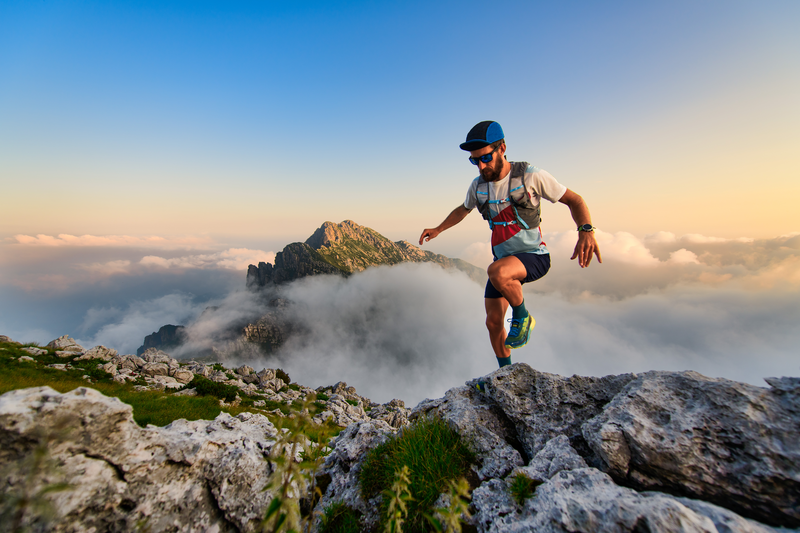
95% of researchers rate our articles as excellent or good
Learn more about the work of our research integrity team to safeguard the quality of each article we publish.
Find out more
REVIEW article
Front. Anal. Sci. , 25 September 2023
Sec. Omics
Volume 3 - 2023 | https://doi.org/10.3389/frans.2023.1258558
This article is part of the Research Topic Thought Leaders in Analytical Science Research View all 12 articles
Ratiometric strategy are an invaluable method that helps to detect and quantify analytes. This approach relies on measuring changes in the ratio of two or more signals to improve the accuracy and sensitivity of the results. Ratiometric strategies are widely used in a variety of fields including biomedical, environmental monitoring and food safety. It is particularly popular when traditional single-signal based detection methods are not feasible, especially when interfering substances severely affect the detection. In addition, ratiometric methods have the potential to improve the accuracy and reliability of analyte detection, leading to better results in a variety of complex environments. The article provides a comprehensive review of ratiometric strategy, focusing on ratiometric fluorescent nanoprobes for the visual detection of analytes. This paper also discusses the design of ratiometric two-photon fluorescent probes for biomedical imaging, the synthesis of ratiometric surface-enhanced Raman scattering nanoprobes for the imaging of intracellular analytes, the development of ratiometric molecularly imprinted electrochemical sensors for detection of electroactive species, and the use of isotopically-labeled internal standards in matrix-assisted laser desorption/ionization for ratiometric analysis. The article not only discusses each technique in detail, including its principles, advantages, potential applications, and limitations, but also highlights recent advances in each method and possible future directions.
A ratiometric strategy is a “self-calibration” method by measuring the analyte-induced change in the ratio of two or more signals in a sensing element (Huang et al., 2018; Jin et al., 2021; Wei et al., 2022). In contrast to single-signal detection, this method has built-in corrections for fluctuations in instrument operation, interference from the sample matrix, variations in the microenvironment around the probe, and changes in the concentration of the probe. Due to these advantages, a ratiometric strategy is extensively implemented in a wide range of analytical techniques, including fluorescence, Raman, absorbance, electrochemistry, and mass spectrometry (Ali et al., 2014; Ke et al., 2015; Li et al., 2015; Peng et al., 2016; Chen et al., 2017; Parrilla et al., 2017; Vargas et al., 2019; Vyas et al., 2019; Njoko et al., 2020; Madhu and Tseng, 2021; Spring et al., 2021; Zhu et al., 2021). Two or more fluorescent dyes and nanomaterials are typically integrated into a ratiometric fluorescence sensing analytical platform. Each probe emits a light signal at a unique wavelength to the analyte. The platform measures the intensity ratio of the emitted light to determine the analyte concentration. Similarly, a ratiometric strategy using two independent wavenumbers in Raman spectroscopy can be applied to determine the concentration of an analyte. Absorbance spectroscopy also employs a ratiometric strategy, where the concentration of an analyte is calculated from the ratio of two absorbance readings. Also, amperometry and potentiometry are electrochemical methods that employ a ratiometric strategy to quantify an analyte. As a target analyte is added, more than two currents and potentials are typically monitored. Another analytical method that benefits from a ratiometric strategy is mass spectrometry. In this method, isotopically labeled standards are used as internal references to provide reliable and accurate quantitative measurements of analytes. The researchers can obtain reliable and accurate quantitative results in various analytical fields by employing these ratiometric analytical techniques. A ratiometric strategy has gained a foothold in various analytical fields, especially for quantifying analytes in a complex sample. In environmental monitoring, ratiometric fluorescence sensors are ideally suited for detecting metal ions and organic contaminants in water samples, making them a powerful tool for monitoring and managing water quality. These sensors are quick and easy to use, with results typically available within minutes. In addition, they are cost-effective and can detect a wide range of contaminants with high accuracy and sensitivity (HeeáLee and SeungáKim, 2015). Moreover, in vivo and in vitro studies, ratiometric phosphorescent sensors are well-developed for monitoring microenvironmental changes and imaging target analytes in living cells and animals. These sensors are also exploited to monitor dynamic events, such as the kinetics of enzyme reactions in living organisms (Jin et al., 2021). In biomedical research, a ratiometric Raman technique integrated with a microscopy-related system is attractive for investigating biomolecular structures at the cellular and sub-cellular levels (Kumar et al., 2016). By adopting the ratiometric Raman technique, researchers can improve the accuracy and precision of measurements compared to quantitative methods based on absolute band Raman intensities. This technique enables the label-free biomolecular fingerprinting of biological samples in their native state. Consequently, a ratiometric strategy has become an indispensable component in a wide range of analytical methods, including fluorescence, Raman, absorbance, electrochemistry, and mass spectrometry, ensuring trustworthy and precise quantitative analysis across different scientific domains.
This review explores a variety of ratiometric measurements in conjunction with various analytical techniques, including precise analytical detection using ratiometric fluorescent nanoprobes and biomedical imaging using two-photon fluorescent probes. Moreover, ratiometric surface-enhanced Raman scattering (SERS) nanoprobes provide Raman-sensitive molecules as a reference signal in the silent cell region, enabling Raman imaging of target analytes in living cells. In the silent region, most intracellular biomolecules are devoid of Raman scattering signals. However, functional groups such as alkyne, azide, and nitrile, as well as substituting C-H for C-D, show characteristic Raman bands in this silent region, which is a fortunate exception. It also highlights how to design ratiometric molecularly imprinted electrochemical sensors and utilize isotope-labeled internal standards to achieve ratiometric analysis in matrix-assisted laser desorption/ionization time-of-flight mass spectrometry (MALDI-TOF-MS). Each technique’s advantages and applications are summarized, providing valuable insights for advancing ratiometric sensing across different fields.
A ratiometric strategy offers several advantages over other analytical methods: 1) Improved accuracy and precision: A ratiometric strategy eliminates many noise sources, such as variations in instrument response or the influence of the sample matrix, which can affect the absolute value of a single signal. By measuring the ratio of two or more signals, ratio sensing can improve the accuracy and precision of measurements (Bigdeli et al., 2019). 2) Increased sensitivity: A ratiometric strategy can improve the reproducibility of measurements by measuring the ratio of two or more signals, thereby increasing sensitivity and lowering the detection limit (Huang et al., 2018; Wei et al., 2022). 3) Excellent selectivitatiometric strategy achieves selectivity by employing multiple signals with distinct responses to the target analyte. By measuring the ratio of these signals, ratiometric sensing ensures selective detection, even in complex sample matrices (Abbasi-Moayed et al., 2018; Gan et al., 2022; Shan et al., 2022; Zhu et al., 2022). 4) Real-time monitoring: By utilizing multiple signals with distinct responses to the target analyte, a ratiometric sensing enables continuous monitoring without sample processing or time-consuming analysis. This real-time capability allows for swift and dynamic measurements in various research applications (Liu et al., 2019; Qu et al., 2020; Kumaravel et al., 2021; Han et al., 2022). 5) Versatility: Ratiometric methods, incorporating various detection techniques such as fluorescence, absorbance, electrochemistry, Raman scattering, and mass spectrometry, emerge as a powerful tool for achieving accurate and sensitive measurements in diverse research areas. With its broad applicability, a ratiometric sensing proves valuable in a variety of analytical chemistry applications, including environmental monitoring, biomedical analysis, and materials science (Bigdeli et al., 2019; Jin et al., 2021; Wei et al., 2022; Šebela, 2023).
Ratiometric fluorescent nanoprobes contain two or more fluorescent components that emit light at different wavelengths, allowing ratiometric sensing and imaging. They has become an ideal tool for visualizing, detecting, and quantifying a wide range of analytes, including ions, molecules, and biomolecules (Kumar et al., 2020; Chuang et al., 2022; Tseng et al., 2022; Luo et al., 2023). The design of ratiometric fluorescent nanoprobes involves carefully selecting fluorescent components, including dyes, quantum dots, upconversion nanoparticles, and metal clusters. These components should have different emission spectra and similar excitation spectra to ensure accurate ratiometric sensing. Nanoprobes should also be designed to target specific analytes by chemical modification or using biomolecules, such as antibodies, inducers, or peptides as recognition elements. For naked-eye detection, nanoprobes should have a high contrast between the two emission channels for easy visualization with the naked eye (Kumar et al., 2020; Tseng et al., 2022; Luo et al., 2023). A key aspect of designing ratiometric fluorescent sensors for visual detection is the thoughtful selection of fluorophores with distinct emission spectra. This choice ensures that the emission wavelengths of the two fluorophores are easily distinguishable to the naked eye. The greater the contrast in emission wavelengths, the more pronounced the color change will be, allowing direct differentiation between different states or analyte concentrations. This approach also applies to ratiometric absorption sensors. Firstly, the interaction between the chromophore and the target analyte results in a distinctive spectral wavelength or absorbance change, producing a noticeable color change. This uniqueness is essential for clear visual discrimination between an analyte and possibly interfering compounds. The human naked eye can perceive alterations in a given ratio through noticeable changes in color, intensity, or brightness. These ratios are commonly assessed using various sensing elements, which can be affixed to a solid support, such as lateral flow immunochromatographic assays, loop-mediated isothermal amplification (LAMP) assays, aptamer sensors, smartphone-based assays, and paper sensors. Alternatively, the sensing element can be incorporated into a solution, enabling visual inspection. Naked-eye detection utilizing ratio sensors offers numerous advantages, including simplicity in operation, the convenience of visual endpoint detection, rapidity in obtaining results, specificity in target identification, and cost-effectiveness. Moreover, these sensor-based approaches do not necessitate specialized equipment or highly trained personnel. However, naked-eye testing using ratio sensors lacks precise quantitative information. Although naked eye readings are instinctive, accurate results are not always possible because the interpretation of color changes can be subjective. In addition, the detection limit of this method may not match the detection limit achieved by instrumental techniques, which may limit sensitivity. Among fluorescence-based assays, naked-eye detection can be achieved by solution-based assays, paper-based assays, and lateral flow immunochromatographic assays (LFAs) (Figure 1A). Solution-based assays involve probes with chromophores and fluorophores that cause changes in absorption spectra and fluorescence emission, respectively, upon binding to analytes. On the other hand, paper-based assays use chromatography paper as an analytical detection platform, where probes attached to chromophores and fluorophores are immobilized on the chromatography paper. After the sample is dispensed, the porous media chromatography paper is able to guide the sample flow through capillary action, and visual detection can be achieved by observing changes in the color of the probes on the paper strip or changes in the fluorescence intensity of the probes. LFAs are designed to detect analytes on paper through immunoassay principles. In the first step, the analyte-specific antibody is attached to the chromophore or fluorophore and then coated onto the conjugate pads. The conjugate pad is then placed on a nitrocellulose membrane containing the test and control lines. The sample flows through the membrane by capillary action. If an analyte is present, it binds to the conjugated antibody or antigen, producing a visible color change on the test line. The secondary antibody on the control line is then connected to the unreacted antibody to ensure correct results.
FIGURE 1. (A) Diverse forms of naked-eye detection in fluorescence-based assays. (B) Fundamental guidelines for building ratiometric two-photon fluorescent probes based on different ratiometric signal patterns and sensing mechanisms. (C) Creation of a ratiometric SERS nanoprobe for detecting target analytes using an internal standard in a region without biomolecule interference. (D) Fabrication of the MIP electrochemical sensor with an internal standard for ratiometric detection of target analytes.
Here are some examples of how to detect environmental pollutants and biomarkers using the naked eye: in solution, on paper, and through lateral flow immunochromatography. Tseng et al. synthesized gold nanoclusters-loaded lysozyme nanoparticles through the reaction of aggregated lysozyme-stabilized gold nanoclusters with glutaraldehyde. The gold nanoclusters and lysozyme nanoparticles in the nanocomposites emitted blue and red fluorescence, respectively, under a single excited wavelength (Tseng et al., 2022). The presence of cyanide etched gold nanoclusters in the dual-emission nanocomposites, inhibiting their Förster resonance energy transfer (FRET) from lysozyme nanoparticles to gold nanoclusters. As a result, the dual-emission nanocomposites were capable of ratiomatrically detect cyanide within a linear range of 3–100 μM. They demonstrated that the proposed nanocomposites provided exceptional selectivity for cyanide and successfully quantified cyanide levels in tap water and soil samples. Furthermore, the nanocomposites were exploited to monitor the release of hydrogen cyanide from cyanogenic glycoside-containing foods. These findings highlight the probe’s potential for diverse environmental analysis and food safety applications.
In addition, a ratiometric fluorescence sensor was developed for the sensitive and selective detection of carbaryl in Iranian apples through 1) the NaOH-mediated hydrolysis of carbaryl to blue-emitting 1-naphthol and 2) the mixing of 1-nanphthol and yellow-emitting cadmium telluride quantum dots (CdTe QDs) (Shahdost-Fard et al., 2021). Using CdTe QDs with yellow fluorescence at 580 nm as a reference, the fluorescence colour changed from yellow to blue as the concentration of carbaryl increased. This ratiometric fluorescent sensor enabled enzyme-free naked-eye detection of carbaryl with a limit of detection (LOD) of 0.12 ng/mL, which is lower than the maximum residue limits (MRLs) specified in the EU Pesticides Database and the USDA Foreign Agricultural Service MRL Database. For example, the MRL for chlorpyrifos in apples is 0.01 mg/kg in the US and 0.05 mg/kg in the EU. The effectiveness of the sensor was confirmed by comparison with high-performance liquid chromatography analysis, demonstrating its potential for rapid and reliable detection of other targets in food safety applications.
Li et al. utilized the mixing of blue- and red-emitting carbon dots for the naked-eye detection of amoxicillin (Li et al., 2023). Hydrogen bonding between amoxicillin and blue-emitting carbon dots led to an increase in the intensity of their blue fluorescence and a change in the color of the solution mixing the two carbon dots from red to blue. A portable hand-held needle sensing device was designed for the direct visual detection of color change of two carbon dot mixtures under UV irradiation in response to amoxicillin stimulation. A smartphone-based illumination device was also fabricated to allow fluorescence imaging of two carbon-dot mixtures after the addition of amoxicillin, which was then quantified based on blue channel/red channel values.
Recently, Miao et al. reported a triple-emission fluorescence sensor consisting of 3-aminophenylboronic acid-derived carbon dots and ovalbumin-stabilized gold nanoclusters for the detection of tetracycline (Miao et al., 2023). The two nanomaterials were hybridized by interacting the cis-diol bond of ovalbumin with the borate group of carbon dots. Tetracycline not only enhanced the red fluorescence of gold nanoclusters through the restriction of their rotational motion but also quenched the blue fluorescence of carbon dots through the inner filter effect process. When the concentration of tetracycline varies between 0.5 and 40 μM, the fluorescence of the sensor changes from blue-violet to yellow-green under UV light; this color change is easy to detect with the naked eye. The proposed hybrid sensor demonstrated the successful determination of tetracycline in real water samples.
Wang et al. described a highly sensitive ratiometric fluorescent lateral flow immunoassay strip for the rapid and accurate detection of cardiac-type fatty acid binding protein, a biomarker associated with acute myocardial infarction (Wang et al., 2021). Fluorescent silica nanoparticles containing gold nanoparticles and red-emitting CdSe/CdS/ZnS quantum dots, along with anti-FABP 10E1 antibodies, were incorporated into the conjugate pad of the testing paper. Simultaneously, green-emitting CdZnSe/CdS/ZnS quantum dots linked to anti-FABP 28 antibodies were affixed in a designated test line of the paper strip. In a positive assay, the above-mentioned quantum dots with fatty acid binding protein in the sample form sandwich-like immunological complex structure at the test line, resulting in the fluorescence color change from green to red. This color change resulted from the target-induced internal filter effect between the loaded gold nanoparticles and green-emitting quantum dots, leading to an enhanced red emission and a reduced green emission. Quantitative detection of heart-type fatty acid binding protein was achieved with a low LOD of 0.21 ng/mL using a custom smartphone-based analytical device and tonality analysis. The ratiometric fluorescent lateral flow immunoassay strip enabled visually distinguishable green-to-red color changes around the threshold concentration of heart-type fatty acid binding protein (6.2 ng/mL), facilitating semi-quantitative diagnosis by the naked eye.
Fluorescent probes typically require short wavelengths of excitation light, which may interfere with the autofluorescence of cells and tissues, thus limiting their applications (Zhang et al., 2021). The advent of two-photon fluorescent probes coupled to two-photon microscopy overcomes these limitations by utilizing long-wavelength femtosecond laser sources to excite fluorescent probes. Since Maria Goeppert-Mayer introduced it in the 1930s (Grzybowski and Pietrzak, 2013), this method has been popular for in vivo imaging. A two-photon action cross-section is related to the quantitative degree of two-photon absorption capacity, mainly in units of the Goeppert-Mayer abbreviation (1 GM = 1 × 10−50 cm3 s/photon). Ratiometric two-photon fluorescent probes are capable of producing two or more emission peaks of different intensities or wavelengths. The intensity of one or more fluorescence peaks can be varied depending on how the RTPF interacts or reacts with a particular target. By establishing a calibration curve between the fluorescence signal ratio and the analyte standard concentration, the analyte level in the complex matrix can be determined. Four fundamental guidelines exist for building RTPF probes based on different ratiometric signal patterns (Figure 1B): 1) A two-emission probe exhibits the same (simultaneous increasing or decreasing) intensity trend upon analyte reappearance (Jun et al., 2018; Ren et al., 2020); 2) In the presence of analytes, a single-emission probe causes the emergence of a new emission peak and the reduction of the original emission peak (Kim et al., 2013; Park et al., 2018); 3) A two-emission probe consists of a reference signal showing minimal variation and a response signal showing significant variation (Sun et al., 2016; Wang et al., 2019; Reo et al., 2019); 4) The reaction (interaction) of a two-emission probe with a target gives rise to an increase in one emission peak and a reduction in the other emission peak (Divya et al., 2014; Ge et al., 2020). The sensing mechanisms of ratiometric two-photon fluorescence probes include one or more of FRET, through-bond energy transfer (TBET), photo-induced electron transfer (PET), excited state intramolecular proton transfer, intramolecular charge/electron transfer (ICT), and energy dissipation (Luo et al., 2017; Ding et al., 2020). Various reaction types are also involved between RTPF probes and targets in live cells and small animals, such as reduction reaction (Lim et al., 2011), nucleophilic addition (Zhao et al., 2018), and supramolecular assembly (Wang et al., 2018).
The following are examples of in vitro and in vivo studies using RTPF in recent years. Liang et al. developed a two-photon ratiometric fluorescent probe for real-time imaging and quantification of nitric oxide (NO) based on the coupling of FRET and PET mechanism (Liang et al., 2022). Coumarin and phthalimide-containing naphthalene dicarboximide in the probe acted as fluorescence donor and acceptor, respectively. The probe has two independent fluorescence emissions, high selectivity and good accuracy, with a detection range of 0.1–200 μM and a detection limit of 19.5 nM for NO. The probe successfully monitored and quantified NO in neural stem cells and different brain regions, revealing the role of NO in the activation and quiescent differentiation of neural stem cells, as well as its dose-dependent effect in promoting neuronal differentiation. Furthermore, it was found that NO concentrations were low in the hippocampal DG region of the brains of Alzheimer’s disease mice, and that treatment with NO-activated neural stem cells improved the symptoms of Alzheimer’s disease mice.
Chen et al. fabricated a two-photon ratiometric fluorescent probe consisting of rhodamine B (energy acceptor), methylhydrazine (recognising element), and quinoline derivatives (energy donor) for sensing Peroxynitrite, with a significant change in fluorescence signal (>200-fold) (S. Chen et al., 2021). Peroxynitrite is a reactive oxygen species (ROS) known to adversely affect physiological processes. The presence of peroxynitrite triggered the oxidation of methylhydrazine in rhodamine B, resulting in a FRET process from quinoline derivatives to rhodamine B. This reaction was completed within 2 min and showed good selectivity for peroxynitrite over other ROS, including hypochlorite. The probe was used to observe fluctuations in peroxynitrite production by arginase 1 in vivo and in vitro, suggesting that arginase 1 plays a key role in regulating peroxynitrite levels.
Valverde-Pozo et al. prepared a near-infrared fluorescent probe for the detection of dipeptidyl peptidase IV (DPP IV) activity, also known as CD26 (Valverde-Pozo et al., 2023). DPP IV is a transmembrane glycoprotein associated with glucose metabolism and T-cell stimulation. It is overexpressed in certain cancer tissues and can be used as a diagnostic marker for lysosomal storage diseases. This probe consisted of a DPP IV-sensitive dipeptide and a two-photon dicyanodiamine-4H-pyran derivative fluorophore. The dipeptide moiety blocked the internal charge transfer of the two-photon probe, thereby quenching its red emission and enhancing its green emission. Once DPP IV catalyzed the cleavage of the probe, the resultant products without dipeptide moiety produced intense red emission and weak green emission through the restoration of the internal charge transfer process. This two-photon ratiometric probe was suitable for super-resolution fluorescence imaging of DPP IV activity in living cells and tumour tissues when excited by two-photons. Zebrafish experiments show that DPP IV levels increased with developmental stage, suggesting the potential of this probe in cases of DPP IV overexpression such as cancer and diabetes.
Prepared a two-photon near-infrared ratiometric fluorescent nanocomposite via a click reaction between alkyne group-linked fluorescent molecules and azide-modified chitosan, followed by the application to the imaging of hydrogen peroxide (ONOO-) in liver injury (Xie et al., 2022). Liver injury is closely associated with ONOO−, making it an important biomarker for the diagnosis and assessment of liver disease. The small fluorescent molecule comprised a donor–π–acceptor structure of naphthalimide derivative, generating near-infrared emission through the FRET process from a donor to an acceptor. The formed nanocomposites possessed several favorable properties, including good water solubility, low background interference, deep tissue penetration and high imaging resolution. The oxonium functional group of the small fluorescent molecules in the nanocomposites reacted with ONOO−, resulting in the separation of naphthalimide derivative fluorophore and salicylic acid. Due to the cleavage of a donor–π–acceptor structure, the green emission of the nanocomposites intensified with increasing the concentration of ONOO−. The proposed nanoprobe exhibits a short reaction time (∼10 s) and a high selectivity and sensitivity for ONOO− detection with a LOD of 15.3 nM. I Significantly, the developed probe was effectively employed for the detection of ONOO- in viable HepG2 cells, as well as in mouse tissues exhibiting liver injury, and within mouse models.
Zhai et al. designed a viscosity-responsive, lysosome-targeted, two-photon fluorescent probe based on a TBET mechanism (Zhai et al., 2022). During autophagy, cells degrade themselves, keeping the body healthy. The study of autophagy and related diseases requires specific viscosity probes that can accumulate in lysosomes, as the viscosity of lysosomes increases during autophagy. This probe consisted of a viscosity-sensitive styrene derivative linked to a TBET energy acceptor and a two-photon excited coumarin derivative as an energy donor. An alkyne bond connects these two fluorescent molecules. Under two-photon excitation at 810 nm, the red emission of styrene derivatives in the probe was progressively enhanced with increasing the solution viscosity, while the green emission of coumarin derivatives remained almost unchanged. The integration of a two-photon confocal technique and the proposed probe offered a better signal-to-noise ratio fluorescence image with deeper penetration. The developed probe was applied to visualize autophagy processes under complex physiological conditions, including inflammation and stroke. As a result of the probe’s observations, ROS and inflammation may have a causal role in triggering autophagy activation during stroke. These findings demonstrate that the inflammatory-autophagy pathway holds promise for treating cerebrovascular diseases. The examples above confirm that the integration of two-photon excitation and near-infrared fluorescence enhances the imaging capabilities of probes in living cells, tissues, and animal models. It can be expected that as these ratiometric fluorescent probes continue to advance, they have great potential for advancing the understanding of a wide range of biological phenomena and, ultimately, for helping to develop targeted therapeutic interventions for a variety of diseases.
A powerful SERS technique can enhance the Raman scattering signal by several orders of magnitude through interactions between molecules and metal nanoparticles or plasmon resonance on rough metal surfaces (Langer et al., 2020; García-Hernández et al., 2023). Electromagnetic enhancement and chemical enhancement that are proposed to contribute to the SERS effect are now generally accepted in the field (Wang et al., 2021; Santhoshkumar et al., 2023; Zhao et al., 2023). Raman scattering signals are enhanced by electromagnetic enhancement when light interacts with metal surfaces, which generates a strong electromagnetic field near the metal nanoparticles. A metallic nanomaterial used in SERS depends on its size, shape, and composition to enhance electromagnetic fields (Santhoshkumar and Murugan, 2021a; Lin et al., 2021; Xianyu et al., 2021). Due to the localized surface plasmon resonance effect, metal nanoparticles with sharp edges or corners, such as gold nanostars, can generate a stronger electromagnetic field (Hao et al., 2007). Silver nanomaterials’ higher polarization rate and lower electron density can also lead to stronger electromagnetic enhancement than gold nanomaterials (Chen et al., 2010; Kang et al., 2018). When metal nanomaterials are functionalized with specific molecules or functional groups, their plasmonic properties are altered, and their interactions with analytes are enhanced (Gehan et al., 2010; Santhoshkumar and Murugan, 2021b; Ishida et al., 2021; Lin et al., 2021; Xianyu et al., 2021). Also, semiconductor nanomaterials enhance R The Raman signal can be significantly enhanced by enhancing the charge transfer between the semiconductor and its adsorbed molecules. During charge transfer, electrons are transferred from the molecule to the surface of the semiconductor nanoparticle or vice versa, forming a charge transfer complex, which in turn increases the polarizability of the molecule and changes its vibrational modes to significantly enhance the Raman signal. Semiconductor nanomaterials are more affordable and easier to synthesize than those based on precious metals. Various semiconductor nanomaterials, such as silicon (Si), titanium dioxide, zinc oxide, and indium tin oxide, have been used in SERS (Kanehara et al., 2009; Wang et al., 2017; Wang et al., 2019; Zhao et al., 2020; Han et al., 2021; Rajput et al., 2022). In addition, MoS2 and WS2 have been shown to have a significant charge transfer mechanism, which is a chemical enhancement in SERS (Masoumi et al., 2022; Santhoshkumar et al., 2023).
The advantages of SERS include high sensitivity and selectivity, the ability to detect molecules at very low concentrations, non-destructive, and can be used for the detection of a wide range of molecules including biomolecules, pharmaceuticals, and environmental contaminants (Cai et al., 2015; Tackman et al., 2018; Geng et al., 2021; Peng et al., 2021; Kamal and Yang, 2022). In addition, SERS can be used for in situ and real-time monitoring, making it a valuable tool for a variety of applications, including biosensing, medical diagnostics, and environmental monitoring (Liu et al., 2021; Quan et al., 2021; Xie et al., 2022; Yue et al., 2022). However, one of the main challenges of SERS is the reproducibility and reliability of the measurements, since the intensity of the SERS signal is highly dependent on the preparation of the metal substrate and the specific experimental conditions used. In addition, the high sensitivity of SERS can sometimes lead to false positives or false negatives due to the presence of interfering molecules or lack of specificity of the SERS probe.
To address these challenges, researchers have proposed the ratiometric SERS method to improve the reproducibility of measurements. In ratiometric SERS, two molecules must be selected: a target molecule to be quantified or detected and a reference molecule. The reference molecule is a stable and well-characterized standard for normalizing the Raman signal. By calculating the ratio of the Raman signal intensities of the two molecules, the effects of variables such as laser power fluctuations, sample heterogeneity, and metal substrate variations can be minimized. The reference molecule should have a SERS enhancement factor comparable to that of the analyte molecule but must not be present in the sample. Using a suitable reference molecule ensures normalization of the SERS signal, compensates for variations in experimental conditions, and reduces the effects of SERS substrate variations. This approach is particularly suitable for complex samples or low-concentration samples where the SERS signal may exhibit considerable variability. Choosing an appropriate reference molecule for ratiometric SERS necessitates considering several factors, including the molecule’s SERS enhancement factor, chemical stability, and lack of interference with the Raman bands of the analyte. Generally, a suitable reference molecule should have a Raman signal in the spectral range of 1800–2,800 cm−1, where most biological molecules have negligible Raman signals (Figure 1C). Alkynes are a class of organic compounds that contain at least one triple bond between two carbon atoms. Symmetric and asymmetric stretching of C-H bonds in alkynes are detected at approximately 3,400 cm−1, while their C≡C bond display. A single stretching band, typically occurring in the range of 2,100–2,200 cm−1. These vibrational modes occur at higher wavenumbers than those typical of biological molecules, such as proteins, nucleic acids, and lipids. When two carbon atoms in an alkyne molecule move in the same direction, either toward or away from one another, symmetric stretching takes place. This movement leads to a stretching vibration of the carbon-carbon triple bond, resulting in a Raman signal in a specific band. For example, 4-((4-mercaptophenyl)diazenyl)phenyl 3-((4-(phenyethynyl)benzyl)thio) propanoate exhibited a Raman peak at 2,207 cm−1 for the sensing of hypoxia (Qin et al., 2019). Also, N-(12-((4-(phenylethynyl)benzyl)amino)dodecyl) polyethylene glycol with a Raman peak at 2,219 cm−1 was exploited to sense alkaline phosphatase (Zhao et al., 2021). Furthermore, a notable illustration is the application of 3-[4-(Phenylethynyl)benzylthio]propanoic acid-modified DNA, which showcased a Raman peak at 2,215 cm−1 and served as an endonuclease-responsive probe (Si et al., 2018).
The following are examples of in vitro and in vivo studies using ratiometric SERS nanoprobes in recent years. Bi et al. synthesized citrate-terminated gold nanoparticles and modified them with the pH-sensitive Raman probe, 4-mercaptopyridine, by forming strong Au-S bonds (Bi et al., 2020). After addition of potassium hexacyanoferrate(II) and ferric chloride, the resulting products were encapsulated into Prussian blue shells. The Prussian blue shell not only exhibited a single C≡N stretch band, which served as a background-free internal standard in living cells, but also had a sub-nanometre porous structure that selectively detected H+ and OH− without interference from biomolecules such as biothiol proteins and DNA. The probe uses the ratiometric Raman signal of 4-mercaptopyridine to Prussian blue shell nitrile to measure pH from 4.0 to 9.0 in complex biological environments. They used the probe to monitor a significant decrease in pH when cells were treated with 6-hydroxydopamine, which triggers cellular degradation processes. They also monitored changes in intracellular pH during autophagy induced by nutrient deficiency. This study demonstrated that this ratiometric pH probe was well-suited to observe autophagy by quantifying intracellular pH fluctuations.
Similarly, Shen et al. reported the production of gold nanoparticle-loaded Prussian blue nanocomposites through the two-step process (Shen et al., 2021). Firstly, the seed growth method was used to prepare 60 nm-sized gold nanoparticles, which were then etched with K3[Fe(CN)6]. This procedure results in the formation of a CN− molecular layer on the gold nanoparticle surface, which is helpful to form a homogeneous coating of Prussian blue. The subsequent step involved the gradual formation of shell on CN−-coated gold nanoparticles after the addition of PbCl2 or CuCl2 instead of FeCl2 as precursors in the presence of K3[Fe(CN)6]. Two types of Prussian blue loaded with gold nanoparticles formed from PbCl2 and CuCl2 precursors showed different SERS signals at 2,122 cm−1 and 2,176 cm−1, respectively. Modification of ligands targeting epithelial cell adhesion molecules and epidermal growth factor receptor, respectively, on two Raman probes allows for simultaneous determination of the relative expression of these two membrane proteins on the cell surface, thus enabling interference-free ratio detection.
Besides, Zhong et al. used 4-azidobenzenthiol-modified gold nanoparticles as a ratiometric SERS nanoprobe for imaging endogenous H2S in individual living cells (Zhong et al., 2022). The thiol moiety of 4-azidobenezenthiol attached to the surface of the citrate-capped gold nanoparticles, while its azide moiety showed a strong Rama signal at 2,137 cm−1 in the cell silencing zone. In addition, the Raman peak at 1,002 cm−1 of 4-azidobenzethiol-modified gold nanoparticles corresponding to C-S stretching was used as an internal standard. The presence of H2S triggered the reduction of the above azide group to an amino group but did not affect the Raman intensity of the C-S stretching. Therefore, the response of this SERS nanoprobe to H2S can be generated with a relative standard deviation of 4.9%. Incubation of HeLa cells with the nanoprobe produced significant SERS signals, mainly concentrated in the cytoplasm. Changes in the ratiometric SERS signal were observed when the H2S scavenger ZnCl2 and S-adenosyl-l-methionine were used to stimulate the cells. This result confirms that this nanoprobe can distinguish between H2S-rich and H2S-deficient cells. They suggest that this nanoprobe has the potential to image endogenous H2S production, differentiate cells based on H2S levels, and observe H2S fluctuations in response to external stimuli at single-cell resolution.
Qin et al. synthesized Ag/Au alloy nanoparticle-adsorbed single-wall carbon nanotubes and modified their surface with azo-alkyne derivatives, forming a ratiometric SERS nanoprobe for sensing hypoxia in hepatic ischemia (Qin et al., 2019). The developed nanoprobe exhibited the Raman signals of 2,207 and 2,578 cm−1 corresponding to azo-alkyne derivatives and single-wall carbon nanotubes, respectively. In a hypoxic environment, azo-alkyne derivatives underwent a sequential reduction process through different reductase-catalyzed reaction. The reductase-mediated cleavage of the azo bond triggered the removal of the alkyne-groups from the nanoprobe, resulting in the loss of characteristic alkyne Raman bands at 2,207 cm–1. Therefore, the determination of the hypoxia level in different cell lines and rat liver tissue samples (from hepatic ischemia surgery) was successfully achieved by monitoring the ratio of two Raman peak intensities (I2578/I2207). Of notable significance, the application of this innovative SERS nanoprobe revealed that hepatic ischemia induces the emergence of a hypoxic state within the liver. These findings demonstratesthat future developments in ratiometric SERS nanoprobes hold exciting potential. Enhanced sensitivity, multiplexing capabilities, and improved biocompatibility will likely play a pivotal role in advancing these probes for more complex and intricate applications. Integration with imaging techniques such as microscopy could enable real-time monitoring of cellular processes.
An electrochemical sensor consists of three basic components: a receptor that is adept at binding to the sample, an analyte that initiates a reaction in the sample, and a sensor that is capable of converting the reaction into an electrical signal. One of these, the amperometric sensor, measures the current flowing between the working and reference electrodes when a consistent voltage is applied, and the magnitude of the current reflects the concentration of the desired analyte (Mistry et al., 2014). Potentiometric sensors, on the other hand, measure concentration by analyzing the potential difference between the working and reference electrodes (Bobacka et al., 2008). This difference conforms to the logarithmic relationship specified by the Nernst equation and is the basis for ion-selective electrodes, which can be used to measure specific ions such as sodium, potassium, and chloride in solution. Impedance sensors, on the other hand, have demonstrated superior performance by using impedance spectroscopy to measure the electrical impedance of a system at different frequencies (He et al., 2020). Shifts in impedance resulting from interactions at the electrode interface or adjustments in the electrolyte’s conductivity provide insights into analyte presence and concentration. In addition, photoelectrochemical sensors harness light-induced alterations in electrochemical dynamics (Shu and Tang, 2019). Typically employing semiconductor electrodes, they orchestrate the generation or augmentation of an electrical signal upon exposure to light. These sensors find applications spanning diverse domains, from driving electron transfer processes like solar cells to initiating electrochemical reactions for analyte detection. In these electrochemical sensors, the recognition elements include a variety of different molecules such as antibodies, aptamers, and nucleic acids. Molecularly imprinted polymers (MIPs) are also a recently developed recognition element (Gui et al., 2018; Sajini and Mathew, 2021; Wei et al., 2022). The template molecule is integrated into the polymer matrix during MIP synthesis, and once the template is removed, a specific recognition site is created for that molecule. This feature makes a highly selective polymer sensitive to the target molecule in complex matrices. In addition, they offer a relatively low production cost and scalable production as an alternative to antibodies. Finally, MIPs are renewable and can be reused many times, which reduces overall cost and environmental impact. However, the synthesis of MIPs requires careful selection of monomers and functional groups and is very time-consuming. In addition, the selectivity of MIPs cannot be easily changed after synthesis, limiting their versatility. In addition, MIPs degrade over time and thus lose their recognition properties, and their stability is affected by environmental conditions such as pH and temperature.
MIPs can be fabricated on electrodes by different methods, such as molecular imprinting by surface polymerization (Moreira et al., 2013), molecular imprinting by electropolymerization (Gui et al., 2018; Menon et al., 2018), molecular imprinting using MIP particles embedded in a slurry or ink (Camargo et al., 2022), and molecular imprinting by sol-gel derivatization techniques (Güney and Cebeci, 2015). In-situ polymerization reactions produce MIPs using template molecules, functional monomers, and crosslinkers. After removing the embedded template molecules, the MIPs build complementary size and function microcavities. Combined with ratiometric signal output modes, ratiometric MIPs are novel electrochemical sensors. A ratiometric MIP electrochemical detection incorporates an electrochemical signal-producing molecule in addition to the analyte, providing intrinsic calibration of the internal signal. The design of ratiometric signal output with dual-signal output, including on/off and on-off modes, eliminates interferences and improve stability. Ratiometric MIP electrochemical detection can be categorized into three types depending on the source of the signal. In the first type, two signals are detected at the same electrode, one from the target molecule and the other from MIPs or nanomaterials such as Cu2+-linked metal-organic frameworks (Hu et al., 2020), poly (methylene blue) (Liu et al., 2022), and polythionine (Yang et al., 2019). The second type involves a signal from the target molecule and another signal from the electrolyte, such as 3,3′,5,5′-tetramethylbenzidine and K3[Fe(CN)6] (Hu et al., 2022a). Thirdly, two electroactive molecules compete for the same binding site at the same electrode, one from the target molecule and the other from its analog as a reference signal (Yi et al., 2019). Ratiometric MIP electrochemical detection shows great potential for a broad range of applications. One of the most important applications is the monitoring of environmental contaminant levels in water and soil samples, such as fluorine-9-bisphenol (Mi et al., 2019). For drug residue monitoring, the ratiometric MIP electrochemical assay can recognize drug residues even in the presence of other interfering compounds and selectively bind to MIP, for example, for the detection of food residues such as chlorpromazine (Liu et al., 2022). In toxin detection, ratiometric MIP electrochemical assays can selectively detect toxins in food, such as mycotoxins (Zhu et al., 2023). In disease screening, ratiometric MIP electrochemical assays can screen for disease markers, such as dopamine in biological fluids (Yang et al., 2019), contributing to the early diagnosis and treatment of the disease. Lastly, pesticide residues in food, including imidacloprid residues, can be effectively detected using the ratiometric MIP electrochemical assay ratio method (Pérez-Fernández et al., 2019).
Examples of recent applications of ratiometric MIP electrochemical detection on real samples are as follows. Wu et al. developed a ratiometric MIP electrochemical sensor for detecting Sunset Yellow based on the integration of Sunset Yellow-selective MIPs with gold nanoparticle-modified indium-tin-oxide electrodes (Wu et al., 2023). In this electrochemical sensor, gold nanoparticles served as a reference signal without interfering with the signal of electroactive sunset yellow. The interesting observation is that the current signal of gold nanoparticles was also enhanced with increasing the Sunset Yellow concentration, referred to as “on-on” mode. Therefore, the MIP-modified electrode not only selectively captured Sunset Yellow from an aqueous solution but also allowed for its precise quantification with a wide detection range (10 nM–100 μM). In addition, the sensor was also demonstrated to detect sunset yellow in food samples with recoveries ranging from 94.0% to 97.0% and relative errors ranging from 5.4% to 8.3%, suggesting that it is promising for practical applications in the detection of sunset yellow.
Hu et al. modified a glassy carbon electrode with electroactive nanocomposites and employed it as a substrate for the formation of ochratoxin A (OTA)-recognised MIPs via the electro-polymerisation of 2,3-toluenediamine in cyclic voltammetry mode (Hu et al., 2022b) (Figure 1D). The nanocomposites, consisting of gold nanoparticles, poly (ionic liquid), flavin mononucleotide-decorated carbon nanotubes, and MoS2 nanosheets, provided a reference signal for ratiometric sensing of OTA. Under the square wave voltammetry mode, a gradual increase in the OTA concentration resulted in an enhanced peak current of the OTA oxidation products together with a reduced peak current of the nanocomposites. The proposed sensor exhibited a linear response for 0.5–15 μM OTA with a LOD of 14 nM. The excellent sensitivity of the MIP-loaded electrochemical sensors allowed the determination of OTA in food and beverages.
Liu et al. developed a MIP-loaded electrochemical sensor for the sensing of cannabidiol in refined oil and human serum samples (Liu et al., 2023). They modified the glassy carbon electrode with nitrogen and sulfur elements-doped carbon materials. This modification proficed the active sites for the electrodeposition of Fe3+-coordinated aminophenanthroline and 3,4-ethylenedioxythiophene, forming a MIP film on the electrode. The formed MIP film, in addition to its ability to recognize target molecules and provide a reference signal, also exhibited enzyme-like catalytic activity for the oxidation of cannabidiol. Thus, as the concentration of cannabidiol increased, the resulting electrochemical current associated with cannabidiol also increased. However, notably, the current generated by the MIP film remained relatively constant despite the increasing concentration of cannabidiol. When 5-hydroxytryptophan was used as a template molecule instead of cannabidiol in the synthesis of MIP films, the resultant MIP-loaded electrochemical sensors provided excellent selectivity toward 5-hydroxytryptophan. More importantly, the ratiometric MIP electrochemical sensors improved measurement repeatability and mitigated the effects of variations between batches of electrodes compared to non-ratiometric ones.
Mahmoud et al. designed a Cu2+-linked MIP film on the modified electrode for the ratiometric detection of glutathione in dietary supplements and human serum samples (Mahmoud et al., 2023). The modified electrode consisted of porous carbon doped with nitrogen and sulphur elements as a MIP-loaded substrate and silver nanoparticles as an internal reference signal. Moreover, the formation of a glutathione-sensitive MIP film was conducted via the electropolymerization of pyrrole-3-carboxylic acid and Cu2+ in the presence of glutathione. The addition of glutathione led to a decreased signal at 0.18 V due to the glutathione-mediated etching of silver nanoparticles and an increased signal at 0.83 V owing to the oxidation of the Ag(I)-glutathione complex. The proposed MIP specific gravity sensor possessed remarkable features of high measurement reproducibility, wide quantitative range, low LOD and good selectivity. The latest use cases highlight the impressive potential of ratiometric MIP electrochemical sensors. Their ability to combine selective recognition with internal reference signals improves accuracy, repeatability, and adaptability for analyzing real samples. As the field of electrochemical sensing continues to evolve, these innovations pave the way for the development of practical and effective solutions for various analytical challenges.
MALDI-TOF-MS is one of the most advanced and sensitive mass spectrometry techniques, first developed by Tanaka, Karas and Hillenkamp in the 1980s (Mandal et al., 2019). MALDI is a soft ionization technique used to ionize large biological macromolecules, such as proteins, peptides, nucleic acids, carbohydrates, and lipids (Eriksson et al., 2013). Due to its gentle ionization process and reduced fragmentation, MALDI-MS can detect intact molecular ions, making it ideally suited for studying complex biomolecular structures and interactions. The MALDI matrix behaves as an energy transfer agent, efficiently transferring the laser energy to the analyte molecules, triggering their desorption and ionization. The matrix’s roles include energy absorption, proton generation, and assisting in analyte ionization. The choice of the matrix depends on the analyte of interest, and commonly used matrices include sinapinic acid for intact proteins, α-cyano-4-hydroxycinnamic acid for peptides, and 2,5-dihydroxybenzoic acid for small molecules. Despite its sensitivity and analytical capabilities, MALDI-TOF MS has historically been considered a non-quantitative technique (Schlosser et al., 2005; Tholey et al., 2006). The main problem with MALDI-TOF MS is the poor reproducibility of the measurements, mainly due to the generation of strong signals in the “sweet spot” regions of the concentration of analyzed molecules (Szaéjli et al., 2008). Due to the unpredictable nature of these spots, achieving consistent detection and quantification in the absence of internal standards is challenging. These spots also hinder automated measurements, limiting the application of MALDI-TOF MS in spatially resolved imaging. The factors responsible for the formation of sweet spots are not fully understood, but the concentration and crystallinity of the matrix appear to play an important role.
To address these challenges and ensure accurate quantitative mass spectrometry results, the relative abundance of molecules in a sample is often assessed using ratiometric MALDI-TOF-MS. Internal standards, especially isotope-labeled analyte analogs, are recognized as effective methods to compensate for fluctuations in the MALDI signal due to variations in ionization efficiency and sample handling. Various stable isotope labeling methods have been developed for accurate and precise quantification of peptides in mass spectrometry-based proteomics (Figure 2). One such method is Isotope-coded affinity tags (ICAT), which employ a chemical labeling approach to attach a specific tag to the thiol group of cysteine residues in proteins (Wdowiak et al., 2021). By selectively labeling proteins or peptides containing cysteine residues with light or heavy isotopes, MALDI-TOF-MS can be used to generate peptide-related mass spectra for quantificative anaysis. Iodoacetamide is commonly used in ICATs for thiol reactivity (Gygi et al., 1999), but it has limitations and side effects, such as poor thiol selectivity and modest adduct formation rates. To address these issues, N-alkylmaleimides (NAMs) have been introduced as an alternative with faster and more selective reactivity (Hansen and Winther, 2009). Recent advances in ICATs led to the successful synthesis of isotope-coded maleimide affinity tags, which react quicker and more specifically to thiols than IAM (Wdowiak et al., 2021). The ICATs also possess a biotin residue at the other end, which facilitates the purification and enrichment of tagged peptides. Two ICAT pairs, which utilized butylene/D8-butylene linkers between maleimide and biotin residues, were found to be effective as MALDI-TOF-MS probes.
FIGURE 2. The mass spectrometry profiles resulting from the reaction of proteins with (A) ICAT, (B) iTRAQ, (C) SILAC, and (D) 18O labeling reagents.
Another labeling method, Isobaric tags for relative and absolute quantitation (iTRAQ), employs a similar chemical approach by attaching a N-hydroxysuccinimide (NHS)-derived tag to the N-terminus and lysine residues of peptides (Pierce et al., 2008). The isotopic reporter group, N-methylpiperazine, generates distinct isotopic variants of the iTRAQ tag, which serve as reporter ions during mass spectrometry analysis. This enables the relative and absolute quantitation of peptides or proteins in different samples. Unlike some other labeling methods, iTRAQ tags do not interfere with peptide fragmentation during MS/MS analysis, increasing the signal intensities of native peptides and enhancing fragmentation efficiency (Rauniyar and Yates, 2014). This leads to more accurate and reliable quantification of peptide signals. With iTRAQ, researchers can directly read the relative abundance of peptide signals without an internal standard by comparing the relative abundance between different experimental conditions.
Stable isotope-labeled amino acids in cell culture (SILAC) is an another powerful quantitative mass spectrometry that involves incorporating stable isotopes into proteins in living cells (Ong et al., 2002). This is achieved by substituting the normal amino acids with their isotopically marked counterparts. The cells are then cultured in media containing either light or heavy amino acid isotopes, resulting in distinct isotopic labeling of proteins. The differentially labeled proteins are then extracted, digested, and analyzed using mass spectrometry, allowing for the comparison of protein abundance between the light and heavy labeled samples. Besides, SILAC has proven to be a versatile method, particularly in pulse-chase experiments (Beller and Hummon, 2022). This technique entails metabolically incorporating stable isotopes, usually heavy versions of amino acids, into newly synthesized proteins. The heavy amino acids are introduced into cell cultures for a brief duration, facilitating selective labeling of specific proteins or protein populations. By employing pulsed SILAC labeling, researchers can track changes in protein expression over time, providing insights into the dynamics of protein regulation during various biological processes. This temporal analysis in proteomics has opened up new avenues for understanding the complexities of cellular responses. A recent study by Rocha et al. exemplifies the utility of SILAC-based proteomic analysis (Rocha et al., 2020). They investigated metabolic and proteomic changes in osteoarthritic mesenchymal stromal cells during chondrogenesis. Through SILAC, they identified altered protein abundances in metabolic pathways, providing valuable information about the molecular events underlying this cellular process. Additionally, the researchers used matrix-assisted laser desorption/ionization mass spectrometry imaging (MALDI-MSI) for metabolomic analysis, which revealed distinct profiles during the initial stages of differentiation.
Lastly, the 18O labeling entails the introduction of heavy oxygen water to label peptides, causing a 2Da mass shift per oxygen atom (Stewart et al., 2001). This approach involves enzymatic cleavage of the peptide in the presence of 18O-labeled water, with the addition of 18O at the C-terminus, although it has the advantage of eliminating the need for commercial isotope synthesis and high labeling efficiency. However, due to the small difference of only 2 Da between labeled and unlabeled peptide pairs, the mass spectrometry signals will inevitably overlap, thus affecting the accuracy of quantification. In addition, sample complexity and small mass differences complicate data analysis and interpretation. Despite its limitations, 18O labeling remains a valuable technique for specific applications. For example, it has been successfully applied to quantitative proteomics studies of post-translational modifications such as phosphorylation and glycosylation (Ye et al., 2009), providing valuable information on the dynamics of these modifications under different cellular conditions.
Despite their strengths, each quantitative mass spectrometry technique mentioned above has limitations. iTRAQ quantification, while widely used, is limited by the potential for ratio compression or distortion. This can lead to inaccuracies in quantifying changes in protein abundance, especially in complex samples with varying protein ratios. On the other hand, ICAT is restricted explicitly to cysteine-containing proteins and peptides. While it offers targeted quantification of proteins with cysteine residues, it may not be suitable for analyzing the broader proteome. SILAC, another popular method, incorporates expensive isotope-labeled amino acids into living cells. While it provides reliable and precise quantification in specific experimental setups, the cost of these labeled amino acids may be prohibitive for some researchers. Additionally, SILAC may not be compatible with all cell types or experimental conditions. Similarly, 18O labeling suffers from low labeling efficiency, potentially resulting in incomplete labeling of peptides and affecting quantification accuracy. Moreover, extensive sample purification might be necessary to remove unreacted 18O-labeled water and ensure reliable results. A common challenge across these labeling techniques is the availability and cost of isotope-labeled standards. These standards are often expensive or commercially unavailable, making their use in quantitative studies impractical. Furthermore, synthesizing some isotopically labeled compounds can be extremely difficult or even impossible, limiting the scope of certain analyses. In short, isotope labeling is a powerful technique for quantitative analysis, but due to its limitations, alternative methods must be considered to gain full coverage of a given analytical problem.
This article emphasizes the ongoing need for further advancements in ratiometric detection and sensing technologies. Researchers are encouraged to concentrate on designing more precise and sensitive ratiometric fluorescent nanoprobes, enhancing naked-eye detection with improved sensitivity and reproducibility. Exploring new applications for these sensors, such as point-of-care diagnostics and real-time environmental monitoring, holds promise. Integration of ratiometric fluorescent nanoprobe with microfluidic devices could enable high-throughput analysis. The development of novel two-photon probes with enhanced two-photon absorption cross-sections and quantum yields remains a crucial pursuit. Such improvements are pivotal for achieving high sensitivity in ratiometric systems and high-resolution imaging. Notably, ratiometric two-photon fluorescent probes offer potential applications in biomedical therapies, including in situ real-time controlled drug release, chemotherapy, immunotherapy, photothermal therapy, photodynamic therapy, and gene therapy. Ratiometric molecularly imprinted electrochemical detection shows potential for overcoming the limitations of conventional electrochemical methods. However, ongoing investigation is necessary to enhance the stability and synthesis process of MIPs. To facilitate real-time monitoring, ratiometric electrochemical sensors can be integrated with microelectromechanical systems. On the ratiometric SERS front, the development of novel Raman-sensitive probes featuring specific recognition elements offers the exciting prospect of simultaneous imaging of distinct organelles within living cells. Furthermore, various extraction methods could be harnessed to enhance SERS sensitivity for analyte detection. Future advancements in MALDI-TOF-MS might focus on refining the accuracy and precision of isotope labeling techniques and exploring more cost-effective strategies for quantitative analysis. Combining ratiometric MALDI-TOF-MS with imaging mass spectrometry could provide spatially resolved analysis of biological samples.
W-BT: Writing–original draft, Writing–review and editing. W-LT: Writing–original draft, Writing–review and editing. MM and SS participated in revising the manuscripts and answering the reviewers’ comments, W-BT: contributed to the preparation of the manuscripts and the collection of references for this review article. W-LT: participated in the preparation and modification of the manuscripts. These authors contributed equally to this work.
The authors declare financial support was received for the research, authorship, and/or publication of this article. We would like to thank the Ministry of Science and Technology (MOST 110-2811-M-110--512) and the NSYSU-KMU Joint Research Project (110-P003), and National Science and Technology Council (NSTC 112-2222-E-212 -002 -MY2) for financial support of this study.
The authors declare that the research was conducted in the absence of any commercial or financial relationships that could be construed as a potential conflict of interest.
The authors declared that they were an editorial board member of Frontiers, at the time of submission. This had no impact on the peer review process and the final decision
All claims expressed in this article are solely those of the authors and do not necessarily represent those of their affiliated organizations, or those of the publisher, the editors and the reviewers. Any product that may be evaluated in this article, or claim that may be made by its manufacturer, is not guaranteed or endorsed by the publisher.
Abbasi-Moayed, S., Golmohammadi, H., Bigdeli, A., and Hormozi-Nezhad, M. R. (2018). A rainbow ratiometric fluorescent sensor array on bacterial nanocellulose for visual discrimination of biothiols. Analyst 143 (14), 3415–3424. doi:10.1039/C8AN00637G
Ali, T. A., Mohamed, G. G., Azzam, E., and Abd-elaal, A. A. (2014). Thiol surfactant assembled on gold nanoparticles ion exchanger for screen-printed electrode fabrication. Potentiometric determination of Ce(III) in environmental polluted samples. Sens. Actuators B Chem. 191, 192–203. doi:10.1016/j.snb.2013.09.110
Beller, N. C., and Hummon, A. B. (2022). Advances in stable isotope labeling: dynamic labeling for spatial and temporal proteomic analysis. Mol. Omics 18 (7), 579–590. doi:10.1039/D2MO00077F
Bi, Y., Di, H., Zeng, E., Li, Q., Li, W., Yang, J., et al. (2020). Reliable quantification of pH variation in live cells using prussian blue-caged surface-enhanced Raman scattering probes. Anal. Chem. 92 (14), 9574–9582. doi:10.1021/acs.analchem.0c00714
Bigdeli, A., Ghasemi, F., Abbasi-Moayed, S., Shahrajabian, M., Fahimi-Kashani, N., Jafarinejad, S., et al. (2019). Ratiometric fluorescent nanoprobes for visual detection: design principles and recent advances-A review. Anal. Chim. Acta 1079, 30–58. doi:10.1016/j.aca.2019.06.035
Bobacka, J., Ivaska, A., and Lewenstam, A. (2008). Potentiometric ion sensors. Chem. Rev. 108 (2), 329–351. doi:10.1021/cr068100w
Cai, Q., Li, L. H., Yu, Y., Liu, Y., Huang, S., Chen, Y., et al. (2015). Boron nitride nanosheets as improved and reusable substrates for gold nanoparticles enabled surface enhanced Raman spectroscopy. Phys. Chem. Chem. Phys. 17 (12), 7761–7766. doi:10.1039/C5CP00532A
Camargo, J. R., Silva, T. A., Rivas, G. A., and Janegitz, B. C. (2022). Novel eco-friendly water-based conductive ink for the preparation of disposable screen-printed electrodes for sensing and biosensing applications. Electrochim. Acta 409, 139968. doi:10.1016/j.electacta.2022.139968
Chen, S., Vurusaner, B., Pena, S., Thu, C. T., Mahal, L. K., Fisher, E. A., et al. (2021). Two-photon, ratiometric, quantitative fluorescent probe reveals fluctuation of peroxynitrite regulated by arginase 1. Anal. Chem. 93 (29), 10090–10098. doi:10.1021/acs.analchem.1c00911
Chen, X., Wo, F., Chen, J., Tan, J., Wang, T., Liang, X., et al. (2017). Ratiometric mass spectrometry for cell identification and quantitation using intracellular “Dual-Biomarkers”. Sci. Rep. 7 (1), 17432. doi:10.1038/s41598-017-17812-1
Chen, Y. S., Frey, W., Kim, S., Homan, K., Kruizinga, P., Sokolov, K., et al. (2010). Enhanced thermal stability of silica-coated gold nanorods for photoacoustic imaging and image-guided therapy. Opt. Exp. 18 (9), 8867–8878. doi:10.1364/OE.18.008867
Chuang, C. H., Chen, W. Y., Tseng, W. B., Lin, A., Lu, C. Y., and Tseng, W. L. (2022). Microwave-mediated synthesis of near-infrared-emitting silver ion-modified gold nanoclusters for ratiometric sensing of hydrosulfide in environmental water and hydrogen sulfide in live cells. ACS Sustain. Chem. Eng. 10 (7), 2461–2472. doi:10.1021/acssuschemeng.1c07440
Ding, H., Yuan, G., Peng, L., Zhou, L., and Lin, Q. (2020). TP-FRET-based fluorescent sensor for ratiometric detection of formaldehyde in real food samples, living cells, tissues, and zebrafish. J. Agric. Food Chem. 68 (11), 3670–3677. doi:10.1021/acs.jafc.9b08114
Divya, K. P., Sreejith, S., Ashokkumar, P., Yuzhan, K., Peng, Q., Maji, S. K., et al. (2014). A ratiometric fluorescent molecular probe with enhanced two-photon response upon Zn2+ binding for in vitro and in vivo bioimaging. Chem. Sci. 5 (9), 3469–3474. doi:10.1039/C4SC00736K
Eriksson, C., Masaki, N., Yao, I., Hayasaka, T., and Setou, M. (2013). MALDI imaging mass spectrometry—A mini review of methods and recent developments. Mass Spectrom. 2, S0022. doi:10.5702/massspectrometry.S0022
Gan, Z., Zhang, T., An, X., Tan, Q., Zhen, S., and Hu, X. (2022). A novel fluorescence-scattering ratiometric sensor based on Fe-NC nanozyme with robust oxidase-like activity. Sens. Actuators B Chem. 368, 132181. doi:10.1016/j.snb.2022.132181
García-Hernández, L. A., Martínez-Martínez, E., Pazos-Solís, D., Aguado-Preciado, J., Dutt, A., Chávez-Ramírez, A. U., et al. (2023). Optical detection of cancer cells using lab-on-a-chip. Biosensors 13 (4), 439. doi:10.3390/bios13040439
Ge, L., Liu, Z., and Tian, Y. (2020). A novel two-photon ratiometric fluorescent probe for imaging and sensing of BACE1 in different regions of AD mouse brain. Chem. Sci. 11 (8), 2215–2224. doi:10.1039/C9SC05256A
Gehan, H. l. n., Fillaud, L., Felidj, N., Aubard, J., Lang, P., Chehimi, M. M., et al. (2010). A general approach combining diazonium salts and click chemistries for gold surface functionalization by nanoparticle assemblies. Langmuir 26 (6), 3975–3980. doi:10.1021/la9033436
Geng, Z. Q., Xu, D., Song, Y., Wang, W. P., Li, Y. P., Han, C. Q., et al. (2021). Sensitive label-free detection of bilirubin in blood using boron nitride-modified nanorod arrays as SERS substrates. Sens. Actuators B Chem. 334, 129634. doi:10.1016/j.snb.2021.129634
Grzybowski, A., and Pietrzak, K. (2013). Maria goeppert-mayer (1906–1972): two-photon effect on dermatology. Clin. Dermatol. 31 (2), 221–225. doi:10.1016/j.clindermatol.2012.06.002
Gui, R., Jin, H., Guo, H., and Wang, Z. (2018). Recent advances and future prospects in molecularly imprinted polymers-based electrochemical biosensors. Biosens. Bioelectron. 100, 56–70. doi:10.1016/j.bios.2017.08.058
Güney, S., and Cebeci, F. C. (2015). Selective electrochemical sensor for theophylline based on an electrode modified with imprinted sol–gel film immobilized on carbon nanoparticle layer. Sensors Actuators B Chem. 208, 307–314. doi:10.1016/j.snb.2014.10.056
Gygi, S. P., Rist, B., Gerber, S. A., Turecek, F., Gelb, M. H., and Aebersold, R. (1999). Quantitative analysis of complex protein mixtures using isotope-coded affinity tags. Nat. Biotechnol. 17 (10), 994–999. doi:10.1038/13690
Han, B., Chen, L., Jin, S., Guo, S., Park, J., Yoo, H. S., et al. (2021). Modulating mechanism of the LSPR and SERS in Ag/ITO film: carrier density effect. J. Phys. Chem. Lett. 12 (31), 7612–7618. doi:10.1021/acs.jpclett.1c01727
Han, L., Meng, C., Zhang, D., Liu, H., and Sun, B. (2022). Fabrication of a fluorescence probe via molecularly imprinted polymers on carbazole-based covalent organic frameworks for optosensing of ethyl carbamate in fermented alcoholic beverages. Anal. Chim. Acta 1192, 339381. doi:10.1016/j.aca.2021.339381
Hansen, R. E., and Winther, J. R. (2009). An introduction to methods for analyzing thiols and disulfides: reactions, reagents, and practical considerations. Anal. Biochem. 394 (2), 147–158. doi:10.1016/j.ab.2009.07.051
Hao, F., Nehl, C. L., Hafner, J. H., and Nordlander, P. (2007). Plasmon resonances of a gold nanostar. Nano Lett. 7 (3), 729–732. doi:10.1021/nl062969c
He, S., Yuan, Y., Nag, A., Feng, S., Afsarimanesh, N., Han, T., et al. (2020). A review on the use of impedimetric sensors for the inspection of food quality. Int. J. Environ. Res. Public Health. 17 (14), 5220. doi:10.3390/ijerph17145220
HeeáLee, M., SeungáKim, J., and Sessler, J. L. (2015). Small molecule-based ratiometric fluorescence probes for cations, anions, and biomolecules. Chem. Soc. Rev. 44 (13), 4185–4191. doi:10.1039/c4cs00280f
Hu, R., Zhang, X., Chi, K. N., Yang, T., and Yang, Y. H. (2020). Bifunctional MOFs-based ratiometric electrochemical sensor for multiplex heavy metal ions. ACS Appl. Mat. Interfaces 12 (27), 30770–30778. doi:10.1021/acsami.0c06291
Hu, X., Tang, Y., Xia, Y., Liu, Y., Zhao, F., and Zeng, B. (2022a). Antifouling ionic liquid doped molecularly imprinted polymer-based ratiometric electrochemical sensor for highly stable and selective detection of zearalenone. Anal. Chim. Acta 1210, 339884. doi:10.1016/j.aca.2022.339884
Hu, X., Xia, Y., Liu, Y., Chen, Y., and Zeng, B. (2022b). An effective ratiometric electrochemical sensor for highly selective and reproducible detection of ochratoxin A: use of magnetic field improved molecularly imprinted polymer. Sens. Actuators B Chem. 359, 131582. doi:10.1016/j.snb.2022.131582
Huang, X., Song, J., Yung, B. C., Huang, X., Xiong, Y., and Chen, X. (2018). Ratiometric optical nanoprobes enable accurate molecular detection and imaging. Chem. Soc. Rev. 47 (8), 2873–2920. doi:10.1039/C7CS00612H
Ishida, T., Yanaga, Y., Yamada, S., and Takahashi, Y. (2021). A versatile method for surface functionalization and hydrophobization of gold nanoparticles. Appl. Surf. Sci. 546, 148932. doi:10.1016/j.apsusc.2021.148932
Jin, H., Jiang, X., Sun, Z., and Gui, R. (2021). Phosphorescence-based ratiometric probes: design, preparation and applications in sensing, imaging and biomedicine therapy. Coord. Chem. Rev. 431, 213694. doi:10.1016/j.ccr.2020.213694
Jun, Y. W., Wang, T., Hwang, S., Kim, D., Ma, D., Kim, K. H., et al. (2018). A ratiometric two-photon fluorescent probe for tracking lysosomal ATP: direct in cellulo observation of lysosomal membrane fusion processes. Angew. Chem. Int. Ed. Engl. 130 (32), 10299–10304. doi:10.1002/ange.201804743
Kamal, S., and Yang, T. C. K. (2022). Silver enriched silver phosphate microcubes as an efficient recyclable SERS substrate for the detection of heavy metal ions. J. Colloid Interface Sci. 605, 173–181. doi:10.1016/j.jcis.2021.07.084
Kanehara, M., Koike, H., Yoshinaga, T., and Teranishi, T. (2009). Indium tin oxide nanoparticles with compositionally tunable surface plasmon resonance frequencies in the near-IR region. J. A. Chem. Soc. 131 (49), 17736–17737. doi:10.1021/ja9064415
Kang, H., Buchman, J. T., Rodriguez, R. S., Ring, H. L., He, J., Bantz, K. C., et al. (2018). Stabilization of silver and gold nanoparticles: preservation and improvement of plasmonic functionalities. Chem. Rev. 119 (1), 664–699. doi:10.1021/acs.chemrev.8b00341
Ke, C. Y., Wu, Y. T., and Tseng, W. L. (2015). Fluorescein-5-isothiocyanate-conjugated protein-directed synthesis of gold nanoclusters for fluorescent ratiometric sensing of an enzyme–substrate system. Biosens. Bioelectron. 69, 46–53. doi:10.1016/j.bios.2015.02.002
Kim, H. J., Heo, C. H., and Kim, H. M. (2013). Benzimidazole-based ratiometric two-photon fluorescent probes for acidic pH in live cells and tissues. J. Am. Chem. Soc. 135 (47), 17969–17977. doi:10.1021/ja409971k
Kumar, A. S. K., Tseng, W. B., Wu, M. J., Huang, Y. Y., and Tseng, W. L. (2020). L-cystine-linked BODIPY-adsorbed monolayer MoS2 quantum dots for ratiometric fluorescent sensing of biothiols based on the inner filter effect. Anal. Chim. Acta 1113, 43–51. doi:10.1016/j.aca.2020.04.006
Kumar, S., Verma, T., Mukherjee, R., Ariese, F., Somasundaram, K., and Umapathy, S. (2016). Raman and infra-red microspectroscopy: towards quantitative evaluation for clinical research by ratiometric analysis. Chem. Soc. Rev. 45 (7), 1879–1900. doi:10.1039/c5cs00540j
Kumaravel, S., Wu, S. H., Chen, G. Z., Huang, S. T., Lin, C. M., Lee, Y. C., et al. (2021). Development of ratiometric electrochemical molecular switches to assay endogenous formaldehyde in live cells, whole blood and creatinine in saliva. Biosens. Bioelectron. 171, 112720. doi:10.1016/j.bios.2020.112720
Langer, J., Jimenez de Aberasturi, D., Aizpurua, J., Alvarez-Puebla, R. A., Auguié, B., Baumberg, J. J., et al. (2020). Present and future of surface-enhanced Raman scattering. ACS Nano 14 (1), 28–117. doi:10.1021/acsnano.9b04224
Li, L., Yang, L., Lin, D., Xu, S., Mei, C., Yu, S., et al. (2023). Hydrogen-bond induced enhanced emission ratiometric fluorescent handy needle for visualization assay of amoxicillin by smartphone sensing platform. J. Hazard. Mat. 444, 130403. doi:10.1016/j.jhazmat.2022.130403
Li, Z. Y., Wu, Y. T., and Tseng, W. L. (2015). UV-light-induced improvement of fluorescence quantum yield of DNA-templated gold nanoclusters: application to ratiometric fluorescent sensing of nucleic acids. ACS Appl. Mat. Interfaces 7 (42), 23708–23716. doi:10.1021/acsami.5b07766
Liang, M., Liu, Z., Zhang, Z., Mei, Y., and Tian, Y. (2022). A two-photon ratiometric fluorescent probe for real-time imaging and quantification of NO in neural stem cells during activation regulation. Chem. Sci. 13 (15), 4303–4312. doi:10.1039/D2SC00326K
Lim, C. S., Masanta, G., Kim, H. J., Han, J. H., Kim, H. M., and Cho, B. R. (2011). Ratiometric detection of mitochondrial thiols with a two-photon fluorescent probe. J. Am. Chem. Soc. 133 (29), 11132–11135. doi:10.1021/ja205081s
Lin, S., Cheng, Z., Li, Q., Wang, R., and Yu, F. (2021). Toward sensitive and reliable surface-enhanced Raman scattering imaging: from rational design to biomedical applications. ACS Sens. 6 (11), 3912–3932. doi:10.1021/acssensors.1c01858
Liu, J., Jiang, X., Zhang, R., Zhang, Y., Wu, L., Lu, W., et al. (2019). MXene-enabled electrochemical microfluidic biosensor: applications toward multicomponent continuous monitoring in whole blood. Adv. Funct. Mat. 29 (6), 1807326. doi:10.1002/adfm.201807326
Liu, R., Jiang, L., Yu, Z., Jing, X., Liang, X., Wang, D., et al. (2021). MXene (Ti3C2Tx)-Ag nanocomplex as efficient and quantitative SERS biosensor platform by in-situ PDDA electrostatic self-assembly synthesis strategy. Sens. Actuators B Chem. 333, 129581. doi:10.1016/j.snb.2021.129581
Liu, Y., Hu, X., Xia, Y., Zhao, F., and Zeng, B. (2022). A novel ratiometric electrochemical sensor based on dual-monomer molecularly imprinted polymer and Pt/Co3O4 for sensitive detection of chlorpromazine hydrochloride. Anal. Chim. Acta 1190, 339245. doi:10.1016/j.aca.2021.339245
Liu, Y., Tang, Y., Cao, J., Zhao, F., and Zeng, B. (2023). A ratiometric electrochemical sensing platform based on multifunctional molecularly imprinted polymer with catalytic activity for the detection of psychoactive substances. Biosens. Bioelectron. 220, 114929. doi:10.1016/j.bios.2022.114929
Luo, F., Zhu, M., Liu, Y., Sun, J., and Gao, F. (2023). Ratiometric and visual determination of copper ions with fluorescent nanohybrids of semiconducting polymer nanoparticles and carbon dots. Spectrochim. Acta A Mol. Biomol. Spectrosc. 295, 122574. doi:10.1016/j.saa.2023.122574
Luo, W., Jiang, H., Tang, X., and Liu, W. (2017). A reversible ratiometric two-photon lysosome-targeted probe for real-time monitoring of pH changes in living cells. J. Mat. Chem. B 5 (24), 4768–4773. doi:10.1039/C7TB00838D
Madhu, M., and Tseng, W. L. (2021). Recent developments in sensing of oversulfated chondroitin sulfate in heparin. A review. J. Food Drug Anal. 29 (4), 533–543. doi:10.38212/2224-6614.3379
Mahmoud, A. M., Mahnashi, M. H., and El-Wekil, M. M. (2023). Ratiometric sensing interface for glutathione determination based on electro-polymerized copper-coordinated molecularly imprinted layer supported on silver/porous carbon hybrid. Anal. Chim. Acta 1272 (1), 341498. doi:10.1016/j.aca.2023.341498
Mandal, A., Singha, M., Addy, P. S., and Basak, A. (2019). Laser desorption ionization mass spectrometry: recent progress in matrix-free and label-assisted techniques. Mass Spectrom. Rev. 38 (1), 3–21. doi:10.1002/mas.21545
Masoumi, Z., Tayebi, M., Kolaei, M., and Lee, B. K. (2022). Efficient and stable core-shell α–Fe2O3/WS2/WOx photoanode for oxygen evolution reaction to enhance photoelectrochemical water splitting. Appl. Catal. B Environ. 313, 121447. doi:10.1016/j.apcatb.2022.121447
Menon, S., Jesny, S., and Kumar, K. G. (2018). A voltammetric sensor for acetaminophen based on electropolymerized-molecularly imprinted poly (o-aminophenol) modified gold electrode. Talanta 179, 668–675. doi:10.1016/j.talanta.2017.11.074
Mi, P., Zhang, Q. P., Zhang, S. H., Wang, C., Zhang, S. Z., Fang, Y. C., et al. (2019). The effects of fluorene-9-bisphenol on female zebrafish (Danio rerio) reproductive and exploratory behaviors. Chemosphere 228, 398–411. doi:10.1016/j.chemosphere.2019.04.170
Miao, J., Ji, W., Yu, J., Cheng, J., Huang, Y., Arabi, M., et al. (2023). A triple-emission ratiometric fluorescence sensor based on carbon dots-Au nanoclusters nanocomposite for detection of tetracycline. Sens. Actuators B Chem. 384, 133636. doi:10.1016/j.snb.2023.133636
Mistry, K. K., Layek, K., Mahapatra, A., RoyChaudhuri, C., and Saha, H. (2014). A review on amperometric-type immunosensors based on screen-printed electrodes. Analyst 139 (10), 2289–2311. doi:10.1039/c3an02050a
Moreira, F. T., Dutra, R. A., Noronha, J. P., and Sales, M. G. F. (2013). Electrochemical biosensor based on biomimetic material for myoglobin detection. Electrochim. Acta 107, 481–487. doi:10.1016/j.electacta.2013.06.061
Njoko, N., Louzada, M., Britton, J., Khene, S., Nyokong, T., and Mashazi, P. (2020). Bioelectrocatalysis and surface analysis of gold coated with nickel oxide/hydroxide and glucose oxidase towards detection of glucose. Colloids Surf. B 190, 110981. doi:10.1016/j.colsurfb.2020.110981
Ong, S. E., Blagoev, B., Kratchmarova, I., Kristensen, D. B., Steen, H., Pandey, A., et al. (2002). Stable isotope labeling by amino acids in cell culture, SILAC, as a simple and accurate approach to expression proteomics. Mol. Cell. Proteom. 1 (5), 376–386. doi:10.1074/mcp.M200025-MCP200
Park, S. J., Kim, Y. J., Kang, J. S., Kim, I. Y., Choi, K. S., and Kim, H. M. (2018). Carboxylesterase-2-selective two-photon ratiometric probe reveals decreased carboxylesterase-2 activity in breast cancer cells. Anal. Chem. 90 (15), 9465–9471. doi:10.1021/acs.analchem.8b02101
Parrilla, M., Cánovas, R., and Andrade, F. J. (2017). Paper-based enzymatic electrode with enhanced potentiometric response for monitoring glucose in biological fluids. Biosens. Bioelectron. 90, 110–116. doi:10.1016/j.bios.2016.11.034
Peng, R., Si, Y., Deng, T., Zheng, J., Li, J., Yang, R., et al. (2016). A novel SERS nanoprobe for the ratiometric imaging of hydrogen peroxide in living cells. Chem. Commun. 52 (55), 8553–8556. doi:10.1039/C6CC03412H
Peng, Y., Lin, C., Long, L., Masaki, T., Tang, M., Yang, L., et al. (2021). Charge-transfer resonance and electromagnetic enhancement synergistically enabling MXenes with excellent SERS sensitivity for SARS-CoV-2 S protein detection. Nano-Micro Lett. 13, 52. doi:10.1007/s40820-020-00565-4
Pérez-Fernández, B., Mercader, J. V., Checa-Orrego, B. I., de la Escosura-Muñiz, A., and Costa-García, A. (2019). A monoclonal antibody-based immunosensor for the electrochemical detection of imidacloprid pesticide. Analyst 144 (9), 2936–2941. doi:10.1039/C9AN00176J
Pierce, A., Unwin, R. D., Evans, C. A., Griffiths, S., Carney, L., Zhang, L., et al. (2008). Eight-channel iTRAQ enables comparison of the activity of six leukemogenic tyrosine kinases. Mol. Cell. Proteom. 7 (5), 853–863. doi:10.1074/mcp.M700251-MCP200
Qin, X., Si, Y., Wang, D., Wu, Z., Li, J., and Yin, Y. (2019). Nanoconjugates of Ag/Au/carbon nanotube for alkyne-meditated ratiometric SERS imaging of hypoxia in hepatic ischemia. Anal. Chem. 91 (7), 4529–4536. doi:10.1021/acs.analchem.8b05487
Qu, J., Zhang, X., Liu, Y., Xie, Y., Cai, J., Zha, G., et al. (2020). N, P-co-doped carbon dots as a dual-mode colorimetric/ratiometric fluorescent sensor for formaldehyde and cell imaging via an aminal reaction-induced aggregation process. Microchim. Acta 187, 355. doi:10.1007/s00604-020-04337-0
Quan, Y., Su, R., Yang, S., Chen, L., Wei, M., Liu, H., et al. (2021). In-situ surface-enhanced Raman scattering based on MTi20 nanoflowers: monitoring and degradation of contaminants. J. Hazard. Mat. 412 (15), 125209. doi:10.1016/j.jhazmat.2021.125209
Rajput, V., Gupta, R. K., and Prakash, J. (2022). Engineering metal oxide semiconductor nanostructures for enhanced charge transfer: fundamentals and emerging SERS applications. J. Mat. Chem. C 10 (1), 73–95. doi:10.1039/D1TC04886D
Rauniyar, N., and Yates, J. R. (2014). Isobaric labeling-based relative quantification in shotgun proteomics. J. Proteome Res. 13 (12), 5293–5309. doi:10.1021/pr500880b
Ren, T. B., Wen, S. Y., Wang, L., Lu, P., Xiong, B., Yuan, L., et al. (2020). Engineering a reversible fluorescent probe for real-time live-cell imaging and quantification of mitochondrial ATP. Anal. Chem. 92 (6), 4681–4688. doi:10.1021/acs.analchem.0c00506
Reo, Y. J., Jun, Y. W., Sarkar, S., Dai, M., and Ahn, K. H. (2019). Ratiometric imaging of γ-glutamyl transpeptidase unperturbed by pH, polarity, and viscosity changes: A benzocoumarin-based two-photon fluorescent probe. Anal. Chem. 91 (21), 14101–14108. doi:10.1021/acs.analchem.9b03942
Rocha, B., Cillero-Pastor, B., Eijkel, G., Calamia, V., Fernandez-Puente, P., Paine, M. R., et al. (2020). Integrative metabolic pathway analysis reveals novel therapeutic targets in osteoarthritis. Mol. Cell. Proteom. 19 (4), 574–588. doi:10.1074/mcp.RA119.001821
Sajini, T., and Mathew, B. (2021). A brief overview of molecularly imprinted polymers: highlighting computational design, nano and photo-responsive imprinting. Talanta Open 4, 100072. doi:10.1016/j.talo.2021.100072
Santhoshkumar, S., and Murugan, E. (2021a). Rationally designed SERS AgNPs/GO/g-CN nanohybrids to detect methylene blue and Hg2+ ions in aqueous solution. Appl. Surf. Sci. 553, 149544. doi:10.1016/j.apsusc.2021.149544
Santhoshkumar, S., and Murugan, E. (2021b). Size controlled silver nanoparticles on β-cyclodextrin/graphitic carbon nitride: an excellent nanohybrid material for SERS and catalytic applications. Dalton. Trans. 50 (48), 17988–18000. doi:10.1039/d1dt02809j
Santhoshkumar, S., Wei, W. S., Madhu, M., Tseng, W. B., and Tseng, W. L. (2023). Chemically engineered sulfur vacancies on few-layered molybdenum disulfide nanosheets for remarkable surface-enhanced Raman scattering activity. J. Phys. Chem. C 127 (18), 8803–8813. doi:10.1021/acs.jpcc.3c01044
Schlosser, G., Pocsfalvi, G., Huszár, E., Malorni, A., and Hudecz, F. (2005). MALDI-TOF mass spectrometry of a combinatorial peptide library: effect of matrix composition on signal suppression. J. Mass Spectrom. 40 (12), 1590–1594. doi:10.1002/jms.937
Šebela, M. (2023). The use of matrix-assisted laser desorption/ionization mass spectrometry in enzyme activity assays and its position in the context of other available methods. Mass Spectrom. Rev. 42 (3), 1008–1031. doi:10.1002/mas.21733
Shahdost-Fard, F., Fahimi-Kashani, N., and Hormozi-Nezhad, M. (2021). A ratiometric fluorescence nanoprobe using CdTe QDs for fast detection of carbaryl insecticide in apple. Talanta 221, 121467. doi:10.1016/j.talanta.2020.121467
Shan, X., Feng, Q., Yang, J., and Wang, P. (2022). Dual-mode detection and efficient annihilation of pathogenic bacteria based on the construction of a ratiometric electrochemiluminescent/electrochemical sensor. Sens. Actuators B Chem. 372, 132633. doi:10.1016/j.snb.2022.132633
Shen, Y. M., Gao, M. Y., Chen, X., Shen, A. G., and Hu, J. M. (2021). Fine synthesis of Prussian-blue analogue coated gold nanoparticles (Au@ PBA NPs) for sorting specific cancer cell subtypes. Spectrochim. Acta A Mol. Biomol. Spectrosc. 252, 119566. doi:10.1016/j.saa.2021.119566
Shu, J., and Tang, D. (2019). Recent advances in photoelectrochemical sensing: from engineered photoactive materials to sensing devices and detection modes. Anal. Chem. 92 (1), 363–377. doi:10.1021/acs.analchem.9b04199
Si, Y., Bai, Y., Qin, X., Li, J., Zhong, W., Xiao, Z., et al. (2018). Alkyne–DNA-functionalized alloyed Au/Ag nanospheres for ratiometric surface-enhanced Raman scattering imaging assay of endonuclease activity in live cells. Anal. Chem. 90 (6), 3898–3905. doi:10.1021/acs.analchem.7b04735
Spring, S. A., Goggins, S., and Frost, C. G. (2021). Ratiometric electrochemistry: improving the robustness, reproducibility and reliability of biosensors. Molecules 26 (8), 2130. doi:10.3390/molecules26082130
Stewart, I. I., Thomson, T., and Figeys, D. (2001). 18O labeling: A tool for proteomics. Rapid Commun. Mass Spectrom. 15 (24), 2456–2465. doi:10.1002/rcm.525
Sun, J., Mei, H., Wang, S., and Gao, F. (2016). Two-photon semiconducting polymer dots with dual-emission for ratiometric fluorescent sensing and bioimaging of tyrosinase activity. Anal. Chem. 88 (14), 7372–7377. doi:10.1021/acs.analchem.6b01929
Szaejli, E., Feheer, T., and Medzihradszky, K. F. (2008). Investigating the quantitative nature of MALDI-TOF MS. Mol. Cell. Proteom. 7 (12), 2410–2418. doi:10.1074/mcp.M800108-MCP200
Tackman, E. C., Trujillo, M. J., Lockwood, T. L. E., Merga, G., Lieberman, M., and Camden, J. P. (2018). Identification of substandard and falsified antimalarial pharmaceuticals chloroquine, doxycycline, and primaquine using surface-enhanced Raman scattering. Anal. Metthods 10 (38), 4718–4722. doi:10.1039/C8AY01413B
Tholey, A., Zabet-Moghaddam, M., and Heinzle, E. (2006). Quantification of peptides for the monitoring of protease-catalyzed reactions by matrix-assisted laser desorption/ionization mass spectrometry using ionic liquid matrixes. Anal. Chem. 78 (1), 291–297. doi:10.1021/ac0514319
Tseng, W. B., Rau, J. Y., Chiou, H. C., and Tseng, W. L. (2022). Synthesis of gold nanoclusters-loaded lysozyme nanoparticles for ratiometric fluorescent detection of cyanide in tap water, cyanogenic glycoside-containing plants, and soils. Environ. Res. 207, 112144. doi:10.1016/j.envres.2021.112144
Valverde-Pozo, J., Paredes, J. M., Widmann, T. J., Griñan-Lison, C., Ceccarelli, G., Gioiello, A., et al. (2023). Ratiometric two-photon near-infrared probe to detect DPP IV in human plasma, living cells, human tissues, and whole organisms using zebrafish. ACS Sens. 8 (3), 1064–1075. doi:10.1021/acssensors.2c02025
Vargas, E., Teymourian, H., Tehrani, F., Eksin, E., Sánchez-Tirado, E., Warren, P., et al. (2019). Enzymatic/immunoassay dual-biomarker sensing chip: towards decentralized insulin/glucose detection. Angew. Chem. Int. Ed. 58 (19), 6376–6379. doi:10.1002/anie.201902664
Vyas, G., Bhatt, S., and Paul, P. (2019). Synthesis of calixarene-capped silver nanoparticles for colorimetric and amperometric detection of mercury (HgII, Hg0). ACS Omega 4 (2), 3860–3870. doi:10.1021/acsomega.8b03299
Wang, H., He, Z., Yang, Y., Zhang, J., Zhang, W., Zhang, W., et al. (2019a). Ratiometric fluorescence imaging of Golgi H2O2 reveals a correlation between Golgi oxidative stress and hypertension. Chem. Sci. 10 (47), 10876–10880. doi:10.1039/C9SC04384E
Wang, J., Jiang, C., Jin, J., Huang, L., Yu, W., Su, B., et al. (2021a). Ratiometric fluorescent lateral flow immunoassay for point-of-care testing of acute myocardial infarction. Angew. Chem. Int. Ed. 133 (23), 13152–13159. doi:10.1002/ange.202103458
Wang, P., Zhou, F., Zhang, C., Yin, S. Y., Teng, L., Chen, L., et al. (2018). Ultrathin two-dimensional covalent organic framework nanoprobe for interference-resistant two-photon fluorescence bioimaging. Chem. Sci. 9 (44), 8402–8408. doi:10.1039/C8SC03393E
Wang, X., Shi, W., Jin, Z., Huang, W., Lin, J., Ma, G., et al. (2017). Remarkable SERS activity observed from amorphous ZnO nanocages. Angew. Chem. Int. Ed. 129 (33), 9983–9987. doi:10.1002/ange.201705187
Wang, X., Shi, W., Wang, S., Zhao, H., Lin, J., Yang, Z., et al. (2019b). Two-dimensional amorphous TiO2 nanosheets enabling high-efficiency photoinduced charge transfer for excellent SERS activity. J. Am. Chem. Soc. 141 (14), 5856–5862. doi:10.1021/jacs.9b00029
Wang, Y., Zhang, M., Ma, H., Su, H., Li, A., Ruan, W., et al. (2021b). Surface plasmon resonance from Gallium-doped Zinc oxide nanoparticles and their electromagnetic enhancement contribution to surface-enhanced Raman scattering. ACS Appl. Mat. Interfaces 13 (29), 35038–35045. doi:10.1021/acsami.1c05804
Wdowiak, A. P., Duong, M. N., Joyce, R. D., Boyatzis, A. E., Walkey, M. C., Nealon, G. L., et al. (2021). Isotope-coded maleimide affinity tags for proteomics applications. Bioconjug. Chem. 32 (8), 1652–1666. doi:10.1021/acs.bioconjchem.1c00206
Wei, J., Liu, C., Wu, T., Zeng, W., Hu, B., Zhou, S., et al. (2022). A review of current status of ratiometric molecularly imprinted electrochemical sensors: from design to applications. Anal. Chim. Acta 1230, 340273. doi:10.1016/j.aca.2022.340273
Wu, L., Wu, T., Zeng, W., Zhou, S., Zhang, W., and Ma, J. (2023). A new ratiometric molecularly imprinted electrochemical sensor for the detection of Sunset Yellow based on gold nanoparticles. Food Chem. 413, 135600. doi:10.1016/j.foodchem.2023.135600
Xianyu, Y., Lin, Y., Chen, Q., Belessiotis-Richards, A., Stevens, M. M., and Thomas, M. R. (2021). Iodide-mediated rapid and sensitive surface etching of gold nanostars for biosensing. Angew. Chem. Int. Ed. 60 (18), 9891–9896. doi:10.1002/anie.202017317
Xie, C., Zhou, Y., Luo, K., Yang, Q., Tan, L., and Zhou, L. (2022a). Activated two-photon near-infrared ratiometric fluorescent nanoprobe for ONOO– detection and early diagnosis and assessment of liver injury. Anal. Chem. 94 (44), 15518–15524. doi:10.1021/acs.analchem.2c04032
Xie, L., Zeng, H., Zhu, J., Zhang, Z., Sun, H. B., Xia, W., et al. (2022b). State of the art in flexible SERS sensors toward label-free and onsite detection:from design to applications. Nano Res. 15 (5), 4374–4394. doi:10.1007/s12274-021-4017-4
Yang, J., Hu, Y., and Li, Y. (2019). Molecularly imprinted polymer-decorated signal on-off ratiometric electrochemical sensor for selective and robust dopamine detection. Biosens. Bioelectron. 135, 224–230. doi:10.1016/j.bios.2019.03.054
Ye, X., Luke, B., Andresson, T., and Blonder, J. (2009). 18O stable isotope labeling in MS-based proteomics. Brief. Funct. genomics proteomics 8 (2), 136–144. doi:10.1093/bfgp/eln055
Yi, Y., Zhang, D., Ma, Y., Wu, X., and Zhu, G. (2019). Dual-signal electrochemical enantiospecific recognition system via competitive supramolecular host–guest interactions: the case of phenylalanine. Anal. Chem. 91 (4), 2908–2915. doi:10.1021/acs.analchem.8b05047
Yue, S., Qiao, Z., Wang, X., and Bi, S. (2022). Enzyme-free catalyzed self-assembly of three-dimensional hyperbranched DNA structures for in situ SERS imaging and molecular logic operations. Chem. Eng. J. 446, 136838. doi:10.1016/j.cej.2022.136838
Zhai, S., Hu, W., Wang, W., Chai, L., An, Q., Li, C., et al. (2022). Tracking autophagy process with a through bond energy transfer-based ratiometric two-photon viscosity probe. Biosens. Bioelectron. 213, 114484. doi:10.1016/j.bios.2022.114484
Zhang, D., Wang, D., Xu, Z., Zhang, X., Yang, Y., Guo, J., et al. (2021). Diversiform sensors and sensing systems driven by triboelectric and piezoelectric nanogenerators. Coord. Chem. Rev. 427, 213597. doi:10.1016/j.ccr.2020.213597
Zhao, L., Li, T., Xu, X., Xu, Y., Li, D., Song, W., et al. (2023). Polyhedral Au nanoparticle/MoOx heterojunction-enhanced ultrasensitive dual-mode biosensor for miRNA detection combined with a nonenzymatic cascade DNA amplification circuit. Anal. Chem. 95 (24), 9271–9279. doi:10.1021/acs.analchem.3c01062
Zhao, M., Liu, D., Zhou, L., Wu, B., Tian, X., Zhang, Q., et al. (2018). Two water-soluble two-photon fluorescence probes for ratiometric imaging endogenous SO2 derivatives in mitochondria. Sens. Actuators B Chem. 255, 1228–1237. doi:10.1016/j.snb.2017.08.053
Zhao, X., Zhao, S., Song, Z. L., Zhang, X., Zhang, S., Song, W., et al. (2021). Alkyne functionalized graphene-isolated-Au-nanocrystal for the ratiometric SERS sensing of alkaline phosphatase with acetonitrile solvent as an internal standard. Sens. Actuators B Chem. 331, 129373. doi:10.1016/j.snb.2020.129373
Zhao, Y., Zhang, Q., Ma, L., Song, P., and Xia, L. (2020). A P/N type silicon semiconductor loaded with silver nanoparticles used as a SERS substrate to selectively drive the coupling reaction induced by surface plasmons. Nanoscale. Adv. 2 (8), 3460–3466. doi:10.1039/D0NA00350F
Zhong, Q., Zhang, R., Yang, B., Tian, T., Zhang, K., and Liu, B. (2022). A rational designed bioorthogonal surface-enhanced Raman scattering nanoprobe for quantitatively visualizing endogenous hydrogen sulfide in single living cells. ACS Sens. 7 (3), 893–899. doi:10.1021/acssensors.1c02711
Zhu, C., Wang, X., Yang, Y., Chen, L., and Yu, D. (2023). Research progress on ratiometric electrochemical sensing of mycotoxins. J. ElectroAnal. Chem. 929 (15), 117115. doi:10.1016/j.jelechem.2022.117115
Zhu, D., Liu, B., and Wei, G. (2021). Two-dimensional material-based colorimetric biosensors: A review. Biosensors 11 (8), 259. doi:10.3390/bios11080259
Keywords: ratiometric, fluorescence, Raman, electrochemical, mass spectrometry
Citation: Madhu M, Santhoshkumar S, Tseng W-B and Tseng W-L (2023) Maximizing analytical precision: exploring the advantages of ratiometric strategy in fluorescence, Raman, electrochemical, and mass spectrometry detection. Front. Anal. Sci. 3:1258558. doi: 10.3389/frans.2023.1258558
Received: 14 July 2023; Accepted: 04 September 2023;
Published: 25 September 2023.
Edited by:
Huan-Tsung Chang, Chang Gung University, TaiwanReviewed by:
Scott G. Harroun, Polytechnique Montréal, CanadaCopyright © 2023 Madhu, Santhoshkumar, Tseng and Tseng. This is an open-access article distributed under the terms of the Creative Commons Attribution License (CC BY). The use, distribution or reproduction in other forums is permitted, provided the original author(s) and the copyright owner(s) are credited and that the original publication in this journal is cited, in accordance with accepted academic practice. No use, distribution or reproduction is permitted which does not comply with these terms.
*Correspondence: Wei-Bin Tseng, dHNlbmd3YkBtYWlsLmR5dS5lZHUudHc=; Wei-Lung Tseng, dHNlbmd3bEBtYWlsLm5zeXN1LmVkdS50dw==
Disclaimer: All claims expressed in this article are solely those of the authors and do not necessarily represent those of their affiliated organizations, or those of the publisher, the editors and the reviewers. Any product that may be evaluated in this article or claim that may be made by its manufacturer is not guaranteed or endorsed by the publisher.
Research integrity at Frontiers
Learn more about the work of our research integrity team to safeguard the quality of each article we publish.