- 1Departamento de Tecnologia Farmacêutica, Faculdade de Farmácia, Universidade Federal Fluminense, Niterói, Rio de Janeiro, Brazil
- 2Departamento de Química Orgânica, Instituto de Química, Universidade Federal Fluminense, Outeiro de São João Batista s/n, Campus do Valonguinho, Niterói, Rio de Janeiro, Brazil
Introduction: Itraconazole is a widely used broad-spectrum antifungal drug on a global scale. However, its poor water solubility necessitates the development of advanced formulations for its effective use. Currently, itraconazole is available commercially in capsule form, with pellets containing the active drug. The extraction of itraconazole from these granules is often required when developing new formulations. Extracting active pharmaceutical ingredients (APIs) from pellets is crucial in ensuring precise and consistent dosing when producing pharmaceutical dosage forms. Surprisingly, no existing methods for extracting itraconazole from pellets have been reported thus far.
Objective: Therefore, this study aimed to develop and evaluate an efficient method for extracting this API from pellets.
Methods: Two extraction methods were assessed: conventional Soxhlet extraction and ultrasound-assisted extraction.
Results and Discussion: The results demonstrated that ultrasound-assisted extraction significantly outperformed the conventional method, yielding higher amounts of itraconazole with a high purity level of 96.8%.
1 Introduction
Itraconazole is a broad-spectrum antifungal drug that selectively inhibits the P450-dependent lanosterol C14α-demethylase enzyme, suppressing the synthesis of ergosterol in the fungal cell membrane, which leads to a disruption in its permeability and function (Bossche et al., 1995). This molecule, which belongs to the class of the 1,2,4-triazoles (Figure 1), presents low water solubility and low gastrointestinal absorption when administered orally, being categorized as a BCS II model drug in the Biopharmaceutics Classification System (BCS). This issue is easily minimized by the formulation of the active pharmaceutical ingredient (API) as pellets (or beads); in such systems, the base pellet contains sucrose or cellulose, which is then coated with the active drug and polymers such as hydroxypropyl methylcellulose (HPMC) being added to improve dissolution and bioavailability (Sardana et al., 2019). Pellets have gained considerable recognition as a versatile drug delivery system due to their numerous advantages, including controlled release, enhanced bioavailability, and simplified formulation (Chaerunisaa et al., 2020).
In this context, extracting active pharmaceutical ingredients from pellets is vital in producing pharmaceutical dosage forms, as it guarantees precise and consistent dosing. Furthermore, it enables accurate characterization, quantification, and quality control of the API, facilitating efficient compliance with regulatory guidelines and standards. Notably, using effective extraction techniques can increase API recovery, reduce waste, and maximize the utilization of valuable raw materials (Kulkarni et al., 1995). The development of methods for extracting APIs from pellets also plays a crucial role in the research field since the development of new formulations takes up tremendous amounts of API, which is not always available in its pure form.
In light of this, developing extraction methods for itraconazole from pellets hold significant importance, particularly considering the lack of reported approaches thus far. While several groups have successfully devised extraction methods for APIs from solid dosage-form drugs, it is worth noting that specific methods tailored to itraconazole are currently lacking in the literature (Hsieh et al., 2017). Lee and co-workers, for instance, reported the use of solid-liquid extraction, filtration and crystallization to obtain high-purity acetaminophen, tetracycline and ibuprofen from unused tablets and capsules (Pratama et al., 2020), while Ventura and co-workers described the use of ionic liquids to extract ibuprofen from pharmaceutical wastes (Silva et al., 2016).
Another promising method for extracting APIs from pharmaceutical formulations, which has yet to be fully explored, is using ultrasound-assisted approaches. These methods show great potential for enhancing extraction efficiency and improving overall extraction yields. However, their application in this context has received limited attention and investigation thus far. Ultrasound-assisted processes are essential from a sustainable chemistry point of view and are very useful for accelerating chemical reactions and improving the extraction rate of analytes from diverse matrices (Carreira-Casais et al., 2021; Kumar et al., 2021; Yusoff et al., 2022). The main advantages of ultrasound-assisted extraction include high efficiency in short times, high reproducibility, low energy consumption, and significant reduction in solvent use (Pingret et al., 2013). This approach is reportedly superior to conventional Soxhlet extraction, grinding or Clevenger distillation, and may even be associated with those if required (Chemat et al., 2017).
Considering all these concepts, this study focuses on developing an efficient method for extracting itraconazole from pellets while ensuring the integrity of the extracted API through comprehensive characterization. Both Soxhlet and ultrasound-assisted extraction approaches were employed in this research, resulting in exceptional yields and high purity of the API.
2 Material and methods
2.1 General methods
Itraconazole pellets (Metrochem API, Hyder-abad, Telangana, India) were donated by Valdequimica Produtos Quimicos Ltd. (Sao Paulo, SP, Brazil). Itraconazole standards were purchased from Merck. Acetonitrile (HPLC grade) was purchased from J.T. Baker Inc. (Phillipsburg, NJ). All the chemicals and solvents were of reagent grade and used without further purification. For the analytical evaluation, all solutions were prepared using ultra-pure water obtained from a Milli-Q Water Millipore purification system (Burlington, MA, United States). The nuclear magnetic resonance.
(NMR) experiments were performed on a 500 MHz spectrometer (Varian VNMR Instruments) using deuterated chloroform as solvent and tetramethylsilane as an internal standard. The Fourier-Transform Infrared Spectroscopy spectra were acquired on a Varian FT-IR 660 spectrometer in the 600–4,000 cm−1 range with 20 scans and 2 cm−1 resolution. High-resolution mass spectra (HRMS) were recorded on a Q-TOF (Bruker, Compact) system; HRMS studies were carried out in positive electrospray ionization (ESI) mode, and the samples were directly infused at a concentration of 200 µg.mL−1 in methanol, using a 10–15 V cone voltage and 3000 V capillary voltage. The chromatographic identification of itraconazole was performed on a Shimadzu HPLC system equipped with a diode array detector (DAD). The analysis was carried out using a C18 analytical column (Fortis®; 150 mm × 4.6 mm, 5 µm) at 25°C with a mobile phase consisting of a mixture of acetonitrile and phosphate-buffered saline 0.05 mol L−1 (pH 8.0 with ammonium hydroxide 1 mol L−1) in the ratio 60:40 (v/v), respectively, at a flow rate of 1.0 mL.min−1. The injection volume was 20 μL, and the detection was carried out at 261 nm (Garcia Ferreira et al., 2019).
2.2 Optimization of the extraction of itraconazole from pellets
Aiming to compare yield and purity, two different extraction methods, namely ultrasound-assisted extraction and Soxhlet, were employed to extract itraconazole from pellets. Dichloromethane was used as a solvent in both cases, and all the experiments were carried out in triplicate, with the results expressed as means.
2.2.1 Ultrasound-assisted extraction
Itraconazole pellets (5 g) were mixed with dichloromethane (250 mL) and submitted to an ultrasonic bath (25 kHz, 100 W) (UNIQUE, Brazil) for 30 min. The remaining solid fraction was separated using vacuum filtration, and the filtrate was concentrated using a rotatory evaporator.
2.2.2 Soxhlet extraction
Dichloromethane (250 mL) was added to a round bottom flask coupled to a Soxhlet extractor containing itraconazole pellets (5 g) placed inside a paper thimble. The extraction was carried out for 1 h, and the mixture containing the extract was concentrated in a rotatory evaporator.
2.3 Itraconazole purification
The extracted itraconazole was purified by employing the recrystallization technique. Initially, several solvents were evaluated: ethanol, ethyl acetate, hexane/dichloromethane (9:1 v/v), petroleum ether/dichloromethane (9:1 v/v). To accomplish that, an appropriate amount of the drug was dissolved in a particular solvent volume at 40.0 ± 0.5°C. Gravity filtration was performed to eliminate insoluble impurities, and the resultant filtrate was collected. Subsequently, the solution was carefully transferred to an ice bath to facilitate the crystallization of itraconazole. The experiments were conducted in triplicate to ensure accuracy and reliability. Finally, the extraction yield of itraconazole was determined by employing the following formula.
The obtained solid was then characterized using the 1H-NMR, FT-IR, HRMS and HPLC techniques.
3 Results and discussion
3.1 Evaluation of methods for the extraction of itraconazole from pellets
The extraction of itraconazole from commercial pellets was carried out by studying the efficiency of the hot extraction procedure carried out in a Soxhlet apparatus and by sonication in an ultrasonic bath. As shown in Table 1, the ultrasound-assisted approach was considerably superior to the Soxhlet approach, furnishing a 73.65% yield after recrystallization (vs. 31.84% yield in the Soxhlet extraction), with a standard deviation of less than 1% (n = 3). It is important to highlight that the ultrasound-assisted extraction was conducted at room temperature for 30 min, while the Soxhlet extraction took 1 h under heating to furnish virtually half the yield. The high efficiency of the ultrasound-assisted extraction can be attributed to cavitation and abrupt collapse of the bubbles in the solvent with the release of energy over the surface of the pellets, locally increasing the temperature and pressure; this turbulence produced by the cavitation phenomena leads to the fragmentation of the solid and improved penetration of the solvent into the fragments of the pellets (Luque-García and Luque de Castro, 2004; Porevsky et al., 2014).
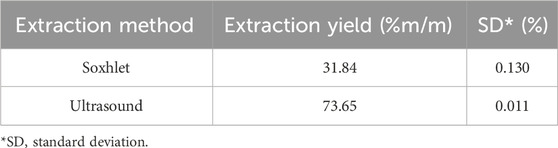
Table 1. Evaluation of Soxhlet and ultrasound-assisted methods for extracting itraconazole from commercial pellets (n = 3).
3.2 Evaluation of parameters for the recrystallization of itraconazole
Recrystallization is one of the most essential techniques for purifying nonvolatile organic solids. A suitable solvent for recrystallization should dissolve the analyte (but not its impurities) at high temperatures but not at room temperature. Thus, we carried out solubility tests for itraconazole in various solvents (Table 2), and the samples were classified as either soluble or insoluble.
As shown in Table 2, itraconazole was insoluble in ethanol and ethyl acetate at 70°C, and even a mixture of chloroform and methanol did not lead to the desired outcome, with the resulting mixtures presenting a highly cloudy aspect. On the other hand, when a hexane/dichloromethane (9:1) mixture was employed, it was possible to notice that the material was slightly more soluble since it presented a less cloudy aspect. To our delight, a petroleum ether/dichloromethane mixture led to the complete solubility of itraconazole at 70°C, with a clear transparent solution being observed.
3.3 Evaluation of the purity of the extracted itraconazole
After the ultrasound-assisted extraction and recrystallization steps, the obtained itraconazole sample was submitted for full characterization to determine whether the API remained structurally unaltered and its purity. The identification and confirmation of the purity of itraconazole were assessed using 1H-NMR spectroscopy, Fourier-transform infrared spectroscopy, high-resolution mass spectrometry and HPLC. The 1H-NMR spectrum of the itraconazole obtained from pellets is shown in Figure 2.
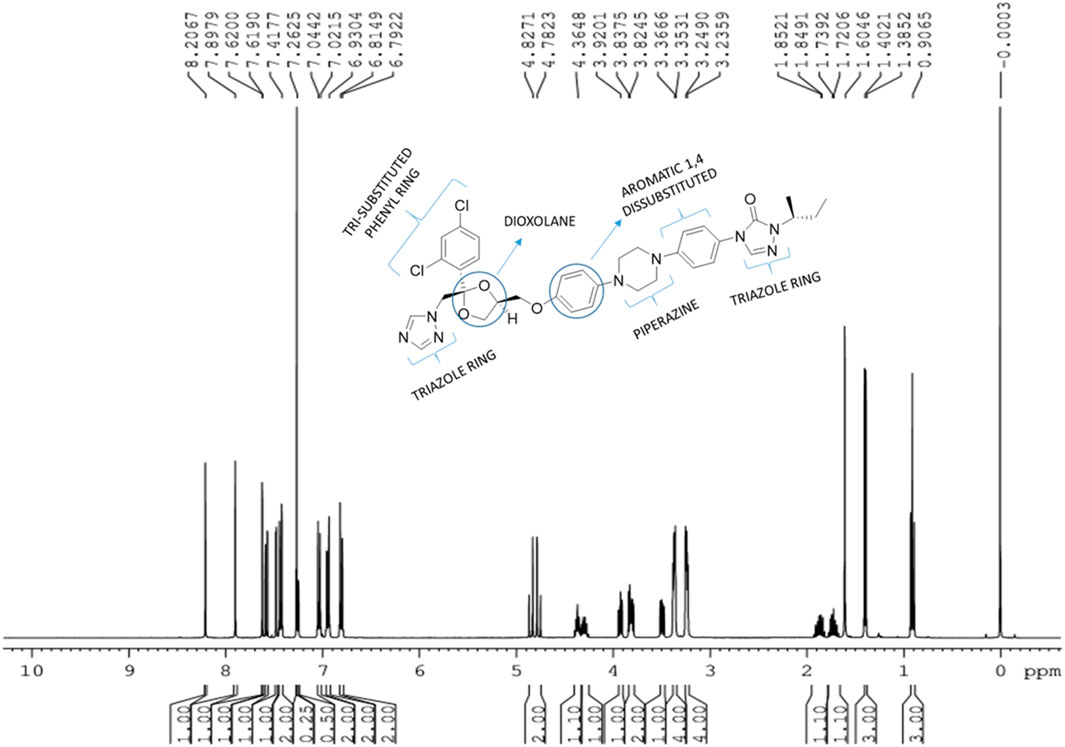
Figure 2. 1H-NMR spectrum (500 MHz, CDCl3) of itraconazole obtained from commercial pellets using the ultrasound-assisted extraction method.
The analysis of the 1H-NMR spectra showed that the extracted itraconazole signals corresponded to those of the standard, confirming the viability of the newly developed process for extracting the API from commercial pellets. In the aliphatic region of the 1H-NMR spectrum, two signals, a triplet and a doublet at 0.90 and 1.38 ppm, respectively, both integrating to 3 protons, were assigned to terminal methyl groups. A doublet of multiplets integrating to 2 protons was observed at 1.61–1.85 ppm, corresponding to the methylene group between the dioxolane ring and the phenyl ether moieties. Another set of multiplets at 3.23–3.24 and 3.35–3.36 ppm, integrating to 4 protons, was attributed to the protons in the piperazine portion of the structure. Multiplets at 3.82–3.85, 3.84–3.90, and 4.27–4.36 ppm, integrating to 2, 2 and 1 proton respectively, were assigned to the resonance of the dioxolane ring protons. The signal corresponding to the protons of the methylene group linking the triazole and dioxolane rings appeared at 4.78–4.82 ppm, integrating to 2 protons. In the aromatic region, multiplets were observed at 6.73–6.87 and 6.93–7.39 ppm, both integrating to 4 protons, corresponding to the protons of the two para-substituted aryl rings. Additionally, two multiplets at 7.17–7.19 and 7.39–7.40 ppm, along with a doublet at 7.41–7.61 ppm, each integrating to 1 proton, were assigned to the tri-substituted phenyl ring. Finally, the three singlets at 7.62, 7.89, and 8.20 ppm are characteristic of the protons of the triazole ring.
The infrared spectrum of the sample displayed the characteristic bands of itraconazole in 3,130, 3,068, 2,964, 2,823, 1,698, 1,585, 1,551, 1,510, 1,451, 1,381, 1,228, 1,184, 1,139, 1,043, 976, 944, 824, and 736 cm−1 (for more details, see Supplementary Figure S1; Supplementary Table S1 in the SI) (Brittain, 2009). The identity of itraconazole was further confirmed by the HRMS results (Supplementary Figure S2). In the mass spectrum, the ions [M+2H]++ and [M+H]+ were observed at 353.1278 and 705.2489 m/z, respectively.
Subsequently, the HPLC-DAD technique was selected as an analytical tool to assess the purity of the itraconazole extracted from commercial pellets. A peak with a retention time of 14.9 min was observed in the obtained chromatogram, corresponding to that of the itraconazole standard (Figure 3). The purity of the extracted itraconazole, as determined by HPLC, was 96.8%, while the peak purity was determined to be 0.082.
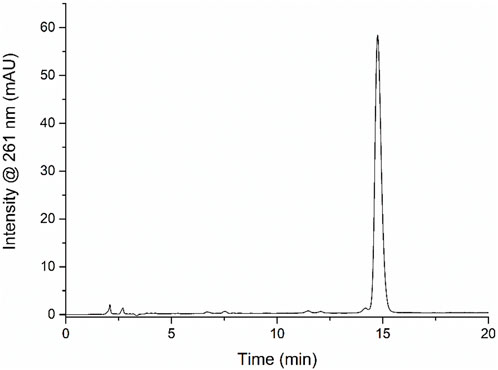
Figure 3. Chromatogram obtained from the analysis itraconazole extracted from commercial pellets at 261 nm. A prominent peak is observed at a retention time of 14.9 min, corresponding to the itraconazole standard. The HPLC-DAD analysis confirmed the purity of the extracted itraconazole to be 96.8%.
4 Conclusion
This study introduces a novel, efficient approach for extracting the active pharmaceutical ingredient (API), itraconazole, from commercial pellets. Two extraction techniques, conventional Soxhlet extraction and ultrasound-assisted extraction, were compared, with the latter showing the most favorable extraction yield. While the ultrasound-assisted method led to a 74% yield in 30 min at room temperature, the Soxhlet method required 1 h under heating to furnish approximately 32% itraconazole yield. To ensure the quality of the isolated drug, comprehensive characterization was performed using spectroscopic techniques and High-Performance Liquid Chromatography (HPLC). The results confirmed that the extracted itraconazole had a high purity level of 96.8% and that the API remained structurally intact throughout the extraction process.
The application of ultrasound irradiation for itraconazole extraction from pellets demonstrated significant potential, which could benefit pharmaceutical manufacturing and development. The ultrasound-assisted extraction described in this work was highly efficient and considerably more environmentally sustainable than traditional methods, as it required shorter reaction times. Despite the numerous advantages, it is important to acknowledge the limitations and challenges associated with using ultrasound-assisted techniques to extract APIs from pharmaceutical formulations, such as scalability. Besides that, using greener solvents is another point that remains to be addressed in this context. As research and development in this field progress, further optimization and standardization of ultrasound-assisted extraction protocols will enable its broader implementation in the pharmaceutical industry, ultimately leading to more efficient and sustainable drug manufacturing processes.
Data availability statement
The raw data supporting the conclusions of this article will be made available by the authors, without undue reservation.
Author contributions
PF: Conceptualization, Data curation, Formal Analysis, Investigation, Methodology, Validation, Visualization, Writing–original draft, Writing–review and editing. CL: Conceptualization, Formal Analysis, Methodology, Supervision, Visualization, Writing–original draft, Writing–review and editing. Md: Data curation, Formal Analysis, Investigation, Supervision, Visualization, Writing–original draft, Writing–review and editing. Fd: Funding acquisition, Investigation, Methodology, Project administration, Resources, Supervision, Visualization, Writing–review and editing. DF: Conceptualization, Funding acquisition, Investigation, Methodology, Project administration, Resources, Supervision, Visualization, Writing–review and editing. VF: Conceptualization, Funding acquisition, Investigation, Methodology, Project administration, Resources, Supervision, Visualization, Writing–review and editing.
Funding
The author(s) declare that financial support was received for the research, authorship, and/or publication of this article. P.G. Ferreira gratefully acknowledges the support of Fundação Carlos Chagas Filho de Amparo à Pesquisa do Estado do Rio de Janeiro (FAPERJ) for providing the scholarship. The authors would also like to express their gratitude to FAPERJ for funding the research project.
Conflict of interest
The authors declare that the research was conducted in the absence of any commercial or financial relationships that could be construed as a potential conflict of interest.
Publisher’s note
All claims expressed in this article are solely those of the authors and do not necessarily represent those of their affiliated organizations, or those of the publisher, the editors and the reviewers. Any product that may be evaluated in this article, or claim that may be made by its manufacturer, is not guaranteed or endorsed by the publisher.
Supplementary material
The Supplementary Material for this article can be found online at: https://www.frontiersin.org/articles/10.3389/frans.2024.1498404/full#supplementary-material
References
Bossche, H. V., Koymans, L., and Moereels, H. (1995). P450 inhibitors of use in medical treatment: focus on mechanisms of action. Pharmacol. Ther. 67, 79–100. doi:10.1016/0163-7258(95)00011-5
Brittain, H. G. (2009). Vibrational spectroscopic studies of cocrystals and salts. 1. The Benzamide−Benzoic acid system. Cryst. Growth Des. 9, 2492–2499. doi:10.1021/cg801397t
Carreira-Casais, A., Otero, P., Garcia-Perez, P., Garcia-Oliveira, P., Pereira, A. G., Carpena, M., et al. (2021). Benefits and drawbacks of ultrasound-assisted extraction for the recovery of bioactive compounds from marine algae. Int. J. Environ. Res. Public Health 18, 9153. doi:10.3390/ijerph18179153
Chaerunisaa, A. Y., Ali, R., Körber, M., and Bodmeier, R. (2020). Quantification of porogen effect on the drug release from single- and multi-layered ethylcellulose coated pellets containing single or combined drugs. Int. J. Pharm. 577, 119050. doi:10.1016/j.ijpharm.2020.119050
Chemat, F., Rombaut, N., Sicaire, A.-G., Meullemiestre, A., Fabiano-Tixier, A.-S., and Abert-Vian, M. (2017). Ultrasound assisted extraction of food and natural products. Mechanisms, techniques, combinations, protocols and applications. A review. Ultrason. Sonochem. 34, 540–560. doi:10.1016/j.ultsonch.2016.06.035
Garcia Ferreira, P., de Souza Lima, C., Noronha, L. L., de Moraes, M. C., Silva, F. de C. da, Lifsitch Viçosa, A., et al. (2019). Development of a method for the quantification of clotrimazole and itraconazole and study of their stability in a new microemulsion for the treatment of sporotrichosis. Molecules 24, 2333. doi:10.3390/molecules24122333
Hsieh, D. S., Lindrud, M., Lu, X., Zordan, C., Tang, L., and Davies, M. (2017). A process for active pharmaceutical ingredient recovery from tablets using green engineering Technology. Org. Process Res. Dev. 21, 1272–1285. doi:10.1021/acs.oprd.7b00146
Kulkarni, S. B., Betageri, G. V., and Singh, M. (1995). Factors affecting microencapsulation of drugs in liposomes. J. Microencapsul. 12, 229–246. doi:10.3109/02652049509010292
Kumar, K., Srivastav, S., and Sharanagat, V. S. (2021). Ultrasound assisted extraction (UAE) of bioactive compounds from fruit and vegetable processing by-products: a review. Ultrason. Sonochem. 70, 105325. doi:10.1016/j.ultsonch.2020.105325
Luque-García, J. L., and Luque de Castro, M. D. (2004). Ultrasound-assisted Soxhlet extraction: an expeditive approach for solid sample treatment. Application to the extraction of total fat from oleaginous seeds. J. Chromatogr. A 1034, 237–242. doi:10.1016/j.chroma.2004.02.020
Pingret, D., Fabiano-Tixier, A.-S., and Chemat, F. (2013). “Ultrassound-assisted extraction,” in Natural product extraction: principles and applications. Editors M. A. Rostagno, and J. M. Prado (Cambridge: The Royal Society of Chemistry), 89–112.
Porevsky, P. A., Ruiz, H. G., and Garciadiego, L. H. (2014). Comparison of Soxhlet extraction, ultrasonic bath and focused microwave extraction techniques for the simultaneous extraction of PAH ́s and pesticides from sediment samples. Sci. Chromatogr. 6, 1–15. doi:10.4322/sc.2014.026
Pratama, D. E., Hsieh, W.-C., Elmaamoun, A., Lee, H. L., and Lee, T. (2020). Recovery of active pharmaceutical ingredients from unused solid dosage-form drugs. ACS omega 5, 29147–29157. doi:10.1021/acsomega.0c03878
Sardana, K., Khurana, A., and Gupta, A. (2019). Parameters that determine dissolution and efficacy of itraconazole and its relevance to recalcitrant dermatophytoses. Expert Rev. Clin. Pharmacol. 12, 443–452. doi:10.1080/17512433.2019.1604218
Silva, F. A., Caban, M., Stepnowski, P., Coutinho, J. A. P., and Ventura, S. P. M. (2016). Recovery of ibuprofen from pharmaceutical wastes using ionic liquids. Green Chem. 18, 3749–3757. doi:10.1039/C6GC00261G
Keywords: itraconazole, pellets, ultrasonic, extraction, antifungal drug
Citation: Ferreira PG, Lima CGS, de Moraes MC, da Silva FdC, Futuro DO and Ferreira VF (2024) Ultrasound-assisted extraction of itraconazole from pellets: an efficient approach for the recovery of active pharmaceutical compounds from solid dosage forms. Front. Anal. Sci. 4:1498404. doi: 10.3389/frans.2024.1498404
Received: 18 September 2024; Accepted: 21 October 2024;
Published: 30 October 2024.
Edited by:
Bruno Alves Rocha, University of São Paulo, BrazilReviewed by:
Leandro Augusto Calixto, Federal University of São Paulo, BrazilDinh Binh Chu, Hanoi University of Science and Technology, Vietnam
Copyright © 2024 Ferreira, Lima, de Moraes, da Silva, Futuro and Ferreira. This is an open-access article distributed under the terms of the Creative Commons Attribution License (CC BY). The use, distribution or reproduction in other forums is permitted, provided the original author(s) and the copyright owner(s) are credited and that the original publication in this journal is cited, in accordance with accepted academic practice. No use, distribution or reproduction is permitted which does not comply with these terms.
*Correspondence: Vitor F. Ferreira, vitorferreira@id.uff.br; Patricia G. Ferreira, patricia.pharma@yahoo.com.br