). Membrane-penetrating carbon quantum dots for imaging nucleic acid structures in live organisms. Angew. Chem. - Int. Ed. 58, 7087–7091. doi:10.1002/anie.201903005
Hanna, S. K., Cooksey, G. A., Dong, S., Nelson, B. C., Mao, L., Elliott, J. T., et al. (2016). Feasibility of using a standardized: Caenorhabditis elegans toxicity test to assess nanomaterial toxicity. Environ. Sci. Nano 3, 1080–1089. doi:10.1039/c6en00105j
Hanna, S. K., Montoro Bustos, A. R., Peterson, A. W., Reipa, V., Scanlan, L. D., Hosbas Coskun, S., et al. (2018). Agglomeration of Escherichia coli with positively charged nanoparticles can lead to artifacts in a standard Caenorhabditis elegans toxicity assay. Environ. Sci. Technol. 52, 5968–5978. doi:10.1021/acs.est.7b06099
Harper, S. L., Hutchison, J. E., Baker, N., Ostraat, M., Tinkle, S., Steevens, J., et al. (2013). Nanoinformatics workshop report: current resources, community needs and the proposal of a collaborative framework for data sharing and information integration. Comput. Sci. Discov. 6, 014008. doi:10.1088/1749-4699/6/1/014008
Hashim, N., Abdullah, S., and Yusoh, K. (2022). Graphene nanomaterials in the food industries: quality control in promising food safety to consumers. Graphene 2D Mater. 7, 1–29. doi:10.1007/s41127-021-00045-5
Hastings, J., Mains, A., Virk, B., Rodriguez, N., Murdoch, S., Pearce, J., et al. (2019). Multi-omics and genome-scale modeling reveal a metabolic shift during C. elegans aging. Front. Mol. Biosci. 6, 2. doi:10.3389/fmolb.2019.00002
Hendler-Neumark, A., Wulf, V., and Bisker, G. (2021). In vivo imaging of fluorescent single-walled carbon nanotubes within C. elegans nematodes in the near-infrared window. Mater. Today Bio 12, 100175. doi:10.1016/j.mtbio.2021.100175
Höss, S., Ahlf, W., Bergtold, M., Bluebaum-Gronau, E., Brinke, M., Donnevert, G., et al. (2012). Interlaboratory comparison of a standardized toxicity test using the nematode Caenorhabditis elegans (ISO 10872). Environ. Toxicol. Chem. 31, 1525–1535. doi:10.1002/etc.1843
How, C. M., and Huang, C.-W. (2023). Dietary transfer of zinc oxide nanoparticles induces locomotive defects associated with GABAergic motor neuron damage in Caenorhabditis elegans. Nanomaterials 13, 289. doi:10.3390/nano13020289
Hunt, P. R. (2017). The C. elegans model in toxicity testing. J. Appl. Toxicol. 37, 50–59. doi:10.1002/jat.3357
ISO (2010). ISO 10872:2010 - water quality — determination of the toxic effect of sediment and soil samples on growth, fertility and reproduction of Caenorhabditis elegans (Nematoda). Geneva, Switzerland: International Organization for Standardization.
ISO (2012). ISO/TR 13014:2012- Nanotechnologies — guidance on physico-chemical characterization of engineered nanoscale materials for toxicologic assessment. Geneva, Switzerland: International Organization for Standardization, 0–33.
Jangid, P., and Prabhu Inbaraj, M. (2021). Applications of nanomaterials in wastewater treatment. Mater. Today Proc. 43, 2877–2881. doi:10.1016/j.matpr.2021.01.126
Jin, L., Dou, T.-T., Chen, J.-Y., Duan, M.-X., Zhen, Q., Wu, H.-Z., et al. (2022). Sublethal toxicity of graphene oxide in Caenorhabditis elegans under multi-generational exposure. Ecotoxicol. Environ. Saf. 229, 113064. doi:10.1016/j.ecoenv.2021.113064
Johnson, M. E., Hanna, S. K., Montoro Bustos, A. R., Sims, C. M., Elliott, L. C. C., Lingayat, A., et al. (2017a). Separation, sizing, and quantitation of engineered nanoparticles in an organism model using inductively coupled plasma mass spectrometry and image analysis. ACS Nano 11, 526–540. doi:10.1021/acsnano.6b06582
Johnson, M. E., Montoro Bustos, A. R., Hanna, S. K., Petersen, E. J., Murphy, K. E., Yu, L. L., et al. (2017b). Sucrose density gradient centrifugation for efficient separation of engineered nanoparticles from a model organism. Gaithersburg, MD: Caenorhabditis elegans. doi:10.6028/NIST.SP.1200-24
Johnston, H. J., Hutchison, G. R., Christensen, F. M., Peters, S., Hankin, S., Aschberger, K., et al. (2010). A critical review of the biological mechanisms underlying the in vivo and in vitro toxicity of carbon nanotubes: the contribution of physico-chemical characteristics. Nanotoxicology 4, 207–246. doi:10.3109/17435390903569639
Kaplan, R. E. W., Webster, A. K., Chitrakar, R., Dent, J. A., and Baugh, L. R. (2018). Food perception without ingestion leads to metabolic changes and irreversible developmental arrest in C. elegans. BMC Biol. 16, 112. doi:10.1186/s12915-018-0579-3
Ke, T., and Aschner, M. (2019). Bacteria affect Caenorhabditis elegans responses to MeHg toxicity. Neurotoxicology 75, 129–135. doi:10.1016/j.neuro.2019.09.002
Kim, M., Eom, H. J., Choi, I., Hong, J., and Choi, J. (2020). Graphene oxide-induced neurotoxicity on neurotransmitters, AFD neurons and locomotive behavior in Caenorhabditis elegans. Neurotoxicology 77, 30–39. doi:10.1016/j.neuro.2019.12.011
Kim, Y., Jeong, J., Yang, J., Joo, S.-W., Hong, J., and Choi, J. (2018). Graphene oxide nano-bio interaction induces inhibition of spermatogenesis and disturbance of fatty acid metabolism in the nematode Caenorhabditis elegans. Toxicology 410, 83–95. doi:10.1016/j.tox.2018.09.006
Kleiven, M., and Oughton, D. (2015). Standard Operating Procedure: Toxicity test with the nematode Caenorhabiditis elegans for the NANoREG core nanomaterials.
Le, G. T. T., Chanlek, N., Manyam, J., Opaprakasit, P., Grisdanurak, N., and Sreearunothai, P. (2019). Insight into the ultrasonication of graphene oxide with strong changes in its properties and performance for adsorption applications. Chem. Eng. J. 373, 1212–1222. doi:10.1016/j.cej.2019.05.108
Leung, M. C. K., Williams, P. L., Benedetto, A., Au, C., Helmcke, K. J., Aschner, M., et al. (2008). Caenorhabditis elegans: an emerging model in biomedical and environmental toxicology. Toxicol. Sci. 106, 5–28. doi:10.1093/toxsci/kfn121
Li, H., Fei, C., Yang, D., Tan, C., Chen, Z., Wang, J., et al. (2020). Synthesis of carbon nitride quantum dots and biocompatibility evaluation using C. elegans as a model organism. Mater. Today Commun. 25, 101383. doi:10.1016/j.mtcomm.2020.101383
Li, J., He, Z., Guo, C., Wang, L., and Xu, S. (2014). Synthesis of carbon nanohorns/chitosan/quantum dots nanocomposite and its applications in cells labeling and in vivo imaging. J. Luminescence 145, 74–80. doi:10.1016/j.jlumin.2013.06.036
Li, P., Xu, T., Wu, S., Lei, L., and He, D. (2017). Chronic exposure to graphene-based nanomaterials induces behavioral deficits and neural damage in Caenorhabditis elegans. J. Appl. Toxicol. 37, 1140–1150. doi:10.1002/jat.3468
Liu, H., Mo, L., Hou, M., and Zhang, J. (2020). Life stage-dependent toxicities of 1-ethyl-3-methylimidazolium bromide on Caenorhabditis elegans. Environ. Pollut. 265, 114848. doi:10.1016/j.envpol.2020.114848
Liu, Y., Zhu, S., Gu, Z., Chen, C., and Zhao, Y. (2022). Toxicity of manufactured nanomaterials. Particuology 69, 31–48. doi:10.1016/j.partic.2021.11.007
Lu, J.-H., Hou, W.-C., Tsai, M.-H., Chang, Y.-T., and Chao, H.-R. (2022). The impact of background-level carboxylated single-walled carbon nanotubes (SWCNTs−COOH) on induced toxicity in Caenorhabditis elegans and human cells. Int. J. Environ. Res. Public Health 19, 1218. doi:10.3390/ijerph19031218
Luo, X., Xu, S., Yang, Y., Li, L., Chen, S., Xu, A., et al. (2016). Insights into the ecotoxicity of silver nanoparticles transferred from Escherichia coli to Caenorhabditis elegans. Sci. Rep. 6, 36465. doi:10.1038/srep36465
Lynch, I., Dawson, K. A., Lead, J. R., and Valsami-Jones, E. (2014). Macromolecular coronas and their importance in nanotoxicology and nanoecotoxicology. Nanosci. Environ. 7, 127–156. doi:10.1016/B978-0-08-099408-6.00004-9
Lynch, I., Nymark, P., Doganis, P., Gulumian, M., Yoon, T.-H., Martinez, D. S. T., et al. (2021). Methods, models, mechanisms and metadata: introducing the Nanotoxicology collection at F1000Research. F1000Res 10, 1196. doi:10.12688/f1000research.75113.1
Madima, N., Mishra, S. B., Inamuddin, I., and Mishra, A. K. (2020). Carbon-based nanomaterials for remediation of organic and inorganic pollutants from wastewater. A review. Environ. Chem. Lett. 18, 1169–1191. doi:10.1007/s10311-020-01001-0
Magurany, K. A., Chang, X., Clewell, R., Coecke, S., Haugabrooks, E., and Marty, S. (2023). A pragmatic framework for the application of new approach methodologies in one health toxicological risk assessment. Toxicol. Sci. 192, 155–177. doi:10.1093/toxsci/kfad012
Mahmoudi, M., Landry, M. P., Moore, A., and Coreas, R. (2023). The protein corona from nanomedicine to environmental science. Nat. Rev. Mater 8, 422–438. doi:10.1038/s41578-023-00552-2
Maiti, D., Tong, X., Mou, X., and Yang, K. (2019). Carbon-based nanomaterials for biomedical applications: A recent study. Front. Pharmacol. 9, 1401. doi:10.3389/fphar.2018.01401
Markiewicz, M., Kumirska, J., Lynch, I., Matzke, M., Köser, J., Bemowsky, S., et al. (2018). Changing environments and biomolecule coronas: consequences and challenges for the design of environmentally acceptable engineered nanoparticles. Green Chem. 20, 4133–4168. doi:10.1039/c8gc01171k
Mohan, N., Chen, C. S., Hsieh, H. H., Wu, Y. C., and Chang, H. C. (2010). In vivo imaging and toxicity assessments of fluorescent nanodiamonds in caenorhabditis elegans. Nano Lett. 10, 3692–3699. doi:10.1021/nl1021909
Mullick Chowdhury, S., Dasgupta, S., McElroy, A. E., and Sitharaman, B. (2014). Structural disruption increases toxicity of graphene nanoribbons. J. Appl. Toxicol. 34, 1235–1246. doi:10.1002/jat.3066
Nouara, A., Wu, Q., Li, Y., Tang, M., Wang, H., Zhao, Y., et al. (2013). Carboxylic acid functionalization prevents the translocation of multi-walled carbon nanotubes at predicted environmentally relevant concentrations into targeted organs of nematode Caenorhabditis elegans. Nanoscale 5, 6088. doi:10.1039/c3nr00847a
OECD (2022). Test No. 317: Guidance document on aquatic and sediment toxicological testing of nanomaterials. Paris, France: OECD.
OECD (2017). Test No. 318: Dispersion stability of nanomaterials in simulated environmental media. Paris, France: OECD. doi:10.1787/9789264284142-en
Ou, L., Song, B., Liang, H., Liu, J., Feng, X., Deng, B., et al. (2016). Toxicity of graphene-family nanoparticles: A general review of the origins and mechanisms. Part Fibre Toxicol. 13, 57. doi:10.1186/s12989-016-0168-y
Petersen, E., Barrios, A. C., Bjorkland, R., Goodwin, D. G., Li, J., Waissi, G., et al. (2023). Evaluation of bioaccumulation of nanoplastics, carbon nanotubes, fullerenes, and graphene family materials. Environ. Int. 173, 107650. doi:10.1016/j.envint.2022.107650
Petersen, E. J. (2015). Control experiments to avoid artifacts and misinterpretations in nanoecotoxicology testing. Gaithersburg, MD: National Institute of Standards and Technology. doi:10.6028/NIST.SP.1200-11
Petersen, E. J., and Henry, T. B. (2012). Methodological considerations for testing the ecotoxicity of carbon nanotubes and fullerenes: review. Environ. Toxicol. Chem. 31, 60–72. doi:10.1002/etc.710
Pho, K. B., and MacNeil, L. T. (2019). Biology is the root of variability: cautionary tales in Caenorhabditis elegans biology. Biochem. Soc. Trans. 47, 887–896. doi:10.1042/BST20190001
Pimtong, W., Samutrtai, P., Wongwanakul, R., and Aueviriyavit, S. (2021). “Predictive models for nanotoxicology: in vitro, in vivo, and computational models,” in Handbook of nanotechnology applications (Amsterdam, Netherlands: Elsevier), 683–710. doi:10.1016/B978-0-12-821506-7.00026-0
Pramanik, A., Kole, A. K., Krishnaraj, R. N., Biswas, S., Tiwary, C. S., Varalakshmi, P., et al. (2016). A novel technique of synthesis of highly fluorescent carbon nanoparticles from broth constituent and in-vivo bioimaging of C. elegans. J. Fluoresc. 26, 1541–1548. doi:10.1007/s10895-016-1854-8
Priyam, A., Afonso, L. O. B., Schultz, A. G., and Singh, P. P. (2021). Investigation into the trophic transfer and acute toxicity of phosphorus-based nano-agromaterials in Caenorhabditis elegans. NanoImpact 23, 100327. doi:10.1016/j.impact.2021.100327
Qu, Y., Li, W., Zhou, Y., Liu, X., Zhang, L., Wang, L., et al. (2011). Full assessment of fate and physiological behavior of quantum dots utilizing Caenorhabditis elegans as a model organism. Nano Lett. 11, 3174–3183. doi:10.1021/nl201391e
Rive, C., Reina, G., Wagle, P., Treossi, E., Palermo, V., Bianco, A., et al. (2019). Improved biocompatibility of amino-functionalized graphene oxide in Caenorhabditis elegans. Small 15, 1902699. doi:10.1002/smll.201902699
Scanlan, L. D., Lund, S. P., Coskun, S. H., Hanna, S. K., Johnson, M. E., Sims, C. M., et al. (2018). Counting Caenorhabditis elegans: protocol optimization and applications for population growth and toxicity studies in liquid medium. Sci. Rep. 8, 904. doi:10.1038/s41598-018-19187-3
Schaechter, M., and Neidhardt, F. (1987). “New biological books,” in Escherichia coli and Salmonella typhimurium: Cellular and molecular biology. Editor F. Neidhardt (Washington: ASM Press), 13–16.
Selck, H., Handy, R. D., Fernandes, T. F., Klaine, S. J., and Petersen, E. J. (2016). Nanomaterials in the aquatic environment: A European union–United States perspective on the status of ecotoxicity testing, research priorities, and challenges ahead. Environ. Toxicol. Chem. 35, 1055–1067. doi:10.1002/etc.3385
Shu, C., Yu, X., Wu, Q., Zhuang, Z., Zhang, W., and Wang, D. (2015). Pretreatment with paeonol prevents the adverse effects and alters the translocation of multi-walled carbon nanotubes in nematode Caenorhabditis elegans. RSC Adv. 5, 8942–8951. doi:10.1039/C4RA14377A
Simon, C. G., Borgos, S. E., Calzolai, L., Nelson, B. C., Parot, J., Petersen, E. J., et al. (2023). Orthogonal and complementary measurements of properties of drug products containing nanomaterials. J. Control. Release 354, 120–127. doi:10.1016/j.jconrel.2022.12.049
Singh, H., Sharma, A., Bhardwaj, S. K., Arya, S. K., Bhardwaj, N., and Khatri, M. (2021). Recent advances in the applications of nano-agrochemicals for sustainable agricultural development. Environ. Sci. Process. Impacts 23, 213–239. doi:10.1039/D0EM00404A
Singh, V., Rawat, K. S., Mishra, S., Baghel, T., Fatima, S., John, A. A., et al. (2018). Biocompatible fluorescent carbon quantum dots prepared from beetroot extract for in vivo live imaging in C. elegans and BALB/c mice. J. Mater Chem. B 6, 3366–3371. doi:10.1039/C8TB00503F
Sinha, S., Biswas, K., De, D., and Bandyopadhyay, J. (2016). Implications of multi wall carbon nanotube over living system using C. elegans as worm model. Mater. Today Proc. 3, 3310–3317. doi:10.1016/J.MATPR.2016.10.012
Sivaselvam, S., Mohankumar, A., Thiruppathi, G., Sundararaj, P., Viswanathan, C., and Ponpandian, N. (2020). Engineering the surface of graphene oxide with bovine serum albumin for improved biocompatibility in Caenorhabditis elegans. Nanoscale Adv. 2, 5219–5230. doi:10.1039/D0NA00574F
Sonkar, S. K., Ghosh, M., Roy, M., Begum, A., and Sarkar, S. (2012). Carbon nano-onions as nontoxic and high-fluorescence bioimaging agent in food chain—an study from unicellular to multicellular C. elegans. Mater. Express 2, 105–114. doi:10.1166/mex.2012.1064
Starnes, D. L., Unrine, J. M., Starnes, C. P., Collin, B. E., Oostveen, E. K., Ma, R., et al. (2015). Impact of sulfidation on the bioavailability and toxicity of silver nanoparticles to Caenorhabditis elegans. Environ. Pollut. 196, 239–246. doi:10.1016/j.envpol.2014.10.009
Stavitskaya, A., Fakhrullina, G., Nigamatzyanova, L., Sitmukhanova, E., Khusnetdenova, E., Fakhrullin, R., et al. (2021). Biodistribution of quantum dots-labelled halloysite nanotubes: A Caenorhabditis elegans in vivo study. Materials 14, 5469. doi:10.3390/ma14195469
Tejeda-Benitez, L., and Olivero-Verbel, J. (2016). Caenorhabditis elegans, a biological model for research in toxicology. Rev. Environ. Contam. Toxicol. 237, 1–35. doi:10.1007/978-3-319-23573-8_1
Tsai, M.-H., Chao, H.-R., Jiang, J.-J., Su, Y.-H., Cortez, M. s. P., Tayo, L. L., et al. (2021). Toxicity of low-dose graphene oxide nanoparticles in an in-vivo wild type of Caenorhabditis elegans model. Aerosol Air Qual. Res. 21, 200559. doi:10.4209/aaqr.200559
Wang, J., Dai, H., Nie, Y., Wang, M., Yang, Z., Cheng, L., et al. (2018). TiO2 nanoparticles enhance bioaccumulation and toxicity of heavy metals in Caenorhabditis elegans via modification of local concentrations during the sedimentation process. Ecotoxicol. Environ. Saf. 162, 160–169. doi:10.1016/j.ecoenv.2018.06.051
Wang, M., Chen, J., Liu, C., Qiu, J., Wang, X., Chen, P., et al. (2017). A graphene quantum dots–hypochlorite hybrid system for the quantitative fluorescent determination of total antioxidant capacity. Small 13, 1700709–1700710. doi:10.1002/smll.201700709
Wu, Q., Li, Y., Li, Y., Zhao, Y., Ge, L., Wang, H., et al. (2013a). Crucial role of the biological barrier at the primary targeted organs in controlling the translocation and toxicity of multi-walled carbon nanotubes in the nematode Caenorhabditis elegans. Nanoscale 5, 11166. doi:10.1039/c3nr03917j
Wu, Q., Yin, L., Li, X., Tang, M., Zhang, T., and Wang, D. (2013b). Contributions of altered permeability of intestinal barrier and defecation behavior to toxicity formation from graphene oxide in nematode Caenorhabditis elegans. Nanoscale 5, 9934. doi:10.1039/c3nr02084c
Wu, Q., Zhao, Y., Li, Y., and Wang, D. (2014a). Molecular signals regulating translocation and toxicity of graphene oxide in the nematode Caenorhabditis elegans. Nanoscale 6, 11204–11212. doi:10.1039/C4NR02688H
Wu, Q., Zhao, Y., Zhao, G., and Wang, D. (2014b). MicroRNAs control of in vivo toxicity from graphene oxide in Caenorhabditis elegans. Nanomedicine Nanotechnol. Biol. Med. 10, 1401–1410. doi:10.1016/j.nano.2014.04.005
Wu, Q., Zhou, X., Han, X., Zhuo, Y., Zhu, S., Zhao, Y., et al. (2016). Genome-wide identification and functional analysis of long noncoding RNAs involved in the response to graphene oxide. Biomaterials 102, 277–291. doi:10.1016/j.biomaterials.2016.06.041
Xiao, G., Chen, H., Krasteva, N., Liu, Q., and Wang, D. (2018). Identification of interneurons required for the aversive response of Caenorhabditis elegans to graphene oxide. J. Nanobiotechnology 16, 45–48. doi:10.1186/s12951-018-0373-y
Xu, J., Wang, L., Mu, Y., Zhang, Y., Wang, M., Wang, Q., et al. (2018). Carbon quantum dots as fluorescent probes for imaging and detecting free radicals in C. elegans. J. Nanosci. Nanotechnol. 18, 763–771. doi:10.1166/jnn.2018.15190
Yang, J., Zhao, Y., Wang, Y., Wang, H., and Wang, D. (2015). Toxicity evaluation and translocation of carboxyl functionalized graphene in Caenorhabditis elegans. Toxicol. Res. (Camb) 4, 1498–1510. doi:10.1039/C5TX00137D
Yang, K., Chen, B., Zhu, X., and Xing, B. (2016). Aggregation, adsorption, and morphological transformation of graphene oxide in aqueous solutions containing different metal cations. Environ. Sci. Technol. 50, 11066–11075. doi:10.1021/acs.est.6b04235
Yao, Y., Zhang, T., and Tang, M. (2022). A critical review of advances in reproductive toxicity of common nanomaterials to Caenorhabditis elegans and influencing factors. Environ. Pollut. 306, 119270. doi:10.1016/j.envpol.2022.119270
Zhao, Y., Wu, Q., and Wang, D. (2016). An epigenetic signal encoded protection mechanism is activated by graphene oxide to inhibit its induced reproductive toxicity in Caenorhabditis elegans. Biomaterials 79, 15–24. doi:10.1016/j.biomaterials.2015.11.052
Zhang, W., Wang, C., Li, Z., Lu, Z., Li, Y., Yin, J.-J., et al. (2012). Unraveling stress-induced toxicity properties of graphene oxide and the underlying mechanism. Adv. Mater. 24, 5391–5397. doi:10.1002/adma.201202678
Zhao, L., Kong, J., Krasteva, N., and Wang, D. (2018). Deficit in the epidermal barrier induces toxicity and translocation of PEG modified graphene oxide in nematodes. Toxicol. Res. (Camb) 7, 1061–1070. doi:10.1039/C8TX00136G
Zhao, Y., Hua, X., Rui, Q., and Wang, D. (2023). Exposure to multi-walled carbon nanotubes causes suppression in octopamine signal associated with transgenerational toxicity induction in C. elegans. Chemosphere 318, 137986. doi:10.1016/j.chemosphere.2023.137986
Zhao, Y., Wu, Q., Li, Y., Nouara, A., Jia, R., and Wang, D. (2014). In vivo translocation and toxicity of multi-walled carbon nanotubes are regulated by microRNAs. Nanoscale 6, 4275. doi:10.1039/c3nr06784j
Zhao, Y., Xu, R., Hua, X., Rui, Q., and Wang, D. (2022). Multi-walled carbon nanotubes induce transgenerational toxicity associated with activation of germline long non-coding RNA linc-7 in C. elegans. Chemosphere 301, 134687. doi:10.1016/j.chemosphere.2022.134687
Zhao, Y., Zhi, L., Wu, Q., Yu, Y., Sun, Q., and Wang, D. (2016b). p38 MAPK-SKN-1/Nrf signaling cascade is required for intestinal barrier against graphene oxide toxicity in Caenorhabditis elegans. Nanotoxicology 10, 1469–1479. doi:10.1080/17435390.2016.1235738
Zhu, S., Oberdörster, E., and Haasch, M. L. (2006). Toxicity of an engineered nanoparticle (fullerene, C60) in two aquatic species, Daphnia and fathead minnow. Mar. Environ. Res. 62, S5–S9. doi:10.1016/j.marenvres.2006.04.059
Keywords: new approach methodologies, alternative models, ecotoxicology, nanosafety, nanobiotechnology, nanoinformatics
Citation: Côa F, Bortolozzo LdS, Ávila DS, Souza Filho AG and Martinez DST (2023) Toxicology of carbon nanomaterials in the Caenorhabditis elegans model: current status, characterization, and perspectives for testing harmonization. Front. Carbon 2:1241637. doi: 10.3389/frcrb.2023.1241637
Received: 16 June 2023; Accepted: 07 August 2023;
Published: 06 September 2023.
Edited by:
Gurumurthy Hegde, Christ University, IndiaReviewed by:
Ekaterina Obraztsova, Institute of Bioorganic Chemistry (RAS), RussiaLuiz K. C. De Souza, Federal University of Amazonas, Brazil
Copyright © 2023 Côa, Bortolozzo, Ávila, Souza Filho and Martinez. This is an open-access article distributed under the terms of the Creative Commons Attribution License (CC BY). The use, distribution or reproduction in other forums is permitted, provided the original author(s) and the copyright owner(s) are credited and that the original publication in this journal is cited, in accordance with accepted academic practice. No use, distribution or reproduction is permitted which does not comply with these terms.
*Correspondence: Antonio G. Souza Filho, agsf@fisica.ufc.br; Diego Stéfani Teodoro Martinez, diego.martinez@lnnano.cnpem.br