- School of Electrical Engineering, Korea University, Seoul, South Korea
The unrelenting technological advancement in the generation of wireless networks in recent years has awakened the motion concerning the inclusion of satellites in personal communications. Leveraging their ability to provide wide coverage, uniform services, wide bandwidth, and so forth, Satellite systems will be expected to co-exist with the current state-of-the-art infrastructure of terrestrial networks. Herein, we present a comparative study on the representative digital modulation techniques for use in personal satellite communications. We discuss the advantages and limitations of different modulation techniques, such as phase shift keying, continuous phase modulation, amplitude phase shift keying, and quadrature amplitude modulation. We also perform evaluations based on spectral efficiency, power efficiency, modulation error ratio, error vector magnitude, and peak-to-average power ratio in the presence of high power amplifier nonlinearities and Doppler effects. Comparisons in the form of tables, illustrations, and curves are also presented. In correspondence to the comparisons made basing on the aforementioned metrics, we conclude that continuous phase modulation is the best candidate modulation scheme for personal satellite communications since it outperforms other schemes by compromising the trade-off between power efficiency, bandwidth efficiency, and immunity to errors. We further present open issues that would reinforce personal satellite communications in terms of reliability, throughput and latency, other than power and spectral efficiency, if combined with appropriate modulation schemes.
1 Introduction
The unrelenting technological advancement in the generation of wireless networks has spawned an exponential increase in the demand for spectrum to support the resulting traffic. One of the reasons for the skyrocketing demand for resources could be arising from the dramatic increase in the manufacturing of complex very large scale integration (VLSI) chips that enable swift data transmission between devices (Insights, 2021). Consequently, these unprecedented challenges by traffic increase could overwhelm the existing terrestrial network (TN) infrastructure in the future. One of the promising solutions could be data traffic offloading (Dimatteo et al., 2011; Lee et al., 2013) supported by different networks, such as wireless local area networks (WLANs) and heterogeneous networks. Nevertheless, performing data offloading to the existing networks has several concerns, such as the implementation cost, complexity, power consumption, security, and privacy, to mention but a few. Another way to improve spectrum usage could be through modulation. However, it poses a threat to power efficiency of the communications system as discussed in the next sections. More research will be needed to overcome the aforementioned problems to realize optimal network architectures, which are bandwidth- and power-efficient, reliable, and high throughput with low latency.
In the light of such requirements, satellites (SATs) can be considered as a complement to the existing TN to support personal communications, especially during insufficient coverage, in the presence of terrain constraints, network overloads, and emergency situations.
Moreover, in the next generation of networks, other technologies, such as free space optical and millimeter wave communications could be considered. However, these are constrained by atmospheric channel, transmission distances, and frequencies (Rangan et al., 2014; Kaushal and Kaddoum, 2017). Hence, this makes the application of SATs to personal communications substantial. A personal communication system relates to the liberty possessed by an individual to access reliable communication while on the move. Personal communications may include wireless communications in the form of data, voice, video, and beyond.
Over the recent years, next-generation SAT systems have been conceived as a key reinforcement for future TN, such as 5G and 6G, with the aim of supporting versatile applications (Kodheli et al., 2017; Boero et al., 2018; Gopal and BenAmmar, 2018; Su et al., 2019). Rigorous research is underway by both academia and industry towards this common cause. For example, one of the world’s leading communications standardization bodies known as the 3rd Generation Partnership Project (3 GPP) 1 has been pivotal in conducting studies to determine the specifications for the anticipated integrated SAT-TN. In (3GPP TR 38.811 V15.4.0, 2020), 3 GPP presents studies on 5G to support non-terrestrial networks (NTN). Therein, discussions related to NTN architecture, channel modeling, and potential impact considerations are provided. The authors in (Su et al., 2019) discuss SAT constellations, interference, and resource management for broadband low earth orbit (LEO) SAT deployment. The authors in (Sadek and Aissa, 2012) present the challenges facing SATCOMs and the state-of-art SATs. They discuss challenges concerning SAT-TN integration with a focus on transparency and interference.
1.1 Motivation
Unlike TNs, which are mainly limited by adjacent channel interference (ACI), NTNs are mainly limited by power due to thermal noise and bandwidth due to bandwidth restrictions (Rydbeck et al., 1996). Therefore further studies will be required to address several challenges related to modulation and coding, link margin requirements, frequency planning and orbit choice all together.
This paper contains the study of modulation techniques to find suitable solutions that can optimize efficiencies while minimizing the trade-offs which exist during the design of personal satellite communication (SATCOM) systems. Additionally, the studies seek to explore the drawbacks that are introduced by modulation in the pursuit of long-distance transmission and boosting the signal’s immunity to interference.
The motivation of this work is derived from three intriguing aspects, namely the integration of NTN and TN (3GPP TR 38.811 V15.4.0, 2020), the existence of nonlinear distortions during signal amplification (Simon, 2005), and the inevitable Doppler effects in communications (Jakes and Cox, 1994; Ali et al., 1998). From the network perspective, TNs have proved capable of providing low-latency and higher data rates. However, due to uncertain changes in customers’ appetite for traffic, and need for wider coverage, TNs are incapacitated, hence drawing attention to the need for integration between NTN and TN. High power amplifier (HPA) nonlinearities, Doppler shift, and propagation delay are some of the most prominent setbacks in SATs, which need immediate solutions. Owing to long-distance transmission in SATs, a high transmit output power is required to guarantee sufficient reception power at the receiver side. Therefore, it would require that the HPAs be operated very close to the saturation point to maximize power efficiency. As a result, HPA nonlinearities are introduced into the transmitted signal. Additionally, the highly mobile nature of SATs and terrestrial users induces variations in carrier frequency and longer propagation delays, which can affect channel estimation. Consequently, frequent handovers, and large signaling overhead are necessary to maintain service continuity (Del Re and Pierucci, 2002a) 2.
Going forward, the discussion herein seeks to pursue power- and spectral-efficient modulation schemes with the given transmitted power and channel bandwidth requirements. Such principal resources require considerable attention since they are not only vulnerable to channel uncertainty but also other constraints, such as HPA nonlinearities and Doppler effect, which are associated with SATCOMs. The utilization of such resources results in maximization of the bandwidth efficiency which is attained at the minimum cost of the average signal power. In this paper, it should be noted that the terms “spectral” and “bandwidth” are used interchangeably.
To improve bandwidth efficiency, spectral-efficient modulation schemes could be deployed, albeit at the cost of higher power requirement and degraded bit error rate (BER) performance. On the other hand, error-correction can be used to attain a given BER performance level with reduced power requirement at the expense of decreased bandwidth efficiency and increased complexity. This trade-off between bandwidth and power attracts the quest for not only bandwidth-efficient but power-efficient modulation schemes. It is also important for power budget, HPA, and waveform designs, etc. Another driving point for this study comes from the fact that modulation significantly contributes to latency, reliability, and throughput. For example, higher-order modulation techniques allow faster transmission rates, and hence higher throughput and low latency. On the other hand, deploying low-order modulation schemes can guarantee reliability.
1.2 Major Contributions
In this paper, we provide literature reviews on SATs and the influence of HPA nonlinearities and Doppler effect on digital modulation schemes for suitable adoption in personal SATCOMs. We summarize our contributions in this paper as follows.
• We introduce detailed personal SATCOMs aspects and share various design rationales. We discuss the need for transition from the traditional stationary SAT networks to the highly mobile SATs, accompanied with the expected personal SATCOM architecture, on-board processing (OBP) capability, channel characteristics, and HPA requirements. We also present the expected benefits and hurdles related to the integration between NTN and TN.
• We provide holistic treatment of modulation requirements for personal SATCOM. This comprehensive contribution enriches the existing surveys and tutorials, which are mostly limited to the study carried out for traditional stationary SAT networks. We examine the strengths and limitations of phase shift keying (PSK), continuous phase modulation (CPM), amplitude phase shift keying (APSK), and quadrature amplitude modulation (QAM). We also subject representative modulation schemes to HPA nonlinearities and Doppler effects, and conduct evaluations based on spectral efficiency, power efficiency, modulation error ratio (MER), error vector magnitude (EVM), and peak-to- average power ratio (PAPR).
• Finally, we discuss the future directions that would reinforce personal SATCOMs, not only in terms of spectral and power efficiency, but also reliability, throughput and latency if combined with appropriate modulation schemes.
The remainder of this article is organized as follows. In Section 2, we present a summary of works related to modulation schemes for TN and NTN. A brief background about SAT orbits, their features, and corresponding services is also presented in this section. In Section 3, we provide a review of the system requirements. In Section 4, modulation techniques for personal SATCOM are introduced. A glimpse of performance comparisons for selected modulation techniques is presented in Section 5. Finally, future and open topics, and a conclusion are presented in Section 6 and Section 7, respectively. To allow the proper flow of this survey, we also the provide a list of abbreviations in Table 1 and the structure of the paper in Figure 1
2 Related Works and Background
2.1 Related Works
Several works involving diverse modulation-based approaches to support various applications both in TN and NTN have been proposed. However, it cannot be inferred that these would be long-lasting solutions to the unprecedented changes in traffic demands especially with the inclusion of SATs in personal communications. Hence, further studies will be necessary.
The authors in (Flohberger et al., 2010) proposed a feature-based method for classification of modulation schemes to aid the design of intelligent receivers in SATCOMs. In (Cardarilli et al., 2002), viable strategies for implementing flexible and fully programmable digital modulator architectures for SAT and space applications were proposed. The BER comparison of advanced modulation schemes for LEO SAT downlink communications was discussed in (Belce, 2003). The authors in (Keysight Technologies, 2020) provided an analysis of the impact of phase noise on the quality of signals for modulation techniques applicable to SATCOMs. In (Oetting, 1979), the author summarizes the characteristics of modulation techniques most applicable to digital radios. Therein, the main comparison is centered on BER which is presented in the form of tabled numerical results.
With the acknowledgement of the existing and ongoing works, supplemental attention towards achieving spectral efficiency and power efficiency, such as adopting robust modulation schemes in integrated SAT-TN, is paramount. Moreover, most of the available works focused on either modulation with application to geostationary orbit (GEO) SATs or cellular systems as standalone networks but not as an integrated system. In our studies we consider an integrated system of LEOs and TN with emphasis on the severe HPA nonlinearities and Doppler effects. Furthermore, our performance metrics go beyond BER which is widely considered by most authors3.
2.2 Background
2.2.1 Different Platforms of NTN
Generally, SATs operate in three main orbits namely, LEO, medium earth orbit (MEO) and GEO (see Figure 2). Other NTN platforms constitute unmanned aircraft system (UAS) including high altitude platform station (HAPS) whose altitude ranges from 8 to 50 km (20 km for HAPS), and high elliptical orbit (HEO) SATs located between 400 and 50,000 km (Su et al., 2019; 3GPP TR 38.811 V15.4.0, 2020).
GEO SATs provide the largest footprint as well as high throughput. However, these experience long round-trip time. Furthermore, servicing a SAT in GEO is quite complex and costly, for instance, replacing faulty components. On the other hand, SATs in MEO and LEO orbits can achieve lower delays compared to GEO but are greatly affected by radiation in the inner Van Allen belt and atmospheric drag (International Telecommunications Union, 2002). Another highly pronounced bottleneck problem in LEO is the Doppler effect (Ali et al., 1998) resulting from high mobility. The aforesaid problems create a trade-off when deciding which orbit to use for a given service.
2.2.2 Category of SAT Services
Incorporating SAT systems into personal communications is potential for global connectivity and enhancing improved service delivery in different economies of the world. Moreover, SATs provide ubiquitous coverage with a possibility of co-existing with TN. Consequently, this co-existence would enable integration of SATs with TN, hence, resulting in higher data rates and high quality of service anywhere anytime. SAT services can be categorized into three (F. R. Group, 2021), namely fixed SAT services (FSS) which provide links between terminals at fixed locations on earth, mobile SAT services (MSS) which provide links from or to mobile terminals and broadcast SAT services (BSS) for broadcasting to multiple receiving stations. Table 2 summarizes some of the service providers for the current state-of-the-art SATs, while Table 3 presents several SAT services with corresponding frequency bands.
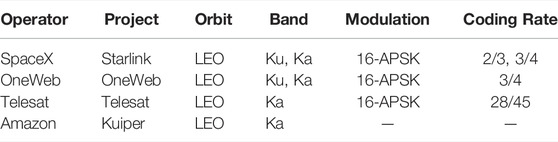
TABLE 2. Comparison of state-of-the-art industry SATCOM project (Sadek and Aissa, 2012; Evans, 1997; Minoli, 2015; Sheriff and Hu, 2001; Penttinen, 2015; Del Portillo et al., 2019; Hindin, 2019) 2–11.
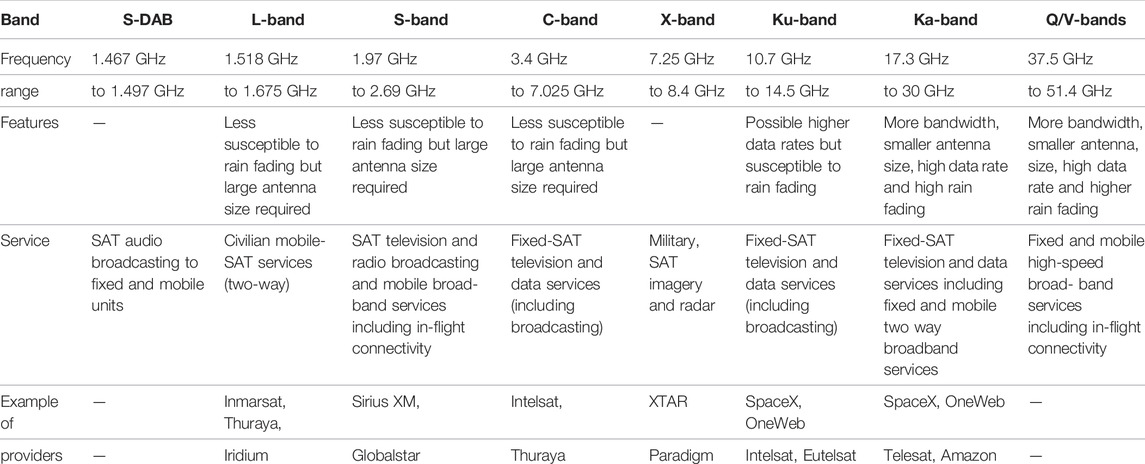
TABLE 3. Characteristics of spectrum for SATCOM (F. R. Group, 2021; Penttinen, 2015; EMEA Satellite Operators Association, 2021; T. E. S. Agency, 2021).
3 System Requirements
3.1 Channel Characteristics
In traditional SATCOMs, the user terminal is connected to non-stationary SAT networks and receives the signal mainly through the gateway (ground station). In contrast, the next generation of personal SATCOMs (see Figure 3) will require a direct link between the SAT and user terminal. Similar to the traditional links, the signal quality of the direct link is affected by several environmental factors, such as rain and cloud attenuation, scintillation, and atmospheric absorption with corresponding elevation angle, altitude above sea level, frequency, and water vapor densities (Panagopoulos et al., 2004; Kanatas and Panagopoulos, 2012). Besides, by considering the deployment scenario of personal SATCOMs, Doppler, shadowing, and multipath effects become severe. Especially, the Doppler effect becomes a serious issue for the personal SATCOMs, due to its fast orbital speed (e.g., around 7.8 km/s) and its high operating frequency (10 ∼30 GHz) (3GPP TR 38.811 V15.4.0, 2020). Figure 4 illustrates the variation of Doppler shift with orbital height. It shows that the lower the altitude of the SAT, the more severe the Doppler effect becomes. Since lower orbits are characterized by large gravitational forces, SATs accelerate faster to minimize the effect of gravity while maintaining the orbit. This leads to increased relative motion associated with high velocities between the SAT and user terminal4.
Therefore the choice of channel model and the band of operation will be important in designing several parameters of the overall integrated system, such as estimating fade margins, assessing the efficiency of modulation and coding schemes, to mention but a few. Some of the notable land mobile SAT channel models are described in (Abdi et al., 2003; Corazza and Vatalaro, 1994; Chun Loo, 1985). Over the past years, due to an increase in the number of service providers and change in traffic demand, lower frequency bands, such as L (1.518 GHz ∼1.675 GHz), S (1.97 GHz ∼2.69 GHz), and C (3.4 GHz ∼7.025 GHz), became saturated, which led to the scramble for higher frequency bands, namely Ku (10.7 GHz ∼14.5 GHz), Ka (17.3 GHz ∼30 GHz), and Q/V (37.5 GHz ∼51.4 GHz) (EMEA Satellite Operators Association, 2021). We briefly summarize the available bands for SAT and their features in Table 3. It is worth noting that there is a trade-off that exists between the choice of band and performance. For instance, lower frequency bands are characterized by less susceptibility to rain attenuation but only achieve lower data rates and require large antenna sizes, whereas higher frequency bands are more susceptible to rain fading though they allow deployment of smaller antenna sizes and higher data rates can be achieved due to availability of more bandwidth. More research about fade mitigation techniques for personal SATCOMs is required to improve interference control, system capacity, and availability. For example, in (Castanet et al., 2003), the authors discuss interference and fade mitigation techniques for Ka and Q/V band SATCOMs based on power control, adaptive waveforms, and diversity5.
3.2 High Power Amplifiers
With the help of a chain of components (transponder), the SAT is able to process uplink signals and retransmit them towards the earth station receiver via the downlink. Figure 5 shows the block diagram of a regenerative SAT transponder whose basic function is to recover, amplify and frequency shift the input signal. The input multiplexer (IMUX) splits the received wide band signals into narrow band channels Processes, such as signal predistortion, demodulation and remodulation, are done by OBP (3GPP TR 38.811 V15.4.0, 2020; Perez-Neira et al., 2019). Output signals from the HPA are combined by the output multiplexer (OMUX) and redirected towards the earth station receiver. Therefore, modulation techniques customized for personal SATCOMs will be required to support the OBP requirements. To this end, HPA serves as one of the most important stages of the SATCOM system. The HPA performance may be evaluated basing on output power, efficiency, and sideband regrowth (Gard et al., 1999).
Regardless of the transmission distance, the existence of path-loss and atmospheric-induced fading requires digital communications systems to sustain adequate reception power at the receiver side. Moreover, HPAs consume about 65% (Gruber et al., 2009) and 80–90% (Lohmeyer et al., 2016) of the available power in TN and SATCOMs, respectively. It is therefore of great necessity to deploy a device capable of generating sufficient transmitter output power based on fixed-but-limited available power. Some of the examples of such devices include solid-state power amplifiers (SSPAs) and traveling-wave tube amplifiers (TWTAs). Generally, the frequency band of operation and the type of application are the key constraints when selecting an amplifier for deployment. For example, for a typical HPA output power range (10–300 W), TWTAs provide more output power at higher frequency bands while SSPAs achieve greater power output at lower frequency bands (Lohmeyer et al., 2016)6.
By operating these HPAs in the linear region with a large input back-off, spectral regrowth in the transmitted signal can be mitigated. Otherwise, spectral regrowth can lead to build up of out-of-band (OOB) side lobes which might result in severe ACI. However, it is costly to deploy linear HPAs with a large dynamic range. Therefore, to maximize power efficiency, it would require that the HPAs be operated very close to the saturation point (Mulinde et al., 2013). As a consequence, undesirable amplitude modulation-amplitude modulation (AM-AM) and amplitude modulation-phase modulation (AM-PM) conversions (distortions) are introduced into the transmitted signal (see Figure 6). AM-AM is due to the change in output voltage, while AM-PM results from change in phase angle of the output voltage for several input signal levels. The desired levels of AM-AM and AM-PM conversions are 1dB/dB and 0rc/dB, respectively (Lohmeyer et al., 2016).
On the other hand, operating in a nonlinear mode at or near saturation incurs spectral spreading which results from the nonlinearity caused by band-limiting the modulation prior to amplification. A replica of distortion terms for the TWTAs and SSPAs can be characterized using models, such as Saleh, Rapp, and Gorbani (Saleh, 1981; Ghorbani and Sheikhan, 1991; Rapp, 1991). It should be noted that the TWTA model is mostly used to characterize HPA nonlinearities in this paper as illustrated by Figure 7.
Figure 8A, Figure 8D, and Figure 8G illustrate the deformation on the constellations due to AM-AM distortions for 16-QAM, 16-APSK, and 16-CPM, respectively. They show the occurrence of the warping effect whereby the amplified signal’s constellation points get compressed inwards, and they no longer lie on the original lattice points. 16-QAM is the most affected whereas 16-APSK and 16-CPM maintain their shapes. Figure 8B, Figure 8E, and Figure 8H display the deformation on the constellations due to AM-PM distortions for 16-QAM, 16-APSK, and 16-CPM, respectively. They show the clustering effect as the amplified signal constellation points are spread out in small clusters resulting from nonlinear inter-symbol interference (ISI). The impact is most pronounced in 16-QAM. Figure 8C, Figure 8F, and Figure 8I portray the resultant constellation structures after subjecting the 16-QAM, 16-APSK, and 16-CPM signals to a combination of AM-AM and AM-PM distortions. It is shown that 16-CPM is the least sensitive to HPA nonlinearities compared to APSK and QAM.
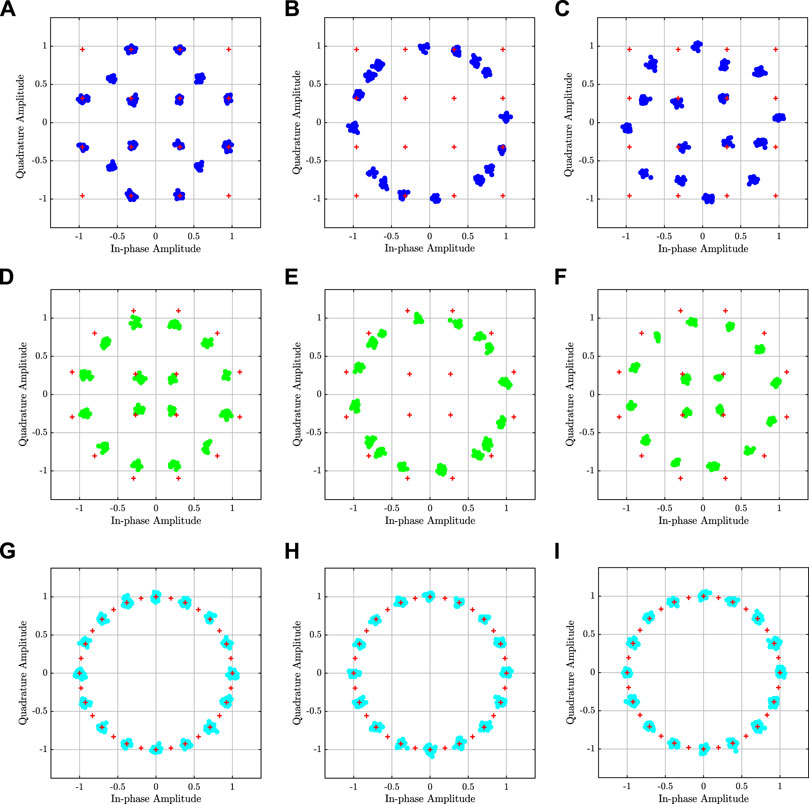
FIGURE 8. Effect of amplification on the constellation structures of 16-QAM, 16-APSK and 16-CPM, modeled using TWTA (Saleh model). Here, (A,D,G) represent AM/AM distortions, (B,E,H) represent AM/PM distortions, while combined AM/AM and AM/PM distortions are illustrated in (C,F,I).
Several techniques have been proposed to combat the effect of nonlinear distortions at the transmitter side in the form of pre-distortion and post-distortion at the receiver side through nonlinear equalization (Ding et al., 2004; Beidas, 2011; Beidas et al., 2015; Joung et al., 2015). Authors in (Joung et al., 2015) provide a summary of HPA centric techniques with an emphasis on the design of HPAs, signals, and networks to improve HPA linearity and efficiency. It should be noted that the extent to which nonlinearity affects the communications system is mainly dependant on the power requirement and modulation scheme in use (Corazza, 2007). Leveraging the ramifications of HPA nonlinearities due to modulation and hardware drawbacks, we are motivated to examine the various modulation schemes for suitability in personal SATCOMs.
It should be noted that besides HPA nonlinearities, other impairments (Horlin and Bourdoux, 2008) on the NTN-TN channel which are not discussed in this paper such as phase noise, carrier frequency offset and I/Q imbalance, are important and therefore equally require research to ensure a joint boost in system performance7.
4 Modulation Techniques
Modulation involves pre-conditioning the signal into a suitable form for transmission over the communication channel. Other than analog, this work focuses more on digital modulation schemes since the latter have exhibited better features, such as more resilience to distortion, reliable and flexible circuitry, cost effectiveness, to mention but a few. Through digital modulation, it is possible to symmetrically split and combine signal components at transmission and reception stages, i.e., in-phase and quadrature components. Modulation has several benefits, such as boosting the signal’s immunity to interference, supporting long-distance transmissions, and multiplexing of different signals. Modulation also makes it possible to reduce antenna sizes.
Generally, the criterion of choosing modulation techniques depends on factors, such as power efficiency, robustness to channel impairments, bandwidth efficiency, and system complexity (Agilent Technologies, 2000). Power efficiency ensures reliable transmission of data with minimal power requirements. A modulation technique that is robust to channel impairments achieves a low BER in the presence of Doppler effects, path-loss, and other severe channel conditions. Low complexity in system design can guarantee affordability. A bandwidth-efficient modulation technique ensures minimum power radiation into adjacent bands hence reducing the effect of ACI (Stuber, 1996). From a practical network perspective, bandwidth is a scarce resource that can only guarantee seamless service if used effectively. In that regard, the transmitted signal is filtered to reduce radiation into adjacent bands. Proper filtering can alleviate the occurrence of ISI, resulting in a drastic boost in spectral efficiency. Contrastingly, the rise and fall times of the filter may also introduce ISI, which could further degrade the performance. Filter design and implementation is also quite complicated especially at higher frequencies. Furthermore, if the filtering is done before amplification, HPA nonlinearities can revert the effect of the filter. To this end, some advanced modulators with integrated predistortion functionalities will be necessary for the next generation of personal SATCOM networks.
In this paper, the discussed modulation schemes are grouped into near constant or constant envelope modulations including PSK and CPM, and nonconstant phase envelope modulations, such as APSK and QAM (Stuber, 1996; Agilent Technologies, 2000; Proakis, 2000; Ziemer, 2001; Anderson et al., 2013). The term constant envelope is derived from the fact that all points on the constellations have a fixed distance from the center. In other words, no signal is modulated on the amplitude (Lee, 1998). It should be noted that in some literature, for instance, the authors in (Xiong, 2006) describe APSK as a constant envelope modulation scheme, however, based on the APSK constellation design and definition of a constant envelope modulation scheme from (Lee, 1998), we consider APSK as a nonconstant envelope modulation scheme. Table 4 presents a summary of strengths, limitations, and major applications for the selected modulation schemes.
4.1 PSK
PSK waveforms may include, binary phase shift keying (BPSK), quadrature phase shift keying (QPSK), and M-ary phase shift keying (M-PSK). PSK schemes, such as BPSK and QPSK are known for their good BER and good power efficiency, hence are suitable for poor channel conditions. However, the performance of PSK schemes is limited by some constraints. For example, for the case of BPSK, coherent detection requires carrier phase recovery, hence making receiver design quite complex (Lee, 1998; Goldsmith, 2005), whereas QPSK incurs rapid phase variations between symbols which results in poor spectral efficiency8.
In SATCOMs, the variants of QPSK, namely offset quadrature phase shift keying (OQPSK) (Gronemeyer and McBride, 1976) and differential quadrature phase shift keying (π/4-DQPSK) (Lee, 1998), can be deployed to achieve better spectral efficiency since they prevent the problem of zero crossings in conventional QPSK. These schemes can support the use of less linear but power-efficient amplifiers in SATCOMs. Figure 9 portrays the constellation diagrams of BPSK, QPSK, and the variants of QPSK. Spectral efficiency as well as higher data rates can further be achieved by increasing the size of modulation size (M), i.e., deploying higher order M-PSK schemes (Haykin, 2008; Rice, 2009). However, this is achieved at the cost of BER performance since the constellation points draw closer to each other as the phase difference between carrier states decreases, thereby rendering the system more sensitive to channel impairments.
4.2 APSK
APSK (Liu et al., 2011; Thomas et al., 1974) seeks to solve the problem of increased risk of errors resulting from the increment of the modulation size of PSK by distributing the same number of constellation points across different concentric circles. The APSK constellation makes it easy to implement the predistortion of HPA nonlinearities through varying the space between rings. Moreover, compared to schemes like QAM, APSK systems have a lower number of amplitude levels (see Figure 10), thus exhibit a lower PAPR. Consequently, having a low PAPR can promote good spectral efficiency and robustness to HPA nonlinearities in SATCOMs. Due to the aforementioned features, an optimized version of APSK was adopted in digital video broadcasting SAT-second generation (DVB-S2) (ETSI, 2006). Unfortunately, it is yet to find its application in cellular networks since it is computationally complex and no Gray mapping exists for APSK constellations, which results in a high independent demapping loss (Liu et al., 2011; Xie et al., 2012). However, efforts to further optimize APSK for extended application in communications have been going on over the past years (De Gaudenzi et al., 2006; Morello and Mignone, 2006; Kayhan and Montorsi, 2012).
4.3 CPM
Linear modulation techniques, such as PSK and QAM exhibit phase discontinuity, a complication which can lead to the widening of the frequency spectrum. Fortunately, by deploying CPM (Aulin et al., 1981; AulinSundberg and Sundberg, 1981; Anderson et al., 2013), phase discontinuity can be eliminated, resulting in improved spectral efficiency and noise immunity of SATCOMs. CPM also induces a coding gain resulting from the inherent memory introduced by the phase-shaping filter (Rimoldi, 1988). However, BER analysis of CPM signals, such as continuous phase frequency shift keying (CPFSK) is quite complex (Aulin and Sundberg, 1984; Goldsmith, 2005). In practical scenarios, more correlators would be required.
Besides CPFSK, other notable examples of CPM waveforms, distinguished by frequency pulse responses, include minimum shift keying (MSK) (Pasupathy, 1979), Gaussian minimum shift keying (GMSK) (Murota and Hirade, 1981), to mention but a few. CPM has been adopted in SATCOMs (ETSI, 2012), deep space (Simon, 2005), optical communications (Ho, 2005), and so on.
4.4 QAM
Compared to other modulation schemes, the rings of QAM (Proakis, 2000) constellation are usually greater in number (see Figure 10). This yields higher amplitude levels and hence poor PAPR. Additionally, the uneven spacing of the rings with some closer to others makes it harder to mitigate HPA nonlinearities (Stuber, 1996; Keysight Technologies, 2020). Although QAM exhibits a high BER and poor power efficiency compared to constant and low-order modulation schemes, such as BPSK and QPSK, it boosts of high bandwidth efficiency since it conveys more information via both the amplitude and phase (Ziemer, 2001). Therefore, it can be used when the channel conditions are good to achieve higher data rates while maintaining tolerable spectral efficiency in personal SATCOMs. QAM is widely used in mobile communications, cable TV, Wi-Fi, and others (Agilent Technologies, 2000).
4.5 Waveforms
Over the recent years, multitone modulation techniques, such as OFDM have exhibited good performance in TN and therefore attract attention for their applicability in the next generation of SAT systems. OFDM (Bingham, 1990; Stuber, 1996) is widely used in mobile and power-line communications. In SATCOMs, OFDM scheme has been adopted in DVB-SH (ITU-R S.2173-1, 2014). OFDM is a successor waveform to the prominent CDMA (Pickholtz et al., 1982; Cook and Marsh, 1983) which is widely used in both TN and SATCOMs because of its good capacity and robustness to ACI. However, compared to SATCOMs, due to topography and terrestrial environment, the problem of ISI in TN resulting from extreme multipath conditions makes it complex to perform equalization. Hence, giving OFDM the edge over CDMA in terms of maximizing data rate and the aforementioned interference problems (Chang, 1966; Saltzberg, 1967)9, 10.
OFDM is famous for its simplicity to implement the equalizer, which results from its ability to convert a frequency-selective channel into several parallel independent frequency-flat subchannels (Saltzberg, 1967; Weinstein and Ebert, 1971; Bingham, 1990). In this way, OFDM achieves a double performance gain from modulation and multiplexing. OFDM has several advantages, such as high spectral efficiency, low complexity receiver design, and robustness against multi-path propagation (Bingham, 1990; Dahlman et al., 2013; Rohde and Schwarz, 2021). Nevertheless, OFDM harbors imperfections, such as a high PAPR (Yiyan Wu and Wu, 2008), sensitivity to frequency and timing offsets (Rohde and Schwarz, 2021), and OOB emission (Van De Beek and Berggren, 2008; Michailow et al., 2012). Since OFDM has a high PAPR, to prevent compression at a high output power level, a large back-off is required (Keysight Technologies, 2020). Hence more research is required to attain a waveform that is both spectral- and power-efficient with considerable sensitivity to frequency and timing offsets. For instance, the authors in (Tan and Stuber, 2002; Thompson et al., 2008; Mulinde et al., 2013; Rahman et al., 2018; Rohde and Schwarz, 2021) discuss generalized frequency shift keying (GFDM), filter-bank multi-carrier (FBMC), constant-envelope single-carrier frequency division multiple access (CE-SC-FDMA), and others, as alternative waveforms for OFDM due to their property of low PAPR.
5 Comparison of Modulation Schemes
This section presents a comparative study of the representative modulation techniques subject to HPA nonlinearities and Doppler effects as a backup for the discussions given in the earlier sections. Table 5 provides a summarized comparative analysis for the selected modulation schemes. It is worth noting that all the results provided in this paper are obtained through simulations in Matlab. The channel model considered in this paper is adopted from (Lin et al., 2016). Doppler shift computation is done using the approach in (3GPP TR 38.811 V15.4.0, 2020). The theoretical equations in (E. TR101290, 2001) are used to compute MER and EVM. To simulate power and bandwidth efficiency, we adopt the theoretical approach in (Akhtman and Hanzo, 2009). We carryout PAPR computations using the formulation in (Cho et al., 2010).
5.1 Error Performance
Besides BER, MER and EVM can be used to measure the accuracy of the modulation technique by comparing the demodulated signal with the reference signal. MER reflects the ratio between the transmitted signal power and the power of error vectors, while EVM represents the magnitude of the difference vector between the I/Q reference signal vector to the I/Q measured signal vector. We exploit MER and EVM as metrics to evaluate error performance of selected modulation schemes. Compared to higher-order modulation schemes, low-level modulation schemes exhibit lower BER performance, and are hence highly recommended for low signal-to-noise ratio (SNR) channel conditions. However, the reverse is true when it comes to MER and EVM regardless of the channel conditions, as depicted in Figure 11 and Figure 12.
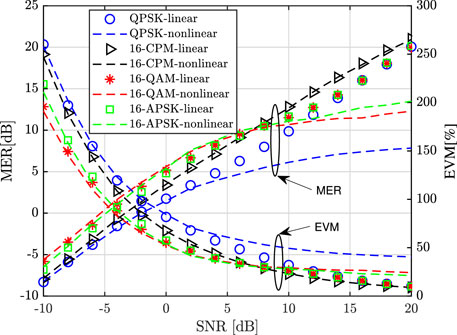
FIGURE 11. MER and EVM performance for QPSK, 16-CPM, 16-QAM, and 16-APSK in the presence of nonlinearities modeled using TWTA (Saleh model).
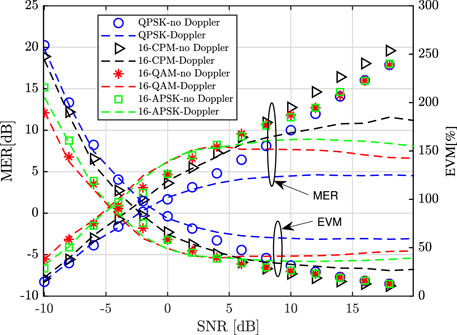
FIGURE 12. MER and EVM performance for QPSK, 16-CPM, 16-QAM, and 16-APSK in the presence of Doppler effects with the velocity of the terminal, 500 km/h, carrier frequency, 20 GHz, SAT at a height of 600 km, maximum elevation angle, 60rc, and the inclination angle of the SAT orbit, 52rc.
Figure 11 shows the trade-off between MER and EVM for representative modulation schemes in the presence of HPA nonlinearities. It shows that for low SNR (for example, at -10 dB), QPSK has the lowest MER (-8.3 dB) and highest EVM (258%) while QAM has the highest MER (-5.62 dB) and the lowest EVM (190%) under the influence of HPA nonlinearities. QAM has a larger distance between the constellation points which helps maintain lower EVM. Unlike CPM, the MER and EVM of QPSK, APSK, and QAM deteriorate due to nonlinear distortions. CPM is able to maintain its performance since it has a constant envelope and reduced phase discontinuity, i.e., at -10 dB SNR, MER = -7.86 dB and EVM = 250% while at 20 dB SNR, MER = 22 dB and EVM = 10%.
Figure 12 portrays the trade-off between MER and EVM for representative modulation schemes in the presence of Doppler shift. It shows that despite all modulation schemes being affected by the Doppler phenomenon, CPM is the most robust scheme followed by APSK, QAM, and QPSK in that order. Having a constant envelope gives CPM an advantage over the rest of the selected modulation schemes. For instance at 20 dB SNR, the MER = 4.6 dB, 11.4 dB, 6.6 dB, and 8 dB and EVM = 58.8%, 26.4%, 46.2%, and 38% for QPSK, CPM, QAM and APSK, respectively11.
From the above results, it can be noted that a high MER and a low EVM would be desirable for a stable communication system. All modulations are affected by HPA nonlinearities and Doppler shift; however, CPM strikes a balance between MER and EVM, hence, proving suitable for applicability to personal SATCOMs.
5.2 Spectral Performance
We consider bandwidth efficiency, power efficiency, and PAPR as metrics to evaluate the spectral performance of our selected modulation schemes In conventional communication systems, low-level modulation schemes are preferred for worst case channel conditions due to their low power requirement which is attained by sacrificing the bandwidth as displayed in Figure 13 and Figure 14.
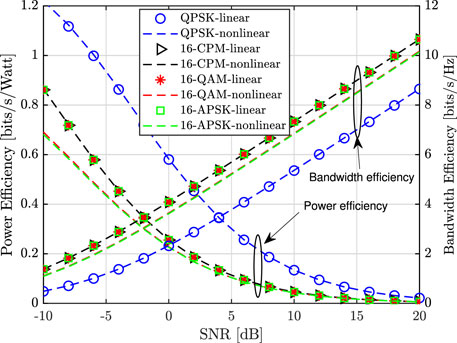
FIGURE 13. Power and bandwidth efficiency for QPSK, 16-CPM, 16-QAM, and 16-APSK in the presence of nonlinearities modeled using TWTA (Saleh model).
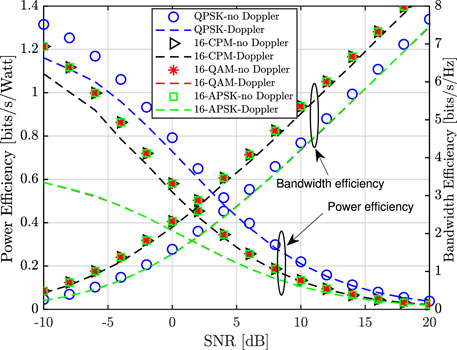
FIGURE 14. Power and bandwidth efficiency for QPSK, 16-CPM, 16-QAM, and 16-APSK in the presence of Doppler effects with the velocity of the terminal, 500 km/h, carrier frequency, 20 GHz, SAT at a height of 600 km, maximum elevation angle, 60rc, and the inclination angle of the SAT orbit, 52rc.
Figure 13 illustrates the trade-off between power and bandwidth efficiency for representative modulation schemes in the presence of HPA nonlinearities. It shows that QPSK has the greatest power efficiency but lowest bandwidth efficiency compared to CPM, QAM, and APSK. For example, in the presence of HPA nonlinearities, the power efficiency at -10dB SNR is about 1.2 and 0.68 bits per second per Watt (bps/W), for QPSK and APSK, respectively. The corresponding bandwidth efficiency at 20dB SNR is about 8.65 and 10.14 bits per second per hertz (bps/Hz) for QPSK and APSK, respectively. It should be noted that CPM has the highest bandwidth efficiency (10.64 bps/Hz) and ranks second in power efficiency (0.86 bps/W). Besides CPM, QPSK is also robust to nonlinear distortions but preference would be given to CPM which has better spectral efficiency due to carrying more data.
Figure 14 displays the trade-off between power and bandwidth efficiency for representative modulation schemes in the presence of Doppler effects. Generally, all modulation schemes are affected by Doppler shift. Nonetheless, CPM appears the most robust to Doppler effects with considerable performance in terms of power efficiency and bandwidth efficiency, i.e., the degradation in power efficiency is compensated for with a relatively low degradation in bandwidth efficiency. It also shows that nonconstant envelope modulation schemes are the most prone to degradation by Doppler. Hence more power would be required to sustain good signal quality. For example, at -10dB SNR, the power efficiency is 1.6 bps/W, 1.09 bps/W, and 0.59 bps/W, for QPSK, CPM, and QAM, respectively. The bandwidth efficiency at 18 dB SNR is 7 bps/Hz, 8 bps/Hz, and 6.82 bps/Hz, for QPSK, CPM, and QAM, respectively. In both Figure 13 and Figure 14, APSK and QAM exhibit similar performances since both carry information in both the amplitude and phase given the same modulation level.
Figure 15 compares the complementary cumulative distribution function (CCDF) of PAPR for OFDM when subjected to selected modulation techniques. It shows a general degradation in performance as the number of subcarriers increases. Due to the absence of phase discontinuities, CPM exhibits the best performance compared to QPSK, APSK, and QAM. For instance, when K = 128, at the CCDF of 10–3, the corresponding PAPR is 7.43, 7.512, and 7.562 for CPM, QPSK, APSK, and QAM, respectively. Therefore, CPM does not require a highly linear HPA since it ensures that a low PAPR is maintained. It also shows that the APSK and QAM exhibit a similar performance, of which APSK performance is greatly attributed to the ring ratio. In this case, we used a ring ratio of 2. Depending on the application, system designers vary several parameters such as the spacing between the rings, the number of rings, and the number of symbols on a ring, to achieve the desired performance. It is also worth noting that current state-of-the-art DVB-SAT deploy coded-APSK, which is optimized specifically for SAT applications.
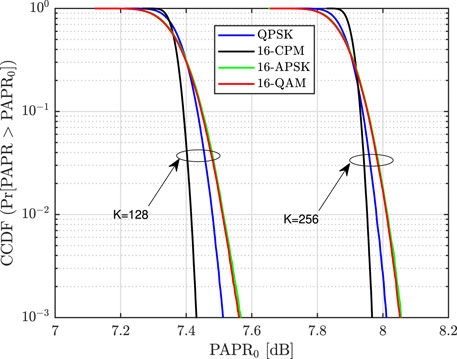
FIGURE 15. CCDF of PAPR for OFDM with the n umber of subcarriers,
Several PAPR reduction techniques have been exploited in literature, such as those with distortion constituting clipping and filtering and amplitude clipping (Yiyan Wu and Wu, 2008; Sandoval et al., 2017). However, the reduction in PAPR is achieved at a cost. For example, it can lead to increased BER, higher computational complexity, and loss in data rate. A low PAPR results in improved spectral efficiency since it allows the use of a low back-off during signal amplification.
Due to the power limitation of HPAs described in Subsection 3.2, modulation schemes, such as QAM, which convey information via multi-level amplitudes require a linear amplifying feature, i.e., amplification requires a wider linear range of operation which leads to reduced power efficiency. This makes them unsuitable for use on channels operated beyond maximum transmitted power efficiency condition. Therefore constant envelope modulation schemes are preferable since they attain bandwidth efficiency through packing all the data in a single-level amplitude while maintaining considerable power efficiency.
5.3 Cost and Complexity
Cost-effective and easy-to-implement aspects are also essential criteria for designing modulation schemes. Several aspects, such as the modulation level, detection techniques, and the choice of application, contribute greatly to the complexity and resulting cost of a particular modulation technique. For example, based on our findings and evaluations, APSK and CPM perform better than QAM but designing their receivers is quite complex and possesses synchronization difficulties.
6 Future and Open Topics
In addition to modulation, other complementary avenues can be exploited to achieve higher joint system performance in terms of throughput, reliability, latency, and efficiency through applying other techniques, such as waveform design, error-control coding, variable/adaptive coding and modulation (VCM/ACM), spatial diversity schemes, enhanced synchronization, and beam management altogether.
6.1 Error Control Coding
In communications systems, engineers deploy error correction and detection (Costello et al., 1998; Clark et al., 2013) schemes to improve the reliability. Such techniques may include forward error control (FEC), automatic repeat request (ARQ), and hybrid automatic repeat request (HARQ). Examples of common error codes used in FEC may include Reed-Solomon (RS), convolutional (CC), Bose-Chaudhuri-Hocquenghem (BCH) codes, low-density parity check (LDPC), polar, and turbo codes (Hagenauer and Lutz, 1987; Costello et al., 1998; Del Re and Pierucci, 2002b; CCSDS, 2011; Clark et al., 2013). Rather than single codes, a concatenated system of codes could be deployed to further boost performance (Forney, 1966). Concatenation brings about a double coding gain by regulating random and burst errors introduced by the channel. By employing error-correction, system BER performance could be improved; however, several trade-off scenarios exist between power, bandwidth, latency, reliability, complexity altogether. For instance, less power could be required to transmit signals but at the expense of decreased bandwidth. Additional processing, such as long interleaving and coding overhead introduced by error correction could lead to an increase in latency, especially for long code lengths (Shirvanimoghaddam et al., 2019). Moreover, whether to do HARQ at either the TN or NTN level or both has to be considered since HARQ is crucial at long round trip transmissions (RTT). This could be done by enhancing the existing HARQ operation, limiting HARQ capabilities, and disabling HARQ for long RTT delays (Kodheli et al., 2017; 3GPP TR 38.811 V15.4.0, 2020; RP-180664, 2018). Furthermore, decoding algorithms could make the system more complex and costly. Developing efficient and less complex FEC and HARQ techniques is an area that requires more research.
6.2 VCM/ACM
ACM can be regarded as a form of resource allocation by which the most appropriate modulation and coding (ModCod) scheme is selected depending on the channel condition. For instance, under favorable channel conditions, a high order modulation technique with low coding redundancy is deployed in order to increase the transmission data rate. Conversely, during a signal fade, the system selects a more robust modulation scheme and a higher coding rate to sustain link availability, and connection quality without increasing the signal power. Variations in the channel could result from weather conditions, propagation distance, mobility, signal obstruction by multiple reflectors, and so forth. ACM is widely used in wireless communications, such as cellular (3GPP TR 25.848 V4.0.0, 2001) and SATs (ETSI, 2006). Although several researchers have investigated and proposed different ACM approaches (Goldsmith and Chua, 1998; Xiaoxin Qiu and Chawla, 1999; Downey et al., 2016; Mota et al., 2019), more research will be necessary to design optimal ACM techniques for unified application in personal SATCOMs to guarantee improved throughput and error rate performance. Besides the channel condition, other factors may be considered when designing ACM techniques, such as the mode of transmission (single or multi-carrier), operation band, HPA nonlinearities, spatial diversity, delay requirements, system complexity, and others. In addition to ACM, VCM (Toptsidis et al., 2012) is another technique for link adaptation that takes advantage of the variability in the SAT slant path geometry resulting from the relative movement between the SAT and the ground terminal. This means that the ModCods are selected based on the angle of elevation of the link.
6.3 Spatial Diversity
The application of multi-antenna systems in SATs (Arapoglou et al., 2011; Kyröläinen et al., 2014; Petropoulou et al., 2014) has been triggered by the shift from lower frequency bands to higher frequency bands such as Ku and Ka, which allow the use of smaller antennas (Kodheli et al., 2021). Additionally, adopting a shorter wavelength enables the design of compact phased array antennas that achieve isotropic gain, and hence offsetting the propagation loss at higher frequencies (Varrall, 2018). Boosting of its benefits, including reliability, throughput, and spectral efficiency, resulting from spatial diversity, interference reduction, spatial multiplexing, and beamforming gain, multiple-input multiple-output (MIMO) technology has been widely used in TNs over the past years (Paulraj et al., 2004; Boccardi et al., 2012). However, the applicability of MIMO in SATCOMs, especially spatial multiplexing, is not yet fully exploited. The main hindrance being, the absence of scatterers in the domain in which SATs operate. The scattering environment makes the fading paths between multi-antenna transmitter and receiver independent, hence, allowing to leverage the benefits of MIMO. Moreover, the MIMO channel matrix becomes rank deficient, leading to performance deterioration (Arapoglou et al., 2011). From the wireless network perspective, spatial diversity (diggavi et al., 2004; Sendonaris et al., 2003) can be exploited to enhance performance improvement in SATCOMs in terms of higher reliability and data rates. Therefore, it is of great necessity to conduct further research concerning the application of multi-antenna systems in a personal SATCOM scenario at the SAT, user terminal, and earth station level. For example, the antenna size, the number of antennas required, interference mitigation, the use of codes, resource allocation, optimal beamforming architectural design, to mention but a few (Yingda et al., 2006; Alegre-Godoy and Vazquez-Castro, 2013; Mysore et al., 2021).
6.4 Enhanced Synchronization
Following the paradigm shift in network generations, there is an expected substantial increase in speed requirements; hence synchronization will be a key streamliner to the seamless delivery of extended services (Lin, 2018). In a radio network, radio clocks that are not well synchronized are prone to time shifts and are less accurate, resulting in interference between cells. From the network perspective, exacerbated problems of Doppler effects could be faced in out-of-sync integrated SATs and TNs. Moreover, OBP functionality is vulnerable to synchronization failures. Hence further research related to synchronization requirements for personal SATCOMs is required. Unlike legacy network generations, such as 3G and 4G whose synchronization was mainly through frequency, next network generations, including 5G and beyond will require the enhancement of phase and time synchronization as well (Li et al., 2017; Mahmood et al., 2019; Ericsson, 2021). Further studies (RP-180664, 2018; R1-1802064, 2018; R1-1904245, 2019) need to be conducted to harmonize transmission and reception procedures taking place in both TN and SATCOM. Synchronization techniques should allow the use of minimum overhead while maintaining bandwidth efficiency.
6.5 Beam Management
The size of footprint of a particular SAT is based on the beam illuminated in the direction of the receiver. Additionally, the deployment of LEOs and the adoption of higher frequency bands have enabled the use of smaller antennas, which support the transition from a single fixed but wide beam to multiple flexible but smaller beams. Consequently, the use of several spot beams allows frequency re-use, which could be catastrophic in terms of ACI if not regulated. Therefore, beam management in personal SATCOMs will be essential for several purposes not limited to interference cancellation. Due to the unpredictable nature of traffic patterns in personal communications, the SAT payloads should be designed with the flexibility to varying traffic, power, cost, and delivery time. The authors in (Jacomb-Hood and Lier, 2000) present the benefits of beam-shape flexibility and design multibeam active phase arrays for SATCOMs. In (R1-1802551, 2018), beam modeling for handheld devices in NTN is presented based on antenna array assumption at lower and higher frequency bands. The beamforming gain is deemed necessary for link budget analysis and estimation of achievable data rate. Therefore, optimal beamforming architectures are desirable for seamless service delivery. Other promising technologies for beam management may include multi-spot beamforming (Del Re and Pierucci, 2002b; Letzepis and Grant, 2008; Su et al., 2019), hybrid beamforming (Molisch et al., 2017), beam hopping (Freedman et al., 2015; Freedman et al., 2017; Nader et al., 2020), precoding (Vazquez et al., 2016), altogether.
6.6 Waveform Design
Besides the detrimental effects of HPA nonlinearities, other channel impairments, such as Doppler shift, and multipath effects are significant in determining the performance of personal SATCOMs. These greatly contribute to ISI and ACI during transmission. Hence waveform design is very important. Whereas transmission in present-day TN is dominated by multi-carrier transmissions (ITU-R S.2173-1, 2014), more research is still required to reinforce the implementation of multi-carrier transmissions in SATCOMs. In the light of such differences in transmission, a joint or similar mode of transmission could be of great necessity to ensure efficiency in the performance of integrated SAT and TN air-interfaces. For instance, OFDM can be adopted for transmission in personal SATCOMs due to simplicity of the receiver design in multipath channels. However, OFDM has a higher PAPR compared to single-carrier waveforms. Moreover, in SAT channels, due to absence of scatterers, the effect of multipath channels is negligible, which makes single-carrier waveforms desirable. Therefore single-carrier waveforms such as, SC-FDMA, can be adopted in the uplink (Papathanassiou et al., 2001; Dalakas et al., 2012). Alternatively, advanced OFDM-like single-carrier and multi-carrier waveforms can be deployed (Tan and Stuber, 2002; Thompson et al., 2008; Mulinde et al., 2013; Farhang-Boroujeny and Moradi, 2016; Guidotti et al., 2016; Rahman et al., 2018). For that matter, the limitations of multi-carrier transmissions discussed in 4.5 should not be neglected.
7 Conclusion
In this paper, the literature review on the performance of the representative digital modulation techniques was presented for implementation in SATs to support personal communications. Power efficiency, spectral efficiency, pros and cons of different schemes alongside several SAT system requirements have been discussed. We have carried out performance comparisons backed up by simulations and to support our findings. Among the representative modulation techniques, CPM has the best performance subject to the different evaluation metrics. Simply put, CPM presents the best compromise between power efficiency, bandwidth efficiency, and error performance. Moreover, by replacing APSK with CPM, system performance can be improved by 20 ∼ 40% in terms of power and bandwidth efficiency.
By combining our studies and results, we have shown that several trade-offs exist when determining the choice of a modulation technique to use. For instance, to attain high data rates and spectral efficiency, higher-order modulation schemes could be adopted. However, such modulation schemes are prone to nonlinear signal distortions and have a high BER performance. Nonconstant envelope modulation schemes are bandwidth-efficient, nevertheless, power inefficient. On the other hand, constant envelope modulation schemes are not only power-efficient but also spectrally efficient. For the case of nonconstant envelope modulation schemes, linear amplification is required. Additionally, advanced predistortion techniques may be deployed in the uplink station to minimize the nonlinearity effect. High efficiency saturated HPAs can be deployed to improve performance in constant envelope modulation schemes. By deploying a modulation scheme that is of less power efficiency but simple to modulate in the uplink, and a more power-efficient scheme in the downlink would result in power saving at SAT transceiver sides but at the expense of performance loss in the uplink. This may be compensated for through increasing power or the antenna gain of the ground station. We have also shown that all the representative modulation schemes are affected by Doppler; hence, advanced Doppler mitigation techniques are required. To counteract the problem of HPA nonlinearity and Doppler effects, it would be beneficial to deploy modulation techniques that are cost-effective, easy-to-implement, and adaptive to channel conditions with associated HPA limitations in order to achieve quality of service in personal SATCOMs. To this end, we therefore hope this work will be a valuable guide to both academia and industry for the development of the next generation networks but not limited to personal SATCOMs.
Data Availability Statement
The original contributions presented in the study are included in the article/Supplementary Material, further inquiries can be directed to the corresponding author.
Author Contributions
JS has worked on writing the original draft and surveyed the various modulation schemes used for the satellite communications. BL and J-HL has worked on reviewing and editing the draft. Y-CK set up the conceptualization and did the supervision.
Conflict of Interest
The authors declare that the research was conducted in the absence of any commercial or financial relationships that could be construed as a potential conflict of interest.
Publisher’s Note
All claims expressed in this article are solely those of the authors and do not necessarily represent those of their affiliated organizations, or those of the publisher, the editors and the reviewers. Any product that may be evaluated in this article, or claim that may be made by its manufacturer, is not guaranteed or endorsed by the publisher.
Acknowledgments
This work was supported by Institute of Information & communications Technology Planning & Evaluation (IITP) grant funded by the Korea government (MSIT) (No. 2021-0-00260, Research on LEO Inter-Satellite Links.
Footnotes
1[Online]. Available at: https://en.wikipedia.org/wiki/Kuiper_Systems.
2[Online]. Available at: https://oneweb.net.
3[Online]. Available at: https://www.3gpp.org/about-3gpp.
4[Online]. Available at: https://www.eutelsat.org.
5[Online]. Available at: https://www.globalstar.com.
6[Online]. Available at: https://www.inmarsat.org.
7[Online]. Available at: https://www.intelsat.com.
8[Online]. Available at: https://www.iridium.com.
9[Online]. Available at: https://www.starlink.com.
10[Online]. Available at: https://www.telesat.com.
11[Online]. Available at: https://www.thuraya.com.
References
3GPP TR 25.848 V4.0.0, 2001 3Gpp Tr 25.848 V4.0.0, (2001). Technical Specification Group Radio Access Network; Physical Layer Aspects of UTRA High Speed Downlink Packet Access, (Release 4), Tech. Rep.
3GPP TR 38.811 V15.4.0, 2020 3Gpp Tr 38.811 V15.4.0 (2020). 3rd Generation Partnership Project; Technical Specification Group Radio Access Network; Study on New Radio (NR) to Support Non-terrestrial Networks (Release 15). Tech. Rep.
Abdi, A., Lau, W. C., Alouini, M., and Kaveh, M. (2003). A New Simple Model for Land mobile Satellite Channels: First- and Second-Order Statistics. IEEE Trans. Wireless Commun. 2 (3), 519–528. doi:10.1109/twc.2003.811182
Agilent Technologies (2000). Digital Modulation in Communications Systems: An Introduction Application Note. [Online]. Available at: https://www.keysight.com/kr/ko/assets/7018-09093/application-notes/5965-7160.pdf.
Akhtman, J., and Hanzo, L. (2009). “Power versus Bandwidth-Efficiency in Wireless Communications: The Economic Perspective,” in 2009 IEEE 70th Veh. Technol. Conf., 1–5. doi:10.1109/vetecf.2009.5379027
Alegre-Godoy, R., and Vazquez-Castro, M. A. (2013). Spatial Diversity with Network Coding for ON/OFF Satellite Channels. IEEE Commun. Lett. 17 (8), 1612–1615. doi:10.1109/lcomm.2013.062113.130963
Ali, I., Al-Dhahir, N., and Hershey, J. E. (1998). Doppler Characterization for LEO Satellites. IEEE Trans. Commun. 46 (3), 309–313. doi:10.1109/26.662636
Anderson, J. B., Aulin, T., and Sundberg, C. E. (2013). Digital Phase Modulation. Berlin, Germany: Springer Science & Business Media.
Arapoglou, P.-D., Liolis, K., Bertinelli, M., Panagopoulos, A., Cottis, P., and De Gaudenzi, R. (2011). MIMO over Satellite: A Review. IEEE Commun. Surv. Tutorials 13 (1), 27–51. doi:10.1109/surv.2011.033110.00072
Aulin, T., Rydbeck, N., and Sundberg, C.-E. (1981). Continuous Phase Modulation-Part II: Partial Response Signaling. IEEE Trans. Commun. 29 (3), 210–225. doi:10.1109/tcom.1981.1094985
Aulin, T., and Sundberg, C.-E. (1984). CPM-an Efficient Constant Amplitude Modulation Scheme. Int. J. Satell. Commun. 2 (3), 161–186. doi:10.1002/sat.4600020304
AulinSundberg, T. C. E., and Sundberg, C. (1981). Continuous Phase Modulation-Part I: Full Response Signaling. IEEE Trans. Commun. 29 (3), 196–209. doi:10.1109/tcom.1981.1095001
Beidas, B. F. (2011). Intermodulation Distortion in Multicarrier Satellite Systems: Analysis and Turbo Volterra Equalization. IEEE Trans. Commun. 59 (6), 1580–1590. doi:10.1109/tcomm.2011.042111.100320
Beidas, B. F., Seshadri, R. I., and Becker, N. (2015). Multicarrier Successive Predistortion for Nonlinear Satellite Systems. IEEE Trans. Commun. 63 (4), 1373–1382. doi:10.1109/tcomm.2015.2401556
Belce, O. (2003). Comparison of Advanced Modulation Schemes for LEO Satellite Downlink Communications. Proc. Int. Conf. Recent Adv. Space Tech., 432–437. doi:10.1109/rast.2003.1303955
Bingham, J. A. C. (1990). Multicarrier Modulation for Data Transmission: an Idea Whose Time Has Come. IEEE Commun. Mag. 28 (5), 5–14. doi:10.1109/35.54342
Boccardi, F., Clerckx, B., Ghosh, A., Hardouin, E., Jöngren, G., Kusume, K., et al. (2012). Multiple-antenna Techniques in LTE-Advanced. IEEE Commun. Mag. 50 (3), 114–121. doi:10.1109/mcom.2012.6163590
Boero, L., Bruschi, R., Davoli, F., Marchese, M., and Patrone, F. (2018). Satellite Networking Integration in the 5G Ecosystem: Research Trends and Open Challenges. IEEE Netw. 32 (5), 9–15. doi:10.1109/mnet.2018.1800052
Cardarilli, G., Del Re, R., Giancristofaro, D., Re, M., and Simone, L. (2002). “Digital Modulator Architectures for Satellite and Space Applications,” in IEEE Int. Conf. Circuits Syst. Commun., 166–169.
Castanet, L., Bolea-Alamañac, A., and Bousquet, M. (2003). Interference and Fade Mitigation Techniques for Ka and Q/V Band Satellite Communication Systems. Proc. 2nd Int. Workshop COST Action. 280.
Ccsds (2011). Recommendation for Space Data System Standards: TM Synchronization and Channel Coding, CCSDS 131.0-B-2 Blue Book. [Online]. Available at: https://public.ccsds.org/Pubs/131x0b2ec1s.pdf.
Chang, R. W. (1966). Synthesis of Band-Limited Orthogonal Signals for Multichannel Data Transmission. Bell Syst. Tech. J. 45 (10), 1775–1796. doi:10.1002/j.1538-7305.1966.tb02435.x
Cho, Y. S., Kim, J., Yang, W. Y., and Kang, C. G. (2010). MIMO-OFDM Wireless Communications with MATLAB. Hoboken, NJ, USA: John Wiley & Sons.
Chun Loo, C. (1985). A Statistical Model for a Land mobile Satellite Link. IEEE Trans. Veh. Technol. 34 (3), 122–127. doi:10.1109/t-vt.1985.24048
Clark, J., George, C., and Cain, J. B. (2013). Error-correction Coding for Digital Communications. Berlin, Germany: Springer Science & Business Media.
Cook, C., and Marsh, H. (1983). An Introduction to Spread Spectrum. IEEE Commun. Mag. 21 (2), 8–16. doi:10.1109/mcom.1983.1091346
Corazza, G. E. (2007). Digital Satellite Communications. Berlin, Germany: Springer Science & Business Media.
Corazza, G. E., and Vatalaro, F. (1994). A Statistical Model for Land mobile Satellite Channels and its Application to Nongeostationary Orbit Systems. IEEE Trans. Veh. Technol. 43 (3), 738–742. doi:10.1109/25.312773
Costello, D. J., Hagenauer, J., Imai, H., and Wicker, S. B. (1998). Applications of Error-Control Coding. IEEE Trans. Inform. Theor. 44 (6), 2531–2560. doi:10.1109/18.720548
Dahlman, E., Parkvall, S., and Skold, J. (2013). 4G: LTE/LTE-advanced for mobile Broadband. Cambridge, MA, USA: Academic Press.
Dalakas, V., Mathiopoulos, P. T., Di Cecca, F., and Gallinaro, G. (2012). A Comparative Study between Sc-Fdma and Ofdma Schemes for Satellite Uplinks. IEEE Trans. Broadcast. 58 (3), 370–378. doi:10.1109/tbc.2012.2193494
De Gaudenzi, R., Guillen i Fabregas, A., and Martinez, A. (2006). Performance Analysis of Turbo-Coded APSK Modulations over Nonlinear Satellite Channels. IEEE Trans. Wireless Commun. 5 (9), 2396–2407. doi:10.1109/twc.2006.1687763
Del Portillo, I., Cameron, B. G., and Crawley, E. F. (2019). A Technical Comparison of Three Low Earth Orbit Satellite Constellation Systems to Provide Global Broadband. Acta Astronautica 159, 123–135. doi:10.1016/j.actaastro.2019.03.040
Del Re, E., and Pierucci, L. (2002). Next-generation mobile Satellite Networks. IEEE Commun. Mag. 40 (9), 150–159. doi:10.1109/mcom.2002.1031842
Del Re, E., and Pierucci, L. (2002). Satellite Personal Communications for Future-Generation Systems: Final Report: COST 252 Action. Berlin, Germany: Springer Science & Business Media.
diggavi, S. N., al-dhahir, N., stamoulis, A., and calderbank, A. R. (2004). Great Expectations: the Value of Spatial Diversity in Wireless Networks. Proc. IEEE 92 (2), 219–270. doi:10.1109/jproc.2003.821914
Dimatteo, S., Hui, P., Han, B., and Li, V. O. (2011). “Cellular Traffic Offloading through WiFi Networks,” in 2011 IEEE Eighth Int. Conf. Mobile Ad-Hoc Sensor Syst., 192–201. doi:10.1109/mass.2011.26
Ding, L., Zhou, G. T., Morgan, D. R., Ma, Z., Kenney, J. S., Kim, J., et al. (2004). A Robust Digital Baseband Predistorter Constructed Using Memory Polynomials. IEEE Trans. Commun. 52 (1), 159–165. doi:10.1109/tcomm.2003.822188
Downey, J. A., Mortensen, D. J., Evans, M. A., Briones, J. C., Tollis, N., et al. (2016). “Adaptive Coding and Modulation experiment with NASA’s Space Communication and Navigation Testbed,” in 34th AIAA Int. Commun. Satellite Syst. Conf., 11.
Emea Satellite Operators Association (2021). Spectrum. [Online]. Available at: https://esoa.net/topics/spectrum/.
Ericsson (2021). 5G Synchronization Requirements and Solutions. [Online]. Available at: https://www.ericsson.com/48e592/assets/local/reports-papers/ericsson-technology-review/docs/2021/5g-synchronization-requirements-and-solutions.pdf.
Etsi (2012). 301 545-2 (2012). Digital Video Broadcasting (DVB); Second Generation DVB Interactive Satellite System (DVB-RCS2); Part 2: Lower Layers for Satellite Standard, V1. 1.1. Sophia Antipolis, France: European Telecommunications Standards Institute, ETSI.
Etsi (2006). “302 307 V1. 1.2 (2006-06): Digital Video Broadcasting (DVB),” in Second Generation Framing Structure, Channel Coding, and Modulation Systems for Broadcasting, Interactive Services, News Gathering, and Other Broadband Satellite Applications.
Evans, J. V. (1997). Satellite Systems for Personal Communications. IEEE Antennas Propag. Mag. 39 (3), 7–20. doi:10.1109/74.598556
Farhang-Boroujeny, B., and Moradi, H. (2016). OFDM Inspired Waveforms for 5G. IEEE Commun. Surv. Tutorials 18 (4), 2474–2492. doi:10.1109/comst.2016.2565566
Flohberger, M., Gappmair, W., and Koudelka, O. (2010). “Modulation Classifier for Signals Used in Satellite Communications,” in Advanced Satellite Multimedia Syst. Conf. and the 11th Signal Process. Space Commun. Workshop, 198–202. doi:10.1109/asms-spsc.2010.5586892
Freedman, A., Rainish, D., and Elinav, D. (2017). “Beam Hopping System Design Considerations,” in 23rd Ka and Broadband Commun. Conf.
Freedman, A., Rainish, D., and Gat, Y. (2015). Beam Hopping How to Make it Possible. Proc. Ka Broadband Commun. Conf.
F. R. Group (2021). A Review of Satellite Communications and Complementary Approaches to Support Distributed Disaster Response. Available at: https://www.dhs.gov/sites/default/files/publications/A%20Review%20of%20%Satellite%20Communications%201-15-14.pdf (Accessed 014, , 2021).
Gard, K. G., Gutierrez, H. M., and Steer, M. B. (1999). Characterization of Spectral Regrowth in Microwave Amplifiers Based on the Nonlinear Transformation of a Complex Gaussian Process. IEEE Trans. Microwave Theor. Techn. 47 (7), 1059–1069. doi:10.1109/22.775437
Ghorbani, A., and Sheikhan, M. (1991). “The Effect of Solid State Power Amplifiers (SSPAs) Nonlinearities on MPSK and M-QAM Signal Transmission,” in Sixth Int. Conf. on Digit. Process. Signals Commun. IET, 193–197.
Goldsmith, A. J., and Chua, S.-G. (1998). Adaptive Coded Modulation for Fading Channels. IEEE Trans. Commun. 46 (5), 595–602. doi:10.1109/26.668727
Gopal, R., and BenAmmar, N. (2018). Framework for Unifying 5G and Next Generation Satellite Communications. IEEE Netw. 32 (5), 16–24. doi:10.1109/mnet.2018.1800045
Gronemeyer, S., and McBride, A. (1976). MSK and Offset QPSK Modulation. IEEE Trans. Commun. 24 (8), 809–820. doi:10.1109/tcom.1976.1093392
Gruber, M., Blume, O., Ferling, D., Zeller, D., Imran, M. A., Strinati, E. C., et al. (2009). “EARTH — Energy Aware Radio and Network Technologies,” in 2009 IEEE 20th Int. Symp. Personal, Indoor and Mobile Radio Commun, 1–5.
Guidotti, A., Colavolpe, G., Vanelli-Coralli, A., and Corazza, G. E. (2016). On the Integration of Terrestrial and Satellite Systems in Future 5G Networks: a Waveform Perspective, Joint Expert Group and Vision Group Workshop 2016. [Online]. Available at: https://networld2020.eu/wp-content/uploads/2016/03/S03P03_SatCom.pdf.
Hagenauer, J., and Lutz, E. (1987). Forward Error Correction Coding for Fading Compensation in Mobile Satellite Channels. IEEE J. Select. Areas Commun. 5 (2), 215–225. doi:10.1109/jsac.1987.1146523
Hindin, J. (2019). Technical Appendix: Application of Kuiper Systems LLC for Authority to Launch and Operate a Non-geostationary Satellite Orbit System in Ka-Band Frequencies. Washington DC: Federal Commun. Commission.
Ho, K. P. (2005). Phase-modulated Optical Communication Systems. Berlin, Germany: Springer Science & Business Media.
Horlin, F., and Bourdoux, A. (2008). Digital Compensation for Analog Front-Ends: A New Approach to Wireless Transceiver Design. Hoboken, NJ, USA: John Wiley & Sons.
Insights, F. B. (2021). Semiconductor Market. [Online]. Available at: https://www.fortunebusinessinsights.com/semiconductor-market-102365.
International Telecommunications Union (2002). ITU Handbook on Satellite Communications. 3rd Edition. Hoboken, NJ, USA: John Wiley & Sons.
Itu-R S.2173-1 (2014). Multi-carrier Based Transmission Techniques for Satellite Systems. Tech. Rep.
Jacomb-Hood, A., and Lier, E. (2000). Multibeam Active Phased Arrays for Communications Satellites. IEEE Microwave 1 (4), 40–47. doi:10.1109/6668.893245
Jakes, W. C., and Cox, D. C. (1994). Microwave mobile Communications. Hoboken, NJ, USA: Wiley-IEEE press.
Joung, J., Ho, C. K., Adachi, K., and Sun, S. (2015). A Survey on Power-Amplifier-Centric Techniques for Spectrum- and Energy-Efficient Wireless Communications. IEEE Commun. Surv. Tutorials 17 (1), 315–333. doi:10.1109/comst.2014.2350018
Kanatas, A. G., and Panagopoulos, A. D. (2012). Radio Wave Propagation and Channel Modeling for Earth-Space Systems. Boca Raton, FL, USA: CRC Press.
Kaushal, H., and Kaddoum, G. (2017). Optical Communication in Space: Challenges and Mitigation Techniques. IEEE Commun. Surv. Tutorials 19 (1), 57–96. doi:10.1109/comst.2016.2603518
Kayhan, F., and Montorsi, G. (2012). “Constellation Design for Transmission over Nonlinear Satellite Channels,” in IEEE Global Commun. Conf. (GLOBECOM), 3401–3406. doi:10.1109/glocom.2012.6503640
Keysight Technologies (2020). Modulation Techniques for Satellite Communications. [Online]. Available at: https://www.keysight.com/kr/ko/assets/7120-1006/white-papers/Modulation-Techniques-for-Satellite-Communications.pdf.
Kodheli, O., Guidotti, A., and Vanelli-Coralli, A. (2017). Integration of Satellites in 5G through LEO Constellations. IEEE Glob. Commun. Conf. 2017, 1–6. doi:10.1109/glocom.2017.8255103
Kodheli, O., Lagunas, E., Maturo, N., Sharma, S. K., Shankar, B., Montoya, J. F. M., et al. (2021). Satellite Communications in the New Space Era: A Survey and Future Challenges. IEEE Commun. Surv. Tutorials 23 (1), 70–109. doi:10.1109/comst.2020.3028247
Kyröläinen, J., et al. (2014). Applicability of MIMO to Satellite Communications. Int. J. Satellite Commun. Netw. 32 (4), 343–357.
Lee, K., Lee, J., Yi, Y., Rhee, I., and Chong, S. (2013). Mobile Data Offloading: How Much Can WiFi Deliver? Ieee/acm Trans. Networking 21 (2), 536–550. doi:10.1109/tnet.2012.2218122
Lee, W. C. Y. (1998). Mobile Communications Engineering: Theory and Applications. New York, NY, USA: McGraw-Hill Education.
Letzepis, N., and Grant, A. J. (2008). Capacity of the Multiple Spot Beam Satellite Channel with Rician Fading. IEEE Trans. Inform. Theor. 54 (11), 5210–5222. doi:10.1109/tit.2008.929961
Li, H., Han, L., Duan, R., and Garner, G. M. (2017). Analysis of the Synchronization Requirements of 5G and Corresponding Solutions. IEEE Comm. Stand. Mag. 1 (1), 52–58. doi:10.1109/mcomstd.2017.1600768st
Lin, J.-C. (2018). Synchronization Requirements for 5G: An Overview of Standards and Specifications for Cellular Networks. IEEE Veh. Technol. Mag. 13 (3), 91–99. doi:10.1109/mvt.2018.2813339
Lin, J., Hou, Z., Zhou, Y., Tian, L., and Shi, J. (2016). “Map Estimation Based on Doppler Characterization in Broadband and Mobile LEO Satellite Communications,” in 2016 IEEE 83rd Veh. Technol. Conf. (VTC Spring), 1–5. doi:10.1109/vtcspring.2016.7504336
Liu, Z., Xie, Q., Peng, K., and Yang, Z. (2011). APSK Constellation with Gray Mapping. IEEE Commun. Lett. 15 (12), 1271–1273. doi:10.1109/lcomm.2011.101211.111825
Lohmeyer, W. Q., Aniceto, R. J., and Cahoy, K. L. (2016). Communication Satellite Power Amplifiers: Current and Future SSPA and TWTA Technologies. Int. J. Satell. Commun. Netw. 34 (2), 95–113. doi:10.1002/sat.1098
Mahmood, A., Ashraf, M. I., Gidlund, M., Torsner, J., and Sachs, J. (2019). Time Synchronization in 5G Wireless Edge: Requirements and Solutions for Critical-MTC. IEEE Commun. Mag. 57 (12), 45–51. doi:10.1109/mcom.001.1900379
Michailow, N., Gaspar, I., Krone, S., Lentmaier, M., and Fettweis, G. (2012). “Generalized Frequency Division Multiplexing: Analysis of an Alternative Multi-Carrier Technique for Next Generation Cellular Systems,” in Int. Symp. on Wireless Commun. Syst. (ISWCS), Paris, France, 171–175. doi:10.1109/iswcs.2012.6328352
Minoli, D. (2015). Innovations in Satellite Communications and Satellite Technology: The Industry Implications of DVB-S2x, High Throughput Satellites, Ultra HD, M2M, and IP. Hoboken, NJ, USA: John Wiley & Sons.
Molisch, A. F., Ratnam, V. V., Han, S., Li, Z., Nguyen, S. L. H., Li, L., et al. (2017). Hybrid Beamforming for Massive MIMO: A Survey. IEEE Commun. Mag. 55 (9), 134–141. doi:10.1109/mcom.2017.1600400
Morello, A., and Mignone, V. (2006). DVB-S2: The Second Generation Standard for Satellite Broad-Band Services. Proc. IEEE 94 (1), 210–227. doi:10.1109/jproc.2005.861013
Mota, M. P., Araujo, D. C., Costa Neto, F. H., de Almeida, A. L. F., and Cavalcanti, F. R. (2019). “Adaptive Modulation and Coding Based on Reinforcement Learning for 5G Networks,” in IEEE Globecom Workshops (GC Wkshps), 1–6. doi:10.1109/gcwkshps45667.2019.9024384
Mulinde, R., Rahman, T. F., and Sacchi, C. (2013). “Constant-envelope SC-FDMA for Nonlinear Satellite Channels,” in 2013 IEEE Global Commun. Conf. (GLOBECOM), 2939–2944. doi:10.1109/glocom.2013.6831521
Murota, K., and Hirade, K. (1981). GMSK Modulation for Digital Mobile Radio Telephony. IEEE Trans. Commun. 29 (7), 1044–1050. doi:10.1109/tcom.1981.1095089
Mysore, B. S., Lagunas, E., Chatzinotas, S., and Ottersten, B. (2021). Precoding for Satellite Communications: Why, How and what Next? IEEE Commun. Lett. 25 (8), 2453–2457. doi:10.1109/lcomm.2021.3058359
Nader, A., Freedman, A., and Peter, N. (2020). Beam Hopping in DVB–S2x. [Online]. Available at: https://dvb.org/wp-content/uploads/2020/02/20200330_webinar_beam_hopping_FINAL.pdf.
Oetting, J. (1979). A Comparison of Modulation Techniques for Digital Radio. IEEE Trans. Commun. 27 (12), 1752–1762. doi:10.1109/tcom.1979.1094370
Panagopoulos, A. D., Arapoglou, P.-D. M., and Cottis, P. G. (2004). Satellite Communications at Ku, Ka, and V Bands: Propagation Impairments and Mitigation Techniques. IEEE Commun. Surv. Tutorials 6 (3), 2–14. doi:10.1109/comst.2004.5342290
Papathanassiou, A., Salkintzis, A. K., and Mathiopoulos, P. T. (2001). A Comparison Study of the Uplink Performance of W-CDMA and OFDM for mobile Multimedia Communications via LEO Satellites. IEEE Pers. Commun. 8 (3), 35–43. doi:10.1109/98.930095
Pasupathy, S. (1979). Minimum Shift Keying: A Spectrally Efficient Modulation. IEEE Commun. Mag. 17 (4), 14–22. doi:10.1109/mcom.1979.1089999
Paulraj, A. J., Gore, D. A., Nabar, R. U., and Bolcskei, H. (2004). An Overview of MIMO Communications-A Key to Gigabit Wireless. Proc. IEEE 92 (2), 198–218. doi:10.1109/jproc.2003.821915
Penttinen, J. (2015). The Telecommunications Handbook: Engineering Guidelines for Fixed, mobile and Satellite Systems. Hoboken, NJ, USA: John Wiley & Sons.
Perez-Neira, A. I., Vazquez, M. A., Shankar, M. R. B., Maleki, S., and Chatzinotas, S. (2019). Signal Processing for High-Throughput Satellites: Challenges in New Interference-Limited Scenarios. IEEE Signal. Process. Mag. 36 (4), 112–131. doi:10.1109/msp.2019.2894391
Petropoulou, P., Michailidis, E. T., Panagopoulos, A. D., and Kanatas, A. G. (2014). Radio Propagation Channel Measurements for Multi-Antenna Satellite Communication Systems: A Survey. IEEE Antennas Propag. Mag. 56 (6), 102–122. doi:10.1109/map.2014.7011023
Pickholtz, R., Schilling, D., and Milstein, L. (1982). Theory of Spread-Spectrum Communications - A Tutorial. IEEE Trans. Commun. 30 (5), 855–884. doi:10.1109/tcom.1982.1095533
R1-1802064 (2018). Considerations on Doppler Compensation for LEO-based NTN,” 3GPP TSG RAN WG1. Tech. Rep.
R1-1904245 (2019). Considerations on Doppler Compensation for Non-GEO-based NTN,” 3GPP TSG RAN WG1. Tech. Rep.
Rahman, T. F., Sacchi, C., Morosi, S., Mazzinghi, A., and Bartolomei, N. (2018). Constant-Envelope Multicarrier Waveforms for Millimeter Wave 5G Applications. IEEE Trans. Veh. Technol. 67 (10), 9406–9420. doi:10.1109/tvt.2018.2854723
Rangan, S., Rappaport, T. S., and Erkip, E. (2014). Millimeter-Wave Cellular Wireless Networks: Potentials and Challenges. Proc. IEEE 102 (3), 366–385. doi:10.1109/jproc.2014.2299397
Rapp, C. (1991). Effects of HPA-Nonlinearity on a 4-Dpsk/ofdm-Signal for a Digital Sound Broadcasting Signal. ESA Spec. Publ. 332, 179–184.
Rimoldi, B. E. (1988). A Decomposition Approach to CPM. IEEE Trans. Inform. Theor. 34 (2), 260–270. doi:10.1109/18.2634
Rohde and Schwarz (2021). 5G Waveform Candidates. Available at: https://scdn.rohde-schwarz.com/ur/pws/dl_downloads/dl_application/application_notes/1ma271/1MA271_0e_5G_waveform_candidates.pdf (Accessed 152.2021.
Rp-180664 (2018). NR-NTN: Preliminary Solutions for NR to Support Non-terrestrial Networks,” 3GPP TSG RAN. Tech. Rep.
Rydbeck, N., Chennakeshu, S., Dent, P., and Hassan, A. (1996). Mobile-satellite Systems: a Perspective on Technology and Trends. Proc. Veh. Technol. Conf. 2, 1013–1017.
Sadek, M., and Aissa, S. (2012). Personal Satellite Communication: Technologies and Challenges. IEEE Wireless Commun. 19 (6), 28–35. doi:10.1109/mwc.2012.6393515
Saleh, A. A. M. (1981). Frequency-independent and Frequency-dependent Nonlinear Models of TWT Amplifiers. IEEE Trans. Commun. 29 (11), 1715–1720. doi:10.1109/tcom.1981.1094911
Saltzberg, B. (1967). Performance of an Efficient Parallel Data Transmission System. IEEE Trans. Comm. Technol. 15 (6), 805–811. doi:10.1109/tcom.1967.1089674
Sandoval, F., Poitau, G., and Gagnon, F. (2017). Hybrid Peak-To-Average Power Ratio Reduction Techniques: Review and Performance Comparison. IEEE Access 5, 27 145. doi:10.1109/access.2017.2775859
Sendonaris, A., Erkip, E., and Aazhang, B. (2003). User Cooperation Diversity-Part I: System Description. IEEE Trans. Commun. 51 (11), 1927–1938. doi:10.1109/tcomm.2003.818096
Sheriff, R. E., and Hu, Y. F. (2001). Mobile Satellite Communication Networks. Hoboken, NJ, USA: John Wiley & Sons.
Shirvanimoghaddam, M., Mohammadi, M. S., Abbas, R., Minja, A., Yue, C., Matuz, B., et al. (2019). Short Block-Length Codes for Ultra-reliable Low Latency Communications. IEEE Commun. Mag. 57 (2), 130–137. doi:10.1109/mcom.2018.1800181
Simon, M. K. (2005). Bandwidth-efficient Digital Modulation with Application to Deep Space Communications. Hoboken, NJ, USA: John Wiley & Sons, 2.
Su, Y., Liu, Y., Zhou, Y., Yuan, J., Cao, H., and Shi, J. (2019). Broadband LEO Satellite Communications: Architectures and Key Technologies. IEEE Wireless Commun. 26 (2), 55–61. doi:10.1109/mwc.2019.1800299
Sundberg, C.-E. (1986). Continuous Phase Modulation. IEEE Commun. Mag. 24 (4), 25–38. doi:10.1109/mcom.1986.1093063
Tan, J., and Stuber, G. (2002). Constant Envelope Multi-Carrier Modulation. Proc. MILCOM 11, 607–611.
T. E. S. Agency (2002). Satellite Frequency Bands. Available at: https://www.esa.int/Applications/Telecommunications_Integrated_Applications/Satellite_frequency_bands (Accessed 014, 2021).
Thomas, C., Weidner, M., and Durrani, S. (1974). Digital Amplitude-phase Keying with M-Ary Alphabets. IEEE Trans. Commun. 22 (2), 168–180. doi:10.1109/tcom.1974.1092165
Thompson, S. C., Ahmed, A. U., Proakis, J. G., Zeidler, J. R., and Geile, M. J. (2008). Constant Envelope OFDM. IEEE Trans. Commun. 56 (8), 1300–1312. doi:10.1109/tcomm.2008.070043
Toptsidis, N., Arapoglou, P.-D., and Bertinelli, M. (2012). Link Adaptation for Ka Band Low Earth Orbit Earth Observation Systems: A Realistic Performance Assessment. Int. J. Satell. Commun. Netw. 30 (3), 131–146. doi:10.1002/sat.1002
Van De Beek, J., and Berggren, F. (2008). Out-of-Band Power Suppression in OFDM. IEEE Commun. Lett. 12 (9), 609–611. doi:10.1109/lcomm.2008.080587
Varrall, G. (2018). 5G and Satellite Spectrum, Standards, and Scale. Norwood, MA, USA: Artech House.
Vazquez, M. A., Perez-Neira, A., Christopoulos, D., Chatzinotas, S., Ottersten, B., Arapoglou, P.-D., et al. (2016). Precoding in Multibeam Satellite Communications: Present and Future Challenges. IEEE Wireless Commun. 23 (6), 88–95. doi:10.1109/mwc.2016.1500047wc
Weinstein, S., and Ebert, P. (1971). Data Transmission by Frequency-Division Multiplexing Using the Discrete Fourier Transform. IEEE Trans. Comm. Technol. 19 (5), 628–634. doi:10.1109/tcom.1971.1090705
Xiaoxin Qiu, Q., and Chawla, K. (1999). On the Performance of Adaptive Modulation in Cellular Systems. IEEE Trans. Commun. 47 (6), 884–895. doi:10.1109/26.771345
Xie, Q., Wang, Z., and Yang, Z. (2012). Simplified Soft Demapper for APSK with Product Constellation Labeling. IEEE Trans. Wireless Commun. 11 (7), 2649–2657. doi:10.1109/twc.2012.051412.112055
Xiong, F. (2006). Digital Modulation Techniques, (Artech House Telecommunications Library). Norwood, MA, USA: Artech House, Inc.
Yingda, C., Kishore, S., and Jing, L. (2006). Wireless Diversity through Network Coding. IEEE Wireless Commun. Netw. Conf. (Wcnc) 3, 1681–1686. doi:10.1109/wcnc.2006.1696541
Yiyan Wu, T., and Wu, Y. (2008). An Overview: Peak-To-Average Power Ratio Reduction Techniques for OFDM Signals. IEEE Trans. Broadcast. 54 (2), 257–268. doi:10.1109/tbc.2008.915770
Keywords: digital modulation techniques, personal satellite communications, high power amplifier nonlinearities, Doppler effects, non-terrestrial networks
Citation: Ssimbwa J, Lim B, Lee J-H and Ko Y-C (2022) A Survey on Robust Modulation Requirements for the Next Generation Personal Satellite Communications. Front. Comms. Net 3:850781. doi: 10.3389/frcmn.2022.850781
Received: 08 January 2022; Accepted: 08 March 2022;
Published: 17 May 2022.
Edited by:
Tetsuya Kawanishi, Waseda University, JapanReviewed by:
Talha Faizur Rahman, Mississippi State University, United StatesDat Pham, National Institute of Information and Communications Technology, Japan
Copyright © 2022 Ssimbwa, Lim, Lee and Ko. This is an open-access article distributed under the terms of the Creative Commons Attribution License (CC BY). The use, distribution or reproduction in other forums is permitted, provided the original author(s) and the copyright owner(s) are credited and that the original publication in this journal is cited, in accordance with accepted academic practice. No use, distribution or reproduction is permitted which does not comply with these terms.
*Correspondence: Young-Chai Ko, a295Y0Brb3JlYS5hYy5rcg==