- 1Unidad de Investigación para el Conocimiento, Uso y Valoración de la Biodiversidad, Centro de Estudios Conservacionistas, Facultad de Ciencias Químicas y Farmacia, Universidad de San Carlos de Guatemala, Guatemala City, Guatemala
- 2General Zoology, Institute for Biology, Martin Luther University Halle-Wittenberg, Halle, Germany
Land use, local management, and seasonal variation significantly impact the ecological dynamics of bee–plant communities and their ecological interactions. These variables negatively affect diversity and ecological interaction networks within human-dominated landscapes. Additionally, seasonal variables such as temperature, rainfall, and resource availability across different seasons play essential roles in shaping bee communities and their interactions with flowering plants. However, little is known about how diversity and ecological interaction networks of non-crop plants in agricultural landscapes respond to intra-seasonal variations, specifically within the rainy season. In this study, we assessed how land use types, coffee crop management, and intra-seasonal variation within the rainy season influenced the composition and diversity of bee and plant communities, and their interaction networks in semi-natural habitats surrounding coffee plantations. We recorded the diversity of bees and plants and analysed their interactions networks metrics, such as specialisation, nestedness, modularity, connectance and bee/plant generality, in 8 pairs of sites. Our findings indicate that human settlements negatively influence bee generality, suggesting that human-dominated land and the introduction of exotic plants reduce floral resources for bees, which may decrease bee visitation. In contrast, extensive semi-natural and forested areas seemed to support bee generality. Additionally, we observed higher visit frequency and richness of bees and plant generality during the second period of the rainy season (July to October), leading to more robust bee–plant interaction networks in the same period. This study enhances our understanding of how land-use types and intra-seasonal climatic variation shape structure of bee floral visitor communities and their interactions with flowering plants. Furthermore, our findings underline the negative impact of human-dominated landscapes on the ecological dynamics of plants visited by bees and their interaction networks.
Introduction
Animal-mediated pollination plays a key role in ~30–35% of global crop production (Klein et al., 2007; Khalifa et al., 2021). For instance, fruit and seed set of crops are generally enhanced by the visitation of bees (Garibaldi et al., 2013), which makes them the most effective pollinators for managed plants (Danforth, 2007). Unfortunately, bees are declining worldwide (Potts et al., 2010; IPBES, 2019; Graham et al., 2024). Several factors have been associated with bee decline, such as 1) land use change, 2) climate change, 3) introduction of alien species, 4) pathogens (González-Varo et al., 2013), and 5) use of pesticides (Thomann et al., 2013; Scheper et al., 2014). Land use change, mediated by the increase of agricultural and urban lands, is considered the main factor causing the decline of bees (Ollerton et al., 2014; Goulson et al., 2015; Dicks et al., 2021). Besides, the reduction of the quality and heterogeneity of habitats has also been observed to negatively affect bee–plant interactions (Moreira et al., 2015). However, variation in landscape structure, such as landscape composition and landscape configuration, across multiple landscape scales, has been observed to influence bee taxa and their interactions with flowering plants (Moreira et al., 2015; Dáttilo et al., 2023). In this sense, determining the response of bee–plant interactions to landscape context is crucial for proposing conservation strategies for both bees and plants in tropical agricultural lands (Saturni et al., 2016; Moreira et al., 2015).
In addition, in tropical agroforestry systems, the presence and timing of mass-flowering crops, along with the management of local vegetation—particularly tree diversity and canopy cover—significantly impact the abundance and richness of bee communities, which are crucial for pollination services in croplands (Jha and Vandermeer, 2010; Reynolds et al., 2022). For example, forest remnants near coffee plantations, particularly those with greater forest edge density (Brosi et al., 2007; Kremen and Miles, 2012), enhance pollinator diversity and activity, leading to increased fruit production (Ricketts, 2004; Klein et al., 2003; De Marco and Coelho, 2004; Brosi et al., 2008; Vergara and Badano, 2009; González-Chaves et al., 2020). Similarly, heterogeneity of semi-natural areas surrounding crop areas favour the abundance of certain functional groups of floral visitors and act as complementary floral resources when crops are not in bloom (Landaverde-González et al., 2017; Galpern et al., 2021; Cavigliasso et al., 2022). Additionally, bee species rely on resources predominantly found in forest and semi-natural areas, such as floral resources (i.e., pollen and nectar), and non-floral resources (i.e., honeydew, resins, and nesting sites in herbs and woody plant stems) (Requier and Leonhardt, 2020; Chui et al., 2022; Ulyshen et al., 2023). Consequently, forest and semi-natural areas can serve as a source of wild pollinators and pollination services for neighbouring habitats, such as crop-growing areas (Simba et al., 2018; Ricketts et al., 2008; Martínez-Núñez et al., 2022; Escobedo-Kenefic et al., 2024). However, crop management effects can spillover into natural/semi-natural areas, potentially affecting the forest’s ability to provide pollinators (Blitzer et al., 2012). Unfortunately, the ecological dynamic of bee communities in the vegetation surrounding coffee plantations and their relationship with land-use and crop management are poorly documented (Machado et al., 2021), principally in the Neotropics. Thus, understanding the ecological dynamics of bee floral visitor communities and their interactions with landscape and crop management in the tropics is vital for maintaining pollination services available to coffee (Machado et al., 2021).
On the other hand, seasonal climatic variation (Ramírez et al., 2015; Samnegård et al., 2015; Souza et al., 2017; Escobedo-Kenefic et al., 2020; Cortés-Flores et al., 2023) and monthly/intra-seasonal climatic variation (Stewart and Waitayachart, 2020; Chávez-González et al., 2020) has a profound impact on the ecological dynamics of bee communities. As a general trend, tropical zones are not characterised by abrupt temperature fluctuations; however, rainfall undergoes changes throughout the year and across interannual periods as well (Barron, 1995; Ciemer et al., 2019). This could lead to changes in the foraging activities of floral visitors and the availability of flowering plants at both temporal scales. Such variation translates into changes in the co-occurrence of species from both trophic levels, and their interactions (Souza et al., 2017; Escobedo-Kenefic et al., 2020; Stewart and Waitayachart, 2020; Cortés-Flores et al., 2023). Despite these observed patterns, research on the impact of these temporal dynamics remains limited, mainly in the Neotropics where much is still unknown. In this sense, describing the temporal dynamics of flower visitor-plant interactions is key to understanding the organisation of the communities of both trophic levels, and the stability of pollination services, especially in tropical habitats (Souza et al., 2017; Escobedo-Kenefic et al., 2020; Ballarin et al., 2022).
In this study, we assessed the impact of land use types, coffee crop management, and climatic intra-seasonal variation on the composition and diversity of bee and plant communities, and their interaction networks in semi-natural areas surrounding coffee crops. Based on previous works, we expected a positive impact of increasing human settlement and semi-natural covers on bee diversity, since both land covers potentially provide rich-flower habitats for bees (Landaverde-González et al., 2017; Escobedo-Kenefic et al., 2020, 2022), and a positive impact of organic crop management on both bee and plant communities (Karanja et al., 2010; Batáry et al., 2011; Kremen and Miles, 2012) and their interaction networks (Escobedo-Kenefic et al., 2020, 2022). Lastly, we predicted a greater diversity of bee flower visitors and an increase in the frequency of interactions between bees and flowering plants during the second part of the rainy season. This expectation was based on phenological shifts triggered by the onset of the first rains, fostering an upsurge in the activity of flower visitors and their interactions with plants (Ballarin et al., 2022; Stewart and Waitayachart, 2020; Chávez-González et al., 2020; Elzinga et al., 2007).
Materials and methods
Area of study
We conducted the study in Guatemala, Central America. The sampling was performed in 8 pairs of sites located in the departments of Quetzaltenango, Suchitepéquez, Sololá, Sacatepéquez, Guatemala, Santa Rosa, Jalapa, and El Progreso, one pair in each department, within an elevation range of 748 to 1802 masl (Supplementary Table S1). In addition, the study area is embedded into two life zones within the pre-mountain stratum, sensu Holdridge criteria: Tropical pre-mountain humid forest, with an annual precipitation range of 1000 to 3125 mm, and temperature range of 18 to 24°C (Supplementary Table S1), and Tropical pre-mountain very humid forest, with an annual precipitation range of 2000 to 4850 mm, and a temperature range of 18 to 24°C (same range of the former life zone) (Supplementary Table S1) (IARNA-URL, 2018).
Throughout this region, the species Coffea arabica L. is cultivated and harvested in shaded conditions, under the canopy of forest remnants and plantations, which are mostly comprised of native Inga spp. Mill (Fabaceae) trees and the exotic Grevillea robusta A.Cunn. ex R.Br. (Proteaceae) (ANACAFE, 2011). In addition, coffee is usually cultivated in association with other crops, such as rambutan Nephelium lappaceum L. (Sapindaceae), mangosteen Garcinia mangostana L. (Clusiaceae), tea Camellia sinensis (L.) Kuntze (Theaceae), and macadamia Macadamia integrifolia Maiden & Betche (Proteaceae), where coffee is dominant. Semi-natural vegetation is characterised mostly by young trees, bushes, and weeds and areas of bare soil, which primarily constitute non-cultivated and poorly vegetated areas. Human settlements are also present around the zones dedicated to coffee cultivation. The removal of small bushes and herbs is a common practice across all land covers, especially in semi-natural and human settlement areas neighbouring coffee crops (Casiá-Ajché et al., 2023). Altogether, crop growing areas (including coffee and other associated plantations), forest, semi-natural vegetation areas, and human settlement areas are the principal land-uses in our study sites.
Experimental design
Our fieldwork was carried out in 8 pairs of sites in semi-natural areas, each consisting of two different managed sites (one agroecological and one conventional), with a distance of at least 1 km from each other (Figure 1; information and coordinates of sites in Supplementary Table S1). “Agroecological management” refers to the management in which the nearby crops have higher proportions of tree shadow cover, low agrochemical use, use of organic fertilisers, high crop biodiversity and more traditional sustainable practices, while “conventional management” refers to a management where coffee crops have lower tree shadow cover, rely on agrochemicals, have lower crop biodiversity, and less use of traditional sustainable practices. For more details see Escobar-González et al. (2024).
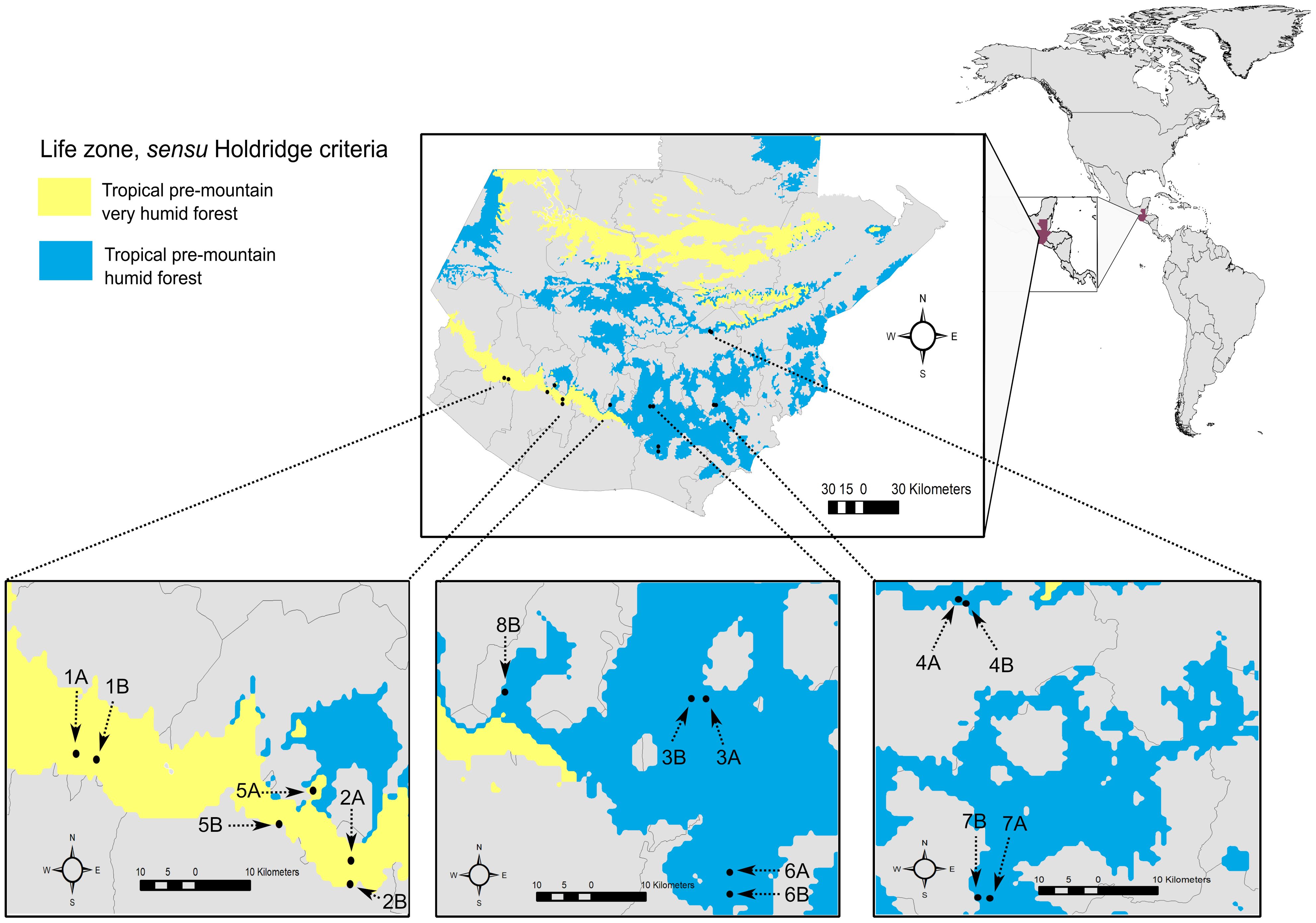
Figure 1. Locations of study sites within the area devoted to coffee cultivation in Guatemala. Arrows points to the localities at each site. The sites are embedded within two life zones, sensu Holdridge criteria. Tropical pre-mountain very humid forest region is shaded yellow and Tropical pre-mountain humid forest region is shaded in blue. Life zones follow the clasification shown by IARNA-URL (2018). Guatemala country is shaded red on the continental map. Sites names: Finca Comunidad Nueva Alianza (A1), Finca Patrocinio (A2), Finca Los Andes (2A), Finca Panamá (2B). Finca Guadabarranca (3A). Finca El Bosque (3B), Parcela Saul N. (4A), Finca La Montañita (4B), Finca San Isidro Chacayá (5A). Finca Milán (5B), Finca Santa Isabel (6A), Finca Joya Grande (6B), Finca Luisa N (7A), Finca Juan N (7B), Finca Buenaventura (8B). For further details on the study sites, see Supplementary Table S1.
At each site, we recorded bee flower visitors in semi-natural areas across the 8 pairs of sites, recording the identity and frequency of bee-visited plants, and their interactions. Based on these observations, we calculated the composition and diversity of both bee and plant communities and constructed their interaction networks. Fieldwork was carried out during the rainy season, from the final part of March 2018 (onset period of the first rains) to October 2021 (Supplementary Table S2). Intra-seasonal periods were defined as high-precipitation peaks (period A: March to June) and (period B: July to October), separated by a short absent or low precipitation period (see Supplementary Figure S1; Supplementary Table S3).
Landscape classification and analyses
We classified land uses into five categories: (i) forest, (ii) semi-natural vegetation, (iii) crops, (iv) bare soil, and (v) human settlements (Supplementary Table S5). Since satellite images for each specific year were not available, the closest available images were used (Supplementary Table S2). Using the coordinates of the sampling sites, we calculated the proportion and edge density of each land use within radii of 1000, 700, 500, and 250 meters, surrounding each sampling sites, for the corresponding years (Supplementary Figure S2; Supplementary Table S4). This was done using Google Earth images version 7.3.4.8642 (Google Earth/Maxar Technologies, 2020) with a resolution of 15 meters, the supervised classification algorithm from the ArcGIS software (ESRI, 2023), and the library landscape metrics 1.5.5 (Hesselbarth et al., 2019) from RStudio (R Core Team, 2022). To define the spatial scale at which bee and plant composition, diversity, and their interaction networks respond to the landscape variables, we evaluated the correlation between the landscape variables and the network and community metrics using Pearson correlation, as in Landaverde-González et al. (2017). The spatial scale with the highest correlation for all metrics was 700 meters, so we used this scale in the subsequent analyses (Supplementary Table S5).
Sampling of bees and their interactions
Bees and their interactions with flowering plants were collected through transect walks in semi-natural vegetation areas surrounding coffee plantations. The observations were exhaustive and conducted over 2.5 hours per site (total of 40 hours), covering 5-minute observation on each of 30 different plants, per sampling event at each locality. To ensure consistent sampling effort across sites, we used 30 individuals—a statistically recommended sample size (Zar, 1999; Mendenhall and Sincich, 2016)—regardless of the species of flowering plants observed, accounting for the diversity of plants around plantations. Bee visits were recorded for individuals that touched or searched for the reproductive parts of the observed flower. Bee visitors and herbarium specimens of observed plants were collected when these could not be identified in situ, for later taxonomic identification. Samplings were performed during the high activity hours for flower visitors (9am–1pm), and during sunny weather conditions. We conducted a total of 42 samplings –transects- (mean transects per locality = 2.8; SD = ± 0. 34) across 8 pairs of sites from 2018 to 2021 (Supplementary Table S2). For each sampling –transect-, we calculated the diversity and composition metrics of both bee and plant communities, as well as the metrics of their interaction networks.
Bee and plant composition and diversity
Both bee and plant individuals were identified to the lowest taxonomic level (genus or species level), using the available identification keys for the region. Bees: Michener et al. (1994); Ayala (1999); Ayala and Griswold (2012); Ayala-Barajas (2016); Balboa et al. (2017); Mérida-Rivas et al. (2022). Plants: Standley and Steyermark (1946–1977). Specimens that could not be identified to species level were defined as morphospecies and classified using morphological criteria. To determine the diversity and composition of both bee and plant communities and to analyse their response to land use types, coffee crop management, and intra-seasonal climatic variation, we calculated: 1) diversity metrics: visit frequency, Chao1 corrected richness (Chao and Shen, 2003), and Shannon diversity index (Shannon and Weaver, 1949); and 2) composition metric: local contribution of beta-diversity (LCBD), based on Sorensen dissimilarity index (Legendre and De Cáceres, 2013). Large LCBD values indicate sites that have strongly different species compositions compared with sites with lower or mean values (Legendre, 2014), which also indicates the ecological uniqueness of sites according to their contribution to global beta-diversity. The diversity metrics were calculated using the Past statistics software, version 4.09 (Hammer et al., 2001), while the LCBD values were calculated using the library adespatial v0.3-8 (Dray et al., 2012), in RStudio software (R Core Team, 2022).
Bee–plant interaction
We constructed species/morphospecies-based interaction networks in which bees were considered the higher trophic level (81 taxa identified to species level; 62 taxa identified to morphospecies), and plants, the lower trophic level (160 taxa identified to species level; 15 taxa identified to morphospecies). From each matrix, we computed the metrics: specialisation (H2′), nestedness (NODF), modularity (Q), and connectance (C), described in detail in Escobedo-Kenefic et al. (2020, 2022). We also compute network generality for both trophic levels (bees and plants). Bee generality quantifies the weighted mean effective number of high-level species (bees) per lower-level species (plants) (Bersier et al., 2002). Conversely, plant generality quantifies the weighted mean effective number of lower-level species (plants) per higher-level species (bees); this metric was calculated following vulnerability by Bersier et al. (2002). The network from La Montañita locality in El Progreso department, northeastern of Guatemala (Site code 4B, network 26, Conventional management, and Tropical pre-mountain humid forest, visited in September 2019) was too small to compute the metrics, so we excluded it from the following analyses (Supplementary Table S6).
Due to the dependence of network metrics on network dimensions, we performed delta (Δ)-transformations of the metrics. On the basis of 1000 random networks, Δ-transformed metrics were calculated as Net (obs) −Net (rand-mean) in which Net (obs) is the raw value of the metric and Net (rand-mean) is the mean value of 1,000 random networks, as presented in Escobedo-Kenefic et al. (2020); Landaverde-González et al. (2021), and Escobedo-Kenefic et al. (2022). All the analyses were performed using the bipartite package (Dormann et al., 2009) in RStudio (R Core Team, 2022). In addition, we used t-tests to evaluate whether Δ-transformed metrics are consistently different than zero; Supplementary Table S7.
Statistical analyses
Regarding our question about analysing the effects of land use and coffee crop management on the composition and diversity of both bee and plant communities, and their interaction networks, we used linear mixed models (LMMs) and generalised linear mixed models (GLMs). The composition metric (LCBD values) and diversity metrics (visit frequency, Chao1 corrected richness and Shannon diversity index) of bee and plant communities, and bee-plant network descriptors (Δ-specialisation (H2), Δ-nestedness, Δ-modularity, Δ-connectance, Δ-bee generality, Δ-plant generality), were defined as response variables. To assess the influence of land use categories and their edge density on the response variables, landscape variables were defined as fixed factors. To evaluate the influence of crop management on the response variables, a categorical variable with two levels for coffee management—agroecological and conventional—was defined as a fixed factor; and to measure the impact of plant diversity metrics on bees, plant community metrics were set also as fixed factors. We set site (n = 8) and sampling year (2018 to 2021) as random factors in all the models used to assess our first question.
To address our second question about analysing the effects of intra-seasonal variation on response variables, we used linear mixed models (LMMs) and generalised mixed models (GLMs). Precipitation period, a categorical variable with two levels for each of the precipitation periods A and B, was defined as a fixed factor. For these last models, we set site (n = 8) and sampling year (2018 to 2021) as random factors.
Using the fitdistrplus package 1.2-1 (Delignette-Muller and Dutang, 2015), we confirmed that our continuous response variables had a normal distribution, except for Δ-plant generality. We then normalised this variable using the Log10 (x) transformation. Finally, we tested all model residuals for normality using a Shapiro-Wilk test, using the Past statistics software, version 4.09 (Hammer et al., 2001). The other model assumptions (linearity and homogeneity of variances) were verified visually. These models were run following the Gaussian distribution and using the nlme package 3.1-131.1 (Pinheiro et al., 2018), in R 2.3-1 (R Core Team, 2022). Additionally, visit frequency of bees adjusted to Poisson distribution and Chao1 corrected richness of bees followed a negative binomial distribution. This was verified using the JMP statistical software (SAS Institute Inc, 2012). Both variables were modelled using their respectively fitted distribution using the lme4 package 1.1-35.5 (Bates et al., 2015) in R 2.3-1 (R Core Team, 2022).
In models with landscape variables as fixed factors, we did a model selection by evaluating the effects of land use variables and their edge density on the response variables in separate models, by applying the Akaike Information Criterion (AIC), and its second order value (AICc), in the AICmodavg library 2.3-1 (Mazerolle, 2020). We repeated the analyses with the selected variables, with AICc values < 2, and reported the most parsimonious models (AICc values < 2), as in Escobedo-Kenefic et al. (2022) (Supplementary Table S8).
Additionally, we compared the composition of bee and plant communities between two intra-seasonal precipitation periods, using a paired permutational multivariate analysis of variance (PERMANOVA) using the Wd*-test available in the MicEco package (Russel, 2021). We used non-metric multidimensional scaling (NMDS) to visualise the variation of composition of bee and plant communities between intra-seasonal precipitation periods. Bray-Curtis’s distance matrix was the distance metric with the best fit (stress = 0.186 for bee communities; stress= 0.135 for plant communities) and was the response variable in the PERMANOVA analysis. In addition, precipitation period, a categorical variable with two levels for each of the precipitation periods A and B, was set as the independent variable. The strata (block) argument was set to site, so randomisations were constrained to occur within each site and not across all the sites.
Results
Composition, diversity, and interactions of bees and plants
We sampled 1702 bees (more details in Supplementary Figure S3; Supplementary Table S9) and identified 81 species and 62 morphospecies of bees from 60 genera. Overall, 1151 specimens were Apidae (67.63%), 343 Halictidae (20.15%), 139 Megachilidae (8.17%), 54 Andrenidae (3.17%) and 15 Colletidae (0.88%). The most frequent species recorded throughout the study sites was the stingless bee Trigona fulviventris Guérin-Méneville, 1845 (14.33%). The remaining taxa did not exceed 6% of the total sampled specimens (Supplementary Table S3). Bee taxa visited 160 species and 15 morphospecies of flowering plants. Bees visited more frequently the following plants: 194 visits registered in Bidens pilosa L. (Asteraceae) (11%) and 93 in Sida acuta Burm.f. (Malvaceae) (5%). The rest of the taxa did not exceed 5% of their frequencies of the total plants (Supplementary Figure S3; Supplementary Table S10). We observed 1702 interactions within bee–plant networks, with Asteraceae family and stingless bees being the most linked taxa (Supplementary Figure S4). Among our study sites, the highest values of local contribution to beta-diversity of both bees and plants were found in: Finca Buenaventura (conventional management and Tropical pre-mountain humid forest) (Site code 8B, LCBD bees = 0.15; LCBD plants = 0.18); Finca El Bosque (Site code 3B, LCBD bees = 0.15; LCBD plants = 0.11) and Finca Panamá (Site code 2B, LCBD bees = 0.16; LCBD plants = 0.11) (both localities with conventional management in Tropical pre-mountain very humid forest). (Supplementary Figures S7, S8).
Effects of land use and coffee crop management on bees and plants
The proportion of land use types (forest, semi-natural vegetation, crop, bare soil, and human settlement covers) and their edge density did not exhibit a significant relationship with the local contribution to beta-diversity (LCBD), visit frequency, Chao1 corrected richness, and Shannon diversity of bees (P > 0.05). For the plant communities, the local contribution to beta-diversity (LCBD), visit frequency, Chao1 corrected richness, and Shannon diversity index were not significantly affected by the proportion of land uses or their edge density (P > 0.05). None of the bee and plant diversity and composition metrics had any significant relationship with the management systems (P > 0.05). None of the plant diversity metrics showed a significant relationship with the bee diversity metrics (P > 0.05).
Effects of land use and coffee crop management on bee–plant interaction networks
Regarding bee–plant interaction networks, there were no significant relationships between the proportion of land uses or their edge density with Δ-connectance, Δ-specialisation (H2’), Δ-nestedness, Δ-modularity, and Δ-plant generality (P > 0.05). We observed that Δ-bee generality was not related to any of the edge density of any land use, but concerning the proportion of land use, it did show a marginal negative relationship with human settlement cover (LMM; t = −1.87; P = 0.08; Table 1; Figure 2). Additionally, Δ-bee generality (across all the sites) was significantly higher than 0 (2.40 mean ± 0.65 SD, P < 0.05) (Supplementary Table S7; Supplementary Figure S10E). None of the Δ-network metrics were significantly different between crop management systems (P > 0.05) (Supplementary Figure S11).

Table 1. Top linear mixed model explaining the effects of land use categories (within 700 m radius buffers) on Δ-bee generality.
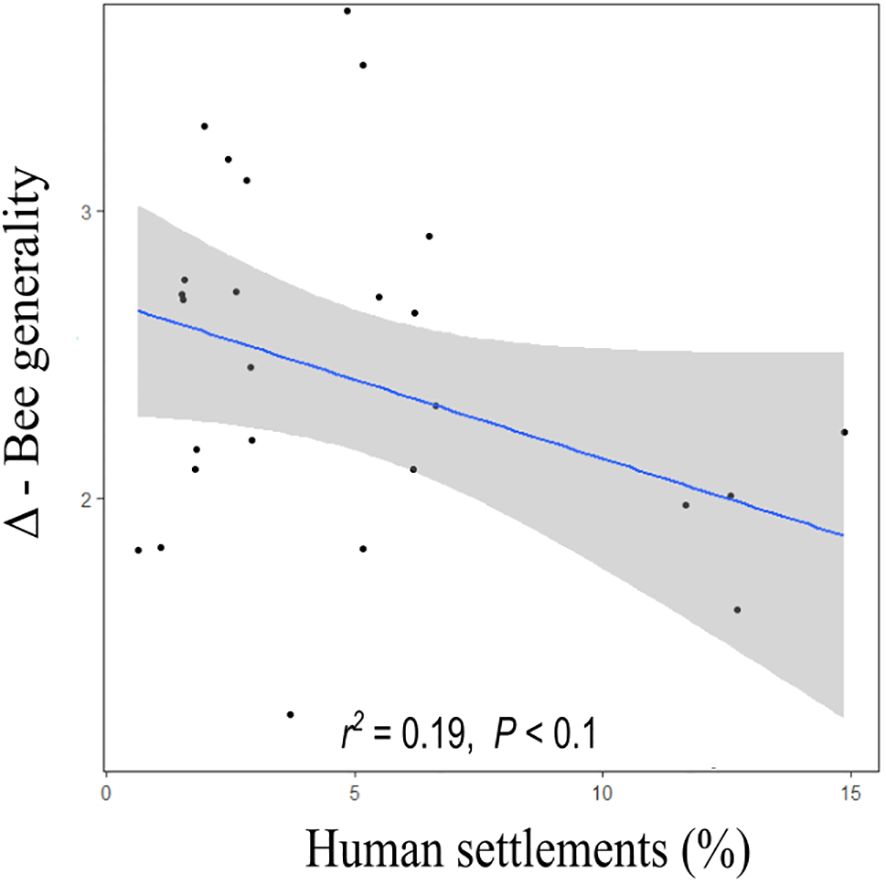
Figure 2. Effects of land use categories within 700 m radius buffers on Δ-bee generality. Significant levels are indicated as (•P<0.1).
Effects of intra-seasonal precipitation variation on composition and diversity of bees and plants
Neither plant local contribution to beta-diversity nor diversity showed changes between periods of intra-seasonal climatic variation (P > 0.05). The visit frequency and Chao1 corrected richness of bees were significantly different between the intra-seasonal precipitation periods; both descriptors were higher in the second part of the rainy season, encompassing the months of July to October (GLM; z = 0.26; P < 0.001; Table 2; Figure 3; and GLM; z = 3.17; P = <0.01; Table 2; Figure 3; respectively).
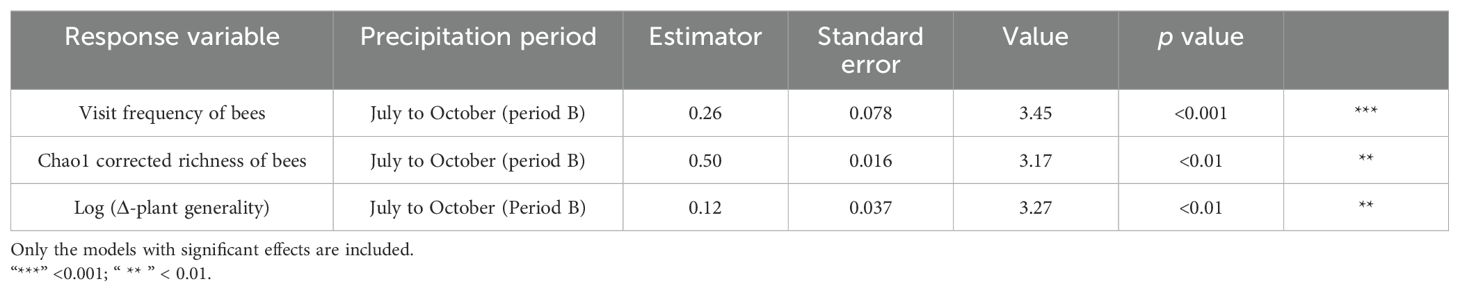
Table 2. Top linear mixed and generalised models explaining the effects of intra-seasonal precipitation periods (period A: March to June; period B: July to August) on Visit frequency of bees, Chao1 corrected richness of bees, and Log (Δ-plant generality).
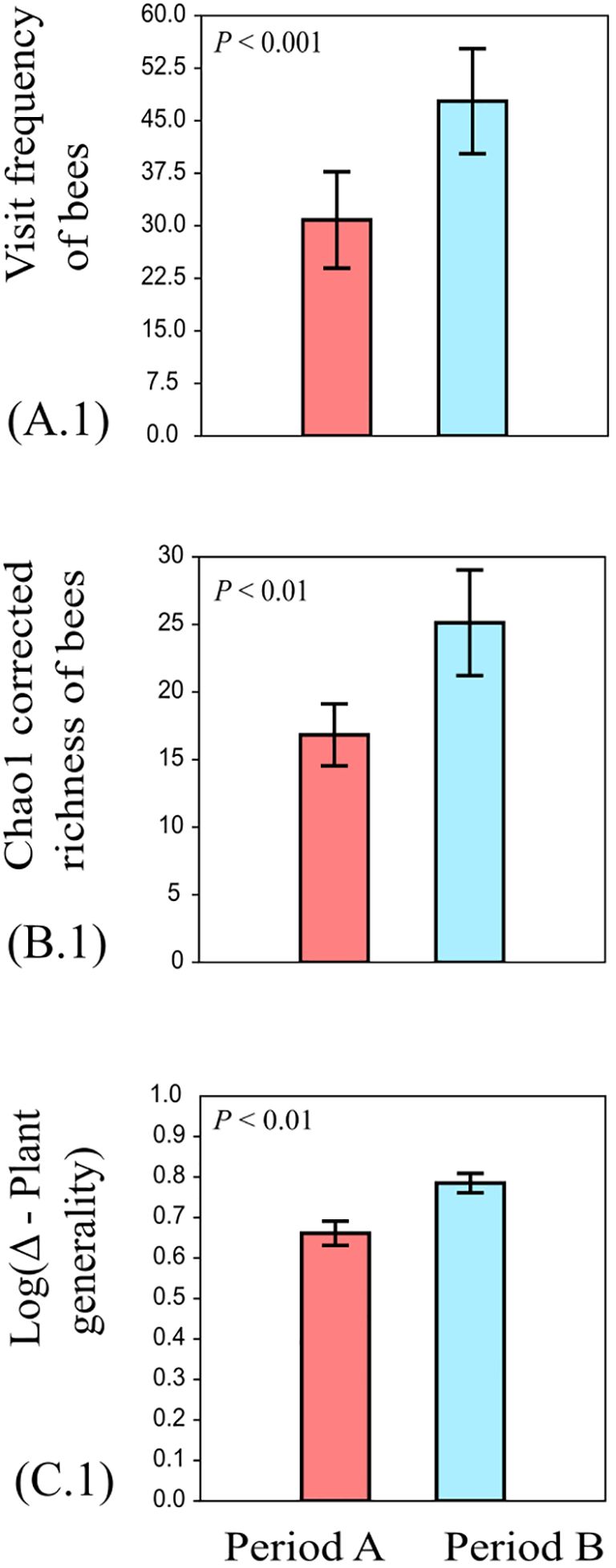
Figure 3. Effect of intra-seasonal precipitation periods (period A: March to June; period B: July to October) on Visit frequency of bees, Chao1 corrected richness of bees, and Log (Δ-plant generality). (A.1) = Visit frequency of bees, (B.1) = Chao1 corrected richness of bees, and (C.1) = Log (Δ-plant generality). Whiskers on bars represent the standard error of mean values. Significant levels are noted as follows: (P<0.001, P<0.01). Red bars = period A (March to June). Blue bars = period B (July to October).
Plant community composition did not vary between the periods of intra-seasonal climatic variation (PERMANOVA, P > 0.05); Supplementary Figure S9. However, bee community differed between the intra-seasonal periods (PERMANOVA, W = 2.30; P < 0.01; Figure 4).
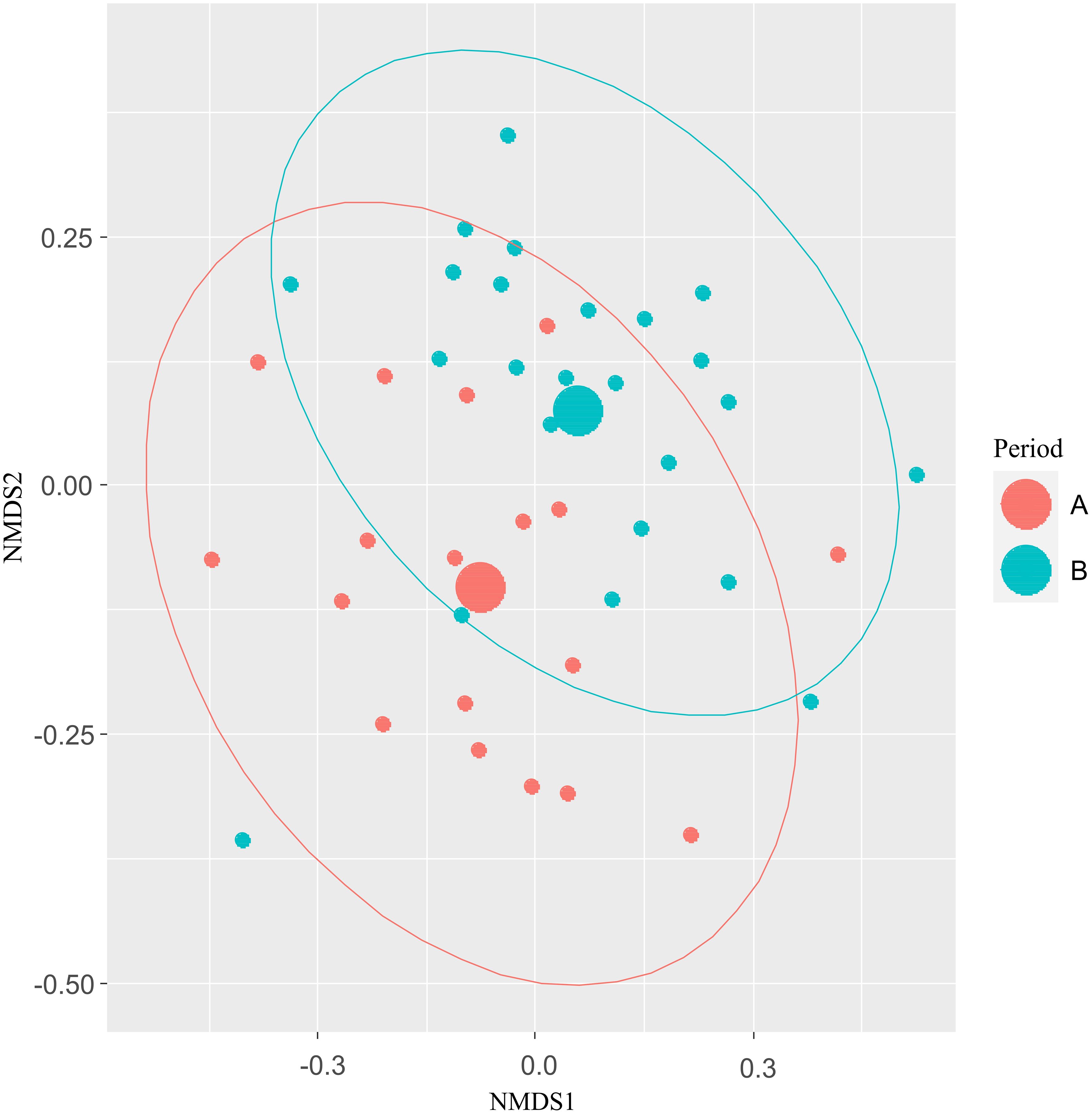
Figure 4. Non-metric multidimensional scaling (NMDS) ordination analysis of bee communities between intra-seasonal precipitation periods (period A: March to June and period B: July to October). Red colour = period A (March to June). Blue colour = period B (July to October).
Effects of intra-seasonal precipitation variation on interaction networks
The network is more generalist during the second period of the rainy season, with bees visiting a more diverse range of plants. However, in both periods, the Asteraceae family remains the most visited plant taxa (Supplementary Figures S5, S6). Similarly, Δ-plant generality was the only metric of interaction networks that showed differences among intra-seasonal precipitation periods; Δ-plant-generality was significantly higher in the second part of the rainy season (t = 3.27; P < 0.01; Table 2; Figure 3). Additionally, Δ-plant generality was significantly higher than 0 in both intra-seasonal precipitation periods: Δ-plant generality period A (March to June), 3.77 mean ± 1.39 SD, P < 0.05; Δ-plant generality: period B (July to October); 5.31 mean ± 1.82 SD, P < 0,05) (Supplementary Table S6; Supplementary Figure S12F).
Discussion
Regarding our first expectation, we observed that an increase in human settlement cover had a negative relationship with bee generality of interaction networks. However, crop management showed no effect on the diversity of both bee and plants communities, or their interaction networks. Regarding our second expectation, we found that the composition of bee communities differed between the early and late rainy season. Additionally, both visit frequency and richness of bees and plant generality of interaction networks were higher in the second period of the rainy season, after a short low-precipitation period. We discuss our results in more detail in the following paragraphs.
Composition and diversity of bees and plants
The number of individuals and species richness of flower visitors recorded in our study are lower than those observed in previous studies conducted in semi-natural areas and coffee crops, with more forest cover in Sololá department in Guatemala (Armas-Quiñonez et al., 2020) and Costa Rica highlands (Cristóbal-Perez et al., 2024). However, unlike our study, both studies conducted monthly sampling, which may explain the greater number of species they observed. In contrast, we observed greater richness of species and abundance compared to other studies conducted in tropical areas. This included studies in coffee crops, in the same sites of this work (Escobar-González et al., 2024), semi-natural areas in the highlands of Guatemala (Escobedo-Kenefic et al., 2020), semi-natural areas and Cucurbita pepo L. crops in Guatemala and Costa Rica (Enríquez et al., 2015; Lobo and Bravo-Méndez, 2021), and semi-natural areas and habanero chili crops in Yucatán, Mexico. In our study, the majority of species belonged to the Apidae family and the second most abundant was the Halictidae family, consistent with other studies in the region (Enríquez et al., 2015; Landaverde-González et al., 2017; Escobedo-Kenefic et al., 2020, 2022; Armas-Quiñonez et al., 2020; Lobo and Bravo-Méndez, 2021; Cristóbal-Perez et al., 2024), indicating a consistent bee family composition across the region.
Our study shows a similar composition of bee-visited plants as reported by Escobedo-Kenefic et al. (2020, 2022) and Flórez-Gómez et al. (2020), where the Asteraceae family was the most visited. In our study, Malvaceae was the second most bee visited family. In contrast, Escobedo-Kenefic et al. (2020) reported Brassicaceae as the second most visited family, while Flórez-Gómez et al. (2020) reported Fabaceae as the second most visited during the rainy season. However, during the dry season, Flórez-Gómez et al. (2020) found that the Fabaceae family was the most visited. This variation seems to depend on the ecosystem and the type of semi-natural area surrounding the study sites.
Effects of land use and coffee crop management on bees and plants
We observed no significant difference in community composition of bee and plant communities in areas surrounding coffee plantations with different coffee management. Previous works have found no changes in composition of bee communities (Brosi et al., 2007; Serralta-Batun et al., 2024) and plant communities (Dominschek et al., 2021; Serralta-Batun et al., 2024) between semi-natural vegetation remanent and agricultural settings, suggesting that the influence of the management of nearby crops does not cause a change in bee and plant communities. Similarly, crop management did not cause a change in floral visitors on coffee at the same sites of this work (Escobar-González et al., 2024). This pattern may be because many coffee plantations are embedded within Guatemala’s national protected areas system, which includes extensive forested and seminatural areas (Supplementary Table S4; Supplementary Figure S2). These natural habitats likely moderate the effects of different management practices, maintaining relatively low-intensity land use across the landscape. While there are differences in management intensity, they may not be extreme enough to significantly impact bee and plant communities of the surrounding areas, as discussed by Escobar-González et al. (2024).
Furthermore, the diversity and composition of bees from vegetation surrounding coffee plantations were not significantly related to any land use variable. Previous investigations have showed that bee visitation frequency and diversity can be affected by local factors such as the abundance and richness of flowering plants surrounding tropical agricultural lands (Landaverde-González et al., 2017; Laha et al., 2020; Escobedo-Kenefic et al., 2020, 2022; Assunção et al., 2022). Semi-natural and agricultural lands have been also found to reduce bee taxonomic and phylogenetic diversity by 16% (Tsang et al., 2024). In addition, we did not observe any relationship between the visit frequency and richness of flowers and bees in the areas surrounding coffee, which might have been caused by a transient dilution of pollinators, likely due to increased competition for pollinators between mass flowering crops such as coffee and wild plants in the surrounding areas (Holzschuh et al., 2016; Grab et al., 2017). We also observed three sites with large values of LCBD for both bee and plant communities, Supplementary Figures S7, S8, indicating that these sites had high species turnover, and were ecologically unique and distinct from the others. This may suggest that they are degraded and species-poor, as discussed by Legendre (2014). Interestingly, all three of these sites are conventional farms with a higher degree of human settlements compared to the other farms as shown in Supplementary Figure S2, suggesting that the type of management and human intervention, at least for these farms, has an impact on the surrounding vegetation, and consequently on pollinators. Intentional removal of wild weeds is a common practice in our study sites, especially in human-dominated land uses such as crop and human settlement cover (Casiá-Ajché et al., 2023). This translates directly into reduced plant diversity available to bees, which may have negative consequences for bee populations surrounding coffee plantations (Machado et al., 2021). However, the extensive natural areas around these farms appear to buffer the unique ecological characteristics of these sites when viewed within the broader landscape context. Previous studies indicate that urbanisation decreases plant richness, with animal-dependent species being more susceptible than those species dispersed by abiotic variables, particularly in tropical regions (McKinney, 2002; Kolbe et al., 2016; Theodorou et al., 2020; Ruas et al., 2022). However, studies in the Neotropics thus far have been limited, and insufficient to fully comprehend which urban drivers, and how the complex relationship between different drivers may have a greater impact on plant diversity and pollinators in tropical urban areas (Ruas et al., 2022; Solís-Montero et al., 2023). In this regard, future studies need to consider the impact of each variable within tropical urban environments on the diversity of plants and pollinators.
Effects of land use and coffee crop management on bee–plant interaction networks
We observed that bee generality decreased with the increase of human settlement cover. Our results align with Amado De Santis et al. (2023) in Argentina and Suni et al. (2022) in California, who reported an increase in specialisation in urban areas, possibly due to the presence of invasive plants, which may be leading to changes in pollinator fidelity for exotic plants in urban areas. Opposite effects have been found in temperate regions, where the heterogeneity of intermediate succession in disturbed semi-natural areas led to the formation of robust ecological networks, with consistent generality despite the varying levels of habitat loss (McWilliams et al., 2019; Deprá et al., 2022). Silva et al. (2020) showed that urbanisation may pose a significant threat to pollinator–plant networks in tropical regions, however, these ecosystems remain understudied, warranting further research. Furthermore, bee generality exceeded 0 across all sites, indicating that bees are more generalised than expected by chance. This suggests that although human settlements tend to reduce the number of flower visitors visiting plants, the system remains generalist, possibly due to the availability of diverse floral resources in the surrounding semi-natural and forested areas. Considering that our sampling areas predominantly encompass agricultural regions, with high forest and semi-natural cover, and with urban areas comprising about 5% of the landscape, our findings suggest that while urbanisation does influence bee generality, it may be present in low enough levels to not significantly disrupt the overall stability of the interaction network. The strong representation of semi-natural and forested areas in our study, as shown in Supplementary Figure S2, likely explains the lack of variability in other network metrics, as these areas may act as a buffer against the effects of crop management on diversity of bees and their interactions with plants. This underscores the robustness of the structure of bee-plant networks at the current intensity of land use and human disturbance from settlements.
Intra-seasonal effects on both bee and plant composition and diversity, and their interactions
We observed a different composition of bee flower visitors during the two periods within the rainy season. Furthermore, visit frequency and richness of bees showed a significant increase in the second period of the rainy season (i.e. July to October). It has been outlined that tropical plant communities often synchronise their flowering with the peak of seasonal rainfall (Lasky et al., 2016). In our study sites, the period from July to October marks the second annual peak of rainfall. The effect of precipitation period could have been caused by precipitation-driven blooming of plant communities (Lasky et al., 2016; Cortés-Flores et al., 2017, 2023). Similarly, several studies have shown that pollinator diversity varies according to floral resource abundance. In natural systems, a strong correlation between pollinators and their services with flower abundance has frequently been observed, which favours pollination during early flowering, although pollinator abundance increases as flowering progresses (Elzinga et al., 2007). Other studies have demonstrated that bee and wasp diversity correlate with the number of plant species and flowers (Abrahamczyk et al., 2011). This correlation is suggested to be due to the availability of flowers and nectar, which increase with rainfall (Ballarin et al., 2022). Other studies observed a significant influence of floral richness and precipitation on both abundance and richness of pollinators. However, despite these influences, the interaction networks remained stable across months and years (Stewart and Waitayachart, 2020) or across varying levels of habitat disturbance (McWilliams et al., 2019; Deprá et al., 2022). Similarly, we observed that most of the network metrics did not vary between the precipitation periods, except for plant generality, which increased during the second period of precipitation. This indicates that with the increase in rainfall during the second period, the number of plant species (lower trophic level) visited by each bee species significantly increases compared to the increase in the number of bee species (high trophic level). This effect is notably more pronounced in the second precipitation period, where heightened precipitation likely supports an increase in the blooming of plant communities. Furthermore, plant generality exceeded 0 in both intra-seasonal precipitation periods, indicating that plants are more generalised than expected by chance during both periods. Altogether the increase in visit frequency and richness of bees may be specifically linked to rainfall-triggered blooming that positively increases plant generality, resulting in a more robust network during the second part of the rainy season.
Conclusions
Our study indicates that urbanisation increased bee specialisation, likely due to the presence of exotic plants in human-disturbed areas. Additionally, we found that bee populations were more generalised than expected by chance, possibly because of the buffering effect provided by extensive semi-natural and forested habitats surrounding coffee plantations in the study area. Bee community compositions varied between two periods within the rainy season. Furthermore, visit frequency and richness of bees increasing during the second period of the rainy season (July to October), likely due to rainfall-triggered blooming within plant communities. Despite the influence of floral resources and precipitation on visit frequency and richness of bees, ecological interaction networks remained stable across months and years in agricultural lands. The only exception was plant generality of interaction networks, which changed across both precipitation periods, suggesting an increase of in the number of visited plant species by bee species during the second precipitation period. Future studies should explore the impact of variations in urban use and detailed intra-seasonal variability, such as precipitation, on plant and pollinator diversity in other areas in tropical environments. Our results underscore the importance of considering local factors and environmental variables in understanding and managing pollinator communities in semi-natural areas surrounding agricultural landscapes.
Data availability statement
The original contributions presented in the study are included in the article/Supplementary Material. Further inquiries can be directed to the corresponding authors.
Ethics statement
The manuscript presents research on animals that do not require ethical approval for their study.
Author contributions
QC-A: Data curation, Formal analysis, Methodology, Writing – original draft, Writing – review & editing. NE-K: Formal analysis, Supervision, Validation, Writing – review & editing. DE-G: Data curation, Methodology, Writing – review & editing. EC: Data curation, Methodology, Writing – review & editing. AM-C: Data curation, Methodology, Writing – review & editing. JM-S: Writing – review & editing, Data curation, Methodology. EE: Formal analysis, Funding acquisition, Supervision, Writing – review & editing, Conceptualization, Project administration. PL-G: Formal analysis, Funding acquisition, Supervision, Validation, Writing – original draft, Writing – review & editing, Conceptualization, Investigation, Methodology, Software.
Funding
The author(s) declare financial support was received for the research, authorship, and/or publication of this article. This work was partially sponsored by the Schlumberger Foundation, Faculty for the Future for the funding provided to PL-G, the Dirección General de Investigación de la Universidad de San Carlos de Guatemala (DIGI) through Research Project 4.8.63.8.60 during 2018 and Research Project 4.8.63.4.41 during 2019, and by the Early Career Fellowship Programme Number 45004272284 from the Organization for Women in Science for the Developing World (OWSD), from 2021 to 2023.
Acknowledgments
We thank Claudia García and Juan Zelada from the Asociación de Reservas Naturales Privadas de Guatemala (ARNPG) for their logistics support during the development of the research, and the 15 owners who allowed us to carry out the research in their coffee farms. We also thank the Centro de Estudios Conservacionistas (CECON) of the Universidad de San Carlos de Guatemala and especially the Unidad de Biodiversidad team for providing continued support in the form of human resources, equipment, and logistics, and Lester González, Cristian Méndez and Daniela Herrera for their help on field sampling.
Conflict of interest
The authors declare that the research was conducted in the absence of any commercial or financial relationships that could be construed as a potential conflict of interest.
Publisher’s note
All claims expressed in this article are solely those of the authors and do not necessarily represent those of their affiliated organizations, or those of the publisher, the editors and the reviewers. Any product that may be evaluated in this article, or claim that may be made by its manufacturer, is not guaranteed or endorsed by the publisher.
Supplementary material
The Supplementary Material for this article can be found online at: https://www.frontiersin.org/articles/10.3389/frbee.2024.1408854/full#supplementary-material
References
Abrahamczyk S., Kluge J., Gareca Y., Reichle S., Kessler M. (2011). The influence of climatic seasonality on the diversity of different tropical pollinator groups. PloS One 6, e27115. doi: 10.1371/journal.pone.0027115
Amado De Santis A. A., Lomáscolo S. B., Chacoff N. P. (2023). Effects of urbanization on the structure of plant-flower visitor network at the local and landscape levels in the northern Argentinian Yungas forest. Front. Sustain. Cities. 5. doi: 10.3389/frsc.2023.1086076
Armas-Quiñonez G., Ayala-Barajas R., Avendaño-Mendoza C., Lindig-Cisneros R., del-Val E. (2020). Bee diversity in secondary forests and coffee plantations in a transition between foothills and highlands in the Guatemalan Pacific Coast. PeerJ 8, e9257. doi: 10.7717/peerj.9257
Assunção R. M., Camargo N. F., Souza L. S., Rocha E. M., Tostes G. M., Sujii E. R., et al. (2022). Landscape conservation and local interactions with non-crop plants aid in structuring bee assemblages in organic tropical agroecosystems. J. Insect Conserv. 26, 933–945. doi: 10.1007/s10841-022-00438-8
Ayala R. (1999). Revisión de las abejas sin aguijón de México (Hymenoptera: Apidae: Meliponini). Folia Entomol. Mex. 106, 1–123. ISSN: 0430-8603.
Ayala R., Griswold T. (2012). Two new species of the bee genus Peponapis, with a key to the North and Central American species (Hymenoptera: Apidae: Eucerini). Rev. Mex. Biodivers. 83, 396–406. ISSN 2007-8706. doi: 10.22201/ib.20078706e.2012.2.1247
Ayala-Barajas R. (2016). Las abejas del género Plebeia Schwarz (Apidae: Meliponini) de México. Entomol. Mex. 3, 937–942. ISSN: 2448-475.
Balboa C., Hinojosa-Díaz I., Ayala R. (2017). New dark species of the bee genus Colletes (Hymenoptera, Colletidae) from Mexico and Guatemala. Zootaxa 4320, 401–425. doi: 10.11646/zootaxa.4320.3
Ballarin C. S., Hachuy-Filho L., Doria M. J. W., Giffu M. M., Polizello D. S., Oliveira P. H., et al. (2022). Intra-seasonal and daily variations in nectar availability affect bee assemblage in a monodominant afforested Brazilian Cerrado. Austral Ecol. 47, 1315–1328. doi: 10.1111/aec.13218
Barron E. J. (1995). “Tropical climate stability and implications for the distribution of life,” in Effects of past global change on life (National Academy Press Studies in Geophysics, Washington, DC), 108–117.
Batáry P., Báldi A., Kleijn D., Tscharntke T. (2011). Landscape-moderated biodiversity effects of agri-environmental management: a meta-analysis. Proc. R. Soc B. 278, 1894–1902. doi: 10.1098/rspb.2010.1923
Bates D., Maechler M., Bolker, Walker S. (2015). Fitting linear mixed-effects models using lme4. J. Stat. Software 67, 1–48. doi: 10.18637/jss.v067.i01
Bersier L. F., Banašek-Richter C., Cattin M. F. (2002). Quantitative descriptors of food-web matrices. Ecol 83, 2394–2407. doi: 10.1890/0012-9658(2002)083[2394:QDOFWM]2.0.CO;2
Blitzer E. J., Dormann C. F., Holzschuh A., Klein A. M., Rand T. A., Tscharntke T. (2012). Spillover of functionally important organisms between managed and natural habitats. Agric. Ecosyst. Environ. 146, 34–43. doi: 10.1016/j.agee.2011.09.005
Brosi B. J., Daily G. C., Ehrlich P. R. (2007). Bee community shift with landscape context in a tropical countryside. Ecol. Appl. 17, 418–430. doi: 10.1890/06-0029
Brosi B. J., Daily G. C., Shih T. M., Oviedo F., Durán G. (2008). The effects of forest fragmentation on bee communities in tropical countryside. J. Appl. Ecol. 45, 773–783. doi: 10.1111/j.1365-2664.2007.01412.x
Casiá-Ajché Q. B., Landaverde-González P., Escobedo-Kenefic N., Enríquez E. (2023). Efecto del paisaje y el manejo agrícola en la diversidad de abejas y en las redes de interacciones planta-polinizador en fincas de café (Coffea arabica L.), de Guatemala. Biology Licenciatura thesis (Guatemala: University of San Carlos de Guatemala).
Cavigliasso P., Phifer C. C., Knowlton J. L., Licata J. A., Flaspohler D. J., Webster C. R., et al. (2022). Influence of landscape composition on wild bee communities: Effects of functional landscape heterogeneity. Agric. Ecosyst. Environ. 340. doi: 10.1016/j.agee.2022.108150
Chao A., Shen T. J. (2003). Nonparametric estimation of Shannon’s index of diversity when there are unseen species in a sample. Environ. Ecol. Stat. 10, 429–443. doi: 10.1023/A:1026096204727
Chávez-González E., Vizentin-Bugoni J., Vázquez D. P., MacGregor-Fors I., Dáttilo W., Ortiz-Pulido R. (2020). Drivers of the structure of plant–hummingbird interaction networks at multiple temporal scales. Oecologia 193, 913–924. doi: 10.1007/s00442-020-04727-4
Chui S. X., Keller A., Leonhardt S. D. (2022). Functional resin use in solitary bees. Ecol. Entomol. 47, 115–136. doi: 10.1111/een.13103
Ciemer C., Boers N., Hirota M., Kurths J., Müller-Hansen F., Oliveira R. S., et al. (2019). Higher resilience to climatic disturbances in tropical vegetation exposed to more variable rainfall. Nat. Geosci. 12, 174–179. doi: 10.1038/s41561-019-0312-z
Cortés-Flores J., Hernández-Esquivel K. B., González-Rodríguez A., Ibarra-Manríquez G. (2017). Flowering phenology, growth forms, and pollination syndromes in tropical dry forest species: Influence of phylogeny and abiotic factors. Am. J. Bot. 104, 39–49. doi: 10.3732/ajb.1600305
Cortés-Flores J., Lopezaraiza-Mikel M., de Santiago-Hernández M. H., Martén-Rodríguez S., Cristóbal-Pérez E. J., Aguilar-Aguilar M. J., et al. (2023). Successional and phenological effects on plant-floral visitor interaction networks of a tropical dry forest. J. Ecol. 111, 927–942. doi: 10.1111/1365-2745.14072
Cristóbal-Perez E. J., Barrantes G., Cascante-Marín A., Hanson P., Picado B., Gamboa-Barrante N., et al. (2024). Elevational and seasonal patterns of plant pollinator networks in two highland tropical ecosystems in Costa Rica. PloS One 19, e0295258. doi: 10.1371/journal.pone.0295258
Dáttilo W., Regolin A. L., Baena-Díaz F., Boscolo D. (2023). Spatial scaling involving the complexity of biotic interactions: integrating concepts, current status, and future perspectives. Curr. Landsc. Ecol. 8, 137–148. doi: 10.1007/s40823-023-00090-1
Delignette-Muller M. L., Dutang C. (2015). fitdistrplus: an R package for fitting distributions. J. Stat. Soft. 64, 1–34. doi: 10.18637/jss.v064.i04
De Marco P., Coelho F. M. (2004). Services performed by the ecosystem: forest remnants influence agricultural cultures’ pollination and production. Biodivers. Conserv. 13, 1245–1255. doi: 10.1023/B:BIOC.0000019402.51193.e8
Deprá M. S., Evans D. M., Gaglianone M. C. (2022). Pioneer herbaceous plants contribute to the restoration of pollination interactions in restinga habitats in tropical Atlantic Forest. Restor. Ecol. 30, e13544. doi: 10.1111/rec.13544
Dicks L. V., Brezee T. D., Ngo H. T., Senapathi D., An J., Aizen M. A., et al. (2021). A global-scale expert assessment of drivers and risks associated with pollinator decline. Nat. Ecol. Evol. 5, 1453–1461. doi: 10.1038/s41559-021-01534-9
Dominschek R., Barroso A. A. M., Lang C. R., de Moraes A., Sulc R. M., Schuster M. Z. (2021). Crop rotations with temporary grassland shifts weed patterns and allows herbicide-free management without crop yield loss. J. Clean. Prod. 306, 127140. doi: 10.1016/j.jclepro.2021.127140
Dormann C. F., Fründ J., Blüthgen N., Gruber B. (2009). Indices, graphs and null models: analyzing bipartite ecological networks. Open Ecol. J. 2, 7–24. doi: 10.2174/1874213000902010007
Dray S., Pélissier R., Couteron P., Fortin M. J., Legendre P., Peres-Neto P. R., et al. (2012). Community ecology in the age of multivariate multiscale spatial analysis. Ecol. Monogr. 82, 257–275. doi: 10.1890/11-1183.1
Elzinga J. A., Atlan A., Biere A., Gigord L., Weis A. E., Bernasconi G. (2007). Time after time: flowering phenology and biotic interactions. Trends. Ecol. Evol. 22, 432–439. doi: 10.1016/j.tree.2007.05.006
Enríquez E., Ayala R., Gonzalez V. H., Núñez-Farfán J. (2015). Alpha and beta diversity of bees and their pollination role on Cucurbita pepo L. (Cucurbitaceae) in the Guatemalan cloud forest. Pan-Pac. Entomol. 91, 211–222. doi: 10.3956/2015-91.3.211
Escobar-González D., Landaverde-González P., Casiá-Ajché Q. B., Morales-Siná J., Cardona E., Mejía-Coroy A., et al. (2024). Fruit production in coffee (Coffea arabica L.) crops is enhanced by the behaviour of wild bees (Hymenoptera: Apidae). Aust. Entomol. 63, 83–95. doi: 10.1111/aen.12673
Escobedo-Kenefic N., Cardona E., Arizmendi M., Domínguez C. A. (2024). Do forest reserves help maintain pollinator diversity and pollination services in tropical agricultural highlands? A case study using Brassica rapa as a model. Front. Bee. Sci. 2. doi: 10.3389/frbee.2024.1393431
Escobedo-Kenefic N., Casiá-Ajché Q. B., Cardona E., Escobar-González D., Mejía A., Enríquez E., et al. (2022). Landscape or local? Distinct responses of flower visitor diversity and interaction networks to different land use scales in agricultural tropical highlands. Front. Sustain. Food Syst. 6. doi: 10.3389/fsufs.2022.974215
Escobedo-Kenefic N., Landaverde-González P., Theodorou P., Cardona E., Dardón M. J., Martínez O., et al. (2020). Disentangling the effects of local resources, landscape heterogeneity and climatic seasonality on bee diversity and plant−pollinator networks in tropical highlands. Oecologia 194, 333–344. doi: 10.1007/s00442-020-04715
Flórez-Gómez N. A., Maldonado-Cepeda J. D., Ospina-Torres R. (2020). Bee-plant interaction networks in a seasonal dry Tropical forest of the Colombian Caribbean. Neotrop. Entomol. 49, 533–544. doi: 10.1007/s13744-020-00804-8
Galpern P., Best L. R., Devries J. H., Johnson S. A. (2021). Wild bee responses to cropland landscape complexity are temporally-variable and taxon-specific: Evidence from a highly replicated pseudo-experiment. Agric. Ecosyst. Environ. 322. doi: 10.1016/j.agee.2021.107652
Garibaldi L. A., Steffan-Dewenter I., Winfree R., Aizen M. A., Bommarco R., Cunningham S. A., et al. (2013). Wild pollinators enhance fruit set of crops regardless of honey bee abundance. Science 339, 1608–1611. doi: 10.1126/science.1230200
González-Chaves A., Jaffé R., Metzger J. P., Kleinert A. M. P. (2020). Forest proximity rather than local forest cover affects bee diversity and coffee pollination services. Landsc. Ecol. 35, 1841–1855. doi: 10.1007/s10980-020-01061-1
González-Varo J. P., Beismeijer J. C., Bommarco R., Potts S., Schweiger O., Smith G. K., et al. (2013). Combined effect of global pressure on animal mediated-pollination. Trends Ecol. Evol. 28, 524–550. doi: 10.1016/j.tree.2013.05.008
Goulson D., Nicholls E., Botías C., Rotheray F. L. (2015). Bee declines driven by combined stress from parasites, pesticides and lack of flowers. Science 347, 1255957. doi: 10.1126/science.1255957
Grab H., Blitzer E., Danforth B., Loeb G., Poveda K. (2017). Temporally dependent pollinator competition and facilitation with mass flowering crops affects yield in co-blooming crops. Sci. Rep. 7, 45296. doi: 10.1038/srep45296
Graham K. K., Glaum P., Hartert J., Gibbs H., Tucker E., Isaacs R., et al. (2024). A century of wild bee sampling: historical data and neural network analysis reveal ecological traits associated with species loss. Proc. R. Soc. B. 29120232837. doi: 10.1098/rspb.2023.2837
Hammer Ø., Harper D. A. T., Ryan P. D. (2001). PAST paleontological statistics software package for education and data analysis. Paleontol. Electron. 4, 1–9. Available at: https://palaeo-electronica.org/2001_1/past/past.pdf.
Hesselbarth M. H. K., Sciani M., With K. A., Wiegand K., Nowosand J. (2019). landscapemetrics: an open-source R tool to calculate landscape metrics. Ecography 42, 1648–1647. doi: 10.1111/ecog.04617
Holzschuh A., Dainese M., González-Varo J. P., Mudri-Stojnić S., Riedinger V., Rundlöf M., et al. (2016). Mass-flowering crops dilute pollinator abundance in agricultural landscapes across Europe. Ecol. Lett. 19, 1228–1236. doi: 10.1111/ele.12657
IARNA-URL. (2018). Ecosistemas de Guatemala basado en el sistema de clasificación de zonas de vida (Guatemala: Instituto de Investigación y Proyección sobre Ambiente Natural y Sociedad de la Universidad Rafael Landívar).
IPBES. (2019). Summary for policymakers of the global assessment report on biodiversity and ecosystem services of the Intergovernmental Science-Policy Platform on Biodiversity and Ecosystem Services. Eds. Díaz S., Settele E. S., Brondízio E. S., Ngo H. T., Guèze M., Agard J., et al (Bonn, Germany: IPBES secretariat).
Jha S., Vandermeer J. H. (2010). Impacts of coffee agroforestry management on tropical bee communities. Biol. Conser. 143, 1423–1431. doi: 10.1016/j.biocon.2010.03.017
Karanja R. ,. H., Njoroge G., Gikungu M., Newton L. E. (2010). Bee interactions with wild flora around organic and conventional coffee farms in Kiambu district, central Kenya. J. Pollinat. Ecol. 2, 7–12. doi: 10.26786/1920-7603(2010)5
Khalifa S. A. M., Elshafiey E. H., Shetaia A. A., Abd El-Wahed A. A., Algethami A. F., Musharraf S. G., et al. (2021). Overview of bee pollination and it is value for crop production. Insects 12, 688. doi: 10.3390/insects12080688
Klein A., Steffan-Dewenter I., Tscharntke T. (2003). Fruit set of highland coffee increases with the diversity of pollinating bees. Proc. R. Soc B. 270, 955–961. doi: 10.1098/rspb.2002.2306
Klein A. M., Vaissière B. E., Cane J. H., Steffan-Dewenter I., Cunningham S. A., Kremen C., et al. (2007). Importance of pollinators in changing landscapes for world crops. Proc. R. Soc B. 274, 303–313. doi: 10.1098/rspb.2006.3721
Kolbe S. E., Miller A. I., Cameron G. N., Culley T. M. (2016). Effects of natural and anthropogenic environmental influences on tree community composition and structure in forests along an urban-wildland gradient in southwestern Ohio. Urban. Ecosyst. 19, 915–938. doi: 10.1007/s11252-016-0531-7
Kremen C., Miles A. (2012). Ecosystem services in biologically diversified versus conventional farming systems: benefits, externalities, and trade-offs. Ecol. Soc 17, 40. doi: 10.5751/ES-05035-170440
Laha S., Chatterjee S., Das A., Smith B., Basu P. (2020). Exploring the importance of floral resources and functional trait compatibility for maintaining bee fauna in tropical agricultural landscapes. J. Insect Conserv. 24, 431–443. doi: 10.1007/s10841-020-00225-3
Landaverde-González P., Enríquez E., Núñez-Farfán J. (2021). The effect of landscape on Cucurbita pepo-pollinator interaction networks varies depending on plants’ genetic diversity. Arthropod. Plant Interact. 15, 917–928. doi: 10.1007/s11829-021-09872-y
Landaverde-González P., Quesada-Euán J. J. G., Theodorou P., Murray E., Hussemann M., Ayala. R., et al. (2017). Sweat bees on hot chilies: provision of pollination services by native bee species in traditional slash-and-burn agriculture in Yucatán Peninsula of tropical Mexico. J. App. Ecol. 54, 1814–1824. doi: 10.1111/1365-2664.12860
Lasky J. L., Uriarte M., Muscarella R. (2016). Synchrony, compensatory dynamics, and the functional trait basis of phenological diversity in a tropical dry forest tree community: effects of rainfall seasonality. Environ. Res. Lett. 11, 115003. doi: 10.1088/1748-9326/11/11/115003
Legendre P. (2014). Replacement and richness difference components. Glob. Ecol. Biogeogr. 23, 1324–1334. doi: 10.1111/geb.12207
Legendre P., De Cáceres M. (2013). Beta diversity as the variance of community data: dissimilarity coefficients and partitioning. Ecol. Lett. 16, 951–963. doi: 10.1111/ele.12141
Lobo J. A., Bravo-Méndez Y. (2021). Diversity and foraging patterns of bees on flowers of Cucurbita pepo (Cucurbitaceae) in Costa Rica. Rev. Biol. Trop. 69, 494–506. doi: 10.15517/rbt.v69i2.44076
Machado A. C. P., Barônio G. J., de Oliveira F. F., Garcia C. T., Rech A. R. (2021). Does a coffee plantation host potential pollinators when it is not flowering? Bee distribution in an agricultural landscape with high biological diversity in the Brazilian Campo Rupestre. J. Sci. Food Agric. 101, 2345–2354. doi: 10.1002/jsfa.10857
Martínez-Núñez C., Kleijn D., Ganuza C., Heupink D., Raemakers I., Vertommen W., et al. (2022). Temporal and spatial heterogeneity of semi-natural habitat, but not crop diversity, is correlated with landscape pollinator richness. J. App. Ecol. 59, 1258–1267. doi: 10.1111/1365-2664.14137
Mazerolle M. J. (2020). AICcmodavg: Model selection and multimodel inference based on (Q)AIC(c). R package version 2.3.1. Available online at: https://cran.r-project.org/package=AICcmodavg (accessed September 2, 2024).
McKinney M. L. (2002). Urbanization, biodiversity, and conservation. BioScience 52, 883–890. doi: 10.1641/0006-3568(2002)052[0883:UBAC]2.0.CO;2
McWilliams C., Lurgi M., Montoya J. M., Sauve A., Montoya D. (2019). The stability of multitrophic communities under habitat loss. Nat. Commun. 10, 2322. doi: 10.1038/s41467-019-10370-2
Mendenhall W., Sincich T. (2016). Statistics for Engineering and the Sciences. 6th ed (New York: Pearson).
Mérida-Rivas J. A., Hinojosa-Díaz I. A., Ayala-Barajas R., Barrientos-Villalobos S., Pozo C., Vadame R. (2022). Revision of carpenter bees of the subgenus Neoxylocopa Michener (Hymenoptera: Apidae) from Mexico and Mesoamerica. Zootaxa 5158, 1–67. doi: 10.11646/zootaxa.5158.1.1
Michener C. D., McGinley R. J., Danforth B. N. (1994). The Bee Genera of North and Central America (Hymenoptera: Apoidea) (Washington, DC, USA: Smithsonian Institution Press).
Moreira E. F., Boscolo D., Viana B. F. (2015). Spatial heterogeneity regulates plant-pollinator networks across multiple landscape scales. PloS One 10, e0123628. doi: 10.1371/journal.pone.0123628
Ollerton J., Erenler H., Edwards M., Crockett R. (2014). Pollinator decline: Extinction of aculeate pollinators in Britain and the role of large scale agricultural changes. Science 346. doi: 10.1126/science.1257259
Pinheiro J., Bates D., DebRoy S., Sarkar D., R Core Team (2018). nlme: Linear and Nonlinear Mixed Effects Models. R package version 3.1-131.1. Available online at: https://CRAN.R-project.org/package=nlme (accessed September 2, 2024).
Potts S. G., Biesmeijer J. C., Kremer C., Neumann P., Schweiger O., Kunin W. (2010). Global pollinator declines: trends, impacts and drivers. Trends Ecol. Evol. 25, 345–353. doi: 10.1016/j.tree.2010.01.007
Ramírez S. R., Hernández C., Link A., López-Uribe M. M. (2015). Seasonal cycles, phylogenetic assembly, and functional diversity of orchid bee communities. Ecol. Evol. 5, 1896–1907. doi: 10.1002/ece3.1466
R Core Team (2022). R: A Language and Environment for Statistical Computing (Vienna: R Foundation for Statistical Computing). Available at: https://www.R-project.org/.
Requier F., Leonhardt S. D. (2020). Beyond flowers: including non-floral resources in bee conservation schemes. J. Insect Conserv. 24, 5–16. doi: 10.1007/s10841-019-00206-1
Reynolds V. A., Cunningham S. A., Rader R., Mayfield M. M. (2022). Adjacent crop type impacts potential pollinator communities and their pollination services in remnants of natural vegetation. Diver. Distrib. 28, 1269–1281. doi: 10.1111/ddi.13537
Ricketts T. H. (2004). Tropical forest fragmentstt enhance pollinator activity in nearby coffee crops. Conser. Biol. 18, 1262–1271. doi: 10.1111/j.1523-1739.2004.00227.x
Ricketts T. H., Regetz J., Steffan-Dewenter I., Cunningham S. A., Kremen C., Bogdanski A., et al. (2008). Landscape effects on crop pollination services: are there general patterns? Ecol. Lett. 11, 499–515. doi: 10.1111/j.1461-0248.2008.01157.x
Ruas R., de B., Mara L., Santana Costa L. M., Bered F. (2022). Urbanization driving changes in plant species and communities – A global view. GECCO 38, e02243. doi: 10.1016/j.gecco.2022.e02243
Russel J. (2021). MicEco: Various functions for microbial community data. R package version 0.9.16. Available online at: https://zenodo.org/record/4733747.YcWJqWjMLb0 (accessed February 5, 2024).
Samnegård U., Hambäck P. A., Eardley C., Nemomissa S., Hylander K. (2015). Turnover in bee species composition and functional trait distributions between seasons in a tropical agricultural landscape. Agric. Ecosyst. Environ. 211, 185–194. doi: 10.1016/j.agee.2015.06.010
Saturni T. F., Jaffé R., Metzger J. P. (2016). Landscape structure influences bee community and coffee pollination at different spatial scales. Agric. Ecosyst. Environ. 235, 1–12. doi: 10.1016/j.agee.2016.10.008
Scheper J., Reemer M., van Kats R., Ozinga W. A., van der Linden G. T. J., Schamineé J. H. J. (2014). Museum specimens reveal loss of pollen host plants as key factor driving wild bee decline in The Netherlands. PNAS 11, 17552–17557. doi: 10.1073/pnas.1412973111
Serralta-Batun L. P., Jiménez-Osornio J. J., Meléndez-Ramírez V., Munguía-Rosas M. A. (2024). Taxonomic and functional diversity of bees in traditional agroecosystems and tropical forest patches on the Yucatan Peninsula. Trop. Conserv. Sci. 17. doi: 10.1177/19400829231225428
Shannon C. E., Weaver W. (1949). The Mathematical Theory of Communication (Champaign: University of Illinois Press).
Silva J. L. S., de Oliveira M. T. P., Cruz-Neto O., Tabarelli M., Lopes A. V. (2020). Plant-pollinator interactions in urban ecosystems worldwide: A comprehensive review including research funding and policy actions. Ambio 50, 884–900. doi: 10.1007/s13280-020-01410-z
Simba L. D., Foord S. H., Thébault E., van Veen F. F., Joseph G. S., Seymour C. L. (2018). Indirect interactions between crops and natural vegetation through flower visitors: the importance of temporal as well as spatial spillover. Agric. Ecosyst. Environ. e253, 148–156. doi: 10.1016/j.agee.2017.11.002
Solís-Montero L., Landaverde-González P., Zamora-Gutierrez V., He X. (2023). Editorial: The forgotten pollinators: the importance and conservation of wild pollinators. Front. Sustain. Food Syst. 7. doi: 10.3389/fsufs.2023.1323557
Souza C. S., Murayama P. K., Aoki C., Sigrist M. R., Raizer J., Gross C. L., et al. (2017). Temporal variation in plant–pollinator networks from seasonal tropical environments: Higher specialization when resources are scarce. J. Ecol. 106, 2409–2420. doi: 10.1111/1365-2745.12978
Standley P. C., Steyermark J. A. (1946–1977). (Eds.) Flora of Guatemala: Volumen 1–13 (Chicago, USA: Field Museum of Natural History).
Stewart A. B., Waitayachart P. (2020). Year-round temporal stability of a tropical, urban plant-pollinator network. PloS One 15, e0230490. doi: 10.1371/journal.pone.0230490
Suni S., Hall E., Bahu E., Hayes H. (2022). Urbanization increases floral specialization of pollinators. Ecol. Evol. 12, e8619. doi: 10.1002/ece3.8619
Theodorou P., Herbst S. C., Kahnt B., Landaverde-González P., Baltz L., Osterman J., et al. (2020). Urban fragmentation leads to lower floral diversity, with knock-on impacts on bee biodiversity. Sci. Rep. 10, 21756. doi: 10.1038/s41598-020-78736-x
Thomann M., Imbert E., Devaux C., Cheptou P. O. (2013). Flowering plants under global pollinator decline. Trends Plan Sci. 18, 353–359. doi: 10.1016/j.tplants.2013.04.002
Tsang T. P. N., Amado De Santis A. A., Armas-Quiñonez A. G., Ascher J., Ávila-Gómez E. S., Báldi A., et al. (2024). Land use change consistently reduces α but not β and γ diversity of bees. Global change biology.
Ulyshen M., Urban-Mead K. R., Dorey J. B., Rivers J. W. (2023). Forests are critically important to global pollinator diversity and enhance pollination in adjacent crops. Biol. Rev. 98, 1118–1141. doi: 10.1111/brv.12947
Vergara C. H., Badano E. I. (2009). Pollinator diversity increases fruit production in Mexican coffee plantations: The importance of rustic management systems. Agric. Ecosyst. Environ. 129, 117–123. doi: 10.1016/j.agee.2008.08.001
Keywords: bee flower visitor, bee–plant interaction network, bee generality, semi-natural areas, rainy season
Citation: Casiá-Ajché QB, Escobedo-Kenefic N, Escobar-González D, Cardona E, Mejía-Coroy A, Morales-Siná J, Enríquez E and Landaverde-González P (2024) Unveiling the effects of land use and intra-seasonal variation on bee and plant diversity and their ecological interactions in vegetation surrounding coffee plantations. Front. Bee Sci. 2:1408854. doi: 10.3389/frbee.2024.1408854
Received: 28 March 2024; Accepted: 22 November 2024;
Published: 17 December 2024.
Edited by:
Katja Hogendoorn, University of Adelaide, AustraliaReviewed by:
Connal Eardley, North-West University, South AfricaChristine Coppinger, University College Dublin, Ireland
Copyright © 2024 Casiá-Ajché, Escobedo-Kenefic, Escobar-González, Cardona, Mejía-Coroy, Morales-Siná, Enríquez and Landaverde-González. This is an open-access article distributed under the terms of the Creative Commons Attribution License (CC BY). The use, distribution or reproduction in other forums is permitted, provided the original author(s) and the copyright owner(s) are credited and that the original publication in this journal is cited, in accordance with accepted academic practice. No use, distribution or reproduction is permitted which does not comply with these terms.
*Correspondence: Quebin Bosbely Casiá-Ajché, cWJvc2JlbHlAcHJvZmVzb3IudXNhYy5lZHUuZ3Q=; Patricia Landaverde-González, cGF0cmljaWEubGFuZGF2ZXJkZUB6b29sb2dpZS51bmktaGFsbGUuZGU=
†These authors have contributed equally to this work