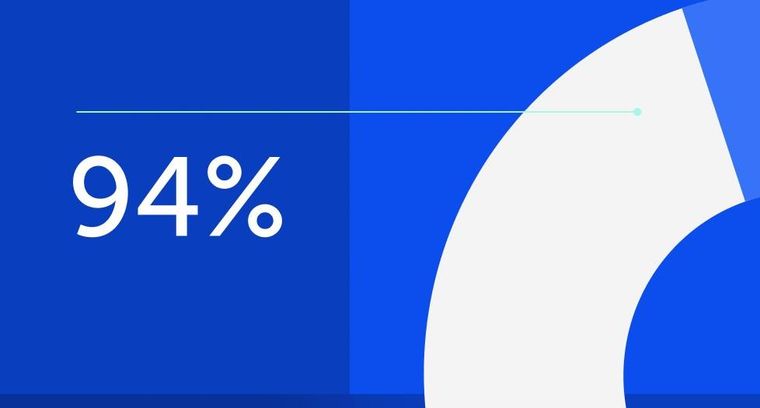
94% of researchers rate our articles as excellent or good
Learn more about the work of our research integrity team to safeguard the quality of each article we publish.
Find out more
BRIEF RESEARCH REPORT article
Front. Arachn. Sci., 26 April 2023
Sec. Arachnid Microbiota and Diseases
Volume 2 - 2023 | https://doi.org/10.3389/frchs.2023.1166039
Scorpion venoms have been studied extensively, mostly aimed at applications for human health, with strong evidence of antimicrobial properties. However, ecological studies on the adaptive role of these antimicrobial properties have been mostly neglected. Here, this study investigated in the scorpion Centruroides granosus Thorell, 1876 (Scorpiones: Buthidae) whether the venom provided protection against the consumption of crickets injected with a pathogenic strain of the bacteria Escherichia coli. Preventing venom injection when consuming contaminated prey decreased scorpion survival as compared to their controls (phosphate-buffered saline (PBS) injection) and scorpions that injected the venom. Scorpions that injected the venom did not show lower survival when consuming contaminated prey as compared to their own control, and there was no difference in survival for the controls of scorpions that were prevented or allowed to inject the venom. Altogether, the results highlight the adaptive benefit of the venom for scorpions when coping with potentially hazardous prey. The implications of the findings are discussed, and this work seeks to encourage more work on the neglected field of venom evolutionary ecology of scorpions and other arthropods.
For invertebrates, arachnids possess some of the most unusual means of defense against pathogens. For example, the silk of Tegenaria domestica has antibacterial properties (Wright and Goodacre, 2012), the subsocial spider Diaea ergandros possesses cuticular antifungals (González-Tokman et al., 2014), ticks can use fragments of the host blood for their own defense against bacteria in the midgut (Nakajima et al., 2005), and social spiders use social immunity against parasites (Straus and Avilés, 2018). Moreover, many venomous arachnids possibly contain microbiomes in their venom glands that contribute partially or completely to the production of antimicrobial components in the venom (Ul-Hasan et al., 2019).
For scorpions and spiders, venoms are a mixture of peptides that serve as protection against predators, for capturing prey, and for pre-digestion of prey, and they are active against bacteria, fungi, viruses, and protozoans (Wang and Wang, 2016). In addition to their importance in applied research, venoms provide the opportunity to study the ecology and evolution of adaptive traits of the toxins that they contain. For instance, the best-known adaptive value is predator–prey interactions (predation and defense); however, they fulfill other functions that remain poorly understood (e.g., food storage, digestion, and offspring care; Schendel et al., 2019). Another potential function might be a defense against pathogens, which is mostly overlooked in the literature on animal venoms (van der Meijden et al., 2017; Schendel et al., 2019). It is reasonable to imagine that the reported antimicrobial properties of scorpion venoms have an adaptive benefit, and it is not simply a byproduct. Still, few studies have investigated the ecological role of scorpion venoms in host–pathogen interactions. Gao et al. (2007) provided some indication that the venom may protect the venom gland from infections, and the spraying behavior of some species may relate to coping with microbes (Torres-Larios et al., 2000). However, none of these studies provide evidence in terms of improved survival. Therefore, we decided to test in a seminatural setting whether the venom may be an important component of the immune weaponry of scorpions by improving survival.
Here, we investigated in vivo whether the inoculation of the venom by the scorpion Centruroides granosus provides protection in terms of survival against the consumption of prey contaminated with the bacteria Escherichia coli. We used this pathogen–host combination since we found that this bacteria strain was virulent against this scorpion species, and their immune system is able to respond against non-lethal challenges (Gálvez et al., 2020). We predicted that when consuming contaminated prey, 1) scorpions that were allowed to inject their venom should show similar survival rates as compared to their control (phosphate-buffered saline (PBS) injection). 2) Scorpions that were not allowed to inject their venom should show lower survival rates as compared to their control. 3) Scorpions that were allowed to inject their venom should show higher survival rates than scorpions that were prevented. In addition, we studied the inhibitory capacity of the venom on the growth of E. coli in vitro by spreading a solution of the venom or the control together with the bacteria in a solid medium. We predicted a lower number of colony-forming units (CFUs) in the presence of venom. This study highlights the relevance of studying scorpions’ venoms in an ecological context beyond predation or defense.
C. granosus is an endemic species from Panama (de Armas et al., 2011), and we collected scorpions from a dirt road in the town of Sajalices (8.6815°N, 79.8661°W) and were fed in the laboratory with the house cricket Acheta domesticus, 2 weeks before the experiments and the venom extraction. Scorpions were placed in separate 460-ml lidded containers provided with water on a piece of cotton. The same type of container was used for the experiments. All scorpions were collected under the permit SE/AH-2-18 issued by the “Ministerio de Ambiente”.
A strain of E. coli—which is virulent for C. granosus (Gálvez et al., 2020)—was used to test whether inoculation of the venom to contaminated cricket increases the survival of the scorpion after consumption. The bacteria were cultured overnight on lysogeny broth at 27°C. Then, 14 ml of the culture (LD50 1 × 107 cells/ml) was centrifuged at 4,000 rpm for 5 min, and the pellet was washed with PBS and resuspended in 14 ml of PBS.
To increase the chances that the scorpions would accept the crickets, all the scorpions were subjected to a week of starvation. Out of the total of scorpions, 94 individuals had their stinger temporarily blocked (SB), which consisted of wrapping around a piece of adhesive tape (1.3 × 3.0 cm), with the help of forceps and CO2 anesthesia. The taping did not allow the scorpions to inject the venom into the cricket. Stinger-free scorpions (SF) did not have a piece of tape and were handled with forceps in a similar manner to the SB scorpions, including the use of CO2 anesthesia.
As a control, half of both the SB and SF scorpions were offered a cricket injected with 50 μl of PBS (SB-Control, n = 47; SF-Control, n = 45). For the bacterial challenge, half of both the SB and SF scorpions were offered a cricket injected with 50 μl of E. coli in PBS (SB-E. coli, n = 47; SF-E. coli, n = 46). All the crickets were offered immediately after injections, and SF scorpions were monitored to assure that the prey was stung. Scorpions of similar sizes (trying to allocate same-sex individuals) were placed in the four treatments, and all the scorpions consumed the crickets, and survival was monitored for 35 days. The tape from the SB scorpions was removed after they consumed the crickets, and SF scorpions were handled in a similar way after consuming the cricket.
We performed a single manual extraction of each scorpion by exciting the scorpion to sting repeatedly on a piece of stretched Parafilm, and we used only female scorpions. E. coli was cultured as described before, and it was diluted to 1 × 106 cells/ml in PBS. A volume of 138 nl of venom was placed in an Eppendorf tube, and then 0.5 μl of the cell culture was added with 50 μl of PBS. For the controls, 138 nl of venom was replaced with 138 nl PBS. By using an L-shaped glass spreader, the solution with the venom was placed in one half of a Petri dish (⌀ = 90 mm) and the control solution in the other half. The plates were cultured overnight (14 h), and the number of CFUs was counted. This was replicated with venoms from 14 scorpions that were not used in the in vivo experiment.
For the in vivo experiment, we performed a survival analysis to test for differences in survival rates in R (R Core Team, 2023). The type of cricket (bacteria-injected/control-injected) and stinger treatment (blocked/free) were specified as fixed factors with full interactions. Scorpions’ sex was added as a factor as well. For the in vitro experiment, the number of CFUs when mixing the bacteria with the venom solution or the control was compared by implementing a paired Wilcoxon test.
Stinger treatment had no effect on the survival of scorpions (X2 = 0.51, d.f. = 182, p = 0.47), and the type of cricket neither influenced the survival of scorpions (X2 = 3.03, d.f. = 181, p = 0.08). However, an interaction between stinger treatment and type of cricket (X2 = 4.1, d.f. = 180, p = 0.04) indicates that contaminated crickets reduced the survival of scorpions that had their stinger blocked (X2 = 7.3, d.f. = 91, p = 0.007, Figure 1A) but had no effect for scorpions that had their stingers free (X2 = 0.10, d.f. = 88, p = 0.75, Figure 1B). Stinger-free scorpions show higher survival when consuming contaminated crickets as compared to stinger-blocked scorpions (X2 = 3.7, d.f. = 90, p = 0.05, Figure 1C), and feeding on control crickets did not cause differences in survival for stinger-free or stinger-blocked scorpions (X2 = 1.05, d.f. = 89, p = 0.30, Figure 1D). The sex of the scorpion had no effect on survival (X2 = 1.04, d.f. = 180, p = 0.31, Supplementary Figure 1).
Figure 1 Kaplan–Meier survival curves of scorpions under different stinger treatments (blocked, SB; free, SF) and fed with crickets subjected to different injections (Control or Escherichia coli). The curves have been split into four panels for clarity: (A) SB scorpions, (B) SF scorpions, (C) SB vs SF scorpions fed with E.coli-injected crickets and (D) SB vs SF scorpions fed with Control-injected crickets. The * indicates significant differences
The venom of the scorpions inhibited the growth of E. coli, as compared to the control solution in terms of CFUs (436 ± 241 vs. 340 ± 292, respectively: V = 84, p = 0.05, n =14, Figure 2).
Figure 2 Growth in vitro of Escherichia coli when mixed with control solution (phosphate-buffered saline (PBS)) or venom and measured as the number of colony-forming units (CFUs). The * indicates significant differences.
Venom use is a fundamental component in the ecology of scorpions for both predation and defense, and this work shows its relevance as part of the immunological repertoire of scorpions. Overall, the three predictions were supported. For instance, preventing the scorpions from injecting venom into contaminated prey reduced their survival after consumption as compared to the individuals that were allowed to inject it. Possessing a simpler adaptive immune system, as compared to insects (Bechsgaard et al., 2016), venoms would provide an extra layer of defense against pathogens. Although a dual role for antimicrobial peptides (AMPs) in their venoms had been suggested for prey digestion and defense against microbes (Yacoub et al., 2020), this study demonstrates experimentally for the first time its immune benefit when foraging on potentially hazardous prey contaminated with pathogenic bacteria.
The result from the in vitro experiment implies that the venom acting on the bacteria is responsible for the improved survival of the scorpions. The efficacy of scorpion venoms to inhibit bacterial growth is well known (Wang and Wang, 2016); including the venom of several Centruroides species (e.g. Dueñas-Cuellar et al., 2015; Valdez-Velazquéz et al., 2016). Moreover, the inactivation of the bacteria by the venom may trigger an immune priming response. In a previous study, C. granosus injected with lipopolysaccharides from E. coli showed higher survival against a lethal dose of the same bacteria as compared to naïve individuals (Gálvez et al., 2020).
One could imagine that this benefit makes scorpions less meticulous when facing infected prey. Theoretical models should consider the implementation of venom in the interaction of infected prey and predator, together with epidemiological consequences, particularly when the predator population is also at risk of infection (Hsieh and Hsiao, 2008), with the venom reducing the risk. Moreover, scorpions could stop or reduce the spread of a pathogen across prey and conspecifics by consuming vulnerable prey (Hethcote et al., 2004). Scorpion venoms may also mitigate the negative effects of consuming infected prey (Flick et al., 2016), but further work is required to measure variables like longevity and fecundity. Experiments with other bacteria are also needed because some bacteria may not be inhibited by the venom (Gao et al., 2007). Moreover, since venom production is costly (Evans et al., 2019) and scorpions optimize their use (“venom optimization hypothesis”, Morgenstern and King, 2013), perhaps scorpions evaluate the health status of prey (e.g., odor) and adjust venom use accordingly. A similar scenario is known from other arthropods; ants can recognize the infectious potential of prey (Pereira and Detrain, 2020) and use venom and behavioral responses as immune defenses (Baracchi and Tragust, 2017). Further work can shed some light into the ability of scorpions to respond toward sick prey.
Venom as an immune defense may provide new insights into the evolution of scorpions’ immune systems. In a phylogenetic context, do species that depend on their pincers for subjugating the prey and rely less on venom (García et al., 2022) have “stronger” immune systems? Given that those species also tend to have less potent venoms (Forde et al., 2022), this prediction only holds if the same toxins acting on prey or predators have antimicrobial properties as well. Additionally, these results offer the possibility to explore multiple aspects of venom ecology related to infection risk from foraging and how it may vary with changing ecological factors that influence venom quality like diet (Tobassum et al., 2018), sex (Gao et al., 2021), predation pressure (Gangur et al., 2017), and ontogeny, which in fact influence the expression of antimicrobial peptides in the venom gland (McElroy et al., 2017). This work demonstrates the adaptive benefit of the antimicrobial properties of scorpion venoms, and it highlights the importance of understanding its influence on basic elements of host–pathogen ecology. Finally, our work seeks to encourage the study of venom ecology and evolution in arthropods in general.
The original contributions presented in the study are included in the article/Supplementary Material. Further inquiries can be directed to the corresponding author.
Scorpion collection and identification by all authors. Experiments performed by all authors. Data analysis by DG. Manuscript drafting by DG. Revision of the draft by all authors. All authors contributed to the article and approved the submitted version.
This study was funded by the Sistema Nacional de Investigación and the Vicerrectoría de Investigación y Postgrado from the University of Panama.
The authors declare that the research was conducted in the absence of any commercial or financial relationships that could be construed as a potential conflict of interest.
All claims expressed in this article are solely those of the authors and do not necessarily represent those of their affiliated organizations, or those of the publisher, the editors and the reviewers. Any product that may be evaluated in this article, or claim that may be made by its manufacturer, is not guaranteed or endorsed by the publisher.
The Supplementary Material for this article can be found online at: https://www.frontiersin.org/articles/10.3389/frchs.2023.1166039/full#supplementary-material
Baracchi D., Tragust S. (2017). “Venom as a component of external immune defense in hymenoptera,” in Evolution of venomous animals and their toxins. Eds. Gopalakrishnakone P., Malhotra A. (Dordrecht: Springer Nature), 213–233. doi: 10.1007/978-94-007-6458-3
Bechsgaard J., Vanthournout B., Funch P., Vestbo S., Gibbs R. A., Richards S., et al. (2016). Comparative genomic study of arachnid immune systems indicates loss of βGRPs and the IMD pathway. J. Evolutionary Biol. 29, 277–291. doi: 10.1111/jeb.12780
de Armas L. F., Teruel R., Kovařík F. (2011). Redescription of centruroides granosus (Thorell 1876) and identity of centrurus granosus simplex thorell 1876(Scorpiones: buthidae). Euscorpius 2011, 1–11. doi: 10.18590/euscorpius.2011.vol2011.iss127.1
Dueñas-Cuellar R. A., Kushmerick C., Naves L., Batista I., Guerrero-Vargas J., Pires O. Jr., et al. (2015). Cm38: a new antimicrobial peptide active against klebsiella pneumoniae is homologous to Cn11. Protein Pept. Lett. 22, 164–172. doi: 10.2174/092986652202150128143048
Evans E. R. J., Northfield T. D., Daly N. L., Wilson D. T. (2019). Venom costs and optimization in scorpions. Front. Ecol. Evol. 7 196. doi: 10.3389/fevo.2019.00196
Flick A. J., Acevedo M. A., Elderd B. D. (2016). The negative effects of pathogen-infected prey on predators: a meta-analysis. Oikos 125, 1554–1560. doi: 10.1111/oik.03458
Forde A., Jacobsen A., Dugon M. M., Healy K. (2022). Scorpion species with smaller body sizes and narrower chelae have the highest venom potency. Toxins 14, 219. doi: 10.3390/toxins14030219
Gálvez D., Añino Y., Vega C., Bonilla E. (2020). Immune priming against bacteria in spiders and scorpions? PeerJ 8, e9285. doi: 10.7717/peerj.9285
Gangur A. N., Smout M., Liddell M. J., Seymour J. E., Wilson D., Northfield T. D. (2017). Changes in predator exposure, but not in diet, induce phenotypic plasticity in scorpion venom. Proc. R. Soc. B: Biol. Sci. 284, 20171364. doi: 10.1098/rspb.2017.1364
Gao B., Tian C., Zhu S. (2007). Inducible antibacterial response of scorpion venom gland. Peptides 28, 2299–2305. doi: 10.1016/j.peptides.2007.10.004
Gao S., Wu F., Chen X., Yang Y., Zhu Y., Xiao L., et al. (2021). Sex-biased gene expression of mesobuthus martensii collected from gansu province, China, reveals their different therapeutic potentials. Evidence-Based Complementary Alternativa Med. 2021, 1967158. doi: 10.1155/2021/1967158
García L. F., Valenzuela-Rojas J. C., González-Gómez J. C., Lacava M., van der Meijden A. (2022). Pinching or stinging? comparing prey capture among scorpions with contrasting morphologies. J. Venomous Anim. Toxins Including Trop. Dis. 28, e20210037. doi: 10.1590/1678-9199-JVATITD-2021-0037
González-Tokman D., Ruch J., Pulpitel T., Ponton F. (2014). Cuticular antifungals in spiders: density- and condition dependence. PLoS One 9, e91785. doi: 10.1371/journal.pone.0091785
Hethcote H. W., Wang W., Han L., Ma Z. (2004). A predator - prey model with infected prey. Theor. Population Biol. 66, 259–268. doi: 10.1016/j.tpb.2004.06.010
Hsieh Y. H., Hsiao C. K. (2008). Predator-prey model with disease infection in both populations. Math. Med. Biol. 25, 247–266. doi: 10.1093/imammb/dqn017
McElroy T., Mcreynolds C. N., Gulledge A., Knight K. R., Smith E., Albrecht E. A. (2017). Differential toxicity and venom gland gene expression in Centruroides vittatus. PLoS One 12, e0184695. doi: 10.1371/journal.pone.0184695
Morgenstern D., King G. F. (2013). Toxicon the venom optimization hypothesis revisited. Toxicon 63, 120–128. doi: 10.1016/j.toxicon.2012.11.022
Nakajima Y. N., Saido-Sakanaka H., Ogihara K., Taylor D., Yamakawa M. (2005). Antibacterial peptides are secreted into the midgut lumen to provide antibacterial midgut defense in the soft tick, ornithodoros moubata (Acari: argasidae). Appl. Entomology Zoology 40, 391–397. doi: 10.1303/aez.2005.391
Pereira H., Detrain C. (2020). Pathogen avoidance and prey discrimination in ants. R. Soc. Open Sci. 7, 191705. doi: 10.1098/rsos.191705
R Core Team (2023). R: a language and environment for statistical computing (Vienna, Austria: R Foundation for Statistical Computing).
Schendel V., Rash L. D., Jenner R. A., Undheim E. A. B. (2019). The diversity of venom: the importance of behavior and venom system morphology in understanding its ecology and evolution. Toxins 11, 666. doi: 10.3390/toxins11110666
Straus S., Avilés L. (2018). Effects of host colony size and hygiene behaviours on social spider kleptoparasite loads along an elevation gradient. Funct. Ecol. 32, 2707–2716. doi: 10.1111/1365-2435.13225
Tobassum S., Tahir H. M., Zahid M. T., Gardner Q. A., Ahsan M. M. (2018). Effect of milking method, diet, and temperature on venom production in scorpions. J. Insect Sci. 18, 1–7. doi: 10.1093/jisesa/iey081
Torres-Larios A., Gurrola G. B., Zamudio F. Z., Possani L. D. (2000). Hadrurin, a new antimicrobial peptide from the venom of the scorpion hadrurus aztecus. Eur. J. Biochem. 267, 5023–5031. doi: 10.1046/j.1432-1327.2000.01556.x
Ul-Hasan S., Rodríguez-Román E., Reitzel A. M., Adams R. M. M., Herzig V., Nobile C. J., et al. (2019). The emerging field of venom-microbiomics for exploring venom as a microenvironment, and the corresponding initiative for venom associated microbes and parasites (iVAMP). Toxicon: X 4, 100016. doi: 10.1016/j.toxcx.2019.100016
Valdez-Velazquéz L., Romero-Gutierrez M., Delgado-Enciso I., Dobrovinskaya O., Melnikov V., Quintero-Hénandez V., et al. (2016). Comprehensive analysis of venom from the scorpion centruroides tecomanus reveals compounds with antimicrobial, cytotoxic and insecticidal activities. Toxicon 118, 95–103. doi: 10.1016/j.toxicon.2016.04.046
van der Meijden A., Koch B., van der Valk T., Vargas-Muñoz L. J., Estrada-Gómez S. (2017). Target-specificity in scorpions; comparing lethality of scorpion venoms across arthropods and vertebrates. Toxins 9, 312. doi: 10.3390/toxins9100312
Wang X., Wang G. (2016). Insights into antimicrobial peptides from spiders and scorpions. Protein Pept. Lett. 23, 707–721. doi: 10.2174/0929866523666160511151320
Wright S., Goodacre S. L. (2012). Evidence for antimicrobial activity associated with common house spider silk. BMC Res. Notes 5, 326. doi: 10.1186/1756-0500-5-326
Keywords: arachnid, bacteria, buthidae, ecological immunology, toxins
Citation: Gálvez D, Bonilla E and Vega C (2023) Scorpion venom and its adaptive role against pathogens: a case study in Centruroides granosus Thorell, 1876 and Escherichia coli. Front. Arachn. Sci. 2:1166039. doi: 10.3389/frchs.2023.1166039
Received: 14 February 2023; Accepted: 04 April 2023;
Published: 26 April 2023.
Edited by:
Dasiel Obregon, University of Guelph, CanadaReviewed by:
Volker Herzig, University of the Sunshine Coast, AustraliaCopyright © 2023 Gálvez, Bonilla and Vega. This is an open-access article distributed under the terms of the Creative Commons Attribution License (CC BY). The use, distribution or reproduction in other forums is permitted, provided the original author(s) and the copyright owner(s) are credited and that the original publication in this journal is cited, in accordance with accepted academic practice. No use, distribution or reproduction is permitted which does not comply with these terms.
*Correspondence: Dumas Gálvez, ZHVtYXMuZ2FsdmV6c0B1cC5hYy5wYQ==
Disclaimer: All claims expressed in this article are solely those of the authors and do not necessarily represent those of their affiliated organizations, or those of the publisher, the editors and the reviewers. Any product that may be evaluated in this article or claim that may be made by its manufacturer is not guaranteed or endorsed by the publisher.
Research integrity at Frontiers
Learn more about the work of our research integrity team to safeguard the quality of each article we publish.